- 1Department of Geological Sciences, University of Cape Town, Cape Town, South Africa
- 2Sociedad Chilena de Arqueología, Santiago de Chile, Chile
- 3Departamento de Antropología, Universidad de Chile, Santiago de Chile, Chile
- 4Bruker Nano GmbH, Berlin, Germany
The analysis of archaeological ceramics has rapidly evolved over the last decades by the application of new analytical techniques. An emerging analytical proposal to fully characterise archaeological ceramics using automated SEM mineralogy is presented. A case study is provided of sets of ceramics from the San Pedro de Atacama oases in the Atacama Desert, northern Chile. Ceramic fragments of different typologies (i.e., Los Morros, Loa Café Alisado and San Pedro Negro Pulido) found in the Ghatchi archaeological sites are analysed. Our results include automated mineralogical maps, which are used to define the components that form the ceramic pastes, i.e., clay matrix and non-plastic inclusions, as well as grain size and mineral abundance information. We show that the pastes that define the studied ceramic types are more complex than previously suggested. The overall composition for these pastes corresponds to clay mineral-rich matrices containing non-plastic inclusions, such as mineral grains, crushed ceramic fragments, and sedimentary to igneous rock fragments, that may vary in composition, size, and abundance among the studied ceramic types. This mineralogical information allows us to discuss possible sources of raw materials by comparing these paste components with geological information. Here we interpret Los Morros and Loa Café Alisado as foreign ceramic types to Ghatchi, whereas the San Pedro Negro Pulido fragments found in this site agree well with the pottery paste recipe typically recognised in the San Pedro de Atacama oases. The petrographic-approach employed here supports the automated SEM mineralogy as a valid option for archaeometric studies of ceramic pastes since includes precise quantitative data formulated from the chemical composition of each component of the paste, which may provide valuable evidence into raw materials and technological styles.
1 Introduction
The petrographic description of pottery vessels, commonly in a fragmented state, has allowed modern society to have information about the geological source of raw materials in prehistory. In addition to the review of complete pieces, archaeological and ethnographical sources, further enable us to understand the pottery traditions of the past (Lechtman 1977; Lemonnier 1986). It is believed that petrographic characterisations reflect the different technological choices made by the potters of the past according to the available resources and their cultural traditions. Indeed, analysis of ceramic pastes combined with morphological and decorative studies have provided significant information about the social dimension of ancient societies (e.g., Shepard 1956; Rye 1981; Rice 1987; Sinopoli 1991). The analysis of archaeological ceramics has been enriched over the last decades by the application of new analytical techniques, which determine the elemental and mineralogical composition of the ceramics (e.g., Quinn 2013; Rice 2015; Hunt 2017; Druc and Velde 2021). These data-sets has made it possible to expand the knowledge about the technological processes employed to create the ceramics (e.g., raw material selection, firing conditions, decoration, etc.), which is crucial to interpret spheres of circulation of human groups (e.g., Hodder, 1982; Shanks and Tilley, 1987; Latour, 1996; Soja, 1997; Lazzari, 1999; Latour, 2005; Albero Santacreu, 2016).
A pilot automated mineralogical study was performed by Knappett et al. (2011) on Bronze Age pottery from Crete (Akrotiri site), which offers a novel quantitative approach to describe archaeological ceramics. Based on automated SEM-EDS using QEMSCAN® technology, they obtained detailed compositional information (mineral identification and quantification, modal abundance) of the ceramics through mineralogical maps. This method has also been tested in different archaeological contexts to gain new insights into ceramic composition and technology (e.g., Menzies et al., 2015; Hilditch et al., 2016). More recently, some petrographic studies based on standard optical petrography have contrasted and successfully complemented with automated mineralogical analyses (e.g., Frigolé et al., 2019; Acevedo et al., 2020; Ogalde et al., 2020). In addition, Ward et al. (2018) used automated mineralogy to determine petrographic constraints of archaeological sediments and soils. Automated mineralogy thus appears to offer exciting innovation to perform accurate petrographic descriptions as offers robust information about the texture in ceramics, including matrix, non-plastic inclusion size distribution and porosity.
The arrangement of non-plastic inclusions, matrix or porosity distribution in the paste is what can be called texture. Texture gives an idea of the amount and type of effort which has gone into producing the paste for the ceramic (Druc and Velde 2021). The ceramic paste texture may vary between different cultures, even from geographically connected areas, and therefore may provide valuable information about cross-cultural knowledge circulation, which is further demonstrated in different studies made in the South Central Andes (including the Andean Circumpuna, after Núñez 1984), (e.g., Sanhueza 2004; Uribe 2006; Puente 2012; Stovel et al., 2013; Falabella et al., 2015; Pereyra Domingorena, 2015; Stovel and Echenique 2015; Uribe and Vidal 2015; Druc and Uribe 2018; Correa et al., 2019; Uribe et al., 2019). These studies have mainly described macroscopic and microscopic characteristics, such as type, shape, decoration, and paste, as well as functionality, and making statistical comparisons of ceramics to establish more significant occurrence and/or cultural origin. A remarkable typological heterogeneity of ceramics is found in different archaeological sites around San Pedro de Atacama (Chile), (e.g., Tarragó 1968; Uribe 2006; Stovel et al., 2013). Even though previous studies identified this variability, more detailed information about the paste of certain ceramic types is still unknown at present due to the limitations inherent in macroscopic petrographic approaches (e.g., Tarragó 1968, 1989; Uribe 2006). In order to filling the gaps in the knowledge about raw materials in ceramics from the Andean Circumpuna, we determine the paste composition of ceramic fragments collected from Formative Period domestic sites of Ghatchi in San Pedro de Atacama. This study incorporates the use of automated SEM mineralogy as an emerging tool to obtain critical new insights into the petrographic composition and mineralogical characteristics of archaeological ceramics. To emphasize, this is the first SEM-based automated mineralogical data of ceramic fragments found in domestic sites near San Pedro de Atacama, such an archaeological area has been largely studied during the last several decades due to its high relevance to the Andean archaeology.
2 Archaeological Setting
2.1 Study Area
From an archaeological perspective, the South Central Andean area comprises several subareas defined by ecological and cultural criteria, which include the Valles Occidentales (southern Peru to northernmost Chile), Circumtiticaca (Titicaca Lake, Peru-Bolivia), Valluna (eastern Bolivia), the Altiplano Meridional (Uyuni Salar, southwestern Bolivia), and Circumpuna (southwesternmost Bolivia, northwestern Argentina and the Atacama Desert in northern Chile), (Núñez 1984, 1991). The Atacama Desert of northern Chile, the driest non-polar desert on Earth, is included within the Circumpuna area, which is characterised by a notable scarcity of vegetation due to the hyperarid climate of the region (Latorre et al., 2005). In the Antofagasta Region, archaeologically relevant oases are situated in the Preandean basins between 2,400 and 2,800 m above sea level (m.a.s.l), such as Calama, San Francisco de Chiu-Chiu, and San Pedro de Atacama. The oases of San Pedro de Atacama (22°55′S; 68°12′W) are in the proximity of the active volcanic front at 2,408 m.a.s.l. This area comprises a very complex geology, which includes a wide range of rocks, including volcanic and sedimentary rocks. This area also includes very low-to low flow rivers (e.g., Vilama and San Pedro rivers) and extensive evaporite deposits (Salar de Atacama). During the pre-Hispanic and Historic periods, the mobility was the adaptive strategy that linked the different Andean cultural groups, connecting the Circumpuna with the San Pedro de Atacama oases. During the Formative Period (1200 BC—500 AD), the human groups that circulated around San Pedro de Atacama practised pastoralism, hunting wild animals, agriculture, and gathering fruits and tubers, thus obtaining essential food for their diet (Agüero and Uribe 2011). The need for storage led to develop ceramic technologies, both domestic and ceremonial (Uribe 2006). Diversity in the production of goods and surpluses was the basis that sustained the circulation of goods and the construction of intra and intercommunal life (Castro et al., 2016).
