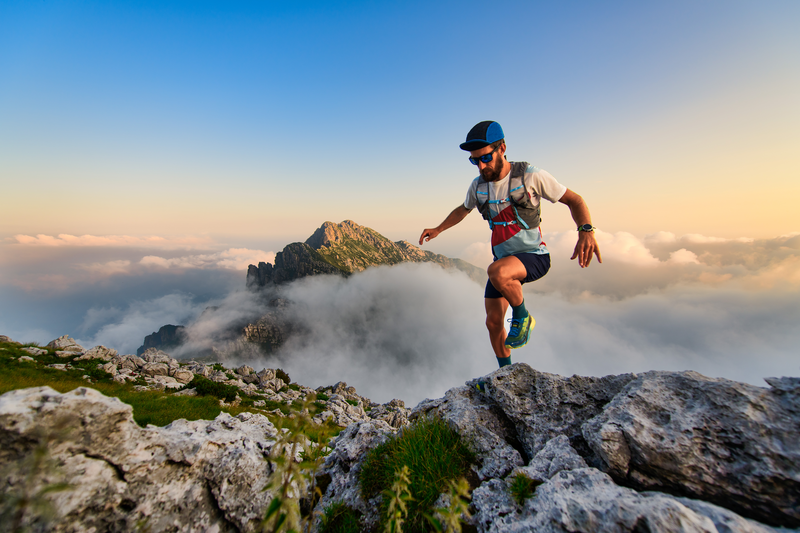
94% of researchers rate our articles as excellent or good
Learn more about the work of our research integrity team to safeguard the quality of each article we publish.
Find out more
ORIGINAL RESEARCH article
Front. Earth Sci. , 24 March 2022
Sec. Economic Geology
Volume 10 - 2022 | https://doi.org/10.3389/feart.2022.824056
This article is part of the Research Topic Progress in Exploration, Development and Utilization of Geothermal Energy View all 28 articles
Geothermal energy and petroleum are two resources that coexist in sedimentary basins, and both of them are closely related to temperature. Based on the surface heat flow and the rock thermophysical properties, the deep geothermal field was calculated, then temperature distribution characteristics and the primary influencing factors on the geothermal state were discussed. The temperature distribution map of the Sichuan Basin for depths of 3,000–9,000 m presented the central and southern areas are characterized by relatively higher temperatures while the northwestern and northeastern areas are characterized by lower temperatures. Strata temperature in the central and southern areas exceeded 150°C as the burial depth reached 5,000 m. At the depth of 7,000 m, the temperatures in the central and southern areas were above 180°C, which is the threshold temperature for hot dry rock (HDR), while the temperatures in the northwestern and northeastern areas were below 160°C, which is close to the lower limit for oil crude oil cracking. The central and southern areas are favorite zones for the exploration of geothermal energy, conversely, the relatively lower temperatures in the northern, northwestern, and eastern areas are conducive to the presence of oil and gas. The unevenly distribution of geothermal field were controlled by the thicknesses of sedimentary layer and the crust, which indicates the lithosphere flexure and thickening caused by compression and depression, rapid deposition in the foreland depressions. The results can provide geothermal understandings for the exploration and development of the petroleum and geothermal resources and could serve as a reference for geodynamic studies in the Sichuan Basin.
Geothermal resources utilization can not only save non-renewable energy but also reduce carbon emissions and pollution to mitigate smog. Therefore, geothermal resources have become a topic of interest in research on the development and exploitation of new energy. Geothermal energy and petroleum are two resources that coexist in sedimentary basins. Oilfields are rich not only in oil and gas but also often in geothermal resources. Thus, geothermal energy is rapidly developed and exploited in oil and gas exploration areas (Wang et al., 2016; Shah et al., 2017). The knowledge and experience gained from oil and gas development is often transferable to the exploitation of geothermal energy. Furthermore, a considerable number of abandoned boreholes are available for re-exploitation of geothermal resources instead of oil and gas. With the valuable technical expertise gained from previous drilling and oil production processes, scientists can use exploratory boreholes to exploit geothermal resources with minor modifications. This reduces the development costs and risks, which has created a booming market for geothermal energy exploitation in oil and gas fields (Li et al., 2016).
