- 1Department of History, Jinan University, Guangzhou, China
- 2School of Archaeology and Ancient History, University of Leicester, Leicester, United Kingdom
- 3Department of Archaeology and Natural History, Australian National University, Canberra, Australia
- 4Group of Bioarchaeology, Key Laboratory of Western China’s Environmental Systems (Ministry of Education), College of Earth and Environmental Sciences, Lanzhou University, Lanzhou, China
- 5Group of Alpine Paleoecology and Human Adaptation (ALPHA), Institute of Tibetan Plateau Research, Chinese Academy of Sciences, Beijing, China
- 6State Key Laboratory of Tibetan Plateau Earth System, Resources and Environment (TPESRE), Beijing, China
A review of ethnological and archaeobotanical evidence shows the potential for a range of starch-rich woody plants, beyond tuberous plants, to have been important foods in prehistoric south subtropical China. In this paper we review the size and shape characteristics of starch granules non-tuberous woody plants (Palms, Cycads and Acorns) that our research has identified as important sources of carbohydrates for prehistoric communities. The study sample consists of 34 modern starch reference samples across eight genera (Palms: Arenga, Caryota,; Cycads:Cycas; and, Acorns: Castanopsis, Fagus, Lithocarpus, Quercus, and Quercus section Cyclobalanopsis). Our descriptive criteria are developed granule descriptors standard in the literature and then assessed for their utility using multiple correspondence analysis. The results demonstrate that both morphometric characteristics and the maximum size of granules are valuable for distinguishing starch granules at various taxonomic levels. Of the five morphometric characteristics recorded in this study sample, granule shape is the most effective variable for granule identification.
1 Introduction
Starch grain analysis contributes to the understanding of several archaeological issues including prehistoric diet (e.g. Loy et al., 1992; Barton 2005; Lu et al., 2005; Liu et al., 2010; Yang and Jiang 2010; Yao et al., 2016), tool use (e.g. Piperno et al., 2004; Revedin et al., 2010; Buckley et al., 2014; Ma et al., 2014) and the emergence of agriculture and plant domestication (e.g. Denham et al., 2003; Pearsall et al., 2004; Perry et al., 2007; Yang et al., 2012). To contribute to these fields, it is normally necessary to attempt a taxonomic identification of recovered starch granules by direct comparison with available reference material and sometimes with published granule descriptions from other studies (e.g. Torrence and Barton 2006; Yang and Perry 2013; Yao et al., 2016; Guan et al., 2020; Lucarini and Radini 2020; Tsafou and García-Granero 2021). A morphometric analysis by granule description is the most common approach (e.g. Loy 1994; Piperno and Holst 1998; Torrence and Barton 2006; Liu et al., 2014; Mercader et al., 2018; Brown and Louderback 2020), but some limitations are recognized including the experience of the analyst, the breadth of the reference collection, the state of granule preservation (e.g. Lamb and Loy 2005; Barton 2009; Yang and Perry 2013; Ma et al., 2019), and complications arising from a similarity of granule size and shape between species (e.g. Liu et al., 2014; Guan et al., 2020). The development of expert systems for granule identification, or at least an increased reliance on quantitative approaches that are not so dependent on subjective decisions made by the analyst, have been recommended (Torrence et al., 2004; Wilson et al., 2010; Liu et al., 2014; Coster and Field 2015; Arráiz et al., 2016; Louderback et al., 2016). To date, no expert system has replaced the need for an experienced analyst to identify granules at a taxonomic level. With the growing importance of this technique in archaeology, and the potential it has to resolve important questions about tool use and diet in prehistoric China, here we apply some statistical procedures to assess the reliability of our recording variables so that we may improve the reliability of identification of ancient starch granules from our study region of south subtropical China.
Starch residue analyses of stone tools from a range of archaeological sites in south subtropical China, dating between 10,000 and 2,000 BP, has already provided direct evidence for the critical horticultural hypothesis, which emphasized the importance of tuberous plants, such as taros (e.g. Colocasia spp.), yams (e.g. Dioscorea spp.), and lotus root (Nelumbo nucifera Gaertn), in the indigenous diet and agricultural system (e.g. Li and Lu 1987; Yin 2000; Lu 2003; Zhao 2005; Yang et al., 2013; Li et al., 2016). Our review of the available historical texts and some archaeological finds from southern China, however, reveal that some lesser-known starch-rich woody plants, including acorns, palms and cycads, may have also contributed significant calories to the communities in this region (Cao et al., 2011; Ge 2015; Geng 2019; Liu 1983; Liu et al., 2012; Zhou et al., 2014; Fuller and Qin 2010; Yang et al., 2013).
Edible acorns (nuts of the Fagaceae family) were once an important food staple for hunter-gatherer and farming communities in the northern hemisphere. Archaeological records document the consumption of large quantities of acorns in the woodlands of Europe, North Africa, Southeast Asia and North America (e.g. Fuller and Qin 2010; Higham 2014; Messner 2011; Humphrey et al., 2014; Kawashima 2016; Lentfer et al., 2013; Noshiro 2016; Sasaki and Noshiro 2018; Stevens and Mcelreath 2015; Tushingham and Bettinger 2013). Preserved acorn exocarps (the tough outer shell) have been recovered from several waterlogged sites in southern China, including the Tianluoshan site (7,000–6,000 BP) in the Yangtze River Basin and the Guye site (5,900–5,000 BP) in the Pearl River Delta (Fuller et al., 2011; Institute of Cultural Relics and Archaeology of Zhejiang Institute of Cultural Relics and Archaeology 2004; Yang et al., 2018). At the Tianluoshan site, acorns (Cyclobalanopsis/Lithocarpus/Quercus) were stored in special purpose subterranean storage pits (Fuller and Qin 2010). Acorn starches have been identified from the surfaces of stone tools at the Xiaohuangshan site (9,000–7,700 BP), charred residues inside the ceramic pottery at the Kuahuqiao site (8,000–7,000 BP), and dental calculus at the Qihe Cave site (c. 9,500 BP), indicating long-term use of this plant resource in the local and regional diets of southern China (Guan et al., 2018; Liu et al., 2010; Yang and Jiang 2010; Yao et al., 2016). However, acorns, as food staples, were gradually replaced by other crops, including domesticated rice (Fuller and Qin 2010). In the historic period, acorns remained important in seasonal diets, but were relegated to a supplementary food or famine food, as noted, for example, in Lu’s Commentaries of History (finished c. 239 BC), or the Compendium of Materia Medica (finished in 1578). Today, some ethnic minorities in southwest China, such as the Dong people and Wa people, still preserve the tradition of acorn consumption, albeit on a small scale (Supplementary Table S1) (Cao et al., 2011; Liu et al., 2012; Zhou et al., 2014).
Another important, though sidelined starchy plant foods in southern China is the palms which store starch in the trunk pith, recorded as Guanglang (桄榔), Suomu (莎木, the possible phonetic name of “sago”), and Mianmu (面木, meaning the trees with flour) (Ge 2015; Geng 2019; Lin 1997). The archaeological evidence for this foodstuff is confirmed only at the Xincun site in the Pearl River Estuary (5,300–4,400 BP) by recovered starch granules from at least two genera of palms (Caryota Lour. and Coryphya L.) (Yang et al., 2013). However, Chinese scholars have identified several genera of palms with edible pith, including guanglang (Arenga spp.), fishtail palms (Caryota spp.), as well as some species of cycads (Cycas spp.), from a series of Chinese historic texts (Ge 2015; Geng 2019; Lin 1997). For example, Hua Yang Guo Zhi (Eastern Jin Dynasty, 317–420 AD) recorded guanglang flour being consumed in the cities of the Yunnan-Guizhou Plateau where cereal crops were lacking. According to Shu Du Fu, this flour was already one of the critical commodities in the markets of the Sichuan basin, a region outside the natural range of these plants, at the time of the Wei-Jin dynasties (c. 220–315 AD), (Flora of China, online at: http://www.iplant.cn/info/%E6%A1%84%E6%A6%94?t=foc). This record shows the importance of this food, and that it was a traded commodity in some regions of southern China in the historic period. The Zhuang people in Longzhou, Guangxi still value guanglang flour as a traditional food and a medicinal supplement for treating diarrhoea (Ge 2015).