2.2 Pottery Studies in San Pedro de Atacama
The study of ceramics from San Pedro de Atacama is first recorded with the excavations and collections of Le Paige (1964). Sinclaire et al. (1997) studied local ceramic fragments and suggested the “Loa Café Alisado” and “San Pedro Negro Pulido” as a local ceramic type, whereas Orellana (1968) and Thomas et al. (1989) mention the “Los Morros” as foreign to San Pedro de Atacama. This interpretation was further supported by Uribe (2006). Tarragó (1989) investigated funerary ceramics from San Pedro de Atacama and classified them according to their decorative and macroscopic characteristics. This work proposed a chronological seriation of the local pottery and defined the “Negro Pulido” ceramic type and its variants. Based on macroscopic characteristics from local vessels, this sequence was more recently expanded by Stovel (2002) and included the “San Pedro Negro Pulido” type as typical from San Pedro de Atacama.
Near to San Pedro de Atacama oases are the Ghatchi archaeological sites in the Vilama River. Ghatchi is located northeast of San Pedro de Atacama and covers an extensive area of 28 km long and 4 km wide (Figure 1). Human settlement in this area has spanned several periods, from the Late Archaic to the Early Formative, indeed several domestic sites have been recognised within Ghatchi (Agüero 2005). The characteristic structures of Ghatchi are stone shelters (Figure 1), where lithic material, bone remains and ceramic fragments are common in the surface and excavation sites (e.g., Agüero and Uribe 2011).
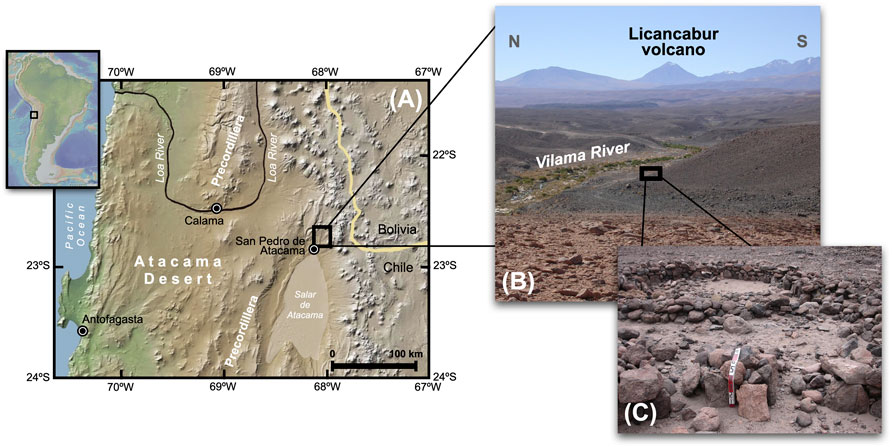
FIGURE 1. Study area. (A) Map of the Central Andes between 21°20′ to 24°S showing the location of the study area. Map created using the “GeoMapApp” (www.geomapapp.org, Ryan et al., 2009). Photographs showing a panoramic view of the Ghatchi archaeological sites in the Vilama River (B) and local Formative Period domestic structures (C).
2.3 Petrography of Ceramic Fragments from Ghatchi
Uribe (2006) studied Formative Period (1200 BC to 400 AD) ceramic fragments found in excavation sites at Ghatchi. This study identified several ceramic types with the “Los Morros” (LMS), “Loa Café Alisado” (LCA) and “San Pedro Negro Pulido” (NP1) being the most common styles at many sites (Figure 2). Uribe (2006) subdivided the LMS type into three main petrographic variants: LMS-A, LMS-B1 and LMS-B2. LMS-A is defined as a volcanic paste with very coarse (3–10 mm) and tabular dark grey non-plastic inclusions. LMS-B1 is composed by a granitic paste with very coarse (4–5 mm) white non-plastic inclusions of quartz and micas, whereas LMS-B2 is a fine-grained variant. The LMS-type pastes normally show high degrees of porosity due to the remotion of coarse inclusions. LCA fragments are characterised by sandy pastes with abundant white non-inclusions (e.g., Sinclaire et al., 1997). NP1 is constituted by granitic pastes with non-plastic inclusions of quartz (e.g., Tarragó 1989).
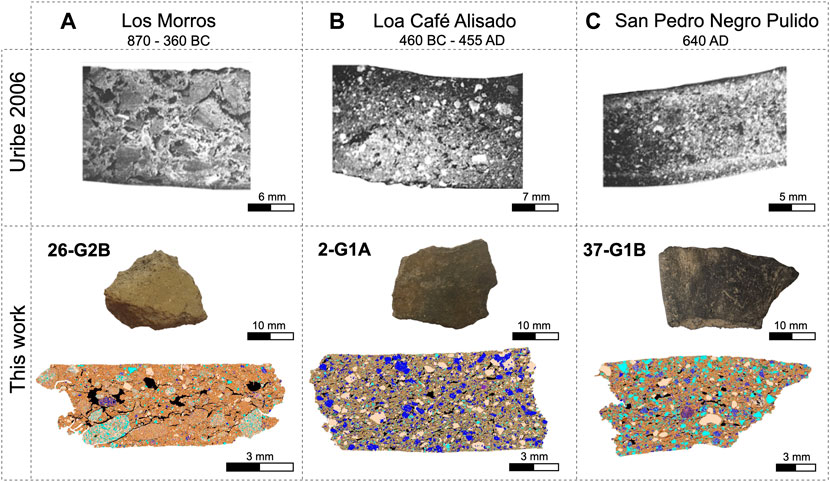
FIGURE 2. Representative photographs and automated mineralogical maps of the studied sample types: (A) Los Morros (LMS), (B) Loa Café Alisado (LCA) and (C) San Pedro Negro Pulido (NP1). Upper and middle and lower panels show examples of fresh pastes (after Uribe 2006), ceramic fragments and automated mineralogical maps, respectively. Thermoluminescence dating from Uribe (2006) and Agüero and Uribe (2011).
3 Materials and Methods
In this study, we analysed twelve (12) ceramic fragments representative of the most common typologies found in excavation sites at Ghatchi (Table 1, Uribe 2006). To fully characterise the mineralogical properties of these fragments, we employed automated mineralogical analysis using the QEMSCAN® technology. Sample preparation, analysis and data processing was carried out at the Maini Scientific Equipment Unit at Universidad Católica del Norte (UCN).
3.1 Sample Preparation
The samples were mounted in 30 mm circular mounts using epoxy resin (Figure 3). We placed either one ceramic fragment (up to 25 mm long) or two smaller fragments in a single mount, optimising time and resources for preparation and further analysis (Figure 3). The mounts were cut and polished to expose a cross-section of the ceramic fragment, and then carbon coated (Figure 3). Prior to automated mineralogical analysis, we obtained high-resolution photos of each mount from a Bruker M4 Tornado (X-ray Microfluorescence) to compare the “fresh paste” with mineralogical maps obtained from automated mineralogical analysis.
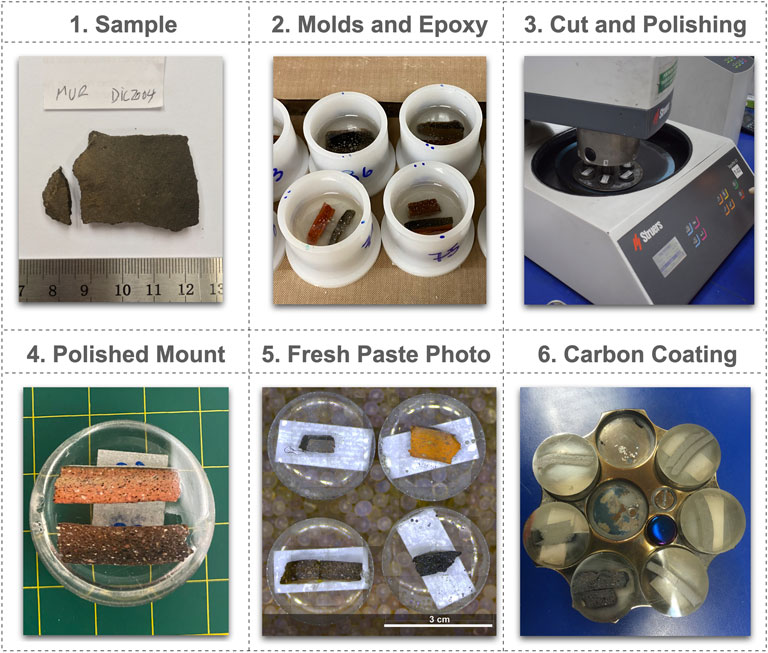
FIGURE 3. Visual scheme of the employed sample preparation methodology. Ceramic fragments (up to 25 mm in size) were mounted in low-volatile epoxy and placed in 30 mm circular mounts. Each sample mount was polished flat, and then carbon coated prior SEM analysis.
