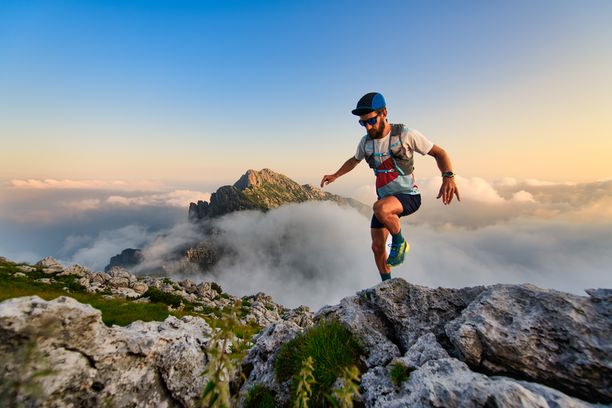
94% of researchers rate our articles as excellent or good
Learn more about the work of our research integrity team to safeguard the quality of each article we publish.
Find out more
ORIGINAL RESEARCH article
Front. Earth Sci., 04 March 2022
Sec. Structural Geology and Tectonics
Volume 10 - 2022 | https://doi.org/10.3389/feart.2022.824368
This article is part of the Research TopicContinental Basin and Orogenic Processes: Tectonic Deformation and Associated Landscape and Environmental EvolutionView all 15 articles
Downstream fining of riverbed gravels is generally linked with the processes of hydraulic sorting and abrasion. Hydraulic sorting is when larger gravel clasts stop moving in response to decreasing flow energy, whereas, finer grains will continue to be carried downstream. Furthermore, transportation of gravel clasts causes abrasion, bringing about a gradual decrease in grain size. Hydraulic sorting and abrasion have different dominant effects on the downstream fining of clasts in rivers with different climatic and tectonic backgrounds. At present, most studies focus on humid areas, and relatively few studies have explored this issue for the northeastern margin of the Tibetan Plateau in arid and semi-arid areas. Detailed investigations of the grain size, lithology, and roundness of riverbed gravels have been performed here along the Taolai, Hongshuiba, and Fengle Rivers, which flow across the northeastern margin of the Tibetan Plateau and debouch into the arid inland of North China. The obtained data were subsequently employed in a hydraulic pattern of grain-size distribution of riverbed gravels in this area, which is characterized by the combined influences of tectonic activity and climatic aridity. Analysis reveals that there is no new rock type appearing in the lithological compositions of riverbed gravels along these rivers, only showing fluctuations in proportions of lithology even though they are adjacent to uplifting mountains. Fresh gravel material from these mountains does indeed mix into the fluvial bedload, inducing a notable decrease in roundness in the Taolai and Hongshuiba bedloads downstream from here. The downstream fining of gravel along the three rivers, with median grain sizes above 128 mm and falling into the range from 20 to 128 mm, can probably be attributed to hydraulic sorting and abrasion. Further analysis suggests that the former presents a high correlation with channel gradient, which may be sustained by fault activity at the northeastern margin of the Tibetan Plateau. The grain-size distribution in these riverbed gravels thus provides insights into the evaluation of fluvial responses to active tectonic uplift.
The interaction between climate and tectonic activity, and their relative roles as driving mechanisms for fluvial processes, have long been topics for debate (e.g., Molnar and England 1990; Molnar 2003), attracting wide attention from geoscientists (e.g., Bridgland 2000; Westaway 2009; Wang et al., 2014). River system evolution is regarded as a result of these two factors in combination, and can record important evidence for their relative influence (e.g., Bridgland et al., 2012; Bridgland and Westaway 2014). In general, riverbed gravels from the same provenance appear to show a downstream decreasing tendency in grain size over tens to hundreds of kilometers (Parker 1991a). Hydraulic sorting occurs when larger gravel clasts stop moving as flow energy declines, whereas finer grains will continue to be carried downstream. Furthermore, transportation of clasts causes abrasion, bringing about a gradual downstream decrease in grain size, unless new material is added. The controlling factors and mechanisms of these two processes remain weakly understood (Rice 1999). A thorough assessment and distinction of their roles in fluvial sediment transportation can provide an excellent insight into river responses to allogenic controls (Hoey and Bluck 1999). Thus, in coarse sediments (>2 mm), downstream clast-size decrease can result from abrasion leading to actual size reduction (Kodama 1994; Humphrey 1997), or from selective transportation and deposition, which is the above-mentioned sorting process (Ferguson et al., 1996), or from their combined action. During the past few decades, the relative influence of these two factors in yielding a downstream decrease in grain size has, however, remained unclear and controversial (Bradley 1970; Shaw and Kellerhals 1982; Ashworth and Ferguson 1989; Parker 1991a; Parker 1991b; Paola et al., 1992; Mikos 1993; Huddard 1994; Ferguson et al., 1996). There is evidence that abrasion can be attributed to mechanical weathering of clasts through corrasion (Rengers and Wohl 2007) and that this is a leading mechanism in producing downstream fining on alluvial fans along many Japanese rivers, flowing through temperate humid climates and active tectonic zones (Kodama 1994). From mathematical analysis on the weight reduction of average clasts, Mikos (1993) also argued that abrasion appears to dominate. In the 1970’s and 1980’s, opinion swung strongly in favor of sorting, because observed downstream fining of fluvial bedload presented rates an order of magnitude higher than laboratory simulations using the same lithologies (e.g., Adams 1980). Further analysis reveals that selective sorting may be controlled primarily by the limited competence of hydraulic forces, which succeed in transporting small particles but fail to transport large particles (Rengers and Wohl 2007). Moreover, more recent advances suggest that this process is linked with certain fundamental geomorphic properties, such as concavity of stream profile (Rengers and Wohl 2007). As stream gradient decreases, flow competence in the transport of sediments also decreases (Gomez et al., 2001), thereby resulting in selective deposition of coarse particles, while fine particles continue in transport (Parker 1991b; Ferguson and Ashworth 1991; Hoey and Ferguson 1997; Ferguson et al., 1998; Gomez et al., 2001). Evidence from bed-load trap measurements and the dispersion of magnetic tracer pebbles also indicate that rapid downstream fining of clasts along a small river can probably be attributed to sorting rather than abrasion (Ferguson et al., 1996). This suggestion is in good agreement with the combined study of bed-load transport rates, grain-size distribution, shear stresses, and tracer pebble movements along three high-power gravel-bed rivers, in Scotland and Norway (Ashworth and Ferguson 1989).
In addition, quantitative field studies of the phenomenon are rendered difficult by a host of site-specific factors, such as hydrologic regime, tributaries bringing in clasts of different lithologies, base-level variation, and the scale over which fining is manifested (Cui et al., 1996). Researchers have thus resorted to techniques that allow the effects of various parameters to be isolated (e.g., Werritty and Florence 1990; Paola et al., 1992). Theoretical formulations (Sternberg 1875), observations of modern rivers (Bradley et al., 1972; Dawson 1988), flume studies (Elgueta-Astaburuaga and Hassan 2019), and numerical models (Hoey and Ferguson 1994; Cui et al., 1996) have significantly enhanced our understanding of sediment transport in mixed-grain-size river systems (Robinson and Slingerland 1998). The effectiveness of sorting, however, had been doubted as a result of empirical and theoretical work in the 1980’s, which suggested that entrainment from mixed-size gravel is almost or entirely unselective, due to hiding and protrusion effects (Andrews 1983; Wiberg and Smith 1987; Kirchner et al., 1990). Further analysis has indicated that high-stage gravel transport probably approaches equal mobility for different sizes, under the impact of these effects (Parker et al., 1982; Ashworth and Ferguson 1989; Wilcock and Southard 1989).
