- 1Key Laboratory of Exploration Technologies for Oil and Gas Resources, Ministry of Education, Yangtze University, Wuhan, China
- 2Hubei Cooperative Innovation Center of Unconventional Oil and Gas, Yangtze University, Wuhan, China
Hydrothermal fluid is one of the factors controlling Archean buried hill reservoirs in Bozhong 19-6. However, there are no clear studies focusing on the influence of hydrothermal alteration products and their lithological characteristics on reservoirs. Through characterization of the alteration reservoir and construction of a new subtraction model of the logging-rock mechanical alteration degree, the comprehensive uses of core, thin section, and electrical imaging logging data are considered as the research objects with metamorphic and igneous rocks. Thus, the relationship between lithologies with different alteration degrees and reservoir quality is revealed. The study shows that feldspar chloritization and sericitization are the main factors controlling the hydrothermal alteration of the reservoir; the overall alteration degree of igneous rocks is high, and the overall alteration degree of metamorphic rocks is low; the reservoir with strongly altered igneous facies is prone to forming dissolution pores, with strong reservoir inhomogeneity and poor reservoir performance (alteration degree is greater than 15%); the reservoir with weakly altered metamorphic facies is prone to developing fractures and a high reservoir productivity (alteration degree is 0); The reservoirs with altered metamorphic facies are numerous in the formation, spatially diverse in type, and second in reservoir quality only to those in the weakly altered metamorphic facies (alteration degree of 0–15%). This method is expected to provide a reference for quickly finding advantageous reservoirs in the Bohai Sag.
1 Introduction
When fluids or hydrothermal fluids act on rocks, their substances undergo physicochemical reaction, resulting in the loss of some or all of the rock’s minerals and the creation of new ones. The enrichment of carbon dioxide in the hydrothermal fluid is prone to the formation of carbonate rock metasomatism (Shibuya et al., 2007). Carbonate metamorphism facilitates the improvement of the physical properties of the reservoir (Ding et al., 2019), and dolomitization can theoretically increase the total porosity by 12–13% (Smith and Davies, 2006). Such alterations occurred in the development zone of fractured-vuggy reservoirs and the gas accumulation zone (Chen et al., 2015; Zhan et al., 2021). For closed geochemical systems larger than 2000 m, feldspar is easy to form into authigenic clay (Yuan et al., 2019). Secondary clay minerals generated by clayey alteration of rocks as filling, along with their own structural water and adsorptive water, reduced the reservoir property (Zhu et al., 2013; Jiang et al., 2019). According to the chemical index of alteration (CIA) (Nesbitt and Young, 1982), feldspar and dark minerals were more prone to alteration with an increased alteration degree (Wei et al., 2017). In the process of weathering, leaching, and hydrothermal flow, feldspar and biotite are very prone to alteration in the Bozhong 19-6 Archaean buried hill structural belt (Zhao et al., 2016). Scholars believe that strong hydrothermal alteration has caused complex lithology and dissolution pores (Hou et al., 2019; Xu et al., 2019), but they did not specifically clarify the impact of different altered rocks on the reservoir.
In the current methods for evaluating rock hydrothermal alteration, scholars have carried out the following studies on igneous reservoirs. Through altered cores at different depths, the original structural background of the altered rock samples is inferred (Pandarinath, 2015). X-ray diffraction analysis of volcanic rocks indicates altered clay minerals that have a strong influence on logging response characteristics (Wang et al., 2013). The neutron log was used to identify the neutral igneous alteration formations (Wang et al., 2018). The mixed matrix volume model for clay produced by alteration was established to calculate water saturation (Zhang et al., 2012). The principal component analysis is used to calculate the porosity of altered reservoirs (Shen et al., 2012). Based on logging response characteristics, a clay alteration degree index is established to semi-quantitatively evaluate alteration effects (Gao et al., 2019). In logging rock mechanics, the predicted rock mechanics parameters are usually used to evaluate brittleness and in situ stress (Diao, 2013; Nagano et al., 2015), which play a crucial role in oil and gas stimulation, frack forecasting, and operation parameter design (Ameen et al., 2009; Zhang, 2012; Yin et al., 2016; Li et al., 2017). Due to rock alteration, the mineral composition and internal texture of rocks will inevitably undergo irreversible shifts. The mechanical parameters of the rock can reflect the material composition and structural characteristics of the rock. Therefore, a new way of logging-rock mechanics parameters has been used to quantitatively evaluate altered formations.