From the above review, we expand our range of important food plants in prehistoric southern China beyond roots and tubers to incorporate starchy palms, cycads and acorns, which may have been underestimated as important foods in previous studies. We therefore seek to expand our range of identifiable starches and look to the statistical analysis of modern reference materials to improve methods of identification as well as establish a reliable identification key for these species.
2 Materials and Methods
2.1 Modern Starch Reference Collections
The starch reference collection for this study comes from a total of 31 reference samples of oak trees (Fagaceae, n = 29) and two new collections of guanglang landraces (Arenga westerhoutii). These specimens are supplemented for analysis by species of palm (Caryota obtusa) and cycads (Cycas pectinata and Cycas panzhihuaensis) already included in the Chinese Modern Starch Reference Database held at the Institute of Geographical Sciences and Natural Resources Research, Beijing (Table 1).
The oaks, Fagaceae, were primarily obtained from the Herbarium of the Kunming Institute of Botany while some Fagus samples were provided by the Herbarium of the Institute of Botany, Chinese Academy of Science. We also collected some Quercus nuts in the field with botanists, such as Q. semecarpifolia nuts from the woodland behind the Huanglong Temple in Yunnan Province and Q. variabilis/Q. franchetii from the Kunming Botanical Garden (Table 1). With the help of botanists, we selected as “intact and mature” samples as possible for this study. All of them were identified to species level by botanists from the Kunming Institute of Botany, Chinese Academy of Sciences. We purchased two “landrace” samples of guanglang flour (Arenga) from local markets in Longzhou, Guangxi. The red sample is the reddish flour extracted from guanglang palm trees over 10 m in height, which have low starch content. It is said that this guanglang landrace is a rare wild landrace which is difficult to cultivate. The white flour is from high-yielding cultivated trees that grow to around five to 6 m tall. Other starch materials including Caryota and Cycas were analyzed from slides held in the China Modern Starch Grain Morphological Database.
2.2 Method of Extraction of Starches From Specimens
Following the protocols developed by Yang et al. (2009), we released the starch grains from the acorn kernels by the following method: 1) the nut, in a small plastic sealed bag, was broken with a hammer; 2) small pieces of broken nut were transported to new sterile test tubes with pure water for 24 h soaking; 3) after soaking, the samples were crushed with clean glass stirring rods to fully release the starches. The two purchased samples of guanglang palms were collected as ground flour. We dissolved 1 g flour into 20 ml of distilled water for the sample preparation.
All the starch solutions of acorns and guanglang flour were pipetted onto clean glass slides and mounted in a 50%/50% glycerine/water solution. The prepared slides were observed using a Zeiss AxioMAT at the Starch and Residue laboratory, School of Archaeology and Ancient History, University of Leicester. Images of the starch granules were taken with Zeiss AxioCam and Zeiss AxioVision software. For statistical purposes, a minimum of 100 granules of each sample were recorded. We measured the longest orientable measurement of each granule and recorded the following morphological features: granule shape; hilum position; presence/absence of fissures; the form of the fissure; presence or absence of lamellae; and surface texture (smooth/rough) (Supplementary Table S2).
2.3 Analyzing Method of Selected Variables
We observed all 34 samples including those newly collected for the database (Table 1), and expect at least 100 starch grains to be measured in each sample. If not enough granules were observed, we continued to sample until exhaustion of the sample itself. The collected data, including maximum granule length and the morphological variables (granule shapes, hilum position, lamellae visibility, fissure types, and surface texture) were recorded. The maximum granule sizes were measured using Zeiss Axiovision. We then tested the utility of granule size as a discriminating feature for starch identification statistically, despite the fact that much granule size overlap makes it difficult to use granule size as an independent discriminator. An assessment of the maximum granule sizes indicates that the values of most species tested do not have a normal distribution (Supplementary Table S4), violating the assumption required for parametric analysis (Wang 2013; Zhang 2014). Accordingly, we employ a non-parametric test to the dataset, the Kruskal–Wallis test for multiple independent samples. These statistical procedures are performed with SPSS v25.0.
We counted the proportion of each category of the recorded morphological variables to observe and summarize their distribution. In our descriptions of granule morphotypes, we follow the approach of previous studies (e.g. Torrence et al., 2004; Torrence and Barton 2006; Yang and Perry 2013; Liu et al., 2014; Mercader et al., 2018), including overall shape, fissure types, lamellae visibility, hilum position and surface texture (Figure 1 and Supplementary Table S2). We further use a geometric typology that separates overall granule shapes into four categories: irregular-shape type; ovate types; polygonal types; and faceted types. The ovate types include drop-shaped (an ovate with a wide shoulder and one pointed end; Figure 1B), pestle-shaped (an ovate with a shoulder at the hilum, tapering toward the distal end; Figure 1E), spindle-shaped (an ovate with two symmetrically pointed ends; Figure 1G), sphere and oval-shaped. And the faceted types are granules with a spherical or ellipsoid surface but abutting each other in the plant cell causing facets to form (Figure 1), including bell-shaped (Figure 1A), faceted spheres (Figure 1C), and spherical caps (Figure 1F). We then calculate the percentage of all the features observed in the starch samples at a species level, in order to identify diagnostic features.
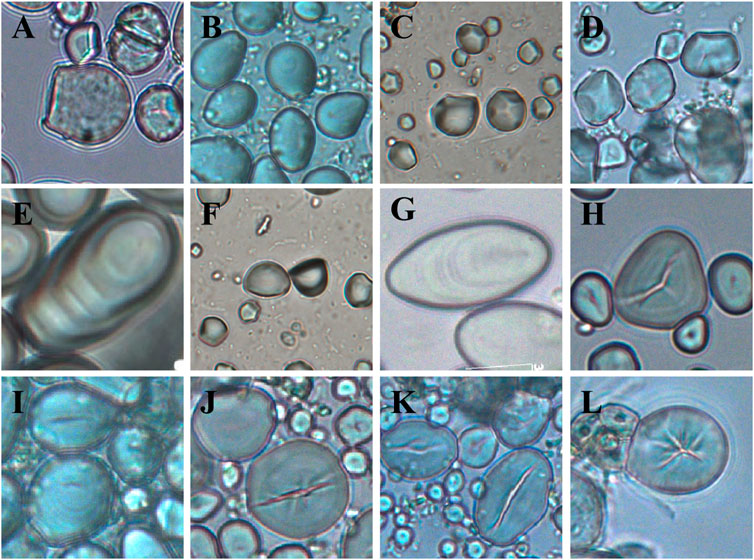
FIGURE 1. Some of the morphological features considered in this paper: (A) bell-shaped granule with rough surface (Cyclobalanopsis glauca); (B) drop-shaped granule (Quercus cocciferoides); (C) faceted spherical granule (Cycas pectinata); (D) irregular polyhedron granule (Castanopsis hystrix); (E) pestle-shaped granule with visible lamellae (Caryota obtusa); (F) spherical cap granule (Cycas pectinata); (G) spindle-shaped granule with visible lamellae (Arenga westerhoutii (red)); (H), triangle granule with v-shaped fissure (Quercus variabilis); (I) linear fissure; (J) and (k) longitudinal fissure; (L), stellate fissure.