The above arguments have prompted a re-examination of whether the observed downstream fining of fluvial clasts can be generated through sorting alone. Despite its significance, the research on this issue remains inadequate; for example, the influencing factors of sorting have yet to be resolved. Moreover, sorting has been inferred without direct measurement of bed-load transport in the same river, and abrasion has been supported or rejected by comparing downstream fining in one river with abrasion tests of supposedly similar sediment from another river in hydraulic conditions of unknown applicability (Ferguson et al., 1996). At present, few studies have focused on this topic in areas characterized by the combination of arid or semi-arid climate and active tectonic processes. Below we thus examine the grain-size trends along the riverbeds of the Taolai, Hongshuiba and Fengle Rivers, together with their hydraulic parameters. These rivers flow from the Qilian Mountains and debouch into the arid interior of North China, which is generally considered as the northeast margin of the Tibetan Plateau. Gravels were measured in transects along these rivers, using pebble counts of median diameter, lithology, and clast rounding. It is the purpose of this paper to explore downstream distribution of grain sizes along the riverbed of these rivers and then further analyze the potential causes behind these trends.
The Beida River, with a length of ∼200 km, derives from the Tuolai and Tuolainan Shan, (Shan = Mountain in Chinese) located within the Southern Qilian Shan, and draining an area of ∼8,847 km2 (Wang 2019). From east to west, this catchment can be divided into the three sub-basins of the Fengle, Hongshuiba, and Taolai Rivers (Figure 1). All these tributaries are fed mainly by glacial meltwater, resulting in an approximately constant discharge (Yang 2008). The Taolai and Hongshuiba Rivers flow from the Qilian Shan, and then excavate separately through either side of the Wenshu Shan, converging finally at the southern front of the Jintanan Shan. In contrast, the Fengle River disappears as it flows from the Qilian Shan into the Gobi.
FIGURE 1. Geological map of the Beida River catchment. Its scale is 1:200,000, revised from the Gansu Geological Bureau (1967). Major faults, rivers, sub-catchments, and mountains have been presented within this catchment. These faults revised from Gansu Geological Bureau (1967). The inset map indicates the location of this catchment in the northeastern margin of the Tibetan Plateau.
The three rivers debouch northward into the arid interior of North China (Wu et al., 2007; Cai et al., 2012) and between the Qilian Shan and Jintanan Shan are characterized by braided gravel-bed floodplains. The bedrock lithology of the Taolai River catchment mainly comprises limestone, quartzite, schist, gneiss, sandstone, and granite, while the Hongshuiba catchment is dominated by sandstone, gneiss, quartzite, and granite. In comparison with above two sub-catchments, the distribution of bedrock types across the Fengle River catchment points to a relatively simple composition, consisting of granite, sandstone, limestone, and diabase (Figure 1). The sediments that have accumulated within the catchments of these three rivers are all fluvial clasts eroded from the Qilian Shan during the Quaternary (Zhang et al., 2020). Moreover, the Wenshu Shan, located between the Qilian Shan and the Jintanan Shan, is mainly composed of conglomerate, presenting a similar lithologic composition in comparison with the catchments of the Taolai and Hongshuiba Rivers (Zhao et al., 2001).
The Beida River catchment is transected by a number of reversed faults, i.e., the Yumen–Beida River fault, the Fodongmiao–Hongyazi fault, the Jiayuguan fault, the Wenshu Shan fault, and the Jintanan Shan fault, which are clearly exposed along the fronts of the Qilian Shan, the Wenshu Shan, and the Jintanan Shan (Figure 1). Fault scarps can be readily observed and traced for hundreds of kilometers along these faults, indicating that tectonic activity around these mountains has remained vigorous during the Quaternary (Song 2006). The northern front of the Qilian Shan is constrained by the NW–SE trending Yumen–Beida River fault and Fodongmiao–Hongyazi fault, generating striking relief. In addition, the Wenshu Shan, situated between the Jintanan Shan and Qilian Shan, is bounded by the Jiayuguan fault to the north and Wenshu Shan fault to the south. These two NW–SE-trending reverse faults have led to the uplift of the Wenshu Shan in an anticlinal pattern (Song 2006; Zheng 2009). Owing to their activity and the resultant upward growth of the Qilian Shan and Wenshu Shan, the surface between the two mountain ranges has been uplifted strikingly, forcing the Taolai and Hongshuiba Rivers to incise deeply (Figures 2A,C). In contrast, as the two rivers flow northwards far away from the Wenshu Shan, their valleys all show a wide and shallow form (Figures 2B,D).
FIGURE 2. Field photos from the Beida River catchment. (A) and (B) Two kinds of Taolai River channels. (C) and (D) View of the Hongshuiba River channel characterized by deep incision and low relief, respectively. (E) and (F) the Fengle River channel. (G) and (H) Ephemeral channels overlying the interfluves between the Taolai, Hongshuiba, and Fengle Rivers. (I) and (J) Sample site illustrating investigation methods in the field. (K) and (L) Field measurement of riverbed width. (M)–(P) Field measurement of riverbed depth.
Fieldwork along the channels of the Taolai, Hongshuiba, and Fengle Rivers mainly comprised the measurement of hydraulic parameters (width and depth), grain size, lithology, and roundness. Owning to the deep downcutting by these rivers between the Qilian Shan and the Wenshu Shan (Figures 2A,C), access to their valleys is rather difficult. These sites, which can be entered by foot into riverbed, thus have been chosen for investigations. Thereafter, measurement sites are more widely spaced downstream. This was partly a reflection of the absence of suitable bars to sample and partly because of the local removal of coarse bed material from the riverbed for local construction purposes. Respectively thirteen, eleven, and seven sites have been used for measurement work along the Taolai, Hongshuiba, and Fengle Rivers (Table 1). Furthermore, in view of potential input of material from slopes, twelve measurement sites on the interfluves between these rivers, marked A–L (Figure 3A), were also been chosen for investigation. These investigation sites along the three rivers are numbered in downstream sequence (Figure 3A), and their downstream distances from the Qilian Shan are employed to mark the location along the river courses. These sites chosen for measurement are generally located within the modern active riverbed, free from artificial disturbance to bed material and thick vegetation cover of the bar surface. River width here refers to the channel width, which was measured using a TruePluse 360 laser rangefinder. Meanwhile, the river depth was measured at 3–5 points along each riverbed cross section to obtain an averaged depth. The riverbed elevation of different reaches was extracted from a DEM with 30-m resolution, yielding the longitudinal profiles of the three rivers. The average riverbed gradient (S) within 2 km upstream and downstream of the measuring points was taken as the riverbed gradient at that point.
FIGURE 3. Statistical data on bedload gravel lithology. (A) The Beida River catchment. All sampling sites and correspondent lithological composition of bedload gravels have been numbered in downstream sequence along its three tributaries, the Taolai, Hongshuiba, and Fengle Rivers. Furthermore, a total of 12 samples taken from the watersheds between these tributaries has been marked from A to L. Fine white lines indicate watershed gullies. (B), (C) and (D) Downstream variations in lithological proportion of the bedload gravels along the Taolai Hongshuiba, and Fengle Rivers, respectively. All the sampling sites are marked by number sequence which is coincident with (A), the shaded area in (B) and (C) indicates that the rivers flow through the Wenshu Shan.