The Bozhong 19-6,100 billion-cubic meter large gas field in the Bohai Bay Basin opens a new chapter in deep gas exploration in the Bohai Sea. However, the lithological diversity of the buried hill gas reservoir is due to the rich types of early original rocks and the multi-stage metamorphism in later stages, resulting in mainly metamorphic rocks and some later igneous rocks (Xu et al., 2019). Hydrothermal flow is among the controlling factors of the reservoir (Xue et al., 2021). During its geological history, the buried hill structural belt experienced multiple phases of magmatic activity and long-term exposure to the surface, which subjected the rocks to weathering, denudation, and hydrothermal fluids, causing alteration of feldspar minerals (Xu et al., 2020). The rocks are subjected to hydrothermal alteration by both carbonate metasomatism and clay alteration (Hou et al., 2019), producing a variety of secondary minerals, especially chlorite, sericite, and iron dolomite.
The study area underwent both igneous rock alteration and metamorphic rock alteration, as well as clay alteration and carbonate metasomatism of rocks in formations. Based on an analysis of experimental core data and log response characteristics, it is determined that mica is metasomatized by iron dolomite, and feldspar is subjected to sericitization and chloritization in the buried hill formations; sericite and chlorite are the main clay alteration minerals, and iron dolomite is the main carbonate alteration mineral. Since there is no universal method for the quantitative evaluation of alteration effects, a new type of alteration degree difference model and a quantitative evaluation chart have been established by combining logging curves and rock mechanics parameters to provide a new understanding and guidance for the reservoir identification and evaluation of Archean buried hill 19-6 in Bozhong.
2 Geological Setting and Alteration Characteristics
Figure 1A illustrates that the Bozhong Sag is the largest Cenozoic subsidence sag center in the Bohai Bay Basin (Zhu et al., 2020), which is located in the thinnest position of the crust in the Bohai Bay Basin, showing a high geothermal value (Xu et al., 2020). The Bozhong 19-6 Archean buried hill structure is located in the southwestern part of the Bozhong Sag (Figure 1A). It is composed of a metamorphic rock base, covered with carbonate, igneous, and glutenite, and interspersed with igneous veins. In the period of geological history, it has experienced multi-stage tectonic movement transformation and weathering leaching, thus forming a large-scale fracture system. The deep hydrothermal fluid in the formation then enters the reservoir space through the fractures and faults, causing large-scale alteration of the rocks in the Bozhong 19-6 reservoir.
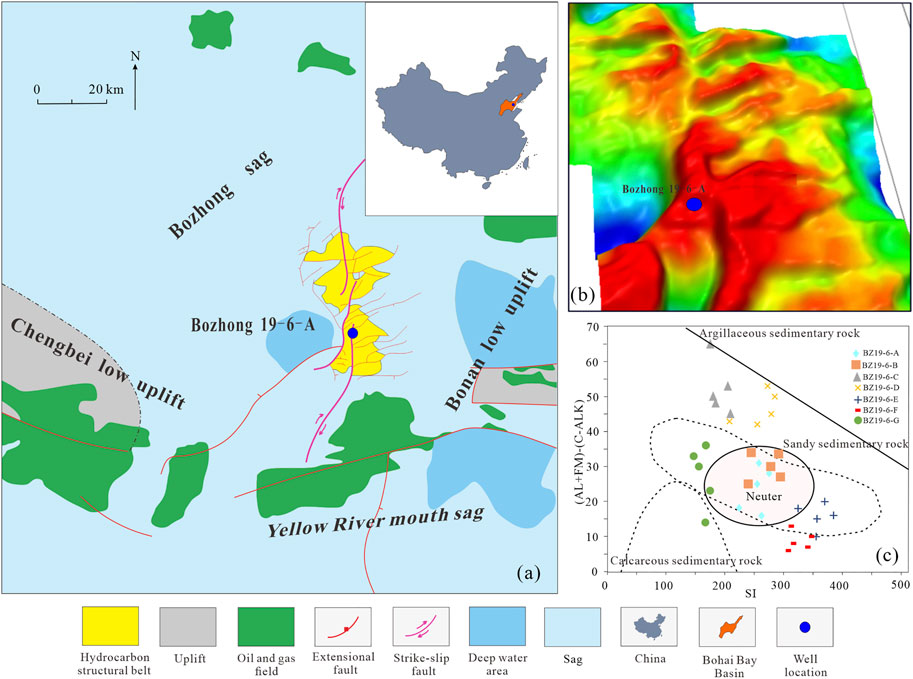
FIGURE 1. Geological background of the Bozhong 19-6 area. (A) Bozhong 19-6 structure and the location of sampling wells (Hou et al., 2019); (B) Three-dimensional view of the Archean buried hill structure; (C) Archean buried hill original rock restoration (Simonen et al., 1953).