Besides the granule description, we applied statistical procedures to reduce dependence on subjective decisions. As multivariate analysis has been effectively applied in previous starch reference analyses (Devaux et al., 1992; Liu et al., 2014; Torrence et al., 2004), we also select a multivariate analysis approach, multiple correspondence analysis (MCA), to analyse our morphometric data through SPSS v25.0. The MCA results are scatter plots in two dimensions (e.g. Figure 5), presenting a data set with categorized variables in a two-dimensional graph (Beh and Lombardo 2014; Devaux et al., 1992; Greenacre 2007; Le Roux and Rouanet 2005; Macheridis 2017). The variables (shown in the columns) are plant taxa, granule shape, fissure pattern, lamellae visibility, hilum position and surface texture, and each object (shown in the rows) represents an individual granule from our dataset. Inertia measures an approximate level of homogeneity within the dataset, the higher the value the greater the correspondence among the data (Greenacre 2007). The centroid of the result plot represents the average distribution in each row, which means that the closer the point comes to the centroid, the less different it is from the rest of the observations (Zhang and Dong 2013). The closer the distribution of the category points in the plot, the more relevant they are in the dataset (Franco 2016). The input dataset for the analysis of all productive species is a 2115∗6 matrix. As the main body of our samples is from Fagaceae samples, we therefore analyzed the Fagaceae samples separately. To avoid outliers, we exclude 107 rows where the shape features have high frequency in palm and cycad starches, as distributional patterns of these plots would give emphasis to these uncommon features (Greenacre 2007). Hence, a 1462∗6 matrix was analyzed using SPSS 25.0.
3 Results and Discussion
3.1 Broad Trends in the Observation Data
In our study sample, a total of 19 species, from seven genera, produced slides with over 100 granules (Table 1). We note that for 15 species of Fagaceae nuts, no, or only few, starch granules were recorded. The Lithocarpus chrysocomus sample released only one 15.89 μm granule (Figure 2: 11) and Fagus sylvatica produced only a few tiny agglomerated granules (Figure 2: 16). The lower starch content of some species, such as Fagus species, is in accordance with published data (Supplementary Table S3). Ida et al. (2017) tested the nutritional content of some edible beechnuts (Fagus spp.) which were once consumed in central Honshu, Japan, indicating that these nuts are high in fats, but low in carbohydrate. But there are some inconsistencies (Supplementary Table S3). For example, Lithocarpus corneus, which has been found to have a starch content of 47.78% of the kernel (Yang 2014), did not release any granules in our study (Table 1).
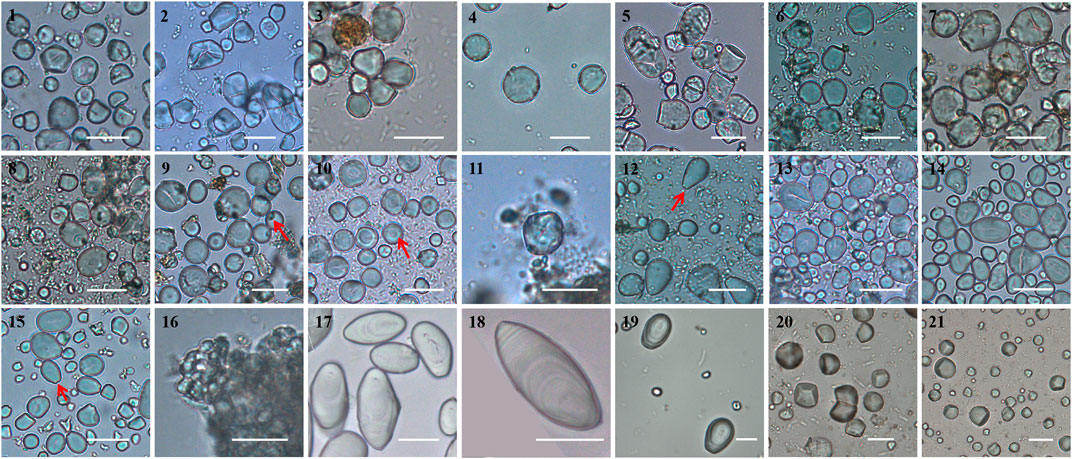
FIGURE 2. Starch granules from woody starch plants. 1–16, acorn kernels:1, Castanopsis platyacantha; 2, Castanopsis hystrix; 3, Castanopsis fargesii; 4, Castanopsis sclerophylla; 5, Cyclobalanopsis glauca; 6, Cyclobalanopsis chapensis; 7, Cyclobalanopsis gambleana; 8, Cyclobalanopsis phanera; 9, Lithocarpus cleistocarpus; 10, Lithocarpus litseifolius; 11, Lithocarpus chrysocomus; 12, Quercus cocciferoides; 13, Quercus oxyphylla; 14, Quercus variabilis; 15, Quercus franchetii; 16, Fagus sylvatica; 17–18, guanglang palm: 17, Arenga westerhouti (red); 18, Arenga westerhouti (white); 19, fishtail palm: Caryota obtusa; 20–21, cycads: 20, Cycas panzhihuaensis; 21, Cycas pectinata. Scale, 20 μm. The red arrows shows the pits in the granules.
From the productive starch samples, we first review the broad trends in the data, specifically the morphological data and granule size for each category of plant in our sample. In terms of maximum length, Lithocarpus cleistocarpus has the smallest starch range in our sample, both in terms of mean size (9.2 μm) and median size (8.7 μm). The starch granules of the Arenga landraces are much larger than those of other plants (Table 1). However, there is, generally, a lot of overlap in the sizes of our samples, especially of acorns, which may make it difficult to identify them by granule size alone (Figure 3 and Table 1).
The first feature we note is surface texture, which differs between acorn and palm/cycad starch granules (Figure 4). It is clear that all palm/cycad starch grains have smooth surfaces, whereas in the acorn samples, there are some granules with a rough surface (Figure 4). Among the starches examined, the palm granules are most likely to have visible lamellae. They also have a higher percentage of granules with an eccentric hilum. Conversely, the fissure patterns make it difficult to classify the granules recorded, as the majority do not bear any fissures (Figure 4).
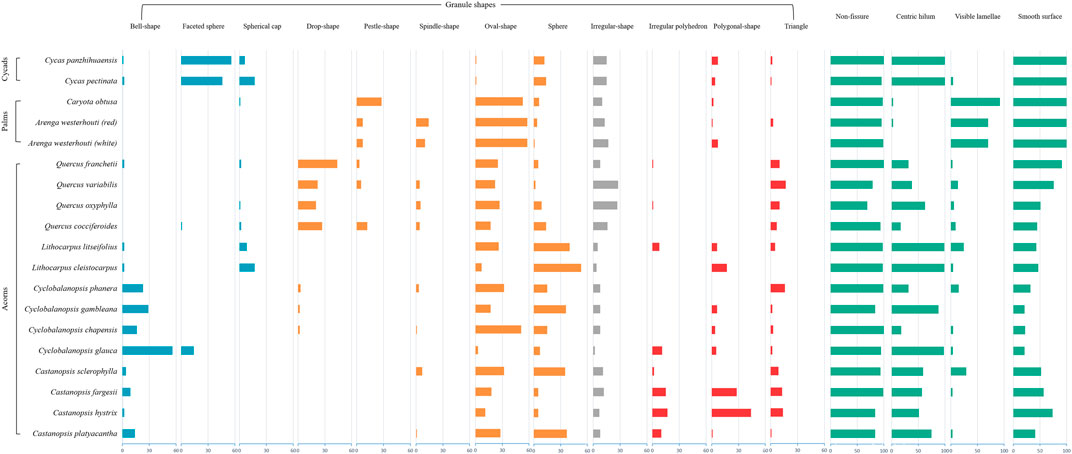
FIGURE 4. Percentage distribution of morphological features among plant species (Data shown in Supplementary Table S2).