The grain size of the sediment was established using sieving (Mosley and Tindale 1985). Within each square, all riverbed clasts were collected to a depth of ∼10 cm, although if the largest clasts exceeded this measurement then the sample depth was increased. Collected clasts were then separated into different grain-size groups by sieving (Figures 2I,J). They were further weighed in the field. Particles with a grain size of 0–2 mm are recorded as sand, while > 2-mm clasts are classified as gravel. The gravel was then divided into the following grain-size groups (unit: mm): 2.0–2.5, 2.5–5, 5–10, 10–20, 20–32, 32–64, 64–128, 128–256, 256–512, and 512–1,024. At each site, a total of 200 gravel clasts were investigated to determine their intermediate axis, lithology, and roundness, based on the random measurement method (Stock et al., 2008).
In order to make a consistent comparison, the median clast diameter of each size group has been transformed into φ by the following equation:
in which D represents the median clast diameter of each size group,
The percentage weight of each grain-size group relative to the total riverbed clasts at a site constitutes a frequency distribution curve, which can be further translated into a cumulative frequency curve. The gravel median diameter corresponding to 5, 16, 50, 84, and 95%, in this cumulative frequency curve then have been employed as characteristic particle sizes (Krumbein 1934; Chen et al., 2018), marked by D5, D16, D50, D84, and D95, respectively. Moreover, based on φ, the sorting property of gravels accumulated at one site can be readily evaluated by the standard deviation of their grain size, which is generally marked as σ, with larger values signifying poorer sorting. According to Folk and Ward (1957), this can be calculated using the formula:
in which φ95 = −log2D5, φ84 = −log2D16, φ16 = −log2D84, and φ5 = −log2D95.
τc here is defined as the critical shear stress, which is required for the initial movement of fluvial clasts, while τb is used to describe the riverbed shear stress. They can be obtained from the Shields constant equations (Meyer-Peter and Muller 1948):
Where,
In which
Based on the rounding-classification standard of Krumbein (1941a), bedload gravels at each site have been classified as 1 = very angular, 2 = angular, 3 = sub angular, 4 = sub rounded, 5 = rounded and 6 = well rounded in the field (Powers 1953), and then their proportions can be calculated in terms of an individual number. An average roundness value (R) is employed here to further describe the rounding characteristics of the gravels at each site, which is defined as the sum of these proportions (Li et al., 2014), with higher value indicating better rounding and vice versa.
These results of the above analyses have been calculated and tabulated in Table 1. In order to elucidate transportation process of the bedload gravels along the Taolai, Hongshuiba, and Fengle Rivers, their lithology, roundness, and grain size, in combination with the calculated indices, have been analyzed in detail.
Apart from twelve sampling sites located on the watersheds between the tributaries of the Beida River, a total of thirteen, eleven, and seven sites has been investigated along its tributaries: the Taolai, Hongshuiba, and Fengle Rivers, respectively (Figure 3A). The lithological composition of the gravels accumulated at the thirteen locations along the Taolai River shows that the proportion of phyllite decreases rapidly downstream from ∼20 to 2%, within 10 km from the Qilian Shan. In contrast, the proportion of quartzite gradually increases from ∼10 to 20% over the same reach (Figure 3B). Near the Wenshu Shan, fluctuation of lithological proportions, however, occurs again, with both phyllite and limestone increasing slightly, and then declining to a stable level, while quartzite and sandstone appear to increase progressively. According to Zhao et al. (2001), the lithostratigraphy over the Wenshu Shan is mainly conglomerate and characterized by sandstone and quartzite, so this fluctuation may be attributable to clast input to the Taolai River bedload from these mountains. In the distribution of bedload gravel lithology at the eleven sampling sites along the Hongshuiba River, the proportions of diabase and phyllite both decrease rapidly downstream from ∼5 to ∼5%–∼1% within 10 km from the Qilian Shan and then turn to a stable tendency (Figure 3C). In contrast, the proportion of quartzite increase remarkably from ∼3% up to 22%. In the vicinity of the Wenshu Shan, the proportion of schist in comparison with other lithologies presents a great fluctuation, which can probably be correlated with the high proportion of schist occurring on the watershed between the Taolai and Hongshuiba Rivers (Figure 3A), given that the Wenshu Shan is composed of sandstone and quartzite (Zhao et al., 2001). This lithological fluctuation therefore demonstrates the input of material to the Hongshuiba bedload from small gullies over the watershed (Figure 3A). Along the Fengle River, granite decreases from ∼74 to 40%, while sandstone increases to reach a relatively steady proportion of ∼20% (Figure 3D) and other lithologies tend towards stability. Some sampling sites within gullies on the watershed between the Hongshuiba and Fengle Rivers show a high sandstone percentage (Figure 3A), suggesting a possible clast contribution to the Fengle River bedload.
Along the Taolai, Hongshuiba, and Fengle Rivers, the results of bedload gravel roundness analysis for different lithologies and grain sizes are presented in Figure 4. As the Taolai River flows away from the Qilian Shan, the variation in its bedload gravel roundness falls into a complex pattern in which it first decreases and then increases, twice over, forming two troughs (Figures 4B,C). Based on field investigation, they are in good correspondence with two reaches affected by the clast input from the Wenshu Shan and human activities (Table 2). Published data have revealed that gravel roundness of clasts formed of the conglomerate constructing the Wenshu Shan is indeed rather low (Zhao et al., 2001). The course of lower Taolai River has been restricted within an artificial channel, leading to original riverbed exposure (Figure 4A). Frost weathering seems to be vigorous here (Chen et al., 2019) causing the gravels accumulated on the dry riverbed to shatter. The bedload gravel roundness for different lithologies and grain sizes in the two reaches perhaps respond to the two styles of influence and yield two correspondent troughs. The downstream variation in bedload gravel roundness along the Hongshuiba River for different lithologies and grain sizes also shows two decreasing tendencies, which are against our expectations (Figures 4D,E). The statistical data on bedload gravel lithology has suggested that the small gullies on the watershed between the Taolai and Hongshuiba Rivers have a steady material contribution to the latter bedload (Figure 3A). Due to artificial reconstruction on the Taolai River, the whole riverbed of the lower Hongshuiba is now also exposed and suffers frost weathering. In view of this good spatial correlation, the two decreasing tendencies in bedload gravel roundness along the Hongshuiba River, therefore, can probably be linked with the two recognized influences: frost weathering and artificial reconstruction. In contrast, the downstream variation in bedload gravel roundness along the Fengle River for different lithologies and grain sizes shows a gradually increasing pattern (Figures 4F,G) which conforms to the universal rule (Krumbein 1941b).
FIGURE 4. The downstream variation in bedload gravel roundness along the Taolai, Hongshuiba, and Fengle Rivers. (A) The Beida River catchment. It can be divided into the three sub-catchments of the Taolai, Hongshuiba, and Fengle Rivers, in which all sampling sites are marked and numbered in downstream sequence. Dashed lines along the courses of the Taolai and Hongshuiba Rivers indicate their dry riverbeds. (B)–(G) The downstream variation in bedload gravel roundness for different gravel sizes and lithologies, respectively, along the Taolai, Hongshuiba, and Fengle Rivers. Their sampling sites are numbered in correspondence with (A).
TABLE 2. Results of roundness for the clasts eroded from the interfluves between the Taolai, Hongshuiba, and Fengle Rivers.