The Archean buried hill of Bozhong 19-6-A is taken as the research object. The well A area is located in the southern area of the Bozhong 19-6 structure. As a whole, it is an anticline structure complicated by a series of faults, with a large trap area and a reservoir burial depth of more than 3,900 m (Figure 1B). Therefore, well A is generally strongly affected by deep hydrothermal fluids.
According to the original rock restoration (Figure 1C), it can be confirmed that the lithology of well A is derived from neutral magmatic rocks. The rocks are mainly gneiss, neutral intrusive rocks (diorite porphyrite), volcanic rocks (andesite), and other mixed rocks. In terms of mineral composition, plagioclase, potash feldspar, and quartz are the main minerals, and they contain a small amount of dark minerals and other minerals (Xu et al., 2020). Neutral magmatic rocks have a strong degree of alteration, in between acidic magmatic rocks and ultrabasic magmatic rocks (Hu, 1980).
Logging data, physical core samples, and rock thin-section samples of well Bozhong 19-6-A, X-ray diffraction data, and gas tests of Bozhong 19-6-A well, Bozhong 19-6-B well, and Bozhong 19-6-C well are required.
2.1 Characteristics of Rock Alteration
1) Altered metamorphic rocks are typically monzonite gneiss and plagioclase gneiss: monzonite gneiss (Figure 2A) features a gneiss-like texture and are subject to slight alteration; the rock grains are coarse and inlaid with each other. Quartz and siliceous matter are recrystallized, plagioclase is subjected to sericitization, and iron dolomite metasomatism is found in the biotite enrichment areas. The cracks are completely filled with iron dolomite, iron minerals, and felsic, and a few dissolution holes are seen. The plagioclase gneiss (Figure 2B) features a scaly granoblastic and gneiss-like texture, with the mineral grains being coarse and tightly inlaid. The quartz features wavy extinction, sericitization, and chloritization of plagioclase, with the mica dominated by biotite and less of muscovite. The cracks are filled with iron dolomite. 2) Altered igneous rocks are typically andesite and porphyry: altered andesite (Figure 2C) in volcanic rocks is in interleaved structure and cataclastic structure, with strong rock alteration and mudding on the surface. Plagioclase is sericitized and chloritized, and a small amount of potassium feldspar and quartz and more authigenic pyrite are mixed in later stages. Residual intergranular pores can be seen in the fragmentation zone, and the fraction of coverage is less than 1%. The intrusive rocks (Figure 2D) are coarsely faceted and strongly altered, with plagioclase showing mica, chlorite, a small amount of potassium feldspar and a small amount of quartz, with siderite, dolomite, and pyrite visible between the slats, and no effective pore space.
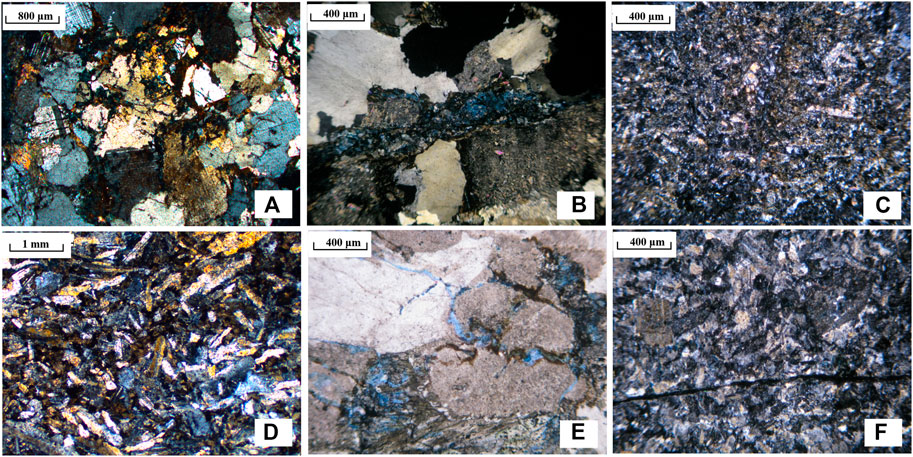
FIGURE 2. BZ19-6-A well rock chips. (A) Monzonite gneiss, 3,925 m, perpendicular polarized light; (B) Plagioclase gneiss, 4,357 m, perpendicular polarized light; (C) Altered andesite, 3,981.5 m, perpendicular polarized light; (D) Diorite porphyrite, 4,076 m, perpendicular polarized light; (E) Local dissolution joint, 4,254 m, plane polarized light; (F) Filling of iron dolomite and pyrite, 4,005 m, perpendicular polarized light.