3.2 Petrographic Analysis by Automated Mineralogy
Automated mineralogical analysis were made using the QEMSCAN®, the English acronym for Quantitative Evaluation of Minerals by SCANning Electron Microscopy. QEMSCAN® is a system configured to measure mineralogical variability from chemical information on a micrometric scale, originally developed to provide fast, automated, and fully quantitative mineralogical data for the mining and mineral processing industry (e.g., Pirrie et al., 2004, 2009; Goodall et al., 2005; Goodall and Scales 2007; Knappett et al., 2011). We performed our analysis in a QEMSCAN® model E430, which is based on a ZEISS EVO 50 SEM combined with Bruker Series 4 EDS detectors at the Maini Scientific Equipment Unit, UCN. As a result of this technique, 2D and false-colour images are obtained for each studied sample, where each pixel preserves its elemental composition and brightness information (BSE), which thus facilitates the subsequent processing of the measurement data. Measurements were performed using iMeasure v5.3.2 and compositionally mapped in the “Fieldscan” operating mode at a field size of 1,500 μm (approximate magnification of ×50) or 2,500 μm (approximate magnification of ×30), (Figure 4). For data reduction, we used the iDiscover v5.3.2 software, which allows the construction of customised filters to quantify mineral abundances represented in mineralogical maps and to classify grains according to size criteria. The outputs used are both visual and numerical and include mineralogical maps, modal mineralogy, and grading tables, which are described in the following sections. Further details of the QEMSCAN® theory and analytical modes are given in Gottlieb et al. (2000) and Pirrie et al. (2004).
3.2.1 Mineralogical Maps
This represents the total open area of the ceramic fragment on the mount and maintains the shape of a cross-section to a ceramic fragment exposed on the surface. It is a “false colour” map, which will represent each preferred mineral or chemical group (Figure 5A). The fields read by the SEM are combined in a mosaic to build a single image that will represent the total area analysed. The original mineralogical map list can be modified to better visualise a single mineral or mineral groups, e.g., the constituents of the silt-clay matrix (Figure 5B). The customisable mineral colour list is kept constant from sample to sample to aid in comparisons. The mineralogical grouping used in this work is shown in Table 2.
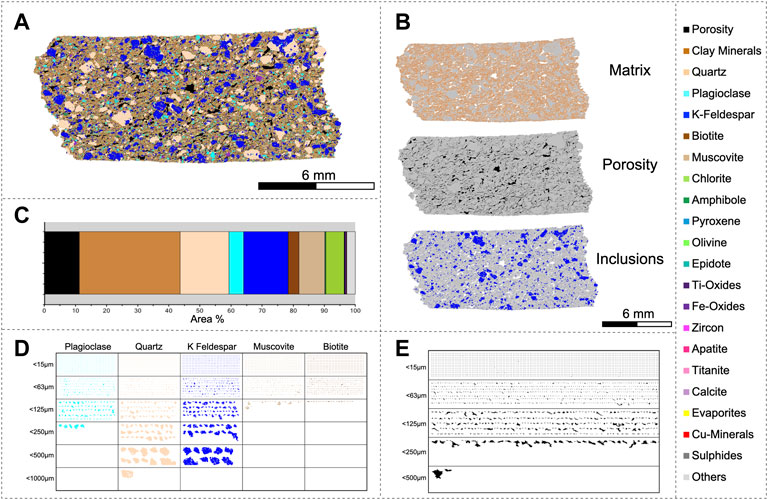
FIGURE 5. iDiscover filters used for automated mineralogical analysis. Sample2-G1A: (A) Mineralogical map of the ceramic fragment; (B) Mineralogical map of the paste components; (C) modal mineralogy; and grading tables for mineral grains (D) and porosity (E).
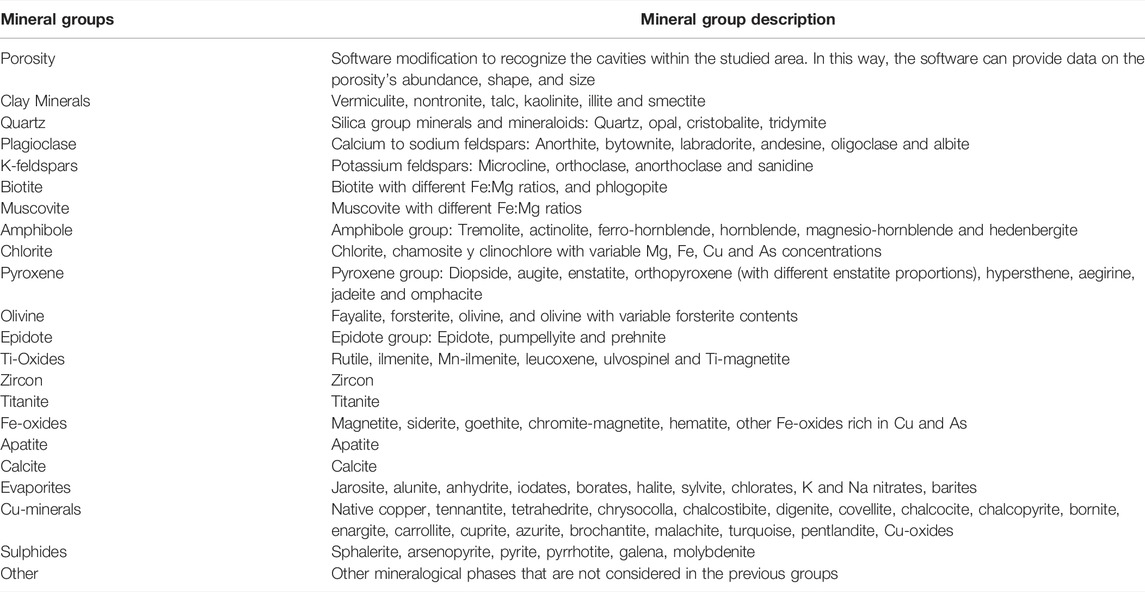
TABLE 2. Summary of the mineralogical list used in the iDiscover software. The selected mineral groups correspond to rock-forming silicates and representative Cu-rich, sulphide and evaporite minerals found frequently in igneous, sedimentary, and metamorphic rocks in the Central Andes.
3.2.2 Modal Mineralogy
The obtained data can be interpreted from numeric data tables or diagrams. These data can be presented as mass% or area%. In this work, the area% was used so that the porosity can be included and evaluated. We set the porosity on the Pre-processor setting by including the “injection” filter to determine porosity as a quantifiable component (Figure 5C).
3.2.3 Grading Tables
This allows to separate grains according to a granulometric fraction (grain size) vs. mineralogical composition, facilitating the granulometric and morphological analysis of the non-plastic inclusions set in the paste (Figure 5D). This additionally permit to recognise very small grains present in the silt-clay matrix, as well as porosity size and distribution (Figure 5E).
4 Results
4.1 Ceramic Petrography
In the same way that petrography is done in geology, the “appearance” or set of textures in ceramics is as vital for the archaeological analyses, since the interpretations of archaeological materials imply decisions about raw materials used, manufacturing processes chosen, ceramic circulation and use, and thus represent the technological choices of the potters and time period (Lechtman, 1977). For this reason, the first filter used for classification are mineralogical maps, where the visual relationship between clay matrix, mineral inclusions and porosity can be observed. Grain size is described using the Wentworth scale (Wentworth 1922). Modal abundance (%) is presented as area%.