In general, the three indices D84, D50, and D16 can be employed as indicators to evaluate the downstream decrease in grain size of bedload gravel (Hoey and Bluck 1999). Along the Taolai, Hongshuiba, and Fengle Rivers, the three indices all show an obvious downstream diminishing tendency, amongst which D84 presents the widest variation range (Table 1). Further analysis reveals that the downstream decrease of D84 and D50 follows a more precise exponential fitting pattern compared with D16 (Figure 5). Their fit coefficients are all rather high, reaching up to >0.75 along the three rivers (Figures 5B–D). This variation pattern is in good agreement with the general rule for gravel-size distribution along riverbeds suggested by Sternberg (1875). Despite a gentle decrease tendency in the downstream direction for D16, its major decline can be clearly observed to occur in the 30-km reaches of the longer Taolai and Hongshuiba Rivers, progressively downstream from the Qilian Shan, in correspondence with D84 and D50. The grain-size frequency distribution curves of bedload gravel along the Taolai and Hongshuiba Rivers show a transformation from bimodal to unimodal pattern, with the peak progressively moving to finer particle sizes in a downstream direction (Figure 6). Meanwhile, they appear to become narrower and combined with decreasing standard deviation σ (Table 1), indicating that the bedload gravel tends towards an enhanced sorting property in the downstream direction. In contrast, the grain-size frequency distribution curves of bedload gravel and standard deviation along the short Fengle River seem to show negligible variation. Moreover, the sand contents of riverbed clast composition are almost constant along the three rivers.
FIGURE 5. Variation pattern of D84, D50, and D16 toward downstream. (A) The Beida River catchment. (B), (C) and (D) Downstream variation in the three indexes along the Taolai, Hongshuiba, and Fengle Rivers, respectively. Their variations all follow an exponential fitting pattern, in which the fitting correlation coefficients for D84 and D50 can reach up to >0.75. All the sampling sites are marked by number sequence which is coincident with (A).
FIGURE 6. Grain-size frequency distribution curves of bedload gravel along the Taolai, Hongshuiba, and Fengle Rivers. The sampling sites are numbered in downstream sequence and in correspondence with the inset map.
The investigation results for bedload gravel lithology and roundness suggest that the clasts eroded from the interfluves between the Taolai, Hongshuiba, and Fengle Rivers can indeed be mixed into their riverbeds (Figure 3A; Table 2). Despite a notable fluctuation in lithological percentage due to this intrusion, the lithological composition of the bedload gravels presents a stable pattern along the three rivers (Figure 3), implying that the fluvial clasts transported through the northeastern margin of the Tibetan Plateau, characterized by active tectonism and dry climate, appear to represent material that has been recycled from earlier deposits (Zhang et al., 2020). Moreover, clast influx here perhaps has little effect on bedload gravel transportation. The downstream decrease in grain size in these bedload gravels thus also follows a good exponential fitting pattern (Figure 5), in good agreement with the general rule for gravel size distribution along riverbeds suggested by Sternberg (1875). Over the past century, numerous studies have attributed the downstream fining of bedload gravel to hydraulic sorting and abrasion (Mackin 1948; Rice 1998; Rice and Church 1998; Gomez et al., 2001; Rice and Church 2001; Sklar et al., 2006). Nevertheless, a debate on the dominant role in both of these can still be enlarged (e.g., Menting et al., 2015). According to the grain-size distribution of the bedload gravels along the three rivers, downstream fining has indeed been demonstrated by the work reported in this paper.
Along the Taolai, Hongshuiba, and Fengle Rivers, the reaches that are free of clast influx and human activities all present a tendency for bedload gravel roundness to increase downstream (Figure 4), linked to the contribution of abrasion to the downstream fining of bedload gravel. However, the sand content amongst bedload clasts seems not to increase due to abrasion, but tends to be almost constant along the three rivers (Table 1). This result suggests that the downstream fining of bedload gravel in this region at the northeastern margin of the Tibetan Plateau can also probably be attributed to hydraulic sorting, in addition to abrasion. In recent years, more studies have focused upon this influence, in which D84 has been argued to decrease in direct proportion to the decline of riverbed shear stress τb (Dunne and Jerolmack 2018). Our data however reveal that some sampling sites along the three rivers do not comply with this relationship (Figure 7). In this way, it seems that hydraulic sorting cannot be regarded as the only explanation for downstream fining of bedload gravel in the study area. Further analysis therefore pays attention to the variation in percentages of grain-size groups for different lithologies. Along the Taolai, Hongshuiba, and Fengle Rivers, the downstream reduction of percentages of grain-size groups >128 mm seems not to be seen in adjacent and smaller grain-size groups, in which the reverse occurs: percentages increase downstream (Figure 8). Based on this variation pattern, the bedload gravels transported by the three rivers can be divided into two parts, with grain sizes >128 mm and <128 mm, for which downstream fining can be attributed to hydraulic sorting and abrasion, respectively.
FIGURE 7. Positive correlation checks between D84 and τb along the Taolai, Hongshuiba, and Fengle Rivers.
FIGURE 8. Variation in the percentages of grain-size groups with different lithologies along the Taolai, Hongshuiba, and Fengle Rivers. The bedload gravels collected from every sampling site were classified according to their lithologies. For each lithology, the variation in the percentages of grain-size groups along the three rivers was then analyzed. (A), (B), and (C) Downstream variations in the percentages of grain-size groups with different lithologies, along the Taolai, Hongshuiba, and Fengle Rivers, respectively. The reduced percentages of grain-size groups >128 mm toward downstream are not seen in the smaller grain-size groups. In contrast, for the groups <128 mm, the reverse applies.
In general, hydraulic sorting ability in rivers is dependent on “hiding effect” and hydrodynamic force (Robinson and Slingerland 1998). Under certain hydraulic conditions, coarse particles in fluvial bedload can be moved more readily by flowing water due to their greater exposure, whereas fine particles in contrast are generally hidden (Einstein 1950; Paola et al., 1992). This phenomenon, which has been defined by Diplas (1987) as “hiding effect”, can reduce the difference in τc, leading to an almost simultaneous movement for clasts of different grain-size (Parker et al., 1982). Further numerical simulation by Robinson and Slingerland (1998) has revealed how this effect inhibits progressive downstream hydraulic sorting. In order to evaluate this effect, Andrews (1983) has provided an empirical correlation, in which the mass ratio of D to D50 falls into a range between 0.3 and 4.2, implying that the correspondent grain-size group perhaps suffers from a closely similar critical shear stress. Following Andrews, we obtained, at each site, the ratio of the mass of those grain-size groups meeting this standard to the total clasts. Along the Taolai, Hongshuiba, and Fengle Rivers, these ratios show only a gentle fluctuation (Figure 9), indicating little impact on hydraulic sorting from this “hiding effect” (Robinson and Slingerland 1998).
FIGURE 9. Downstream variation in the ratio of those grain-size groups with D/D50 in the range between 0.3 and 4.2 to all the clasts collected at each site. Different symbols are used for the three study rivers. It can be clearly seen that this ratio is maintained in the narrow range 0.66–0.82 moving downstream.
Since the “hiding effect” has been excluded, hydraulic sorting in the study area can only be linked with hydrodynamic force. Numerical simulation has suggested that it will fluctuate within the variation range of τc, if the ratio of τb to τc is lower than 20, and favoring deposition of the coarse clasts with τc>τb (Robinson and Slingerland 1998). Therefore, this ratio is employed here to evaluate the influence of hydrodynamic force on hydraulic sorting. The obtained results show that the ratios of τb to τc at every sampling site are <20 (Figure 10), implying an inadequate deviation between hydrodynamic force and the critical shear stress τc that would be required for the initial movement of fluvial clasts (Robinson and Slingerland 1998); thus sorting seems to be an important factor in causing downstream fining in the study area.