We chart the four categories of granule shapes with different colors (Figure 4). The grey type includes irregular-shape granules. The faceted types are in blue, while the ovate-types in orange, the polygonal types (e.g. Figure 1D) in red. Of the facet types, bell-shaped granules are concentrated in the Cyclobalanopsisand Casntanospsis sample, especially Cyclobalanopsis glauca (Figure 2: 5) which accounts for 56% of its total sample (Figure 4 and Supplementary Table S2). The faceted spherical granules are mainly found in the cycad samples, which separately account for 46 and 56% of the study sample (Figure 4 and Supplementary Table S2). Spherical cap granules occur in small proportions in both Cycas and Lithocarpus. For ovate types, oval and spherical granules are widely distributed in every sample. Drop-shaped granules are primarily observed in Quercus while pestle-shaped and spindle-shaped granules are most frequent in palms. Most species produce some form of polygonal granules (Figure 4). However, we note an irregular type, with wavy edges or concave/convex surfaces (Figure 1D), mostly observed in Castanopsis. The two species of this genus, C. hystrix (Figure 2: 2) and C. fargesii (Figure 2: 3) also have a high percentage of normal polygonal granules.
3.2 Non-Parametric Test of Maximum Granule Size
Although the overlap in size of our samples cannot be ignored (Figure 3), the Kruskal–Wallis test indicates significant differences among the granule size data (H = 1422.199; df = 18; p < 0.05). Further pairwise comparison results (Table 2) reveal the differences in the size distributions. Combining the median granules size (Table 1) and adjusted significance values (Table 2), we first note the large-sized granule group containing Cycas panzhihuaensi and two landraces of Arenga (Figure 3, Group 3), for which the median size is larger than 20 microns (Table 1). However, there is no significant difference between the three samples. The group of two Lithocarpus samples and three Quercus samples (Figure 3, Group 1) have the same size distributions with a median size smaller than 10 microns (Table 1).
The remaining species produce medium-sized granules (Figure 3, Group 2), which can be roughly classified into two groups. The first contains five species, Castanopsis fargesii, Castanopsis platyacantha, Cyclobalanopsis chapensis, Quercus cocciferoides, and Castanopsis sclerophylla, which have similar size distributions, but can be distinguished from other species (Table 2). The second contains four species, Castanopsis hystrix, Cyclobalanopsis glauca, Cyclobalanopsis gambleana, and Caryota obtusa (Table 2). The median size of the former group (12.0–13.1 microns) is smaller than the latter (14.4–15.4 microns) (Table 1).
The size of Cyclobalanopsis phanera has a similar distribution to Quercus variabilis, Lithocarpus litseifolius, Castanopsis fargesii, and Castanopsis platyacantha, the median size of which is 11.0 microns, while Cycas pectinata (median size = 13.6 microns) has no statistically significant difference in size from the most abundant species (n = 9) (Table 2).
3.3 Multiple Correspondence Analysis of Morphological Data
3.3.1 Discrimination of all Starches at Species Level
The first-time analysis indicates that the variable fissure pattern has the least discrimination value within the dataset (Supplementary Table S5), which corresponds to our overview of the data (Figure 4). Hence, we exclude this column from the second analysis. The second-time analysis at species level shows the differences in our reference starches, with the first dimension explaining 52.3% of the inertia and the second dimension explaining 38.8% (Supplementary Table S5). The result plot (Figure 5) shows that we can distinguish species within the genus Fagaceae.
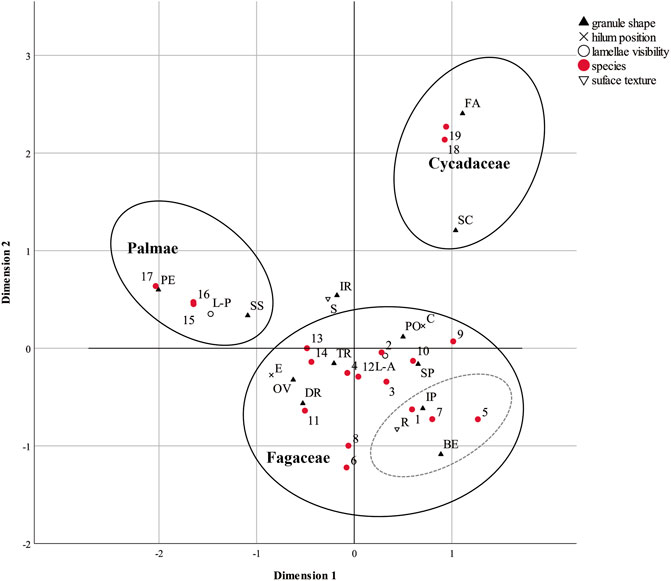
FIGURE 5. MCA result of morphometric data of starches at the species level. Labels: Species: 1, Castanopsis platyacantha; 2, Castanopsis hystrix; 3, Castanopsis fargesii; 4, Castanopsis sclerophylla; 5, Cyclobalanopsis glauca; 6, Cyclobalanopsis chapensis; 7, Cyclobalanopsis gambleana; 8, Cyclobalanopsis phanera; 9, Lithocarpus cleistocarpus; 10, Lithocarpus litseifolius; 11, Quercus cocciferoides; 12, Quercus oxyphylla; 13, Quercus variabilis; 14, Quercus franchetii; 15, Arenga westerhoutii (white); 16, Arenga westerhoutii (red); 18, Caryota obtusa; 18, Cycas pectinata; 19, Cycas panzhihuaensis. Shapes: BE: bell shape, DR: drop shape, FA: faceted sphere, IP: irregular polyhedron, IR: irregular shape, OV: oval shape, PE: pestle shape, PO: polygonal shape, SP: sphere, SC: spherical cap, SS: spindle shape, TR: triangle. Lamellae visibility: L-A: lamellae absence, L-P: lamellae presence. Surface texture: R: rough surface, S: smooth surface. Ellipses are not statistical, but hand-drawn to isolate key woody plant forms (Acorns, Cycads, Palms).
The Cycadaceae cluster is located in the first quadrant, i.e. the positive direction of both dimensions. This cluster contains two granule shapes, faceted spherical type and spherical cap type, indicating the close correlation between these and the two cycad samples. The other morphological features, according to the discrimination measures (Supplementary Table S5) and the point locations (Figure 5), which characterise the granules in this cluster are no lamellae, centric hilum, and smooth surfaces. These characteristics are fully consistent with our observations of the overall trends (Figure 4).
Along the first dimension, we note another cluster of three Palmae samples, with the points of pestle-shaped, spindle-shaped, and visible lamellae indicating the major morphological features of the Palmae granules (Figure 5). As this cluster is also located in the second quadrant, the Palmae granules are distinguishable by other variables, including those that strongly affect the negative direction of the first dimension, eccentric hilum, and the positive direction the second dimension, smooth surfaces. But the results of the two guanglang landraces show a high degree of consistency, so we cannot distinguish them further by their morphological features.
The points representing Fagaceae species are far away from the palm and cycad samples, revealing evident differences in morphological features (Figure 5). The most consistent difference is that the granules from edible acorns tend to have rough surfaces, which can be seen in the percentage distribution of the morphological features (Figure 4). We also note that the four Cyclobalanopsis species and Castanopsis platyacantha, in the dashed circle of Figure 5, may be distinguishable from other species, as they are relatively far away from the centroid. The granules may have a bell shape or irregular polygonal shape, with rough surfaces. However, the Fagaceae species are relatively close to the centroid of the plot, suggesting that they may represent the average features of our dataset. This may be because the sample of Fagaceae is larger than the other two genera. Hence, we decide to analyse the Fagaceae independently, to find their morphological differences.
3.3.2 Discrimination of Fagaceae Granules at Both Species Level and Genus Level
The analysis of the Fagaceae starches, at both the species level and the genus level, shows that the variables fissure type and lamellae visibility have weak discriminant value (Supplementary Table S5), consistent with Figure 4, that is, the Fagaceae starches commonly have no fissures or lamellae. Therefore, we focus on the variables of shape, hilum position and granule surface texture within the dataset which could be used to discriminate acorn starches.
The results at the species level show the differences in our reference starches, with the first dimension explaining 51.7% of the inertia and the second dimension explaining 41.0% (Supplementary Table S5). Of the two binary variables, based on the discrimination measures (Supplementary Table S5), dimension 1 is related to hilum position, i.e. species along the positive direction of dimension 1 tend to have centric hilum, while dimension 2 is surface texture. In this case, we note five rough clusters of Fagaceae starches in the plot (Figure 6A).