4.2 Los Morros
Five samples were analysed: 17-G2B, 18-G2B, 24-G2B, 26-G2B and 28-G2B. Samples 17-G2B and 18-G2B are classified as LMS-B1 by Uribe (2006), and present similar petrography among them (Figure 6). They are characterised by coarse sand-sized pastes. 17-G2B and 18-G2B have a clay matrix with an abundance of 50 and 49%, respectively, mainly composed of clay minerals and micas (muscovite > biotite > chlorite), (Table 3; Figure 6). Non-plastic inclusions are in abundance of 37 and 40%, with a size range between fine to very coarse sand, reaching in some cases the size of fine gravel. Their shapes range from subangular to rounded, with poor to moderate selection and distribution. Four types of non-plastic inclusions were found: 1) volcanic origin characterised by the presence of plagioclase and pyroxene and possibly glass in the groundmass; 2) sedimentary origin composed by biotite, muscovite, and clay minerals; 3) plagioclase and quartz grains; and 4) crushed ceramic fragment inclusions can be identified. The ceramic fragment inclusions appear white-ish with darker small angular grains in the fresh paste photograph, whereas they are compositionally uniform on the mineralogical map (Figure 6). Furthermore, the sample 17-G2B, a border-shaped ceramic fragment is observed (Figure 6). The porosity in the samples 17-G2B and 18-G2B is moderate, with percentages close to 13 and 11%, respectively, and with planar and elongated shapes surrounding the larger clasts (i.e., secondary porosity, cf. Druc and Velde 2021).
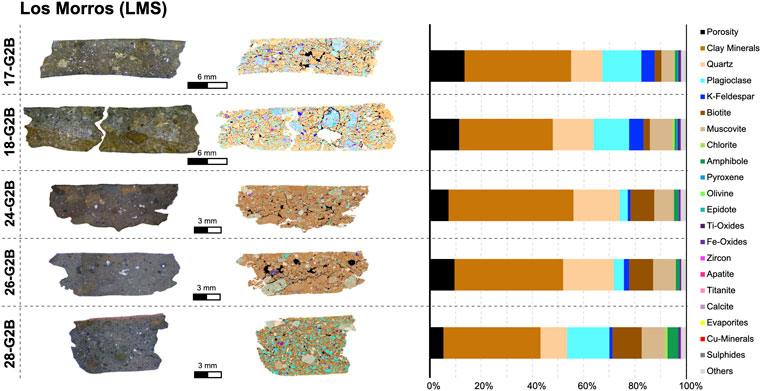
FIGURE 6. Fresh pastes (left), mineralogical maps (middle), and modal mineralogy (right) of the studied Los Morros (LMS) ceramic samples.
Sample 24-G2B, variant LMS-B1 (Uribe 2006), presents a coarse to very coarse granulometry. This sample has a clay matrix with an abundance of 58%, composed of clay minerals, quartz, and micas (quartz > biotite), (Figure 6). The non-plastic inclusions are of subangular to sub-rounded shapes, with poor selection and distribution, being in an abundance of 35% with a size ranging from fine to very coarse sand (Table 3). Most of these inclusions are fine sedimentary rock fragments—some rich in clay minerals (similar to the matrix), and others rich in quartz and plagioclase and subordinate clay minerals. The porosity is moderate (7%) with planar to irregular shapes.
Sample 26-G2B, variant LMS-A (Uribe 2006), shows coarse to very coarse non-plastic inclusions, subangular to rounded shape, moderate to low selection, and a good to moderate distribution. They present a clay matrix with an abundance of 50%, composed of clay minerals, quartz and micas (quartz = biotite > muscovite > chlorite), (Figure 6). The non-plastic inclusions have an abundance of 40% (Table 3), they range in size from fine to very coarse sand, and in some cases, they reach the size of fine gravel. Most of these inclusions are sedimentary rock fragments and quartz grains (Figure 6). The rock fragments present iron and titanium oxides, apatite, amphiboles, pyroxenes, and olivine as accessory minerals. The porosity in this sample is 10%, mainly elongated flat to irregular in shape.
Sample 28-G2B, variant LMS-B2 (Uribe 2006), shows a granulometry of non-plastic inclusions of very fine to coarse sand. These inclusions are in an abundance of 36%, with subangular to rounded shapes, with a poor selection and a moderate distribution. They are composed of crystals of plagioclase, quartz, biotite, muscovite and iron and titanium oxides (Figure 6; Table 3), as well as coarse sand-sized granitic rock fragments. The matrix is clay-micaceous (muscovite-biotite > chlorite with a 59% in abundance (Figure 6; Table 3). The porosity is low with abundance of 5%, and irregular to planar shapes.
4.3 Loa Café Alisado
Four samples were analysed: 2-G1A, 5-G1A, 12-G1B and 29-G1B. These fragments were classified as LCA by Uribe (2006). 2-G1A, 5-G1A and 12-G1B show similar petrography. The clay matrix, with abundance between 50 and 52% (Table 3), is composed of clay minerals, clay-sized micas (generally muscovite > biotite > chlorite) and quartz (Figure 7). These samples are characterised by coarse sand-sized non-plastic inclusions set in a fine to medium sand pastes. The inclusions show subangular to rounded shapes, of good quality moderate selection and distribution, with abundance between 37 and 40%. The inclusions are mainly of granitic rock fragments, which are made of K-feldspar, quartz, and plagioclase (Table 3). As accessory minerals, iron and titanium oxides, amphiboles, and epidote are observed. The porosity is moderate, ca. 10%, and mainly irregular or planar in shape, and to a lesser extent, elongated.
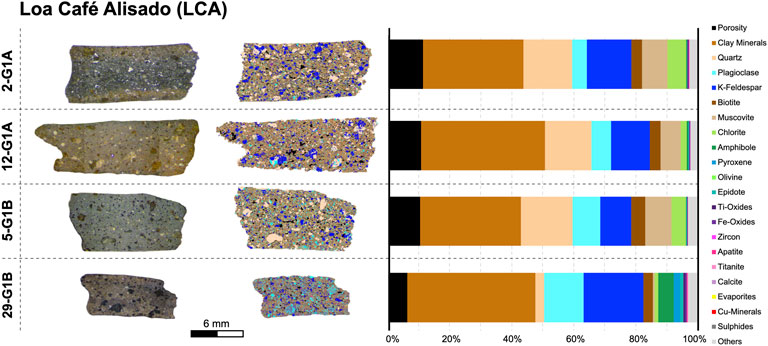
FIGURE 7. Fresh pastes (left), mineralogical maps (middle), and modal mineralogy (right) of the studied Loa Café Alisado (LCA) ceramic samples.
Sample 29-G2B is composed by a clay matrix (49%), very fine to medium sand in size, of clay minerals, K-feldspar, chlorite and biotite grains and very low proportions of quartz and muscovite (Figure 7). The non-plastic inclusions are 45% in abundance (Table 3), with subangular to sub-rounded shapes and a moderate selection and distribution (Figure 7). They are andesitic volcanic rock fragments, which are made of plagioclase microlite-rich groundmass. Non-plastic inclusions of K-feldspar and plagioclase grains are also observed within the paste. Epidote, amphibole, iron and titanium oxides, pyroxene and apatite are found as accessory minerals. The porosity is moderate (6%), with irregular shapes.
4.4 San Pedro Negro Pulido
Three samples were analysed: 30-G1A, 31-G1A and 37-G1B. These samples were classified as NP1 by Uribe 2006. All these fragments have similar petrography. They are composed of fine to very fine pastes. The clay matrix with an abundance between 68 and 58% (Table 3) is composed of clay mineral and clay-sized micas (muscovite > biotite >> chlorite), (Figure 8). The non-plastic inclusions have a size range between very fine to coarse sand, with an abundance between 26 and 35% (Table 3), with subangular to rounded shapes, good selection and moderate to good distribution. These inclusions are composed of plagioclase and quartz grains and volcanic rock fragments. The rock fragments are made of phenocrysts of plagioclase, quartz and amphibole set in a partially devitrified groundmass. Amphibole, K-feldspar, biotite, iron and titanium oxides appear as accessory minerals. The porosity of these samples is moderate (6–7%) with irregular to elongated shapes.