The Sichuan Basin is a petroliferous basin in Southwest China. The Puguang and Yuanba gas fields in the basin are important for natural gas production. The Sichuan Basin is also rich in geothermal resources with approximately 300 hot springs distributed in the peripheral mountains (Wang, 2005). Numerous medium- and low-temperature geothermal areas near Chongqing have been developed for domestic heating and industrial and agricultural production (Lv et al., 2010). However, the exploitation of geothermal resources in the research area mainly are hot springs or shallow geothermal wells in the mountain areas, the exploitation of deep geothermal energy has not yet developed in the hinterland of the basin. The Sichuan Basin is densely populated, and its agricultural and industrial sectors are well developed. Its high-temperature (≥150°C) geothermal resources should also be developed to supply clean energy. Research on the geothermal fields and terrestrial heat flow in the Sichuan Basin began in the 1980s. Over the past 4 decades, numerous studies have been conducted on the basin and the surrounding areas, including temperature measurements from hundreds of wells, the thermal physical properties of the rocks, and various terrestrial heat flow data (Yuan et al., 2006; Xu et al., 2011; Li et al., 2020). These studies have provided basic knowledge on the thermal state of the Sichuan Basin. For example, higher heat flow has been observed in the central and southern areas of the basin, while lower heat flow has been identified in the northeastern, northwestern, and southeastern areas. Scientists have enriched their knowledge of the stratigraphy through oil and gas exploration to augment their data on the thermal and physical properties of the rock.
Deep geothermal field is the most basic parameter to evaluate geothermal resources, and also affect hydrocarbon generation, migration, and accumulation processes. However, the deep temperature fields had not been stated over all, limited to the distribution and depth of the drilling and logging of boreholes. Further investigation on the geothermal fields of the basin is necessary. In this study, data collected from previous studies were used to calculate the deep geothermal fields in the basin and evaluate its distribution characteristics and primary influencing factors. The results should benefit the exploration and development of the petroleum and geothermal resources in the basin and could serve as a reference for geodynamic studies.
The Sichuan Basin is located in the western margin of the Upper Yangtze Plate and is a marine and continental superimposed basin, in which the foreland basins developed on a cratonic platform (Guo et al., 1996, Meng et al., 2005; Liu et al., 2006) (Figure 1A). It is characterized by a distinctive rhombic rim that reflects the evolution of deep fractures in the Upper Yangtze and the development of thrust–fold structures. The central part of the Sichuan Basin is a rigid basement that formed in the Neoproterozoic–Mesoproterozoic era; it is uplifted with thin sedimentary layers. The northwestern and southeastern parts of the basin are a ductile basement that is covered with thick sedimentary layers (Wang et al., 2002). The Sichuan Basin has deposits of sedimentary rocks that are up to 10,000 m thick that can be dated to the Sinian Period (Guo et al., 1996; Wang et al., 2002; Liu et al., 2006), with diverse rock types and depositional systems. The Sinian–Middle Triassic strata are characterized by marine facies that are dominated by carbonate rocks such as limestone and dolomite and that contain mudstone and sandstone. From the Middle–Late Triassic Period onwards, a thick set of foreland basin deposition had formed, contains sandstone, mudstone, and other terrestrial clastic deposits (Figure 1B).
FIGURE 1. (A) Geological setting and the location of wells which have steady state temperature logging data in the Sichuan Basin (the temperature data were published by Xu et al., 2011 and Li et al., 2017) and (B) stratigraphic histogram of the study area. The geological map was based on Zhu et al. (2018a); Zhu et al. (2018b), the distribution and zoning of the Emeishan Large Igneous Province (ELIP) was based on He et al. (2003), He et al. (2007), basalt thickness of the wells in Southwestern Sichuan was based on Zhang et al. (2006) and the lithological column was modified from Rao et al. (2011).