It can be observed from the thin section of rocks (Figure 2A–D, ) that hydrothermal alteration can give rise to carbonate metasomatism and clay alteration. In the metamorphic rocks and igneous rocks, the alteration universally occurred such as iron dolomite metasomatism (carbonate metasomatism), sericitization, chloritization (clay alteration), and associated iron minerals. The degree of hydrothermal alteration of igneous rocks is stronger than that of metamorphic rocks.
2.2 Log Characterization
2.2.1 Reservoir Characteristics
The main types of the reservoir space in the Bozhong 19-6 buried hill area are fracture, dissolution pore, and pore fracture (Tong et al., 2012; Xue and Lei, 2018). The porosity of the reservoir intervals ranges from 1.2 to 12.8%, with an average of 4.3%. The permeability ranges from 0.02 mD to 11.80 mD, with an average of 0.97 mD (Hou et al., 2019).
In the long-term weathering and leaching process of the buried hill reservoir, feldspar minerals are altered and dissolved. The altered iron dolomite features the unstable chemical properties of calcium carbonate and can also be dissolved to form dissolved pores (Figure 2E), resulting in a pore space, that can also be used as cement (Zhu et al., 2016; Zhao et al., 2018; You et al., 2018). Sericite and chlorite feature stable chemical properties, good dispersion, and suspension in aqueous media and organic solvents. They contain a large amount of structural water and adsorbent water, which serve as a large amount of clay cementation filling in the pores (Figure 2F) and accelerate the dissolution. Therefore, the log responses of the reservoir will exhibit the marked characteristics of water and clay content (Zhu et al., 2013), relatively high pores, and relatively low permeability.
2.2.2 Reservoir Physical Properties
From the comprehensive logging (Figure 3), it can be seen that the physical properties of the entire reservoir are very irregular from a vertical perspective. It includes 3,968–3,986 m (Figure 2C 3,981.5 m), with the core porosity (CPOR) between 9.3 and 17% and the core permeability (CPERM) between 0.024 mD and 0.03 mD, while granitic gneiss is not significantly altered at 3,900–3,010 m with CPOR of 8.7% and CPERM of 0.26 mD. Through comparison, it is found that the reservoir transformation by hydrothermal alteration has poorer reservoir properties: CPOR (9.3–17%) is higher than the average porosity of the reservoir (1.2–12.8%), but CPERM (0.024–0.03 mD) is lower than the average permeability of the reservoir (0.02–11.80 mD).
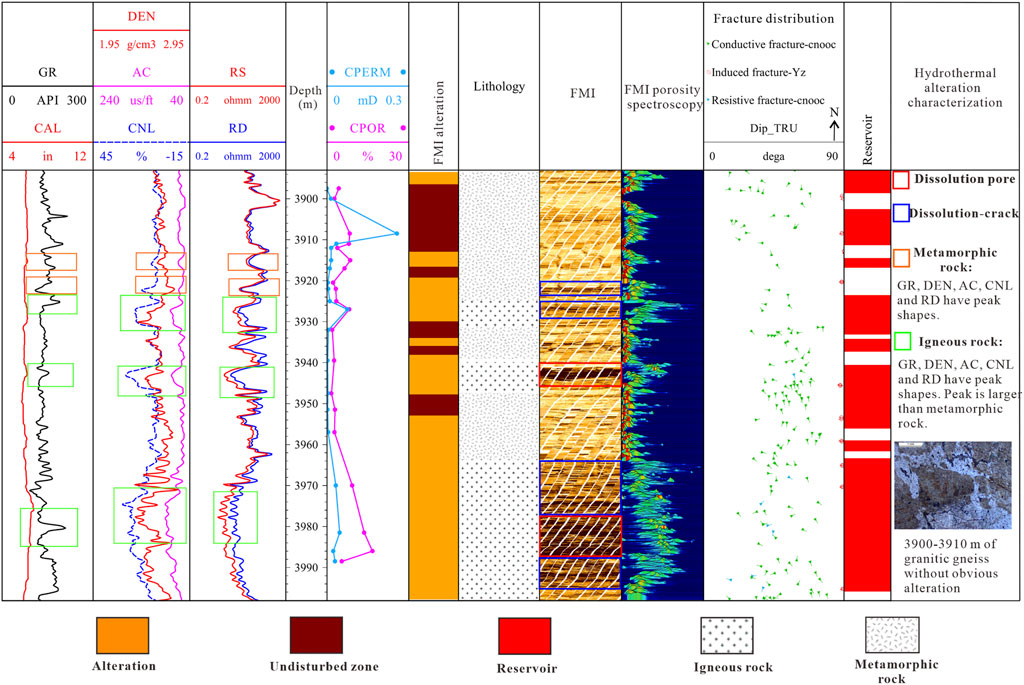
FIGURE 3. Composite log in well Bozhong19-6-A. Characteristics of buried hill reservoirs by logging curves, core physical property data, and electrical imaging.