FIGURE 10. Downstream variation in the ratio of τb to τc at each sampling site. All the ratios obtained are <20.
During the past century, numerous works have focused on fluvial clasts to obtain some general variation patterns of downstream changes in their roundness and grain size, based on a series of flume experiments (Krumbein 1941b; Paola et al., 1992; Elgueta-Astaburuaga and Hassan 2019). Further numerical simulations have subsequently established some driving mechanisms for these patterns (Hoey and Ferguson 1994; Cui et al., 1996), in which downstream fining of bedload gravel has often been attributed to hydraulic sorting and abrasion (Parker 1991a; Paola et al., 1992, Hoey and Bluck 1999; Wilcock and Kenworthy 2002; Rice 2005). However, there is still no consensus on the factors that control their function in river systems. In this paper, hydraulic sorting has been linked with hydrodynamic force, leading to downstream fining of bedload gravels with grain size >128 mm. According to Schumm (1977), riverbed gradient and discharge have been regarded as potential controlling factors on hydrodynamic force. In recent studies, these are also confirmed to be able to drive variation in hydrodynamic force along riverbeds (Gilet et al., 2020). The Taolai, Hongshuiba, and Fengle Rivers originate from the Qilian Shan, and then debouch northward into the arid interior of North China (Figure 1). Owing to glacial meltwater supply, they seem to have had almost stable discharge over timescales of years (Yang 2008), which has been proved from hydraulic monitoring data (http://www.ncdc.ac.cn). The prominent hydraulic sorting as a mechanism for downstream fining of bedload gravels >128 mm in the three rivers can, therefore, probably only be correlated with riverbed gradient, rather than discharge.
In view of the excellent match between the 30-m-resolution DEM and the landscape across the northeastern margin of the Tibetan Plateau (Pan et al., 2015), it has been possible to extract the riverbed elevations of the Taolai, Hongshuiba, and Fengle from these data, in which high values show close correspondence to the occurrence of thrust faults (Figure 11). The smoothing method of the extracted longitudinal profiles refers to Schwanghart and Scherler 2017. At sampling sites with bedload gravels >128 mm, the second derivatives of the obtained three curves were then calculated as a measurement for their gradient variations. Correlation analysis between the percentages of grain-size groups >128 mm and gradient variations at these sites shows a notable positive relationship (Figure 11). Since the ratios of τb to τc at every sampling site are all <20 (Figure 10), the adjustment in hydrodynamic force due to gradient variation along the three rivers can probably explain the deposition of coarse gravels >128 mm, creating a hydraulic sorting effect. For gravel beds, intrinsic fluvial behaviors can however provide timely adjustment to offset the variation in riverbed gradient by means of deposition and/or incision (Thayer et al., 2016). Thus, the persistence of perturbations in downstream gradient along the study rivers perhaps requires the continued influence of tectonic activity, such as fault movements. The three rivers excavating through the tectonically active northeastern margin of the Tibetan Plateau are transected by the Yumen-Beida River fault and Fodongmiao-Hongyazi fault (Figure 1). Previous work has constrained the vertical uplift rates along these reverse faults to be 0.7–1.2 mm/a, since the Late Pleistocene (Liu 2017; Yang et al., 2018; Hetzel et al., 2019), which is consistent with the present GPS data (Zhao et al., 2015; Hetzel et al., 2019). The persistent variation in riverbed gradient along the three rivers can probably be attributed to tectonic uplift generated by these faults (Yang et al., 2018; Hetzel et al., 2019; Wang et al., 2020). The link between grain-size distribution in riverbed gravels and tectonic uplift thus seems to be confirmed in the study area. In fact, grain-size distribution in riverbed gravel has also been employed as an indicator for tectonic activity in the Central Alps, northern frontal Himalayas, around Taiwan and the Mediterranean (Attal and Lavé 2006; Whittaker et al., 2010; Wang et al., 2015; Gilet et al., 2020). The percentages of grain-size groups >128 mm in the riverbed gravels along the three study-area rivers can thus be understood as an evaluator of the fluvial response to tectonic uplift.
FIGURE 11. Correlation analysis between the percentages of grain-size groups >128 mm and gradient variations along the Taolai, Hongshuiba, and Fengle Rivers. (A) Riverbed elevations along the three rivers. These are extracted from a 30-m-resolution DEM, in which high values correspond closely with thrust fault occurrences. At the sampling sites with some grain-size groups >128 mm, the second derivatives of the three curves were then calculated as a measurement for gradient variations. (B) Positive correlation between the obtained variations in riverbed gradient and the percentages of grain-size groups >128 mm. There seems to be an excellent exponential relationship along the three rivers.
The Taolai, Hongshuiba, and Fengle Rivers, as the three sub-catchments of the Beida River system, cut through the northeastern margin of the Tibetan Plateau. Despite a notable fluctuation in bedload lithologies, caused by clast input from neighboring uplands, the composition of their bedload gravels shows a stable downstream trend, implying transportational recycling of fluvial clasts through a region that is characterized by active tectonism and dry climate. Detailed investigation and analysis of grain-size distribution suggest that downstream fining of bedload gravel in these rivers follows the general rule, within which the downstream fining of the gravels with grain size >128 mm has been attributed in these study-area rivers to hydraulic sorting. Moreover, the variation in riverbed gradient of the three rivers, explained as a driving force for hydraulic sorting, may be linked with active tectonism. The percentages of grain-size groups >128 mm in the riverbed clasts along the three rivers, therefore, can be understood as an evaluator of the fluvial response to tectonic uplift in the northeastern margin of the Tibetan Plateau.
The original contributions presented in the study are included in the article/Supplementary Material, further inquiries can be directed to the corresponding authors.
ZD completed the writing and editing to the article. ZH designed the research and improve this manuscript. ZH, DB, BP, and DC focused on the scientific issues involved in the article. ZD and QM finished the data collection in the field. ML, XL, YY, and DC performed the data analyses and then prepared the figures and tables. All authors contributed to the article and approved the submitted version.
This research is financially supported by the National Natural Science Foundation of China (Grant no. 41730637, 42041006, and 41871001) and 111 Program from State Administration of Foreign Experts Affairs and Ministry of Education of the People’s Republic of China (Grant no. BP2018001).
The authors declare that the research was conducted in the absence of any commercial or financial relationships that could be construed as a potential conflict of interest.
All claims expressed in this article are solely those of the authors and do not necessarily represent those of their affiliated organizations, or those of the publisher, the editors and the reviewers. Any product that may be evaluated in this article, or claim that may be made by its manufacturer, is not guaranteed or endorsed by the publisher.
We really appreciate the driver Shiping Wei for helping us to collect data in the field. This paper has benefited from valuable suggestions and data analysis by Yanan Zhang, Weijin Guan and Jiakun Wu.