The tectono-thermal evolution of the Sichuan Basin can be divided into four stages. The first stage was the formation of the cratonic basin in the Early Paleozoic. A relatively low background heat flow was recorded despite the ongoing weak lithospheric tension (He et al., 2014; Zhu et al., 2016). The second stage occurred during the Early Permian–Middle Triassic, which was controlled by two events: the lithospheric re-extension and the Emeishan mantle plume. In particular, the Emeishan mantle plume increased the heat flow to its peak value in the southwestern part of the basin, which was at the intermediate zone of the mantle plume (Zhu et al., 2016; Zhu et al., 2018a, Zhu et al., 2018b). The third stage occurred during the Late Triassic–Early Cretaceous when the Sichuan Basin and surrounding orogenic belt started to evolve into an inland basin because of the closure of the Paleo-Tethys Ocean. As the lithosphere and sedimentary layers thickened, the heat flow continued to decrease (He et al., 2011; He, 2014). The fourth stage reflects the tectono-thermal evolution since the Cretaceous when uplift and exhumation started in the Sichuan Basin (Zhu et al., 2016). Currently, the terrestrial heat flow in the Sichuan Basin is unevenly distributed. A higher heat flow can be observed at the central area, while lower heat flows have been observed in other areas, and the Sichuan Basin can be regarded as a “cold basin” due to the low mean heat flow (Xu et al., 2011).
Boreholes can be used to measure the temperature at different depths directly. In contrast, temperatures of undrilled strata cannot be directly measured but can only be obtained by calculation. The main mode of heat transfer in the crust is conduction, thus, the temperature of stratum i deep in a sedimentary basin can be calculated by using the one-dimensional heat conduction equation (Qiu et al., 2004):
where Ti0 is the temperature for the top surface of the stratum, qi0 is the heat flow for the top surface of the stratum, Ki is the thermal conductivity of the stratum, Ai is the heat generation rate of the stratum, and d is the thickness of the stratum. If the heat generation rate of the stratum decreases exponentially with the depth, this results in the following equation:
where q0 is the heat flow of the top surface of the stratum (mW/m2), K is the rock thermal conductivity of the stratum (W/(mK)), A′ is the heat generation rate of the stratum (µW/m3), and D is the thickness of a stratum containing radioactive elements.
Eq. 1 is mostly used to calculate deep temperatures in sedimentary strata, and Eq. 2 is mostly used to calculate temperatures below the basement. Note that these equations do not apply below the upper mantle. There are two reasons: strata are rich with radioactive elements in the crust within about 10–20 km above the upper mantle, and heat conduction at that depth is no longer a decisive influencing factor for the temperature.
According to Eqs 1, 2, the boundary conditions for calculating the temperature of deep strata include the heat flow and temperature of the top surface. For the surface heat flow, data from Xu et al. (2011) were adopted. For the surface temperature, the Sichuan Basin thermostatic zone temperature of 16°C was adopted (according to Wang et al., 2017). The thermal physical parameters of the rock required for calculation included the rock thermal conductivity and radiogenic heat generation rate of the strata.
In the Sichuan Basin, numerous studies have been conducted to test thermal conductivity. In this study, more than 1,300 thermal conductivity measurements from previous research (Li, 1990; Han and Wu, 1993; Wang et al., 1995; Xu et al., 2011; Li et al., 2017; Tang et al., 2019; Li et al., 2020) were collected. These were then used to calculate the weighted average of the thermal conductivity of different lithologies tested by different researchers (Supplementary Appendix S1). The thermal conductivity of each sedimentary formation was established based on the lithological composition of the main strata (Table 1). The thermal conductivity of the Cretaceous and Jurassic strata was relatively low at 2.5 W/(m·K), while that of the Permian, Ordovician, and Cambrian was approximately 3.0 W/(m·K). The thermal conductivity of the Triassic, Devonian, Carboniferous, and Sinian strata was greater than 3.0 W/(m·K).
TABLE 1. The lithology composition and the thermal conductivity (K) and heat production rate (A) of sedimentary layers in the Sichuan Basina.