2.2.3 Log Response Characteristics
According to the characteristics of clay minerals and structural water in the altered reservoir, from the logging response characteristics (Figure 3), the natural gamma ray (GR) tends to increase, and its average lower limit is higher than that of the undisturbed formations (Table 1); its curve spikes occur, as shown at 3,945 m and 3,960–3,980 m. The logging density (DEN) tends to decrease, and its average lower limit is lower than that of the undisturbed formations (Table 1); its curve spikes occur, as shown at 3,940–3,948 m and 3,960–3,980 m. The logging neutron (CNL) tends to increase, and the average lower limit is higher than that of the undisturbed formations (Table 1), and its serrated spikes occur, as shown at 3,925–3,931 m and 3,960–3,990 m. Acoustic slowness (AC) tends to increase, and its average lower limit is higher than that of the undisturbed formations (Table 1), with its curve fluctuation being relatively gentle, as shown at 3,940–3,948 m and 3,960–3,980 m. The dual lateral resistivity (RD, RS) has a decreasing trend, and its average lower limit is lower than that of the undisturbed formations (Table 1), and its curve spikes occur, as shown at 3,940–3,948 m.

TABLE 1. Average lower limit of logging curves in the buried hill formations of the Bozhong 19-6-A area.
2.2.4 Electrical Imaging Features
The electrical imaging porosity spectrum reflects the distribution of pore sizes in the formation wash zone. When a layer belongs to a relatively homogeneous formation, the porosity spectrum mostly features a single spike shape; when a layer is highly heterogeneous, the porosity spectrum shows multiple or irregular peaks. It can be observed from Schlumberger’s formation microscanner image (FMI) and the FMI porosity spectrum in Figure 3 that the dark imaging area of the strongly altered reservoir is dissolution pores, and the porosity spectrum presents an irregular and multi-peak shape, while the unaltered reservoir at 3,902–3,908 m only presents a gentle pattern of a single spike or double spikes. The electrical imaging data also found that the reservoir transformation by hydrothermal alteration has strong heterogeneity and large pores. Electrical imaging and identification of corresponding rock thin slices can briefly obtain formation alteration in well A (Figure 3 FMI alteration division).
3 Methods
3.1 Logging Model for Determining the Degree of Rock Alteration
The alteration of rock exerts an influence on the mineralogical composition, pore space structure, and flow of the rocks. The log response is an integrated reflection of rock properties. Hence, when the rock is strongly altered, the log response of various curves will show particular characteristics.
Therefore, the ratio method is performed separately for the CNL and DEN curves and the AC and RD curves, which have a strong response to the alteration effect. The ratio method can not only reflect the degree of alteration but also eliminate the influence of different lithologies, and the following model is obtained (Gao et al., 2019):
1) Indicator of CNL–DEN alteration (Ind) is shown as follows:
Due to the degree of alteration, the ratio of CNL–DEN will increase with the degree of alteration, and the increase in the clay content will lead to the increase of CNL and the decrease of DEN. Generally, the decrease in the density ranges from 0 to 10%, and the increase in neutron ranges from 0 to 50%. Therefore, the weight of the density curve should be increased, and the empirical weight a is taken as 2. CNL stands for the compensation neutron log, %; DEN stands for the compensation density, g/cm3.