5 Discussion
5. 1 Automated SEM Mineralogy and Pottery
Automated SEM mineralogy was used as the basis for our petrographic approach of ceramic fragments (cf. Knappett et al., 2011; Hilditch et al., 2016). The most significant advantage is the quality of the data obtained, precise quantitative data formulated from the chemical composition of each component of the paste, i.e., clay matrix and non-plastic inclusions, which are calculated employing standard parameters for each mineral composition. As the studied ceramic fragments were sourced from an archaeological site located in a volcanic environment, they may also contain volcanic glass and rock fragments. Volcanic glass does not have a specific chemical composition (i.e., non-stoichiometric behaviour, Knappett et al., 2011), and thus it must be adjusted for each case study. To incorporate glass in our mineralogical maps, we built a test mineral list on the iDiscover software including the average composition of volcanic glass previously used for automated SEM mineralogy in Central Andean lavas (González-Maurel et al., 2019). However, the compositional data obtained revealed large internal differences and differed from the glass data obtained by González-Maurel et al. (2019). Given that our studied ceramic samples reveal a wide compositional heterogeneity (Table 3), a representative glass composition was not possible to calibrate in our mineralogical list. Consequently, possible volcanic glass grains set in the matrix or as non-plastic inclusions were not distinguished and the volcanic glass is thus not discussed further in this study. In the case of the rock fragment inclusions, they were not automatically identified by employing SEM analysis. On the iDiscover software, we tested a new filter to facilitate their automated recognition by separating rock fragments from mineral inclusions when delivering mineral abundance percentages, however, as with volcanic glass, must be adjusted for each case study. Although automated classification of polymineralic geological materials seems not yet possible with automated SEM systems, rock fragment compositions can be interpreted from the obtained mineralogical data and therefore are discussed thoroughly in the following sections.
5.2 Archaeological Ceramic Fragments From Ghatchi
The studied ceramic fragments from Ghatchi reveal a wide range of petrographic variability, which is in agreement with previous macroscopic descriptions made for these fragments (Uribe 2006). Notably, this heterogeneity is more evident when pastes, including their components, are analysed microscopically (Figure 2). Here, we include new inter-grain scale information for the ceramic types recognised by Uribe (2006). In addition, we discuss possible sources of raw materials by comparing the paste components with existing lithological information from local geological maps published by the Chilean Geological Survey (SERNAGEOMIN). This in turn allow us to evaluate the technological styles related to raw material availability and/or cultural traditions (e.g., Lechtman 1977; Sillar and Tite 2000; Quinn 2013) around the San Pedro de Atacama oases.
5.2.1 Los Morros
Our sample set included the three variants for Los Morros ceramic type from Ghatchi, i.e., 26-G2B as LMS-A; 17-G2B, 18-G2B and 24-G2B as LMS-B1; and 28-G2B as LMS-B2 (after Uribe 2006). The clay matrix, mainly composed by clay minerals, quartz, and micas, is relatively similar for all the studied samples. However, the non-plastic inclusions contained in these samples reveal a large variability in abundance and composition, especially in rock and crushed ceramic fragments. The samples 26-G2B and 24-G2B contain very similar non-plastic inclusions of plagioclase and quartz grains and sedimentary rock fragments (Figure 6). Although these samples represent two distinct macroscopic types (e.g., LMS-A and LMS-B1), they appear to share common origin. Plagioclase and quartz are two of the most common mineral phases in igneous, sedimentary, and metamorphic rocks in northern Chile and elsewhere, and thus they cannot be used as tracer for potential sources. In contrast, sedimentary outcrops are less frequent in northern Chile, but many located around the San Pedro de Atacama oases (e.g., San Pedro and Vilama formations). Sedimentary rocks from the San Pedro Formation (Brüggen 1942) are mostly sandstones and siltstones rich in evaporite minerals, whereas Vilama Formation (Moraga et al., 1974) is composed by fossiliferous mudstones, calcareous sandstones and volcanosedimentary rocks (Henríquez et al., 2014). These lithologies however do not reconcile with the sedimentary rock fragments contained in 24-G2B and 26-G2B, which we interpret as claystone and siltstone of plagioclase, quartz, and muscovite (Figure 6). This implies that the raw materials are not found in the geological landscape and thus may represent a foreign type of pottery. Similar, the samples 17-G2B and 18-G2B include non-plastic inclusions of, in order of decreasing abundance, volcanic rock fragments, sedimentary rock fragments, plagioclase and quartz grains, and crushed ceramic fragments. We interpret these volcanic rock fragments as andesitic to dacitic lavas, which could be potentially sourced from volcanic lava fields erupted from volcanoes close to San Pedro de Atacama (e.g., Putana, Sairecabur, Licancabur, Lascar). However, the sedimentary rock fragments found in 17-G2B and 18-G2B are not entirely consistent with the sedimentary rocks found in local rock formations (i.e., San Pedro and Vilama, Henríquez et al., 2014). In addition, crushed ceramic fragments does not match with any ceramic type found in Ghatchi archaeological sites (i.e., LMS, LCA and NP1). Therefore, as with samples 24-G2B and 26-G2B, most of the raw material for 17-G2B and 18-G2B seems to be foreign to San Pedro de Atacama. Similar, the sample 28-G2B contains abundant fine mineral grains and granitic rock fragments, the latter usually associated to felsic plutonic complexes located in the Andean Precordillera to the south of the Salar de Atacama (Ramírez and Gardeweg, 1982) and would reflect other type of foreign raw material. The variability revealed by the non-plastic inclusion compositional data thus appears to indicate that the LMS ceramic type is sourced via circulation of objects rather than represents local pottery. This agrees well with previous archaeological constraints for LMS fragments found in other archaeological sites between the Loa River basin (∼22°25′S; 68°47′W) and the Salar de Atacama (∼23°29′S; 68°19′W), (Orellana 1968, 1989; Benavente 1981; Thomas et al., 1989; Sinclaire et al., 1997; Uribe 2006).
5.2.2 Loa Café Alisado
The samples 2-G1A, 5-G1B, 12-G1A and 29-G1B from Ghatchi were classified macroscopically as the LCA ceramic type (after Uribe 2006). The sample 29-G1B, however, differs in composition with the other LCA samples. The clay matrix of the samples 2-G1A, 5-G1B and 12-G1A is composed by clay minerals, muscovite, biotite, chlorite, and quartz, whereas the clay matrix of the sample 29-G1B contains clay minerals, K-felspar, chlorite and biotite and very small proportions of quartz and muscovite. Similar, the samples 2-G1A, 5-G1B and 12-G1A are characterised for K-feldspar-quartz-plagioclase granite rock fragments and, in order of decreasing abundance, quartz, K-feldspar and minor plagioclase grain inclusions, in contrast to the sample 29-G1B that contains andesitic rock fragments and mineral grain inclusions of, in order of decreasing abundance, plagioclase, K-feldspar, amphibole and pyroxene (Figure 7). The recurrent appearance of granite inclusions within a homogeneous clay matrix, such as observed in the samples 2-G1A, 5-G1B and 12-G1A, constitutes a characteristic feature for the LCA ceramic type in the Atacama Desert (e.g., Uribe and Vidal 2012, 2015; Riera-Soto et al., 2020). This implies the potters used a specific supply source of raw materials, which does not vary during the Formative Period. Since volcanic and sedimentary rock deposits form the landscapes around the San Pedro de Atacama oases (Henríquez et al., 2014), a possible granite source would be related to the well-exposed plutonic complexes (e.g., Limón Verde, Chuquicamata) outcropping in the Andean Precordillera (e.g., Ramírez and Gardeweg, 1982; Marinovic and Lahsen, 1984; Tomlinson et al., 2010), which is further consistent with previous studies in LCA fragments (e.g., Sinclaire et al., 1997; Uribe and Vidal, 2015; Riera-Soto, 2019). Although the samples 2-G1A, 5-G1B and 12-G1A appear to be representative of the LCA ceramic type, the sample 29-G1B is manufactured with different raw material (e.g., andesitic rock fragments, low quartz abundance) and thus suggesting a different origin, e.g., from volcanic fields near to San Pedro de Atacama. However, macroscopic observations show similar textures for all LCA samples (Figure 7), which would indicate a homogenised recipe with variable raw materials as part of a specialised knowledge, that seems to be foreign to Ghatchi, and maybe being part of the Loa-Tarapacá circulation (Sinclaire et al., 1997; Uribe 2006; Uribe and Vidal 2015).