The heat generation rate of the sedimentary layers was measured (Table 1, Zhu et al., 2018a). Among sedimentary rocks, mud shale generally has a relatively high heat generation rate, followed by carbonate rocks of terrestrial origin or those that contain clastic materials from a terrestrial margin. Marine carbonate rocks have a relatively low heat generation rate. The heat generation rate of terrestrial strata in the Sichuan Basin was approximately 1.0 µW/m3, and that of the marine strata was lower at around 0.6 µW/m3. The heat generation rate of the Silurian system can be as high as 1.09 µW/m3 because of the high proportion of sandy mudstone. The heat generation rate of rocks below the basement was estimated by using the seismic wave velocity (vp) (Rybach and Buntebarth, 1984):
where A is the rock heat generation rate (µW/m3) and vp is the seismic wave velocity (km/s) at a pressure of 100 MPa. Table 2 presents the estimated heat generation rates of rocks in the upper and lower crust beneath the sedimentary layers of the Sichuan Basin.
TABLE 2. Crustal structure and heat generation rate in the Sichuan Basina.
Figure 2 showed the temperature distribution map of the Sichuan Basin for depths of 3,000–9,000 m, which calculated by one-dimensional heat conduction equation, taking the surface heat flow, the thermostatic zone temperature, the thermal conductivity and heat production rate of different formations and the formation depths as parameters and boundary conditions. The central and southern areas are characterized by relatively high temperatures at all depths, while the northwestern and northeastern areas are characterized by low temperatures. It is obviously that the strata temperature is lower than 100°C at the depth of 3000 m excluding some high temperatures in central and southern areas (Figure 2A). At the depth of 4000 m (Figure 2B), the maximum temperature in the central and southern areas reach 150°C, which is the threshold of high-temperature geothermal resources, while the temperatures in other areas are below 120°C. Strata temperature in the central and southern areas exceed 150°C as the burial depth reach 5,000 m (Figure 2C), while it is lower than 140°C in most other areas. At the depth of 6,000 m (Figure 2D), the temperature is above 150°C for large parts of the central and southern areas, and the maximum temperature reach 185°C, while the temperature is below 120°C for most parts of the northwestern and northeastern areas. Similar temperature distribution patterns were observed at the depth of 7,000–9,000 m (Figure 2E–2G). At the depth of 7,000 m (Figure 2E), the temperatures in the central and southern areas are above 180°C, which is the threshold temperature for hot dry rock (HDR). The temperatures in the northwestern and northeastern areas are below 160°C, which is close to the lower limit for crude oil cracking. The relatively low temperatures in the northern, northwestern, and eastern areas of the Sichuan Basin are conducive to the presence of oil and gas. Given an economic depth should be considered regarding its cost for the exploitation of geothermal energy and also the petroleum resources, it is suggested that the temperature distributions of the depth within 4–6 km are applicable for geothermal resources evaluation and these of the depth within 7–9 km are referential for oil and gas.
FIGURE 2. Temperature distribution at the depth of 3,000–9000 m (A–G) in the Sichuan Basin. The red dash lines indicate the main faults which controlled the boundary of the basin and its surrounding mountains or divide the tectonic units inside the basin.
Note that the strata temperature is influenced not only by the heat flow and thermal physical properties but also by the burial depth of a formation. Therefore, for specific formations (e.g., oil and gas reservoirs), the highest temperatures are more likely to be observed in areas with greater burial depths. In this case, this would correspond with the northwestern and northeastern areas of the Sichuan Basin, which characterized by lower heat flow and temperature gradient but have a thick sediment layer.
Terrestrial heat flow directly reflects the geothermal and geological background of a region. The terrestrial heat flow in the Sichuan Basin is 35.4–68.8 mW/m2 with an average of 53.2 mW/m2. This is below the average of 60 mW/m2 in the Lower Yangtze region and 63 mW/m2 for mainland China (Xu et al., 2011; Yuan et al., 2006). Regarding the distribution, the terrestrial heat flow was greater in the uplifted area and lower in the depressed area. The terrestrial heat flow is above 60 mW/m2 in the central and southwestern areas of the Sichuan Basin and is 50–60 W/m2 in the northwestern area (Figure 3A). The terrestrial heat flow is lower in the eastern area, particularly in the northeast where it is approximately 40 mW/m2 at the front edge of the Dabashan fold belt. The deep temperature distribution in the Sichuan Basin shows a good correlation with the terrestrial heat flow, which is primarily influenced by the geotectonics and lithospheric thermal structure. The mantle heat flow in the Sichuan Basin is 12–49 mW/m2 with higher values in the southwestern and central areas and lower values in the northeastern area (Figure 3B). This is similar to the surface heat flow distribution. The intense magma activities and eruptions mainly took placed in Permian (the Emeishan mantle plume and basalt eruption) and raised the heat flow to its peak value (Zhu et al., 2016). There was no obvious magma activity after Jurassic in the basin, thus the surface heat flow can be considered an stable conductive heat flow.