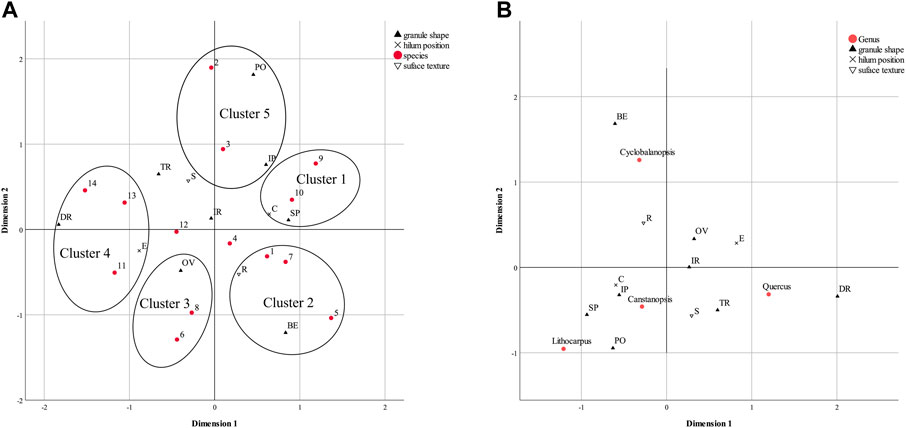
FIGURE 6. MCA result of morphometric data of Fagaceae starches: (A) at the species level; (B) at the genus level. Labels: Species: 1, Castanopsis platyacantha; 2, Castanopsis hystrix; 3, Castanopsis fargesii; 4, Castanopsis sclerophylla; 5, Cyclobalanopsis glauca; 6, Cyclobalanopsis chapensis; 7, Cyclobalanopsis gambleana; 8, Cyclobalanopsis phanera; 9, Lithocarpus cleistocarpus; 10, Lithocarpus litseifolius; 11, Quercus cocciferoides; 12, Quercus oxyphylla; 13, Quercus variabilis; 14, Quercus franchetii. Shapes: BE: bell-shape, DR: drop-shape, FA: faceted sphere, IP: irregular polyhedron, IR: irregular shape, OV: oval shape, PE: pestle shape, PO: polygonal shape, SP: sphere, SC: spherical cap, SS: spindle-shape, TR: triangle. Lamellae visibility: L–A: lamellae absence, L–P: lamellae presence. Surfaces texture: R: rough surface, S: smooth surface.
Cluster 1 contains the points for two Lithocarpus species with spherical granules. This indicates a correspondence between spherical granules and these two species. These granules also have a centric hilum and smooth surface. Cluster 2 shows the associations between bell-shaped granules and the species Castanopsis platyacantha, Cyclobalanopsis glauca and Cyclobalanopsis gambleana. These granules tend to have a centric hilum but rough surface. Along dimension 1, there are two clusters in the negative direction, representing granules with eccentric hilum. Cluster 3 indicates that the granules of Cyclobalanopsis chapensis and Cyclobalanopsis phanera are similar in morphology, characterized as oval with rough surfaces. Cluster 4 includes Quercus cocciferoides, Q. variabilis and Q. franchetii. These granules mainly have a drop shape but can be classified further. Compared to the other two species, the starches of Q. cocciferoides are more likely to have rough surfaces, consistent with the percentage calculation (Figure 4).
Cluster 5 is located in the positive direction of the second dimension, representing an overall trend for granules with smooth surfaces. This cluster contains two species of Castanopsis, C. hystrix and C. fargesii, and has polygonal features, showing their correspondence. According to the point locations, C. hystrix tends to produce normal polygonal granules, but C. fargesii may produce irregular types. This result is consistent with the trends represented by the granule shape percentages. We note that the C. hystrix granules may have a higher occurrence of an eccentric hilum. Based on the percentage calculation (Supplementary Table S2), the difference in this variable between these two species is minor.
The result at the genus level (Figure 6B) show that discrimination is possible at this taxonomic level, with the first dimension explaining 48.8% of the inertia and the second dimension explaining 38.6% (Supplementary Table S5). In the results plot, the feature hilum position, which has a large measure of discrimination in the first dimension, distinguishes Quercus from others, specifically, Quercus granules have a higher probability of having an eccentric hilum than the other three genera. Similarly, Cyclobalanopsis starches are more likely to have rough surfaces.
This result also reveals the most identifiable shape features of the four Fagaceae genera. The identification keys include bell-shaped granules for Cyclobalanopsis and drop-shaped granules for Quercus. The Castanopsis and Lithocarpus granules seem to be similar in shape, irregular polyhedrons, polygons and spheres. However, according to the percentage distribution of the shape features (Figure 4), we suggest that spherical granules are common in Lithocarpus starch sample, while polygonal granules, especially irregular types, are Castanopsis. This result matches our previous analysis of morphological characteristics at the species level.
4 Identification Scheme of Woody Plant Starches
The identification of productive woody starch granules in this study combines two methods of mutual verification: a traditional morphmetric analysis made by an expert and automated computer-based statistical analyses. The statistical analyses confirms that both the numerical variables of maximum granule size and nominal morphological variables are useful to discriminate starches of various plant taxa, supporting previous attempts to identify plants using a morphometric method (e.g. Torrence et al., 2004; Yang and Perry 2013; Liu et al., 2014; Coster and Field 2015). Non-parametric tests statistically evaluate the distribution differences of granule sizes between the samples examined. Multiple correspondence analysis can be used to visualize the correlation between morphometric data and plant taxa in a two-dimensional plot and calculate the contribution of each categorized variable, which can help an analyst select those that are most critical for discriminating starches.
In our cases, the fissure types of the starches from woody plants make the least contribution in starch identification. However, we can reasonably identify granules from tested edible acorn kernels, cycad pith and palm pith in southern China on the basis of size and other morphological features. The identification scheme is summarized as follows.
(1) The spindle-shaped or pestle-shaped granules, with eccentric hilum, clear visible lamellae, smooth surface and non-fissure are the typical starches from starchy palm pith including Caryota obtuse (Figure 2:19) and the two landrace samples of Arenga westerhoutii (Figure 2:17–18). The size data can be used to make an inter-genus distinction, as the maximum granule sizes of the two guanglang landraces (mean size = 26.4/29.5 microns) are statistically larger than those of fishtail palm (mean size = 18.1 microns) (Table 1).
(2) The diagnostic morphological features of the two Cycas species are fully identical. They have faceted sphere or spherical cap shapes with a smooth surface and centric hilum, but without any fissures or visible lamellae (Figure 2: 20–21). However, the two Cycas are statistically different in size, with granules from C. panzhihuaensis (mean size = 20.6 microns) larger than those from C. pectinata (mean size = 14.1 microns) (Table 1), which can be used to make an inter-species identification.
(3) Starch granules from acorn kernels are more likely to have rough surfaces and various morphological features by comparison. Based on our analyses, though, we can discriminate further at genus level.
(a) Quercus granules (Figure 2:12–15) are most distinguishable within the acorn starches, as they have a typical granule form, which has drop shape, eccentric hilum, but no fissures or visible lamellae. In our observations, some of them have a small pit at their pointed ends (Figure 2:12), making them more identifiable. Among the species collected, Q. cocciferoides are distinguishable, because the maximum granule size of this species (mean size = 13.1 microns) is statistically larger than the other three species (mean size = 10.0/10.1/10.2 microns) which are in the smallest granule sample of our dataset.
(b) A rough bell-shape granule with centric hilum is the most identifiable type of Cyclobalanopsis at the genus level (Figure 2: 5–8). Of the four species studied, the starch samples of C. glauca (Figure 2: 5) and C. gambleana (Figure 2: 7) are dominated by these typical granules. The maximum size data do not show a statistically significant difference between them (Table 2). The other two species, C. chapensis (Figure 2: 6) and C. phanera (Figure 2: 8), have identifiable granules, but are more likely to produce oval granules, according to both traditional analyses (Figure 4) and the MCA results (Figure 6A). Their granule sizes are also smaller than the former two species, of which C. phanera is the smallest (mean size = 11.5 microns).