2) Indicator of AC-RD alteration (Iar) is shown as follows:
Due to the degree of alteration, the ratio of AC–RD will increase with the degree of alteration, and the increase in the clay content will give rise to the increase of AC and the decrease of RD. AC is less affected by lithology, while RD is more affected (the resistivity values of igneous rocks and metamorphic rocks differ greatly). Similarly, the value of empirical weight is taken as 0.5. AC stands for the acoustic slowness, μs/ft; Rt stands for the formation resistivity, Ω·m.
Based on Eqs 1, 2, the factor for logging alteration degree (IA) can be followed:
3.2 Rock Mechanics Model for Determining the Degree of Rock Alteration
The mechanical parameters of the rock reflect the mechanical properties of the rock. The time difference between P-wave and S-wave is taken from the array acoustic logging data, and the Poisson’s ratio, elastic modulus, volume modulus, and shear modulus of the rock are calculated by combining the logging density to determine the integrity, stability, and fracture degree of the rock (He et al., 2008; Liu et al., 2009; Liu et al., 2018). The log rock dynamics parameters have good parameter continuity. Experimental studies show that the dynamic and static parameters of a complete rock are very close and can basically be converted into each other (Liu et al., 2009).
The rock stability factor (Rg) is the product of the rock volume modulus and shear modulus. Since alteration will destroy the morphological structure of the undisturbed rock, the more serious the morphological damage of the rock, the smaller the stability coefficient will be. Its expression is as follows:
where Kb stands for the dynamic volume modulus of elasticity, GPa; G stands for the dynamic shear modulus, GPa;
The rock fracture coefficient (Rf) eliminates the influence of rock properties on the elastic modulus. The larger the deformation of rock mass, the smaller the elastic modulus is and the larger the rock fracture coefficient is, which can be as follows:
where
Rf–Rg ratio method: the form and structure of the undisturbed rock are changed under the alteration. The stronger the alteration is, the larger the fracture coefficient of rock is and the smaller the stability coefficient of rock is. Since the rock fracture coefficient is affected by alteration to a slightly greater extent than the rock stability coefficient, the order of magnitude difference between the parameters is so great that empirical weights can be applied to the rock stability coefficient to reduce the effect of lithology. The Rf–Rg mechanical alteration degree factor of the rock is as follows:
where the value of experience weight a is the same as Eq. 1.
3.3 Alteration Degree Subtraction Model
To make the evaluation of the undisturbed formation quantitative, the normalized IA curve is differentiated from the normalized IB curve, and a value less than or equal to 0 is considered to be an undisturbed formation. Combined with Eqs 3–6, the mathematical expression of the alteration degree model is defined as follows:
where the conventional logs and the rock mechanics parameters need to be normalized, and the dimension is 1. As shown in Figure 3, the interval of 3,968–3,986 m is regarded as the standard alteration layer, and the interval of 3,902–3,908 m is regarded as the standard undisturbed layer. Taking the standard layer as the reference, the value of a is finally determined to be 0.298 by using the core calibration.
Well Bozhong 19-6-A as the research object (Figure 4), Iar, Ind, and IA obtained from logging curves, and IB obtained from rock mechanics parameters can all be changed by peak values to reflect the degree of formation alteration. Izby has inherited the characteristics of IA and IB well, and the undisturbed formation less than 0 can be seen intuitively through optimization. The degree of alteration of igneous rocks is stronger than that of metamorphic rocks in the figures, which is consistent with the results of rock thin slices and logging response characteristics.
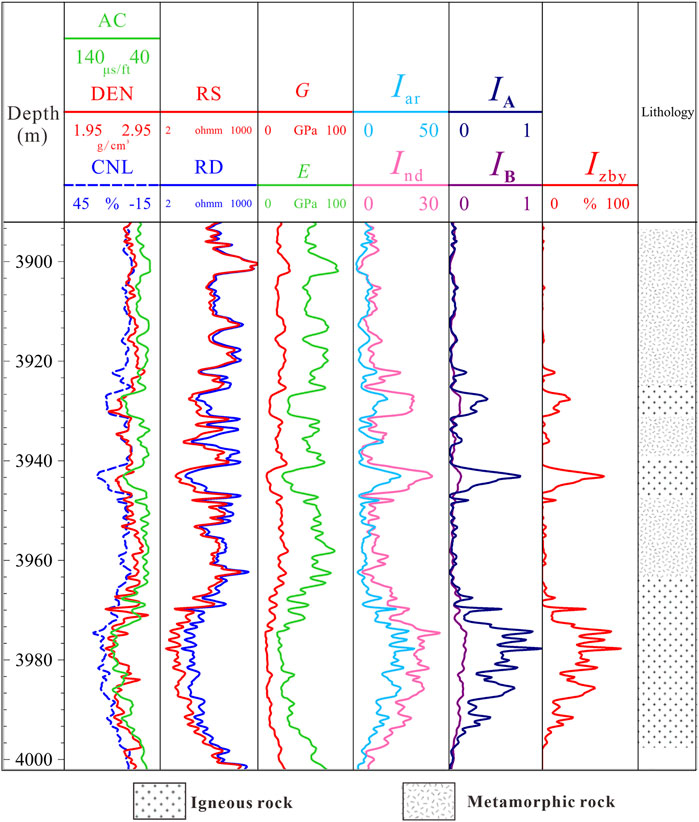
FIGURE 4. Degree of hydrothermal alteration of buried hill formation. Logging curves and rock mechanics parameters indicate the degree of alteration according to the peak size.