FIGURE 3. distribution (A) (edited after Xu et al., 2011; Zhu et al., 2018a and Qiu et al., 2022), the mantle heat flow distribution (B) and the burial depth of the folded basement (edited after Luo, 1998) of the Sichuan Basin. The mantle heat flow was calculated based on the surface heat flow, the heat production rate in the sedimentary layers, the basement and the other parts of the crust. An obvious correlation can be found from both of the surface heat flow, mantle heat flow and the basement burial depth.
At the basin scale, the average mantle heat flow is approximately 30 mW/m2, while the average crustal heat flow is 32 mW/m2. The ratio of the crustal heat flow to the mantle heat flow is close to 1:1, which indicates that the Sichuan Basin has a cold mantle and cold crust. In the basin, the surface and mantle heat flow distributions show negative correlations with the thicknesses of the sedimentary layers and crust. This is indicative of the thermal effects of tectonics and sedimentation during the development of foreland basins. The heat flow in the Sichuan Basin slowly decreased during the Late Triassic–Early Cretaceous, and its tectono-thermal evolution was primarily controlled by two factors. First, the thermal perturbation caused by the lithospheric thinning during the Late Permian–Early Middle Triassic began to decrease (Zhu et al., 2016), which gradual decreased the heat flow of the basin basement. Second, the formation and evolution of the foreland basin and its later uplifting and exhumation reduced the heat flow (Huang et al., 2012). The reduced heat flow caused by foreland basin evolution and uplift and exhumation is primarily controlled by the following factors. First, lithosphere flexure and thickening, as well as gradual cooling, which were caused by compression and depression. Second, the surface heat flow was affected by the deposition in the foreland depressions. Third, exhumation caused by the compressional deformation can also reduce the surface heat flow in the mountain areas (Figure 4).
FIGURE 4. Cartoon shows the main controlling factors on the unevenly distribution of geothermal field in the Sichuan Basin and its surrounding mountains (the geological model was modified after Zhu et al., 2019).
The difference in thermal conductivity among different sedimentary layers and crystalline rocks also affects the deep temperature fields and the redistribution of the heat flow. The temperature gradient negatively correlates with the strata thermal conductivity under a constant heat flow. In fact, thermal conductivity has a more significant impact than the heat production of strata in sedimentary basins, unless ultra-high radioactivity layers exist. Various temperature gradients were vertically revealed by the deep geothermal fields (Figure 5) and some temperature logging data of boreholes (Figure 6). Two different average temperature gradients in clastic formations and limestone formations were obtained in borehole C5: it is lower in the latter formations which has a relative higher thermal conductivity. As a contrast, the temperature gradient is relative steady in borehole Cq128, for only clastic formations revealed (Figure 7).
FIGURE 5. Temperature gradient distribution within the depth of 1,000m–3000 m (A) and 5,000m–7000 m (B) in the Sichuan Basin.
Furthermore, non-uniform temperature and the difference in thermal conductivity causes thermal refraction, which leads heat to converge to the uplifted basement area with high thermal conductivity. The upper sedimentary layers form an insulating cover, make these uplifts into favorable geothermal targets.
Controlled by the thicknesses of sedimentary layer and the crust, the heat flow and deep temperature distributed unevenly in the Sichuan Basin. The central and southern areas have higher temperatures than northeastern and northwestern areas in the same depth, due to the high heat flow.
Lithosphere flexure and thickening caused by compression and depression, rapid deposition in the foreland basin, the exhumation in the surrounding mountains reduce the surface heat flow in foreland depressions in the northwestern and northeastern basin and their surrounding mountains.
Different thermal conductivities of strata affect the deep temperature fields and lead to thermal refraction. Uplifts in sedimentary basins characterized by higher heat flow and covered by relative insulating layers form the geothermal favorable targets.