Adams, J. (1980). Contemporary Uplift and Erosion of the Southern Alps, New Zealand: Summary. Geol. Soc. America Bull. 91 (1), 2–4. doi:10.1130/0016-7606(1980)91<2:cuaeot>2.0.co;2
Andrews, E. D. (1983). Entrainment of Gravel from Naturally Sorted Riverbed Material. Geol. Soc. America Bull. 94, 1225–1231. doi:10.1130/0016-7606(1983)94<1225:eogfns>2.0.co;2
Ashworth, P. J., and Ferguson, R. I. (1989). Size-selective Entrainment of Bed Load in Gravel Bed Streams. Water Resour. Res. 25, 627–634. doi:10.1029/WR025i004p00627
Attal, M., and Lavé, J. (2006). Changes of Bedload Characteristics along the Marsyandi River (central Nepal): Implications for Understanding Hillslope Sediment Supply, Sediment Load Evolution along Fluvial Networks, and Denudation in Active Orogenic Belts. Geol. Soc. America Spec. Paper 398, 143–171. doi:10.1130/2006.2398(09
Bradley, W. C. (1970). Effect of Weathering on Abrasion of Granitic Gravel, Colorado River (Texas). Geol. Soc. America Bull. 81, 61–80. doi:10.1130/0016-7606(1970)81[61:eowoao]2.0.co;2
Bradley, W. C., Fahnestock, R. K., and Rowekamp, E. T. (1972). Coarse Sediment Transport by Flood Flows on Knik River, Alaska. Geol. Soc. America Bull. 83, 1261–1284. doi:10.1130/0016-7606(1972)83[1261:cstbff]2.0.co;2
Bridgland, D. R. (2000). River Terrace Systems in north-west Europe: an Archive of Environmental Change, Uplift and Early Human Occupation. Quat. Sci. Rev. 19 (13), 1293–1303. doi:10.1016/S0277-3791(99)00095-5
Bridgland, D. R., and Westaway, R. (2014). Quaternary Fluvial Archives and Landscape Evolution: a Global Synthesis. Proc. Geologists' Assoc. 125 (5), 600–629. doi:10.1016/j.pgeola.2014.10.009
Bridgland, D. R., Westaway, R., Romieh, M. A., Candy, I., Daoud, M., Demir, T., et al. (2012). The River Orontes in Syria and Turkey: Downstream Variation of Fluvial Archives in Different Crustal Blocks. Geomorphology 165-166, 25–49. doi:10.1016/j.geomorph.2012.01.011
Cai, M., Fang, X., Wu, F., Miao, Y., and Appel, E. (2012). Pliocene-Pleistocene Stepwise Drying of Central Asia: Evidence from Paleomagnetism and Sporopollen Record of the Deep Borehole SG-3 in the Western Qaidam Basin, NE Tibetan Plateau. Glob. Planet. Change 94-95, 72–81. doi:10.1016/j.gloplacha.2012.07.002
Chen, D. B., Chen, J. J., Hu, X. F., Su, H., Chen, Y., and Zhang, J. (2018). Characteristics and Analysis on the Sediment Grain Size along the Liyuan River on the North Piedmont of the Qilian Shan and its Geological Significance. Quat. Sci. 38 (6), 1336–1347. doi:10.11928/j.issn.1001-7410.2018.06.02
Chen, L. P., Geng, H. P., Zhang, J., Zhao, Q. M., and Pan, B. T. (2019). Spatial Distribution of Bedrock Rebound Value (Schmidt Hammer) across the Heihe River basin and its Implication. J. Glaciology Geocryology 41 (2), 364–373. doi:10.7522/j.issn.1000-0240.2019.0302
Cui, Y., Paola, C., and Parker, G. (1996). Numerical Simulation of Aggradation and Downstream Fining. J. Hydraulic Res. 34 (2), 185–204. doi:10.1080/00221689609498496
Huddart, D. (1994). Rock-type Controls on Downstream Changes in Clast Parameters in Sandur Systems in Southeast Iceland. Sepm Jsr 64A (2a), 215–225. doi:10.1306/D4267D61-2B26-11D7-8648000102C1865D
Dawson, M. (1988). Sediment Size Variation in a Braided Reach of the Sunwapta River, Alberta, Canada. Earth Surf. Process. Landforms 13, 599–618. doi:10.1002/esp.3290130705
Diplas, P. (1987). Bedload Transport in Gravel-Bed Streams. J. Hydraulic Eng. 113 (11), 277–292. doi:10.1061/(asce)0733-9429(1987)113:3(277)
Dunne, K. B. J., and Jerolmack, D. J. (2018). Evidence of, and a Proposed Explanation for, Bimodal Transport States in Alluvial Rivers. Earth Surf. Dynam. 6 (3), 583–594. doi:10.5194/esurf-6-583-2018
Einstein, H. A. (1950). The Bed-Load Function for Sediment Transport in Open Channel flowsTechnical Bulletin. U.S. Department Agric. Soil Conservation Serv. 1026 (9), 1–89.
Elgueta-Astaburuaga, M. A., and Hassan, M. A. (2019). Sediment Storage, Partial Transport, and the Evolution of an Experimental Gravel Bed under Changing Sediment Supply Regimes. Geomorphology 330, 1–12. doi:10.1016/j.geomorph.2018.12.018
Ferguson, R., and Ashworth, P. (1991). Slope-induced Changes in Channel Character along a Gravel-Bed Stream: the Allt Dubhaig, Scotland. Earth Surf. Process. Landforms 16 (1), 65–82. doi:10.1002/esp.3290160108
Ferguson, R., Hoey, T., Wathen, S., and Werritty, A. (1996). Field Evidence for Rapid Downstream Fining of River Gravels through Selective Transport. Geol 24 (2), 179–182. doi:10.1130/0091-7613(1996)024<0179:fefrdf>2.3.co;2
Ferguson, R. I., Hoey, T. B., Wathen, S. J., Werrity, A., Hardwick, R. I., and Sambrook-Smith, G. H. (1998). “Downstream Fining of River Gravels: Integrated Field, Laboratory and Modeling Study,” in Gravel-Bed Rivers in the Environment. Editors Klingeman, P. C., Beschta, R. L., Komar, P. D., and Bradley, J. B. (Highlands Ranch, CO: Water Resources Publications), 85–114.