4 Results and DISCUSSION
4.1 Reservoirs Subjected to Hydrothermal Alteration
The reservoir in the Archean buried hill structural belt of Bozhong 19-6 was subjected to very strong weathering-leaching and hydrothermal fluid control processes (Xue et al., 2021). Through the transformation of hydrothermal alteration, dissolution pores are common in the reservoir. Rock thin sections (Figure 2), electrical imaging, and rock physics (Figure 3) show that the more strongly the rocks were altered by hydrothermal fluids, the larger the dissolution pores that are likely to form. There may be a certain degree of positive correlation between the degree of reservoir alteration and the development of fractures. Undisturbed formation fractures are developed, and strongly altered formation dissolution pores are developed. Therefore, the stronger the degree of alteration, the less developed are the fractures.
Fracture type reservoirs are mainly subject to fracture systems formed by the action of multiple phases of geological tectonic movement and weathering leaching under tectonic uplift. However, structural uplift has caused the buried hill to be exposed to the surface for a long time, and deep thermal fluid enters the metamorphic buried hill reservoir through cracks and faults. In the process of weathering and leaching, the dissolved CO2 in fresh water entered into the metamorphic buried hill through fractures and joints and reacted with the felsic minerals, forming enlarged dissolved pores along the fractures, which greatly improved the reservoir properties. Deeply heated fluids and magmatic-hydrothermal fluids lead to alteration of the feldspar, including dissolution, causing further fracture expansion to form pores, while in closed geochemical systems greater than 2000 m, feldspar dissolution increases the pore space, but its alteration of authigenic clay formation leads to reduced seepage capacity (Yuan et al., 2019). In general, the inhomogeneity of dissolved pore-type reservoirs will be stronger.
Under the action of hydrothermal alteration, the original rock produced new minerals such as iron dolomite, chlorite, sericite, and iron minerals, which caused the lithology to change. This complex lithology makes the heterogeneity of the reservoir stronger, and the secondary minerals reduce the effectiveness of the reservoir, usually resulting in high porosity and low permeability. It is often impossible to accurately evaluate the key physical parameters of the reservoir.
At present, it has been discovered that there are mainly two kinds of alterations: clay alteration (sericitization and chloritization) and carbonate metasomatism (dolomitization). However, based on the current available data, only thin slices have found evidence of carbonate metasomatism. It is speculated that most of the dolomite has been dissolved under the early leaching action, which promoted the formation of dissolved pores.
4.1.1 The Main Controlling Factors of the Degree of Rock Hydrothermal Alteration
Through the characterization of rock alteration of buried hill reservoirs, the primary minerals of metamorphic rocks and igneous rocks have undergone chloritization, sericitization, and iron dolomitization. According to the rock thin sections (Figure 2), the metamorphic rocks and igneous rocks have obvious alteration characteristics of chloritization and sericitization. The rock surface is mudded. Log curve characteristics (Figure 3) show that the strong hydrothermal alteration reservoir has typical clay characteristics (Zhu et al., 2013): the log curve shows the high value of CNL relative to the undisturbed formation, the low value of DEN relative to the undisturbed formation, the high value of AC relative to the undisturbed formation, and the RD is lower than the undisturbed formation.
Thin section and well logging studies show that the clay alteration (chloritization and sericitization) of rocks is the main controlling factor of rock hydrothermal alteration in the Bozhong 19-6 Archean buried hill. It provides a reliable basis for the establishment of alteration in degree models and reservoir evaluations.