The central and southern areas characterized by higher heat flow and temperatures are advantageous for geothermal energy exploitation. The relatively low temperatures in the northern, northwestern, and eastern areas of the Sichuan Basin are conducive to the presence of oil and gas.
The original contributions presented in the study are included in the article/Supplementary Material, further inquiries can be directed to the corresponding author.
CZ was the primary finisher of this paper, NQ provided the funding of this paper. TX, TC, and RD completed part of data processing work for this paper.
This study was supported by the National Natural Science Foundation of China (Grant No. 41830424 and 41772248).
The authors declare that the research was conducted in the absence of any commercial or financial relationships that could be construed as a potential conflict of interest.
All claims expressed in this article are solely those of the authors and do not necessarily represent those of their affiliated organizations, or those of the publisher, the editors and the reviewers. Any product that may be evaluated in this article, or claim that may be made by its manufacturer, is not guaranteed or endorsed by the publisher.
The Supplementary Material for this article can be found online at: https://www.frontiersin.org/articles/10.3389/feart.2022.824056/full#supplementary-material
Guo, Z. W., Deng, K. L., Han, Y. H., Liu, Y. K., Yin, J. T., Wang, Q. G., et al. (1996). The Formation and Development of Sichuan Basin. Beijing: Geological Publishing House.
Han, Y. H., and Wu, C. S. (1993). Geothermal Gradient and Heat Flow Values of Some Deep wells in Sichuan Basin. Oil Gas Geology. 14 (1), 80–84. doi:10.11743/ogg19930111
He, L. (2014). Permian to Late Triassic Evolution of the Longmen Shan Foreland Basin (Western Sichuan): Model Results from Both the Lithospheric Extension and Flexure. J. Asian Earth Sci. 93 (oct. 05), 49–59. doi:10.1016/j.jseaes.2014.07.007
He, B., Xu, Y.-G., Chung, S.-L., Xiao, L., and Wang, Y. (2003). Sedimentary Evidence for a Rapid, Kilometer-Scale Crustal Doming Prior to the Eruption of the Emeishan Flood Basalts. Earth Planet. Sci. Lett. 213, 391–405. doi:10.1016/s0012-821x(03)00323-6
He, B., Xu, Y.-G., Huang, X.-L., Luo, Z.-Y., Shi, Y.-R., Yang, Q.-J., et al. (2007). Age and Duration of the Emeishan Flood Volcanism, SW China: Geochemistry and SHRIMP Zircon U-Pb Dating of Silicic Ignimbrites, post-volcanic Xuanwei Formation and clay Tuff at the Chaotian Section. Earth Planet. Sci. Lett. 255, 306–323. doi:10.1016/j.epsl.2006.12.021
He, L., Xu, H., and Wang, J. (2011). Thermal Evolution and Dynamic Mechanism of the Sichuan Basin during the Early Permian-Middle Triassic. Sci. China Earth Sci. 54 (12), 1948–1954. doi:10.1007/s11430-011-4240-z
He, L. J., Huang, F., Liu, Q. Y., Li, C. R., and Wang, J. Y. (2014). Tectono-thermal Evolution of Sichuan Basin in Early Paleozoic. J. Earth Sci. Env 36 (2), 10–17.
Huang, F., Liu, Q. Y., and He, L. J. (2012). Tectono-thermal Modeling of the Sichuan Basin since the Late Himalayan Period. Chin. J. Geophys. 55 (11), 3742–3753. doi:10.6038/j.issn.0001-5733.2012.11.021
Li, Q. Y. (1990). Study on Deep thermal Characteristics of the Sichuan Basin. Chengdu University of Technology.
Li, H. T., Li, J. Y., and Zhang, G. Y. (2016). Research and Comprehensive Development and Utilization of Buried hill Geothermal Resources in Huabei Oilfield. Beijing: Petroleum Industry Press.