Folk, R. L., and Ward, W. C. (1957). Brazos River Bar [Texas]; a Study in the Significance of Grain Size Parameters. J. Sediment. Res. 27 (1), 3–26. doi:10.1306/74D70646-2B21-11D7-8648000102C1865D
Gilet, L., Gob, F., Gautier, E., Houbrechts, G., Virmoux, C., and Thommeret, N. (2020). Hydro-morphometric Parameters Controlling Travel Distance of Pebbles and Cobbles in Three Gravel Bed Streams. Geomorphology 358, 107117. doi:10.1016/j.geomorph.2020.107117
Gomez, B., Rosser, B. J., Peacock, D. H., Hicks, D. M., and Palmer, J. A. (2001). Downstream Fining in a Rapidly Aggrading Gravel Bed River. Water Resour. Res. 37 (6), 1813–1823. doi:10.1029/2001WR900007
Hetzel, R., Hampel, A., Gebbeken, P., Xu, Q., and Gold, R. D. (2019). A Constant Slip Rate for the Western Qilian Shan Frontal Thrust during the Last 200 Ka Consistent with GPS-Derived and Geological Shortening Rates. Earth Planet. Sci. Lett. 509, 100–113. doi:10.1016/j.epsl.2018.12.032
Hoey, T. B., and Bluck, B. J. (1999). Identifying the Controls over Downstream Fining of River Gravels. J. Sediment. Res. 69 (1), 40–50. doi:10.2110/jsr.69.40
Hoey, T. B., and Ferguson, R. I. (1997). Controls of Strength and Rate of Downstream Fining above a River Base Level. Water Resour. Res. 33 (11), 2601–2608. doi:10.1029/97WR02324
Hoey, T. B., and Ferguson, R. (1994). Numerical Simulation of Downstream Fining by Selective Transport in Gravel Bed Rivers: Model Development and Illustration. Water Resour. Res. 30, 2251–2260. doi:10.1029/94WR00556
Hoover Mackin, J. (1948). Concept of the Graded River. Geol. Soc. America Bull. 59, 463–512. doi:10.1130/0016-7606(1948)59[463:cotgr]2.0.co;2
Humphrey, L. (1997). Weathering-controlled Abrasion in a Coarse-Grained, Meandering Reach of the rio grande: Implications for the Rock Record. GSA Bull. 109 (9), 1080–1088. doi:10.1130/0016-7606(1997)109<1080:WCAIAC>2.3.CO;2
Kirchner, J. W., Dietrich, W. E., Iseya, F., and Ikeda, H. (1990). The Variability of Critical Shear Stress, Friction Angle, and Grain Protrusion in Water-Worked Sediments. Sedimentology 37, 647–672. doi:10.1111/j.1365-3091.1990.tb00627.x
Kodama, Y. (1994). Experimental Study of Abrasion and its Role in Producing Downstream Fining in Gravel-Bed Rivers. J. Sediment. Res. 64 (1), 76–85. doi:10.2110/jsr.64.76
Krumbein, W. C. (1941b). The Effects of Abrasion on the Size, Shape and Roundness of Rock Fragments. J. Geology. 49 (5), 482–520. doi:10.1086/624985
Li, Y., Jin, Z. K., Jin, T., and Shi, L. (2014). Geological Significance of Magmatic Gravel Roundness. Acta Sedimentologica Sinica 32 (2), 189–197. doi:10.14027/j.cnki.cjxb.2014.02.004
Liu, X. W. (2017). “Characteristics of Active Tectonics and the Deformation Pattern of the Jiuxi Basin at the Western Qilian Shan,” (Lanzhou: Lanzhou University). Doctoral dissertation.
Menting, F., Langston, A. L., and Temme, A. J. A. M. (2015). Downstream Fining, Selective Transport, and Hillslope Influence on Channel Bed Sediment in Mountain Streams, Colorado Front Range, USA. Geomorphology 239, 91–105. doi:10.1016/j.geomorph.2015.03.018
Meyer-Peter, E., and Muller, R. (1948). “Formulation for Bed Load Transport,” in Proceedings of 2nd meeting of the International Association for Hydraulic Structures Research, Delft, June 7, 1948, 39–64.
Mikos, M. (1993). Fluvial abrasion of gravel sediments: ETH Zurich, Mitteilungen der Versuchsanstalt für Wasserbau. Hydrologie und Glaziologie 123, 321.
Molnar, P., and England, P. (1990). Late Cenozoic Uplift of Mountain Ranges and Global Climate Change: Chicken or Egg?. Nature 346, 29–34. doi:10.1038/346029a0
Mosley, M. P., and Tindale, D. S. (1985). Sediment Variability and Bed Material Sampling in Gravel-Bed Rivers. Earth Surf. Process. Landforms 10 (5), 465–482. doi:10.1002/esp.3290100506
Pan, B., Li, Q., Hu, X., Geng, H., and Gao, H. (2015). Bedrock Channels Response to Differential Rock Uplift in Eastern Qilian Mountain along the Northeastern Margin of the Tibetan Plateau. J. Asian Earth Sci. 100, 1–19. doi:10.1016/j.jseaes.2014.12.009
Paola, C., Parker, G., Seal, R., Sinha, S. K., Southard, J. B., and Wilcock, P. R. (1992). Downstream Fining by Selective Deposition in a Laboratory Flume. Science 258 (5089), 1757–1760. doi:10.1126/science.258.5089.1757
Parker, G., Klingeman, P. C., and McLean, D. G. (1982). Bedload and Size Distribution in Paved Gravel-Bed Streams. J. Hydr. Div. 108, 544–571. doi:10.1061/jyceaj.0005854
Parker, G. (1991a). Selective Sorting and Abrasion of River Gravel. I: Theory. J. Hydraulic Eng. 117, 131–147. doi:10.1061/(asce)0733-9429(1991)117:2(131)
Parker, G. (1991b). Selective Sorting and Abrasion of River Gravel. II: Applications. J. Hydraulic Eng. 117 (7), 150–171. doi:10.1061/(asce)0733-9429(1991)117:2(150)
Powers, M. C. (1953). A New Roundness Scale for Sedimentary Particles. Sepm Jsr 23, 117–119. doi:10.1306/D4269567-2B26-11D7-8648000102C1865D
Rengers, F., and Wohl, E. (2007). Trends of Grain Sizes on Gravel Bars in the Rio Chagres, Panama. Geomorphology 83 (3-4), 282–293. doi:10.1016/j.geomorph.2006.02.019
Rice, S., and Church, M. (1998). Grain Size along Two Gravel-Bed Rivers: Statistical Variation, Spatial Pattern and Sedimentary Links. Earth Surf. Process. Landforms 23, 345–363. doi:10.1002/(sici)1096-9837(199804)23:4<345:aid-esp850>3.0.co;2-b
Rice, S. P., and Church, M. (2001). Longitudinal Profiles in Simple Alluvial Systems. Water Resour. Res. 37, 417–426. doi:10.1029/2000WR900266
Rice, S. P., and Haschenburger, J. K. (2004). A Hybrid Method for Size Characterization of Coarse Subsurface Fluvial Sediments. Earth Surf. Process. Landforms 29, 373–389. doi:10.1002/esp.1043
Rice, S. (1999). The Nature and Controls on Downstream Fining within Sedimentary Links. J. Sediment. Res. 69 (1), 32–39. doi:10.2110/jsr.69.32
Rice, S. (1998). Which Tributaries Disrupt Downstream Fining along Gravel-Bed Rivers?. Geomorphology 22, 39–56. doi:10.1016/S0169-555X(97)00052-4
Robinson, R. A. J., and Slingerland, R. L. (1998). Origin of Fluvial Grain-Size Trends in a Foreland basin; the Pocono Formation on the central Appalachian Basin. J. Sediment. Res. 68 (3), 473–486. doi:10.2110/jsr.68.473
Schumm, S. A. (1977). Arroyos and Environmental Change in the American Southwest. Earth Sci. Rev. 13 (2), 211–212. doi:10.1016/0012-8252(77)90042-3
Schwanghart, W., and Scherler, D. (2017). Bumps in River Profiles: Uncertainty Assessment and Smoothing Using Quantile Regression Techniques. Earth Surf. Dynam. 5 (4), 821–839. doi:10.5194/esurf-5-821-2017
Shaw, J., and Kellerhals, R. (1982). The Composition of Recent Alluvial Gravels in Alberta Riverbeds. Alberta Res. Counc. Bull. 41, 151.
Sklar, L. S., Dietrich, W. E., Foufoula-Georgiou, E., Lashermes, B., and Bellugi, D. (2006). Do gravel Bed River Size Distributions Record Channel Network Structure?. Water Resour. Res. 42 (6). doi:10.1029/2006WR005035
Song, C. H. (2006). “Tectonic Uplift and Cenozoic Sedimentary Evolution in the Northern Margin of the Tibetan Plateau,” (Lanzhou, Gansu Province, the People’s Republic of China: College of Earth and Environment Sciences, Lanzhou University). [dissertation].