(c) The granules of Lithocarpus are statistically smaller (with size medians smaller than 10 microns) (Figure 3), while the two species L. litseifolius and L. cleistocarpus cannot be distinguished by size. They are also similar in morphology, having spherical shapes, centric hilum and smooth surfaces, without any fissures or visible lamellae (Figure 2: 9–10). Such granule form seems to be widespread in many other plants, for example, lotus seeds (Nymphaea tetragone) (Wan et al., 2011). Therefore, we return to the granule images, and note that there is a small pit at the centre of the granule (Figure 2: 10) which may help to distinguish this genus.
(d) The Castanopsis granules (Figure 2: 1–4) are characterized by irregular polygonal shapes, with centric hilum and smooth surfaces, although the percentage distribution of shape features suggests that this is not the major form in some species (Figure 4). On the basis of size, C. hystrix granules (mean size = 14.3 microns) are larger than those of the other three Castanopsis species.
The study shows that it is possible to identify starches among plant taxa using both numeric size data and nominal morphological features. Traditional identification is effective for setting up an identification scheme, and multiple correspondence analysis applied to morphological data is also a useful approach in the early stage of discriminating identifiable features of modern reference collections.
Data Availability Statement
The raw data supporting the conclusions of this article will be made available by the authors, without undue reservation.
Author Contributions
Conceived and designed the experiments: HB and XY. Performed the experiments: ZL and WW. Analyzed the data: ZL, HB and XY. Contributed reagents/materials/analysis tools: XY and HB. Wrote the paper: ZL, HB and XY. Collected the samples in fieldwork: ZL, WW and XY.
Funding
Funding for this research was provided by National Natural Science Foundation of China (Grant No. 41771231 and 4137121) and China Scholarship Council (201604910602).
Conflict of Interest
The authors declare that the research was conducted in the absence of any commercial or financial relationships that could be construed as a potential conflict of interest.
Publisher’s Note
All claims expressed in this article are solely those of the authors and do not necessarily represent those of their affiliated organizations, or those of the publisher, the editors and the reviewers. Any product that may be evaluated in this article, or claim that may be made by its manufacturer, is not guaranteed or endorsed by the publisher.
Acknowledgments
We thank Prof. Changjiang Liu from Institute of Botany, Chinese Academy of Sciences for providing Fagus nuts; Lu Li from Southwest Forestry University for other modern sample collection in Yunan and identification some modern samples to species. We also thank the University of Leicester School of Archaeology and Ancient History Starch and Residue Laboratory.
Supplementary Material
The Supplementary Material for this article can be found online at: https://www.frontiersin.org/articles/10.3389/feart.2022.815351/full#supplementary-material
Footnotes
1Recent molecular studies of the oak grouping, Cyclobalanopsis, has revised this Southeast Asian group to the section level within the genus Quercus (see Denk et al., 2017; Hipp et al., 2020; Zhou et al., 2022). As we understand it, the use of the term Cyclobalanopsis refers in part, to the particular phenotypic expression of acorns in having acorns with distinctive cups bearing concrescent rings of scales; they commonly also have densely clustered acorns, though this does not apply to all of the species. Phenotypic differences will have mattered to hunter-gatherers collecting these plantswhile they may have processed and eaten them in similar ways to all other Quercus, the visual differences in the acorns, while not significant in terms of their molecular evolution, are likely to have featured in ethno-taxonomies. Differences in growth habitat will also have mattered to hunter-gatherers and be significant in our consideration of their use as a food in prehistory. To align our paper with previous studies and to reduce confusion in comparisons of starch granules, we continue to refer to Cyclobalanopsis sp. in this paper, while recognising it is technically inaccurate in current botanical scholarship.
References
Arráiz, H., Barbarin, N., Pasturel, M., Beaufort, L., Domínguez-Rodrigo, M., and Barboni, D. (2016). Starch Granules Identification and Automatic Classification Based on an Extended Set of Morphometric and Optical Measurements. J. Archaeol. Sci. Rep. 7, 169–179. doi:10.1016/j.jasrep.2016.03.039
Barton, H. (2009). “Starch Granule Taphonomy: the Results of a Two Year Field Experiment,” in Archaeological Science under a Microscope: Studies in Residue and Ancient DNA Analysis in Honour of Thomas H. Loy. Editors M. Haslam, G. Robertson, A. Crowther, S. Nugent, and L. Kirkwood (Canberra: ANU E Press), 129–140. doi:10.22459/ta30.07.2009.09
Barton, H. (2005). The Case for Rainforest Foragers: the Starch Record at Niah Cave, Sarawak. Asian Perspect. 44, 56–72. doi:10.1353/asi.2005.0005
Beh, E. J., and Lombardo, R. (2014). Correspondence Analysis: Theory, Practice and New Strategies. Chichester: John Wiley & Sons.
Brown, G. H., and Louderback, L. A. (2020). Identification of Starch Granules from Oak and Grass Species in the Central Coast of California. J. Archaeol. Sci. Rep. 33, 102549. doi:10.1016/j.jasrep.2020.102549
Buckley, S., Usai, D., Jakob, T., Radini, A., and Hardy, K. (2014). Dental Calculus Reveals Unique Insights into Food Items, Cooking and Plant Processing in Prehistoric Central Sudan. PLOS ONE 9, e100808. doi:10.1371/journal.pone.0100808
Cao, L., Xu, H., and Xi, S. (2011). Ethnobotanical Research on Edible Plants of Gannan the Hakkas in Jiangxi Province. J. Gannan Normal Univ. 3, 53–56. doi:10.1016/j.jep.2008.01.016
Coster, A. C. F., and Field, J. H. (2015). What Starch Grain Is that? A Geometric Morphometric Approach to Determining Plant Species Origin. J. Archaeol. Sci. 58, 9–25. doi:10.1016/j.jas.2015.03.014
Denham, T. P., Haberle, S. G., Lentfer, C., Fullagar, R., Field, J., Therin, M., et al. (2003). Origins of Agriculture at Kuk Swamp in the Highlands of New Guinea. Science 301, 189–193. doi:10.1126/science.1085255
Denk, T., Grimm, G. W., Manos, P. S., Deng, M., and Hipp, A. L. (2017). “An Updated Infrageneric Classification of the Oaks: Review of Previous Taxonomic Schemes and Synthesis of Evolutionary Patterns,” in Oaks Physiological Ecology. Exploring the Functional Diversity of Genus Quercus L. Editors E. Gil-Pelegrín, J. J. Peguero-Pina, and D. Sancho-Knapik (Cham, Switzerland: Springer International Publishing AG). doi:10.1007/978-3-319-69099-5_2
Devaux, M. F., Qannari, E. M., and Gallant, D. J. (1992). Multiple-correspondence Analysis Optical Microscopy for Determination of Starch Granules. J. Chemom. 6, 163–175. doi:10.1002/cem.1180060307
Di Franco, G. (2016). Multiple Correspondence Analysis: One Only or Several Techniques? Qual. Quant. 50, 1299–1315. doi:10.1007/s11135-015-0206-0
Fuller, D., Qin, L., Zhao, Z., Zheng, Y., Hosoya, L., Chen, X., et al. (2011). “Archaeobotanic Analysis for the Tianluoshan Site,” in The Center for the Study of Chinese Archaeology in Peking University and Institute of Cultural Relics and Archaeology of ZhejiangA Comprehensive Study on the Natural Remains of Tianluoshan Site (Beijing: Cultural Relics Press), 47–96.
Fuller, D. Q., and Qin, L. (2010). Declining Oaks, Increasing Artistry, and Cultivating Rice: the Environmental and Social Context of the Emergence of Farming in the Lower Yangtze Region. Environ. Archaeol. 15, 139–159. doi:10.1179/146141010x12640787648531
Ge, W. (2015). Study on the Use of Guanglang (Sago-type Palms) Among Ethnic Groups in South china. Agric. Archaeol. 6, 253–259.