Li, C. R., Rao, S., Hu, S. B., Wang, J. Y., Wei, Z. H., Huang, Q. Q., et al. (2017). Present-day Geothermal Field of the Jiaoshiba Shale Gas Area in Southeast of the Sichuan Basin, SW China. Chin. J. Geophys. 60 (2), 617–627. doi:10.6038/cjg20170216
Li, X. L., Liu, S. W., Xu, M., Li, X. D., and Hao, C. Y. (2020). Estimation of Subsurface Formation Temperature and its Implications for Hydrocarbon Generation and Preservation in the Upper Yangtze Area, south China. Acta Geologica Sinica 94 (6), 1896–1910. doi:10.1111/1755-6724.13809
Liu, H. P., Li, J. M., Li, X. Q., Liu, L. Q., Li, X. J., and Hu, S. H. (2006). Evolution of Cratonic Basins and Carbonate-Evaporite Sedimentary Sequence Hydrocarbon Systems in China. Geoscience 20 (1), 1–18. doi:10.3969/j.issn.1000-8527.2006.01.001
Luo, Z. (1998). New Recognition of Basement in Sichuan Basin. J. Chengdu Univ. Technol. 25, 191–200.
Lv, Y. X., Hu, W., Du, C. L., and Yang, Z. G. (2010). Review on the Progress of Geothermal Water Exploration Research in Chongqing. Chin. J. Underground Space Eng. 6 (s2), 1544–1547. doi:10.3969/j.issn.1673-0836.2010.z2.005
Meng, Q.-R., Wang, E., and Hu, J.-M. (2005). Mesozoic Sedimentary Evolution of the Northwest Sichuan Basin: Implication for Continued Clockwise Rotation of the South China Block. Geol. Soc. America Bull. 117 (3), 396–410. doi:10.1130/b25407.1
Qiu, N. S., Hu, S. B., and He, L. J. (2004). Theory and Application of thermal Regime Study in Sedimentary Basins. Beijing: Petroleum Industry Press.
Qiu, N., Chang, J., Zhu, C., Liu, W., Zuo, Y., Xu, W., et al. (2022). Thermal Regime of Sedimentary Basins in the Tarim, Upper Yangtze and North China Cratons, China. Earth-Science Rev. 224, 103884. doi:10.1016/j.earscirev.2021.103884
Rao, S., Tang, X. Y., Zhu, C. Q., Hu, S. B., and Wang, J. Y. (2011). The Application of Sensitivity Analysis in the Source Rock Maturity History simulation:An Example from Palaeozoic marine Source Rock of Puguang-5 Well in the Northeast of Sichuan Basin. Chin. J. Geology. 46 (1), 213–225. doi:10.3969/j.issn.0563-5020.2011.01.017
Rybach, L., and Buntebarth, G. (1984). The variation of heat generation, density and seismic velocity with rock type in the continental lithosphere. Tectonophysics 103(1-4), 335–344. doi:10.1016/0040-1951(84)90095-7
Shah, M., Vaidya, D., Dhale, S., Sircar, A., Sahajpal, S., and Yadav, K. (2017). “Exploitation and Utilization of Oilfield Geothermal Resources in INDIA,” in Nanotechnology for Energy and Water. Editors G. Anand, J. K. Pandey, and S. Rana (Switzerland AG: Springer Nature), 47–52. doi:10.1007/978-3-319-63085-4_7
Tang, B., Zhu, C., Xu, M., Chen, T., and Hu, S. (2019). Thermal Conductivity of Sedimentary Rocks in the Sichuan Basin, Southwest China. Energy Exploration & Exploitation 37 (2), 691–720. doi:10.1177/0144598718804902
Wang, D. Y. (2005). Geological Genesis Analysis of the Niangchunchi Hot spring in Mianzhu, Sichuan, China. J. Chengdu Univ. Tech. (Nat. Sci. Ed. 32 (5), 479–485. doi:10.1360/gs050303
Wang, L. S., Li, C., Shi, Y. S., and Wang, Y. H. (1995). Distributions of Geotemperature and Terrestrial Heat Flow Density in Lower Yangtze Area. Chin. J. Geophys. 38 (4), 469–476.
Wang, Z. C., Zhao, W. Z., Zhang, L., and Wu, S. X. (2002). Tectonic Sequences and Natral Gas Prospecting in the Sichuan basin. Beijing: Geological Press House.