5.2.3 San Pedro Negro Pulido
The samples 30-G1A, 31-G1A and 37-G1B were classified macroscopically as NP1 by Uribe (2006). These ceramic fragments present similar clay matrix compositions (Figure 8). The non-plastic inclusions are also similar in all the samples studied, which are made of plagioclase, quartz, biotite, and amphibole grains and volcanic rock fragments (Figure 8). We interpret the volcanic rock fragments as andesitic to dacitic lavas or ignimbrites, which could be sourced from volcanic fields surrounding San Pedro de Atacama (Salisbury et al., 2010; Henríquez et al., 2014). It is also worth noting that the Vilama River have its origin near the foot of the volcanoes and are fed by various tributaries that cross different volcanic fields. This implies that the volcanic rock fragments could be collected either from a local volcanic deposit or downstream at Ghatchi as eroded material. Since we have not found any other type of non-plastic inclusion in the studied NP1 fragments from Ghatchi and considering the local geological framework, we suggest that the NP1 ceramic type might represent local pottery. This interpretation agrees well with recent findings from Echenique et al. (2018) on NP1 ceramic fragments from the Aldea de Coyo archaeological site also located in San Pedro de Atacama. For the NP1 ceramics, we may suggest that the potters resorted to a known geological environment, which possibly remained constant during the Formative Period, and allowed them to collect the raw material from the same source(s) to produce the first most representative pottery from the San Pedro de Atacama oases.
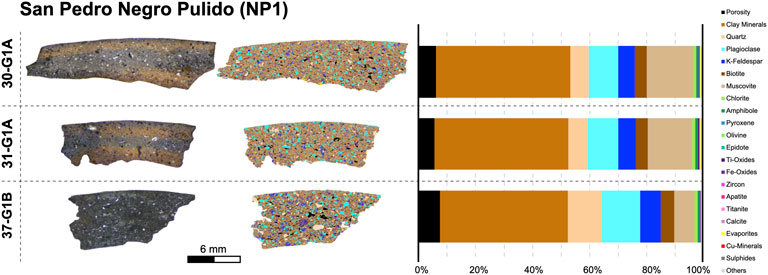
FIGURE 8. Fresh pastes (left), mineralogical maps (middle), and modal mineralogy (right) of the studied San Pedro Negro Pulido (NP1) ceramic samples.
6 Conclusion
The ceramic fragments used in this study were collected from excavations at the Ghatchi archaeological sites in the Vilama River, northeast of San Pedro de Atacama. Previous petrographic studies recognised three ceramic types in this site: Los Morros (LMS), Loa Café Alisado (LCA) and San Pedro Negro Pulido (NP1). Here we employed automated mineralogical analyses to fully characterise representative ceramic fragments of these typologies. The proposed methodology provided robust data to identify textural and compositional variations in the paste and its components. The clay matrix compositions are distinct for each ceramic type, which may suggest a specialised local knowledge to produce a particular type of pottery. These variations combined with non-plastic inclusions mineralogical information, and together with macroscopic observations, showed evidence about the technological choices made by the potters during the Formative Period according to their cultural traditions and the available resources in their territories or around. Overall, the LMS ceramic type from Ghatchi is characterised by a clay mineral-quartz-mica-rich matrix with variants made of mineral, sedimentary and volcanic rock fragment and/or crushed ceramic inclusions; LCA type by a clay mineral-mica-rich matrix with mineral and granitic rock fragment inclusions; and NP1 type by a clay mineral-mica-rich matrix with mineral and andesitic to dacitic rock fragment inclusions. LMS and LCA are demonstrated to be foreign to Ghatchi, and likely being part of inter-regional pottery circulation. The NP1 ceramic fragments, on the other hand, are interpreted as autochthonous since resembles the typical ceramic type produced in the San Pedro de Atacama oases.
To emphasize, the archaeometric-focused approach employed here contribute to the search for possible methodological combinations that allow effective and efficient research on raw materials and ceramic circulation in different archaeological contexts. Indeed, a detailed petrographic study on ceramic pastes is a tool that, together with e.g., chemical, morphological and decorative studies, will lead research groups to reach major social and cultural dimensions (technological styles) of archaeological pottery. This study in turn contributes to an interdisciplinary understanding of the analytical data obtained from archaeological materials. Similar studies should always be approached from a joint professional workflow, which allows the correct application of an analytical technique to archaeological materials suitable for the chosen technique.
Data Availability Statement
The original contributions presented in the study are included in the article/supplementary material, further inquiries can be directed to the corresponding author.
Author Contributions
CRS—conceptualization, investigation, visualization, formal analysis, methodology, resources, writing—original draft, writing—review and editing, funding acquisition. CA—conceptualization, investigation, methodology, resources, writing—review and editing, funding acquisition. OGM—conceptualization, investigation, visualization, formal analysis, methodology, writing—review and editing. MU—conceptualization, first analysis, resources, review, funding acquisition. AM—formal analysis, methodology, review, funding acquisition.
Funding
This work has been funded by the Unidad de Equipamiento Científico (MAINI-Universidad Católica del Norte, Chile) and the FONDECYT projects No. 1030931 and No. 1181829. CR-S is a PhD candidate granted by the Beca de Doctorado en el Extranjero, Becas-Chile Convocatoria 2019, No. 72200512 (Agencia Nacional de Investigación y Desarrollo Chile).
Conflict of Interest
Author AM was employed by the company Bruker Nano GmbH.
The remaining authors declare that the research was conducted in the absence of any commercial or financial relationships that could be construed as a potential conflict of interest.
Publisher’s Note
All claims expressed in this article are solely those of the authors and do not necessarily represent those of their affiliated organizations, or those of the publisher, the editors and the reviewers. Any product that may be evaluated in this article, or claim that may be made by its manufacturer, is not guaranteed or endorsed by the publisher.
Acknowledgments
We thank María Monserrat Barraza, Carolina Ossandón and María González for their assistance during sample preparation and execution of automated mineralogical analyses at MAINI-UCN, and Rene van der Merwe (University of Cape Town) for the sample preparation advising. We also thank Verónica Acevedo, Isabelle Druc, Fernanda Falabella, Lorena Sanhueza, Petrus le Roux and Lucas Pereyra-Domingorena. A very special thanks to Nala for her never-ending support. The authors are grateful for the constructive comments and suggestions that helped to significantly improve this manuscript from Wayne Powell and Patrick Quinn, and Duncan Pirrie for editorial handling. This work was supported by the FONDECYT projects No. 1030931 and No. 1181829, and the Instituto de Investigaciones Arqueológicas y Museo of Universidad Católica del Norte. CR-S is a PhD candidate granted by the Beca de Doctorado en el Extranjero, Becas-Chile Convocatoria 2019, No. 72200512 (Agencia Nacional de Investigación y Desarrollo Chile).
References
Acevedo, V. J., Staropoli, L., Riera-Soto, C., Soto, D., Herrera, V., and Rossi, L. (2020). Avances en tecnología arqueométrica para el estudio de cerámicas arqueológicas de ámbito circumpuneño. Anu. Arqueol. 12, 67–85. doi:10.35305/aa.v12i12.46
Agüero, C. (2005). Aproximación al asentamiento humano temprano en los oasis de San Pedro de Atacama. Estud. Atacameños 30, 29–60. doi:10.4067/S0718-10432005000200003
Agüero, C., and Uribe, M. (2011). Las sociedades formativas de San Pedro de Atacama: Asentamiento, cronología y proceso. Estud. Atacameños, Arqueol. Antropol. Surandinas 42, 53–78. doi:10.4067/S0718-10432011000200004
Albero Santacreu, D. (2016). Interpreting Long-Term Use of Raw Materials in Pottery Production: An Holistic Perspective. J. Archaeol. Sci. Rep. 16, 505–512. doi:10.1016/j.jasrep.2016.04.008
Benavente, A. (1981). Chiu Chiu 200: Un campamento de pastores. Memoria de Título en Arqueología. Santiago: Universidad de Chile, Facultad de Ciencias Sociales, Departamento de Antropología.
Brüggen, J. (1942). “Geología de la Puna de San Pedro de Atacama y sus formaciones de areniscas y arcillas rojas,” in Congreso Panamericano de la Ingeniería de Minas y Geología, No. 1 Santiago, 2, 342–367. Anales.
Castro, V., Berenguer, J., Gallardo, F., Llagostera, A., and Salazar, D. (2016). “Vertiente Occidental circumpuneña. Desde las sociedades posarcaicas hasta las preincas (ca. 1.500 años a.C. a 1.470 años d.C.),” in Prehistoria en Chile: Desde sus primeros habitantes hasta los incas. Editors F. Falabella, M. Uribe, L. Sanhueza, C. Aldunate, and J. Hidalgo (Santiago: Editorial Universitaria), 239–283.