Geng, Z. (2019). Study on the Policy Adjustment of Ancient Staple Crops and Environmental Changes: Case Study of Guanglang Palms (Sago-type Palms) in the Southern Areas of China. China Agric. Univ. J. Soc. Sci. Ed. 36, 109–115. doi:10.13965/j.cnki.gzmzyj10026959.2020.12.014
Greenacre, M. J. (2007). Correspondence Analysis in Practice. second edition. Boca Raton: CRC Press.
Guan, Y., Tian, C., Peng, F., Guo, J., Wang, H., Zhou, Z., et al. (2020). Plant Diet during the Pleistocene-Holocene Transition in Northwest China: Evidence from Starch Remains from Pigeon Mountain Site in Ningxia Province. Quat. Int. 559, 110–118. doi:10.1016/j.quaint.2020.03.016
Guan, Y., Zhou, Z., Fan, X., and Gao, X. (2018). Neolithic Human Diet Revealed by the Dental Residues in West Fujian. Acta Anthropol. Sin. 37, 631–639. doi:10.16359/j.cnki.cn111963/q.2017.0056
Higham, C. (2014). Early Mainland Southeast Asia: From First Humans to Angkor. Bangkok: River Books Press.
Hipp, A. L., Manos, P. S., Hahn, M., Avishai, M., Bodénès, C., Cavender‐Bares, J., et al. (2020). Genomic Landscape of the Global Oak Phylogeny. New Phytol. 226, 1198–1212. doi:10.1111/nph.16162
Humphrey, L. T., De Groote, I., Morales, J., Barton, N., Collcutt, S., Bronk Ramsey, C., et al. (2014). Earliest Evidence for Caries and Exploitation of Starchy Plant Foods in Pleistocene Hunter-Gatherers from Morocco. Proc. Natl. Acad. Sci. U.S.A. 111, 954–959. doi:10.1073/pnas.1318176111
Ida, H., Takeuchi, S., and Takasaki, S. (2017). Beechnuts as a Local Specialty in a Snowy Semi-mountainous Area in Central Japan Based on Their Production and Nutritional Traits. J. Jpn. For. Soc. 99 (1), 10–17. doi:10.4005/jjfs.99.10
Kawashima, T. (2016). Food Processing and Consumption in the Jōmon. Quat. Int. 404, 16–24. doi:10.1016/j.quaint.2015.08.040
Lamb, J., and Loy, T. (2005). Seeing Red: the Use of Congo Red Dye to Identify Cooked and Damaged Starch Grains in Archaeological Residues. J. Archaeol. Sci. 32, 1433–1440. doi:10.1016/j.jas.2005.03.020
Le Roux, B., and Rouanet, H. (2005). Multiple Correspondence Analysis (MCA). InGeometric Data Analysis. Dordrecht: Springer.
Lentfer, C., Matthews, P., Gosden, C., Lindsay, S., and Specht, J. (2013). Prehistory in a Nutshell: a Lapita-Age Nut-Cracking Stone from the Arawe Islands, Papua New Guinea. Archaeol. Ocean. 48, 121. doi:10.1002/arco.5008
Li, G., and Lu, X. (1987). The Original Agriculture Form of Ethnic Minorities in South China (Beijing. Beijing: Agriculture Press).
Li, Z., Zhao, J., Li, Q., Ma, Z., Liu, C., Shang, J., et al. (2016). Study on Plant Remains from the Shixiongshan Site of Qin-Han Dynasties in Hilly Area of Guangdong Province. Quat. Sci. 36 (5), 1253–1262. doi:10.11928/j.issn.1001-7410.2016.05.20
Lin, H. (1997). The Study of Cycas Plants in Chinese Historic Documents. Agric. Hist. China 16, 96–102.
Liu, C., Du, F., Wang, J., Guo, S., Xi, Z., and Leng, T. (2012). An Ethnobotanical Study on the Wild Edible Plant Resources of Wa Nationality. J. West China For. Sci. 41, 42–49. doi:10.16473/j.cnki.xblykx1972.2012.05.001
Liu, L., Field, J., Weisskopf, A., Webb, J., Wang, H., and Chen, X. C. (2010). The Exploitation of Acorn and Rice in Early Holocene Lower Yangzi River china. Acta Anthropol. Sin. 29, 317–336. doi:10.16359/j.cnki.cn111963/q.2010.03.006
Liu, L., Ma, S., and Cui, J. (2014). Identification of Starch Granules Using a Two-step Identification Method. J. Archaeol. Sci. 52, 421–427. doi:10.1016/j.jas.2014.09.008
Louderback, L. A., Herzog, N. M., and Pavlik, B. M. (2016). A New Approach for Identifying Starch Granules of Wild Food Plants from Arid Western North America. Starch – Stärke 68, 1–7. doi:10.1002/star.201600167
Loy, T. H., Spriggs, M., and Wickler, S. (1992). Direct Evidence for Human Use of Plants 28,000 Years Ago: Starch Residues on Stone Artefacts from the Northern Solomon islands. Antiquity 66, 898–912. doi:10.1017/s0003598x00044811
Loy, T. (1994). “Methods in the Analysis of Starch Residues on Prehistoric Stone Tools,” in Tropical Archaeobotany. Editor J. Hather (London: Routledge), 86–114.
Lu, H., Yang, X., Ye, M., Liu, K.-B., Xia, Z., Ren, X., et al. (2005). Millet Noodles in Late Neolithic China. Nature 437, 967–968. doi:10.1038/437967a
Lu, L. (2003). “A Preliminary Analysis for the Residues on the Surface of Unearthed Stone Tools in Zengpiyan Site,” in Institute of Archaeology, Chinese Academy of Social Sciences, Antiquities Task Force in Guangxi Zhuang Autonomous Region, Museum of Zengpiyan Site in Guilin, and Antiquities Task Force in GuilinGuilin Zengpiyan. (Beijing: Cultural Relics Press), 646–651.
Lucarini, G., and Radini, A. (2020). First Direct Evidence of Wild Plant Grinding Process from the Holocene Sahara: Use-Wear and Plant Micro-residue Analysis on Ground Stone Tools from the Farafra Oasis, Egypt. Quat. Int. 555, 66–84. doi:10.1016/j.quaint.2019.07.028
Ma, Z., Perry, L., Li, Q., and Yang, X. (2019). Morphological Changes in Starch Grains after Dehusking and Grinding with Stone Tools. Sci. Rep. 9, 2355–2413. doi:10.1038/s41598-019-38758-6
Ma, Z., Li, Q., Huan, X., Yang, X., Zheng, J., and Ye, M. (2014). Plant Microremains Provide Direct Evidence for the Functions of Stone Knives from the Lajia Site, Northwestern China. Chin. Sci. Bull. 59, 1151–1158. doi:10.1007/s11434-014-0174-0
Macheridis, S. (2017). The Use of Multiple Correspondence Analysis (MCA) in Taphonomy: the Case of Middle Helladic Asine, Greece. Int. J. Osteoarchaeol. 27, 477–487. doi:10.1002/oa.2571
Mercader, J., Abtosway, M., Bird, R., Bundala, M., Clarke, S., Favreau, J., et al. (2018). Morphometrics of Starch Granules from Sub-saharan Plants and the Taxonomic Identification of Ancient Starch. Front. Earth Sci. 6, 146. doi:10.3389/feart.2018.00146
Messner, T. C. (2011). Acorns and Bitter Roots Starch Grain Research in the Prehistoric Eastern Woodlands. (Tuscaloosa: University of Alabama Press).