Sternberg, H. (1875). Untersuchungen über längen-und querprofil geschiebeführender flüss. Z. für Bauwesen. 25, 483–550.
Stock, J. D., Schmidt, K. M., and Miller, D. M. (2008). Controls on Alluvial Fan Long-Profiles. Geol. Soc. America Bull. 120 (5-6), 619–640. doi:10.1130/B26208.1
Thayer, J. B., Phillips, R. T. J., and Desloges, J. R. (2016). Downstream Channel Adjustment in a Low-Relief, Glacially Conditioned Watershed. Geomorphology 262, 101–111. doi:10.1016/j.geomorph.2016.03.019
Wang, J. F. (2019). Analysis on Runoff Variation in the Beida River Basin under the Influence of Climate Change and Human Activities. J. Arid Land Resour. Environ. 33 (3), 86–91. doi:10.13448/j.cnki.jalre.2019.079
Wang, J., Li, A., Xu, K., Zheng, X., and Huang, J. (2015). Clay mineral and Grain Size Studies of Sediment Provenances and Paleoenvironment Evolution in the Middle Okinawa Trough since 17ka. Mar. Geology. 366, 49–61. doi:10.1016/j.margeo.2015.04.007
Wang, Y., Oskin, M. E., Zhang, H., Li, Y., Hu, X., and Lei, J. (2020). Deducing Crustal‐Scale Reverse‐Fault Geometry and Slip Distribution from Folded River Terraces, Qilian Shan, China. Tectonics 39 (1), 1–18. doi:10.1029/2019TC005901
W. C. Krumbein, W. C. (1941a). Measurement and Geological Significance of Shape and Roundness of Sedimentary Particles. Sepm Jsr 11, 64–72. doi:10.1306/D42690F3-2B26-11D7-8648000102C1865D
W. C. Krumbein, W. C. (1934). Size Frequency Distributions of Sediments. Sepm Jsr 4 (2), 65–77. doi:10.1306/D4268EB9-2B26-11D7-8648000102C1865D
Werritty, A., and Florence, S. (1990). Downstream Fining in a Gravel-Bed River in S. Poland: Lithologic Controls and the Role of Abrasion. St. Andrews, Fife, Scotland, U.K: Department of Geography and Geology, University of St. Andrews.
Westaway, R. (2009). Active Crustal Deformation beyond the SE Margin of the Tibetan Plateau: Constraints from the Evolution of Fluvial Systems. Glob. Planet. Change 68 (4), 395–417. doi:10.1016/j.gloplacha.2009.03.008
Whittaker, A. C., Attal, M. l., and Allen, P. A. (2009). Characterising the Origin, Nature and Fate of Sediment Exported from Catchments Perturbed by Active Tectonics. Basin Res. 22 (6), 809–828. doi:10.1111/j.1365-2117.2009.00447.x
Wiberg, P. L., and Smith, J. D. (1987). Calculations of the Critical Shear Stress for Motion of Uniform and Heterogeneous Sediments. Water Resour. Res. 23, 1471–1480. doi:10.1029/WR023i008p01471
Wilcock, P. R., and Kenworthy, S. T. (2002). A Two-Fraction Model for the Transport of Sand/gravel Mixtures. Water Resour. Res. 38 (10), 12–21. doi:10.1029/2001WR000684
Wilcock, P. R., and Southard, J. B. (1989). Bed Load Transport of Mixed Size Sediment: Fractional Transport Rates, Bed Forms, and the Development of a Coarse Bed Surface Layer. Water Resour. Res. 25, 1629–1641. doi:10.1029/WR025i007p01629
Wu, F., Fang, X., Ma, Y., Herrmann, M., Mosbrugger, V., An, Z., et al. (2007). Plio-Quaternary Stepwise Drying of Asia: Evidence from a 3-Ma Pollen Record from the Chinese Loess Plateau. Earth Planet. Sci. Lett. 257 (1–2), 160–169. doi:10.1016/j.epsl.2007.02.029
Yang, H., Yang, X., Huang, X., Li, A., Huang, W., and Zhang, L. (2018). New Constraints on Slip Rates of the Fodongmiao-Hongyazi Fault in the Northern Qilian Shan, NE Tibet, from the 10Be Exposure Dating of Offset Terraces. J. Asian Earth Sci. 151, 131–147. doi:10.1016/j.jseaes.2017.10.034
Yang, S. X. (2008). “Water Resources Research on the Phase I of the Jia-Jiu Coal Electricity’s Base in Gansu Province, China,” (Shaanxi: Northwest Agriculture and Forestry University). [master’s thesis].
Yizhou, W., Huiping, Z., Dewen, Z., Wenjun, Z., Zhuqi, Z., Weitao, W., et al. (2014). Controls on Decadal Erosion Rates in Qilian Shan: Re-evaluation and New Insights into Landscape Evolution in north-east Tibet. Geomorphology 223, 117–128. doi:10.1016/j.geomorph.2014.07.002
Zhang, J., Geng, H., Pan, B., Hu, X., Chen, L., Wang, W., et al. (2020). Climatic Zonation Complicated the Lithology Controls on the Mineralogy and Geochemistry of Fluvial Sediments in the Heihe River basin, NE Tibetan Plateau. Quat. Int. 537, 33–47. doi:10.1016/j.quaint.2020.01.016
Zhao, B., Huang, Y., Zhang, C., Wang, W., Tan, K., and Du, R. (2015). Crustal Deformation on the Chinese mainland during 1998-2014 Based on GPS Data. Geodesy and Geodynamics 6 (1), 7–15. doi:10.1016/j.geog.2014.12.006
Zhao, Z., Fang, X., and Li, J. (2001). Late Cenozoic Magnetic Polarity Stratigraphy in the Jiudong Basin, Northern Qilian Mountain. Sci. China Ser. D-earth Sci. 44 (1), 243–250. doi:10.1007/bf02911993
Keywords: riverbed gravel, grain size, hydraulic sorting, abrasion, channel slope
Citation: Dong Z, Pan B, Hu Z, Mo Q, Bridgland D, Li M, Li X, Yang Y and Chen D (2022) Evaluation of the Fluvial Response to Tectonic Uplift From Grain-Size Distribution in Riverbed Gravels at the Northeastern Margin of the Tibetan Plateau. Front. Earth Sci. 10:824368. doi: 10.3389/feart.2022.824368
Received: 29 November 2021; Accepted: 27 January 2022;
Published: 04 March 2022.
Edited by:
Rong Yang, Zhejiang University, ChinaReviewed by:
Xiubin Lin, Zhejiang University, ChinaCopyright © 2022 Dong, Pan, Hu, Mo, Bridgland, Li, Li, Yang and Chen. This is an open-access article distributed under the terms of the Creative Commons Attribution License (CC BY). The use, distribution or reproduction in other forums is permitted, provided the original author(s) and the copyright owner(s) are credited and that the original publication in this journal is cited, in accordance with accepted academic practice. No use, distribution or reproduction is permitted which does not comply with these terms.
*Correspondence: Baotian Pan, cGFuYnRAbHp1LmVkdS5jbg==; Zhenbo Hu, emhiaHVAbHp1LmVkdS5jbg==
Disclaimer: All claims expressed in this article are solely those of the authors and do not necessarily represent those of their affiliated organizations, or those of the publisher, the editors and the reviewers. Any product that may be evaluated in this article or claim that may be made by its manufacturer is not guaranteed or endorsed by the publisher.
Research integrity at Frontiers
Learn more about the work of our research integrity team to safeguard the quality of each article we publish.