Correa, I., Gallardo, F., Uribe, M., Echenique, E., Blanco, J. F., Flewett, S., et al. (2019). “Pottery from Funerary Mound Along the Arid Atacama Desert Coast, Chile. Chemistry, Circulation, and Exchange Between the Inlands and Coast During the Formative Period,” in Ceramics of the Indigenous Cultures of South America. Studies of Production and Exchange Through Compositional Analysis. Editors M. D. Glascock, H. Neff, and K. J. Vaughn (Albuquerque: University of New Mexico Press), 147–160.
Druc, I., and Uribe, M. (2018). Un volcán en la cerámica: Indicios de producción en el altiplano de Isluga, Tarapacá-Chile. Comechingonia Rev. Arqueol. 22, 11–35. doi:10.37603/2250.7728.v22.n1.26674
Echenique, E., Killick, D., and Stovel, E. (2018). Exploring Operational Chains and Technological Styles in the Production of Domestic Polished Wares from San Pedro de Atacama, Chile. J. Archaeol. Sci. Rep. 12, 771–782. doi:10.1016/j.jasrep.2018.08.048
Falabella, F., Sanhueza, L., Correa, I., Fonseca, E., and Roush, C. C. (2015). Tradiciones tecnológicas del Período Alfarero Temprano de Chile Central: Un estudio de bordes, materias primas y pastas de vasijas de cocina en la microrregión de Angostura. Chungara 47 (3), 353–368. doi:10.4067/S0717-73562015005000017
Frigolé, C., Riera-Soto, C., Menzies, A., Barraza, M., Benítez, A., and Winocur, D. (2019). Estudio de pastas cerámicas del centro-oeste argentino: microscopía óptica y QEMSCAN. Bol. Arqueol. PUCP 27, 67–85. doi:10.18800/boletindearqueologiapucp.201902.004
González-Maurel, O., Godoy, B., Le Roux, P., Rodríguez, I., Marín, C., Menzies, A., et al. (2019). Magmatic Differentiation at La Poruña Scoria Cone, Central Andes, Northern Chile: Evidence for Assimilation During Turbulent Ascent Processes, and Genetic Links with Mafic Eruptions at Adjacent San Pedro Volcano. Lithos 338-339, 128–140. doi:10.1016/j.lithos.2019.03.033
Goodall, W. R., and Scales, P. J. (2007). An Overview of the Advantages and Disadvantages of the Determination of Gold Mineralogy Using Automated Mineralogy. Miner. Eng. 20, 506–517. doi:10.1016/j.mineng.2007.01.010
Goodall, W. R., Scales, P. J., and Butcher, A. R. (2005). The Use of QEMSCAN and Diagnostic Leaching in the Characterization of Visible Gold in Complex Ores. Miner. Eng. 18, 887–886. doi:10.1016/j.mineng.2005.01.018
Gottlieb, P., Wilkie, G., Sutherland, D., and Ho Tun, E. (2000). Using Quantitative Electron Microscopy for Process Mineralogy Applications. J. Minerals Mater. Characterisation Eng. 52 (4), 24–27. doi:10.1007/s11837-000-0126-9
Henríquez, S., Becerra, J., and Arriagada, C. (2014). Geología del área San Pedro de Atacama, Región de Antofagasta. Servicio Nacional de Geología y Minería, Carta Geológica de Chile. Ser. Geol. Básica 171, 1. mapa escala 1:100.000.
Hilditch, J., Pirrie, D., Knappett, C., Rollinson, G. K., and Momigliano, N. (2016). “Taking the Rough with the Smooth: Using Automated SEM-EDS to Integrate Coarse and Fine Ceramic Assemblages in the Bronze Age Aegean,” in Insight from Innovation: New Light on Archaeological Ceramics,. Editors E. Sibbesson, B. Jervis, and S. Coxon (Oxford: Oxbow), 74–96. Southampton Monographs in Archaeology New Series 6.
Hunt A. (Editor) (2017). The Oxford Handbook of Archaeological Ceramic Analysis (Oxford: Oxford University Press), 724.
Knappett, C., Pirrie, D., Power, M. R., Nikolakopoulou, I., Hilditich, J., and Rollinson, G. K. (2011). Mineralogical Analysis and Provenancing of Ancient Ceramics Using Automated SEM–EDS Analysis (QEMSCAN): A Pilot Study on LB I Pottery from Akrotiri, Thera. J. Archaeol. Sci. 38 (2), 219–232. doi:10.1016/j.jas.2010.08.022
Latorre, C., Betancourt, J. L., Rech, J. A., Quade, J., Holmgren, C., Placzek, C., et al. (2005). “Late Quaternary History of the Atacama Desert,” in 23°S: The Archaeology and Environmental History of the Southern Deserts. Editors M. Smith, and P. Hesse (Canberra: National Museum of Australia Press), 73–90.
Latour, B. (2005). Reensamblar lo social. Una introducción a la teoría actor-red. Buenos Aires: Manantial.
Lazzari, M. (1999). “Distancia, espacio y negociaciones tensas: El intercambio de objetos en arqueología,” in Sed non satiata. Teoría social en la arqueología latinoamericana contemporánea. Editors A. Zarankin, and F. Acuto (Buenos Aires: Ediciones del Tridente), 117–151.
Le Paige, G. (1964). El precerámico en la cordillera atacameña y los cementerios del Período Agroalfarero de San Pedro de Atacama. Antofagasta, Chile: Anales de la Universidad del Norte, Vol. 3.
Lechtman, H. (1977). “Style in Technology. Some Early Thoughts,” in Material Culture: Styles, Organization, and Dynamics of Technology. Editors H. Lechtman, and R. S. Merrill (St. Paul: West Publishing Co), 3–20.
Lemonnier, P. (1986). The Study of Material Culture Today: Toward an Anthropology of Technical Systems. J. Anthropol. Archaeol. 5, 147–186. doi:10.1016/0278-4165(86)90012-7
Marinovic, N., and Lahsen, A. (1984). Hoja Calama, Región de Antofagasta. Santiago: Servicio Nacional de Geología y Minería. Carta Geológica de Chile N°58, 140. Sernageomin1:250.000.
Menzies, A., Uribe, M., Erazo, F., Ossandon, C., and Fonseca, P. (2015). “Automated Mineralogical Analysis of Archaeological Samples from Northern Chile: Case Study II – Ceramics,” in XIV Congreso geológico chileno: 4-8 de octubre 2015. La Serena, Colegio de Geólogos de Chile, 371–374.
Moraga, A., Chong, G., Fortt, M. A., and Henríquez, H. (1974). Estudio geológico del Salar de Atacama, Provincia de Antofagasta, 29. Santiago: Instituto de Investigaciones Geológicas. Boletín, 59.
Núñez, L. (1991). Cultura y conflicto en el oasis de San Pedro de Atacama. Santiago: Editorial Universitaria, 289.
Núñez, L. (1984). “Tráfico de complementariedad de recursos entre las tierras altas y el Pacífico en el Área Centro Sur Andina,” in Tesis Doctoral en Antropología (Tokio: Departamento de Antropología Cultural, Universidad de Tokio).
Ogalde, J., Korpisaari, A., Riera-Soto, C., Arriaza, B., Paipa, C., Leyton, P., et al. (2020). Archaeometric Analysis of Ceramic Production in Tiwanaku State (C. 500-1000 Ce): An Exploratory Study. Archaeometry 63, 53–67. doi:10.1111/arcm.12597
Orellana, M. (1989). Los tipos alfareros tempranos de Calar y su contexto aldeano. Paleoetnológica 5, 73–86.
Pereyra Domingorena, L. (2015). Estudio petrográfico de la cerámica arqueológica del primer milenio D.C. al sur de los valles calchaquíes (Noroeste Argentino). Chungara 47 (10), 1–11. doi:10.4067/S0717-73562015005000018
Pirrie, D., Butcher, A. R., Power, M. R., Gottlieb, P., and Miller, G. L. (2004). Rapid Quantitative Mineral and Phase Analysis Using Automated Scanning Electron Microscopy (QEMSCAN): Potential Applications in Forensic Geoscience. Geol. Soc. Lond. Spec. Publ. 232, 123–136. doi:10.1144/gsl.sp.2004.232.01.12
Pirrie, D., Power, M. R., Rollinson, G. K., Wiltshire, P. E. J., Newberry, J., and Campbell, H. E. (2009). “Automated SEM-EDS (QEMSCAN®) Mineral Analysis in Forensic Soil Investigations; Testing Instrumental Variability,” in Criminal and Environmental Soil Forensics. Editors K. Ritz, L. Dawson, and D. Miller (Springer), 411–430.