Wang, S., Yan, J., Li, F., Hu, J., and Li, K. (2016). Exploitation and Utilization of Oilfield Geothermal Resources in China. Energies 9 (10), 798. doi:10.3390/en9100798
Wang, W. L., Wang, G. L., Zhu, X., and Liu, Z. M. (2017). Characteristics and Potential of Shallow Geothermal Resources in Provincial Capital Cities of China. Geology. China 44 (6), 1062–1073. doi:10.12029/gc20170602
Wang, Y. H., Tang, L., Huang, C. M., Fu, Y., Jiang, B., and Guan, Y. (2019). Study on One Dimensional Velocity Model of Sichuan Area. Earthquake Res. Sichuan 2019(3), 8–12. doi:10.13716/j.cnki.1001-8115.2019.03.003
Xu, M., Zhu, C. Q., Tian, Y. T., Rao, S., and Hu, S. B. (2011). Borehole Temperature Logging and Characteristics of Subsurface Temperature in Sichuan Basin. Chin. J. Geophys. 54 (4), 224–233. doi:10.1002/cjg2.1604
Yuan, Y.-S., Ma, Y.-S., Hu, S.-B., Guo, T.-L., and Fu, X.-Y. (2006). Present-day Geothermal Characteristics in South China. Chin. J. Geophys. 49 (4), 1005–1014. doi:10.1002/cjg2.922
Zhang, R. X., Wang, X. Z., Lan, Q., and Kang, B. P. (2006). Reservoir Evaluation of Emeishan Basalts in Southwest Sichuan. Nat. Gas Explor Dev. 29, 17–20. doi:10.3969/j.issn.1673-3177.2006.01.004
Zhu, C., Hu, S., Qiu, N., Rao, S., and Yuan, Y. (2016). The thermal History of the Sichuan Basin, SW China: Evidence from the Deep Boreholes. Sci. China Earth Sci. 59 (1), 70–82. doi:10.1007/s11430-015-5116-4
Zhu, C., Xu, M., Qiu, N., and Hu, S. (2018a). Heat Production of Sedimentary Rocks in the Sichuan Basin, Southwest China. Geochem. J. 52 (5), 401–413. doi:10.2343/geochemj.2.0530
Zhu, C., Hu, S., Qiu, N., Jiang, Q., Rao, S., and Liu, S. (2018b). Geothermal Constraints on Emeishan Mantle Plume Magmatism: Paleotemperature Reconstruction of the Sichuan Basin, SW China. Int. J. Earth Sci. (Geol Rundsch) 107 (1), 71–88. doi:10.1007/s00531-016-1404-2
Keywords: geothermal field, thermal conductivity, radioactive heat production, heat flow, Sichuan Basin
Citation: Zhu C, Xu T, Qiu N, Chen T, Xu M and Ding R (2022) Distribution Characteristics of the Deep Geothermal Field in the Sichuan Basin and its Main Controlling Factors. Front. Earth Sci. 10:824056. doi: 10.3389/feart.2022.824056
Received: 28 November 2021; Accepted: 21 February 2022;
Published: 24 March 2022.
Edited by:
Yanlong Kong, Institute of Geology and Geophysics (CAS), ChinaReviewed by:
Yingchun Wang, Chengdu University of Technology, ChinaCopyright © 2022 Zhu, Xu, Qiu, Chen, Xu and Ding. This is an open-access article distributed under the terms of the Creative Commons Attribution License (CC BY). The use, distribution or reproduction in other forums is permitted, provided the original author(s) and the copyright owner(s) are credited and that the original publication in this journal is cited, in accordance with accepted academic practice. No use, distribution or reproduction is permitted which does not comply with these terms.
*Correspondence: Chuanqing Zhu, emh1Y3FAY3VwLmVkdS5jbg==
Disclaimer: All claims expressed in this article are solely those of the authors and do not necessarily represent those of their affiliated organizations, or those of the publisher, the editors and the reviewers. Any product that may be evaluated in this article or claim that may be made by its manufacturer is not guaranteed or endorsed by the publisher.
Research integrity at Frontiers
Learn more about the work of our research integrity team to safeguard the quality of each article we publish.