Noshiro, S. (2016). Change in the Prehistoric Use of Arboreal Resources in japan: From Their Sophisticated Management in the Jomon Period to Their Intensive Use in the Yayoi to Kofun Periods. Quat. Int. 397, 484–494. doi:10.1016/j.quaint.2015.05.037
Pearsall, D. M., Chandler-Ezell, K., and Zeidler, J. A. (2004). Maize in Ancient Ecuador: Results of Residue Analysis of Stone Tools from the Real Alto Site. J. Archaeol. Sci. 31, 423–442. doi:10.1016/j.jas.2003.09.010
Perry, L., Dickau, R., Zarrillo, S., Holst, I., Pearsall, D. M., Piperno, D. R., et al. (2007). Starch Fossils and the Domestication and Dispersal of Chili Peppers ( Capsicum Spp. L.) in the Americas. Science 315, 986–988. doi:10.1126/science.1136914
Piperno, D. R., and Holst, I. (1998). The Presence of Starch Grains on Prehistoric Stone Tools from the Humid Neotropics: Indications of Early Tuber Use and Agriculture in Panama. J. Archaeol. Sci. 25, 765–776. doi:10.1006/jasc.1997.0258
Piperno, D. R., Weiss, E., Holst, I., and Nadel, D. (2004). Processing of Wild Cereal Grains in the Upper Palaeolithic Revealed by Starch Grain Analysis. Nature 430, 670–673. doi:10.1038/nature02734
Revedin, A., Aranguren, B., Becattini, R., Longo, L., Marconi, E., Lippi, M. M., et al. (2010). Thirty Thousand-Year-Old Evidence of Plant Food Processing. Proc. Natl. Acad. Sci. U.S.A. 107, 18815–18819. doi:10.1073/pnas.1006993107
Sasaki, Y., and Noshiro, S. (2018). Did a Cooling Event in the Middle to Late Jomon Periods Induced Change in the Use of Plant Resources in japan? Quat. Int. 471, 369–384. doi:10.1016/j.quaint.2017.10.037
Stevens, N. E., and Mcelreath, R. (2015). When Are Two Tools Better Than One? Mortars, Millingslabs, and the California Acorn Economy. J. Anthropol. Archaeol. 37, 100–111. doi:10.1016/j.jaa.2014.12.002
Torrence, R., Wright, R., and Conway, R. (2004). Identification of Starch Granules Using Image Analysis and Multivariate Techniques. J. Archaeol. Sci. 31, 519–532. doi:10.1016/j.jas.2003.09.014
Tsafou, E., and García-Granero, J. J. (2021). Beyond Staple Crops: Exploring the Use of ‘invisible’ Plant Ingredients in Minoan Cuisine through Starch Grain Analysis on Ceramic Vessels. Archaeol. Anthropol. Sci. 13, 1–16. doi:10.1007/s12520-021-01375-4
Tushingham, S., and Bettinger, R. L. (2013). Why Foragers Choose Acorns before Salmon: Storage, Mobility, and Risk in Aboriginal California. J. Anthropol. Archaeol. 32, 527–537. doi:10.1016/j.jaa.2013.09.003
Wan, Z., Yang, X., Ge, Q., and Jiang, M. (2011). Morphological Characeteristics of Starch Grains of Root and Tuber Plant in South China. Quat. Sci. 31 (4), 736–745. doi:10.3969/j.issn.10017410.2011.04.18
Wilson, J., Hardy, K., Allen, R., Copeland, L., Wrangham, R., and Collins, M. (2010). Automated Classification of Starch Granules Using Supervised Pattern Recognition of Morphological Properties. J. Archaeol. Sci. 37, 594–604. doi:10.1016/j.jas.2009.10.024
Yang, J., Ge, J., Liu, L., Ding, Y., and Tan, Y. (2009). Gap Phase Regeneration Recruitment of Mixed Conifer-Broadleaf Forests in Wolong Nature Reserve. Front. For. China 4, 153–158. doi:10.1007/s11461-009-0031-5
Yang, S. (2014). The Research and Exploitation of Starch Plants of Fagaceae in China. Jiangsu Agric. Sci. 42 (05), 324–327. doi:10.15889/j.issn.10021302.2014.05.004
Yang, X., Barton, H. J., Wan, Z., Li, Q., Ma, Z., Li, M., et al. (2013). Sago-type Palms Were an Important Plant Food Prior to Rice in Southern Subtropical China. PLoS ONE 8, e63148. doi:10.1371/journal.pone.0063148
Yang, X., and Jiang, L. (2010). Starch Grain Analysis Reveals Ancient Diet at Kuahuqiao Site, Zhejiang Province. Chin. Sci. Bull. 55, 1150–1156. doi:10.1007/s11434-009-0545-0
Yang, X., Li, Z., Wang, W., and Cui, Y. (2018). South Dispersal of Rice Farming: the Beginning Time and Subsistence Background of Rice Farming in Lingnan Region. J. Archaeol. Museology 01, 33–47.
Yang, X., and Perry, L. (2013). Identification of Ancient Starch Grains from the Tribe Triticeae in the North china Plain. J. Archaeol. Sci. 40, 3170–3177. doi:10.1016/j.jas.2013.04.004
Yang, X., Zhang, J., Perry, L., Ma, Z., Wan, Z., Li, M., et al. (2012). From the Modern to the Archaeological: Starch Grains from Millets and Their Wild Relatives in China. J. Archaeol. Sci. 39, 247–254. doi:10.1016/j.jas.2011.09.001
Yao, L., Yang, Y., Sun, Y., Cui, Q., Zhang, J., and Wang, H. (2016). Early Neolithic Human Exploitation and Processing of Plant Foods in the Lower Yangtze River, China. Quat. Int. 426, 56–64. doi:10.1016/j.quaint.2016.03.009
Yin, S.Kunming (2000). Man and Forest: Swidden Agriculture in the Perspective of Ecological Anthropology. (Kunming: Yunnan Education Publishing House).
Zhang, L. (2014). Principles of the Kruskal-Wallis Test and its Empirical Analysis. J. Suzhou Univ. Sci. Technol. Nat. Sci. 1, 14–15. doi:10.3969/j.issn.16720687.2014.01.003
Zhang, W., and Dong, W. (2013). Advanced Tutorials of Statistical Analyses Using SPSS. Beijing: Higher Education Press.
Zhao, Z. (2005). Archaeobotanical Studies on the Origin of Agriculture and Civilization. Soc. Sci. Manag. Comment 2, 82–91.
Zhejiang Institute of Cultural Relics and Archaeology (2004). Zhejiang Provincial Institute of Cultural Relics and Archaeology and Xiaoshan Museum. Beijing: Cultural Relics Press, 241–270.
Zhou, B.-F., Yuan, S., Crowl, A. A., Liang, Y.-Y., Shi, Y., Chen, X.-Y., et al. (2022). Phylogenomic Analyses Highlight Innovation and Introgression in the Continental Radiations of Fagaceae across the Northern Hemisphere. Nat. Commun. 13, 1320. doi:10.1038/s41467-022-28917-1
Keywords: starch identification, non-parametric test, multiple correspondence analysis, woody plants, southern China
Citation: Li Z, Barton H, Wang W and Yang X (2022) Description of Starch Granules From Edible Acorns (Oak), Palms, and Cycads in Southern China. Front. Earth Sci. 10:815351. doi: 10.3389/feart.2022.815351
Received: 15 November 2021; Accepted: 26 April 2022;
Published: 16 May 2022.
Edited by:
David K. Wright, University of Oslo, NorwayReviewed by:
Anders Lindstrom, Nong nooch botanical garden, ThailandThomas Hart, Franklin and Marshall College, United States
Copyright © 2022 Li, Barton, Wang and Yang. This is an open-access article distributed under the terms of the Creative Commons Attribution License (CC BY). The use, distribution or reproduction in other forums is permitted, provided the original author(s) and the copyright owner(s) are credited and that the original publication in this journal is cited, in accordance with accepted academic practice. No use, distribution or reproduction is permitted which does not comply with these terms.
*Correspondence: Huw Barton, aGpiMTVAbGVpY2VzdGVyLmFjLnVr; Xiaoyan Yang, eHlhbmdAbHp1LmVkdS5jbg==