Puente, V. (2012). Atravesando fronteras. Prácticas compartidas e identidades sociales negociadas durante el tardío prehispánico. Una discusión desde la alfarería ordinaria del Valle del Bolsón (Belén, Catamarca). Relac. Soc. Argent. Antropol. 37, 65–87.
Quinn, P. S. (2013). “Ceramic Petrography,” in The Interpretation of Archaeological Pottery & Related Artefacts in Thin Section (Oxford: Archaeopress).
Ramírez, C. F., and Gardeweg, M. (1982). “Hoja Toconao, Región de Antofagasta,” in Carta Geológica de Chile N°54 (Santiago: Servicio Nacional de Geología y Minería), 122. Sernageomin1:250.000.
Rice, P. M. (2015). Pottery Analysis, A Source Book. Second Edition. Chicago: University of Chicago Press.
Riera-Soto, C. (2019). Estudio petrográfico de las cerámicas formativas en la cuenca del río Vilama y oasis de San Pedro de Atacama (Norte de Chile): Materias primas en circulación, tesis de maestría. San Pedro de Atacama: Instituto de Investigaciones Arqueológicas y Museo, Universidad Católica del Norte.
Riera-Soto, C., Uribe, M., Menzies, A., and Barraza, M. (2020). Avances en petrografía automatizada: cerámicas tempranas de Guatacondo, Norte de Chile (900 a.C – 200 d.C.). Bol. Arqueol. PUCP 26, 141–157. ISSN 1029-2004. doi:10.18800/boletindearqueologiapucp.201901.009
Ryan, W. B., Carbotte, S. M., Coplan, J. O., O'hara, S., Melkonian, A., Arko, R., et al. (2009). Global Multi‐Resolution Topography Synthesis. Geochem. Geophys. Geosystems 10 (3). doi:10.1029/2008GC002332
Rye, O. (1981). Pottery Technology: Principles and Reconstruction. Manuals on Archaeology 4, Taraxacum, Washington. doi:10.1525/aa.1982.84.3.02a00810
Salisbury, M. J., Jicha, B. R., De Silva, S. L., Singer, B. S., Jiménez, N. C., and Ort, M. H. (2010). 40Ar/39Ar Chronostratigraphy of Altiplano-Puna Volcanic Complex Ignimbrites Reveals the Development of a Major Magmatic Province. Geol. Soc. Am. Bull. 123 (5-6), 821–840. doi:10.1130/b30280.1
Sanhueza, L. (2004). Estilos tecnológicos e identidades sociales durante el Período Alfarero Temprano en Chile Central: Una mirada desde la alfareríaTesis para optar al grado de Magíster en Arqueología. Santiago: Universidad de Chile.
Shanks, M., and Tilley, C. (1987). Social Theory and Archaeology. Albuquerque: University of New Mexico Press.
Shepard, A. O. (1956). Ceramics for the Archaeologist. Washington D.C.: Carnegie Institution of Washington. The Foundational Book.
Sillar, B., and Tite, M. S. (2000). The Challenge of Technological Choices for Materials Science Approaches in Archaeology. Archaeometry 42 (1), 2–20. doi:10.1111/j.1475-4754.2000.tb00863.x
Sinclaire, C., Uribe, M., Ayala, P., and González, J. (1997). “La alfarería del Período Formativo en la Región del Loa Superior: Sistematización y tipología,” in Actas del XIV Congreso Nacional de Arqueología Chilena, Copiapó, Chile, October 13–18, 1997 (Contribución Arqueológica), 285–314. Tomo II.
Soja, E. (1997). “The Socio-Spatial Dialectic,” in Reading Human Geography. The Poetics and Politics of Inquiry. Editors T. Barnes, and D. Gregory (London: Arnold), 244–255.
Stovel, E., and Echenique, E. (2015). Polished Household Thinwares from San Pedro de Atacama, Chile: Reflections on Shape and Color. Chungara 47 (3), 469–488. doi:10.4067/s0717-73562015005000044
Stovel, E. (2002). “The Importance of Being Atacameño: Political Identity and Mortuary Ceramics in Northern Chile,” in Doctoral of Philosophy in Antropology Dissertation (New York: Binghamton University State, University of New York).
Stovel, E., Whitehead, W., and Deibel, M. (2013). Relaciones cerámicas y sociales entre San Pedro de Atacama y el Loa Superior durante el Período Intermedio Tardío a través del análisis de fluorescencia de rayos x portátil. Estud. Atacameños 46, 47–60. doi:10.4067/s0718-10432013000200004
Tarragó, M. (1989). Contribución al conocimiento arqueológico de las poblaciones de los oasis de San Pedro de Atacama en relación con los otros pueblos puneños, en especial el sector septentrional del valle Calchaquí,” in Tesis de doctorado en Historia, Especialidad Antropología (Rosario: Universidad Nacional de Rosario, Facultad de Humanidades y Artes).
Thomas, C., Massone, C., and Benavente, A. (1989). Sistematización cerámica de seis yacimientos de la Provincia El Loa (II Región). Paleoetnológica 5, 121–151.
Tomlinson, A. J., Blanco, N., and Dilles, J. H. (2010). “Carta Calama, Región de Antofagasta,” in Servicio Nacional de Geología y Minería, Carta Geológica de Chile (Santiago). 1:50.000.
Uribe, M. (2006). “Sobre cerámica, su origen y complejidad social en los Andes del Desierto de Atacama, norte de Chile,” in Esferas de interacción prehispánicas y fronteras nacionales modernas. Los Andes Sur Centrales. Editor H. Lechtman (Lima: Instituto de Estudios Peruanos), 449–502.
Uribe, M., Vidal, E., Glascock, M., Menzies, A., Muñoz, M., and Roush, C. (2019). “A Compositional Characterization of Ceramic Production and Circulation During the Formative Period in Tarapacá, Northern Chile,” in Ceramics of the Indigenous Cultures of South America. Studies of Production and Exchange Through Compositional Analysis. Editors M. D. Glascock, H. Neff, and K. J. Vaughn (Albuquerque: University of New Mexico Press), 161–172.
Uribe, M., and Vidal, E. (2015). “Pottery and Social Complexity in Tarapacá: Reviewing the Development of Ceramic Technology in the Atacama Desert (Northern Chile),” in Ceramic Analysis in the Andes. Editor I. Druc (Blue Mounds, WI: Deep University Press), 15–35.
Uribe, M., and Vidal, E. (2012). Sobre la secuencia cerámica del Período Formativo de Tarapacá (900 a.C.-900 d.C.): Estudios en Pircas, Caserones, Guatacondo y Ramaditas, Norte de Chile. Chungara 44 (2), 209–245. doi:10.4067/s0717-73562012000200003
Ward, I., Merigot, K., and Mcinnes, B. I. A. (2018). Application of Quantitative Mineralogical Analysis in Archaeological Micromorphology: A Case Study from Barrow Is., Western Australia. J. Archaeol. Method Theory 25, 45–68. doi:10.1007/s10816-017-9330-6
Keywords: automated SEM mineralogy, petrography, archaeometry, archaeological ceramics, Atacama Desert
Citation: Riera-Soto C, Agüero C, González-Maurel O, Uribe M and Menzies A (2022) Automated SEM Mineralogy and Archaeological Ceramics: Applications in Formative Period Pottery From the Atacama Desert. Front. Earth Sci. 10:807865. doi: 10.3389/feart.2022.807865
Received: 02 November 2021; Accepted: 26 May 2022;
Published: 29 June 2022.
Edited by:
Duncan Pirrie, University of South Wales, United KingdomReviewed by:
Wayne Powell, Brooklyn College (CUNY), United StatesPatrick Quinn, University College London, United Kingdom
Copyright © 2022 Riera-Soto, Agüero, González-Maurel, Uribe and Menzies. This is an open-access article distributed under the terms of the Creative Commons Attribution License (CC BY). The use, distribution or reproduction in other forums is permitted, provided the original author(s) and the copyright owner(s) are credited and that the original publication in this journal is cited, in accordance with accepted academic practice. No use, distribution or reproduction is permitted which does not comply with these terms.
*Correspondence: Camila Riera-Soto, cnJzY2FtMDAxQG15dWN0LmFjLnph