4.1.2 Evaluation Results of Hydrothermal Alteration
By analyzing log curves of 61 cores with different lithologies in Bozhong 19-6, the Izby will be connected with CNL (Figure 5A), RD (Figure 5B), AC (Figure 5C), and DEN (Figure 5D), respectively. Since the main controlling factor for the degree of rock alteration is the clay of the rock, the characteristic value of the logging response of the carbonaceous mudstone was used as a threshold to distinguish the strong alteration effect, and finally, the rock alteration degree chart was drawn (Figures 5A–D).
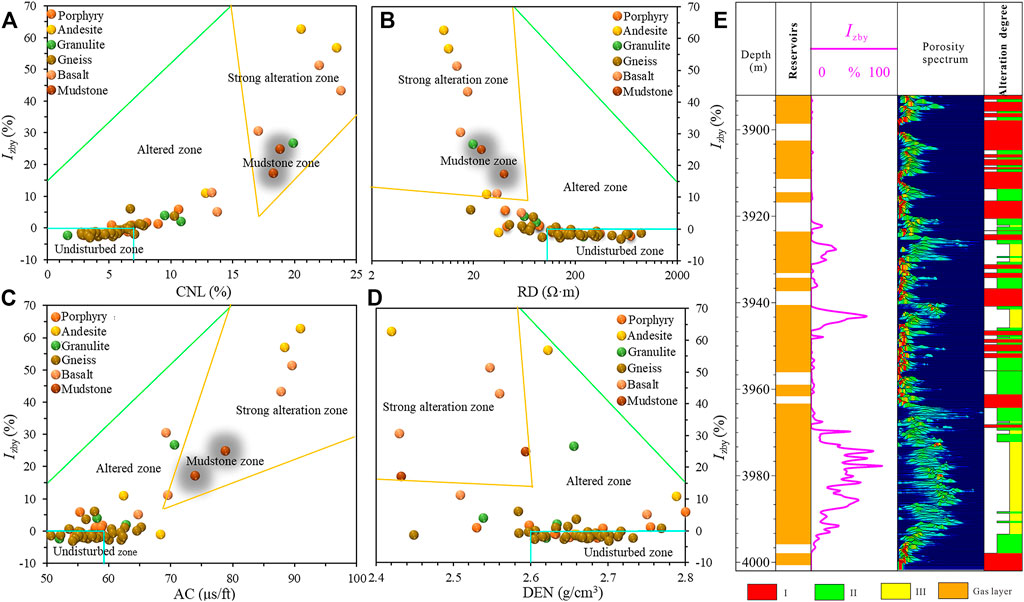
FIGURE 5. Qualitative evaluation of the alteration degree. (A) Izby and CNL; (B) Izby and RD; (C) Izby and AC; (D) Izby and DEN. (E) Division of altered strata based on the Izby peak. The mudstone zone is a range of mudstone characteristic values.
It can be observed from Figure 5 that CNL and RD have the best quality control of the degree of alteration; AC has the second best quality control of the degree of alteration; DEN has the worst quality control of the degree of alteration. The metamorphic series are the least altered, with gneisses less than 5 and granulite approximately equal to 5. The intrusive rock is the next most altered, with diorite porphyries approximately equal to 5. The volcanic rocks are the most altered, with andesites and basalts greater than 10.
Considering that the weak hydrothermal alteration will still maintain the integrity of the original rock, the logging response has no obvious characteristics. Therefore, this kind of slight alteration is also considered as undisturbed rock hereof. The buried hill altered formation is divided into three classes (Table 2), according to the alteration degree evaluation chart (Figures 5A–D): class I is the undisturbed formation, class II is the altered formation, and class III is the strongly altered formation.
The reservoirs in the Bozhong 19-6 Archean buried hill structural zone are gas layers, as can be seen from the gas layer in well A (Figure 5E): the response of IA to the degree of alteration is more intense, but in gas reservoirs with small IA values, the response change is small. IB has the same phenomenon. Therefore, neither IA nor IB can quantitatively classify alteration reservoirs, but the undisturbed reservoirs less than 0 are distinguished by Izby. The strong alteration reservoirs greater than 15 are distinguished by Izby. Using the results of the evaluation (Table 2), the results of the entire formation were classified as I, II, and III (Figure 5E).
Based on the classification of the Izby alteration degree, it can be observed from the relationship between core porosity and Izby (Figure 6A) that the porosity for more than half of the altered rocks is 4.3% greater than the average porosity of the buried hill reservoir. As can be seen from the core permeability and Izby (Figure 6B), most rocks in classes II and III have lower permeability than in class I. Also, from Figure 6A and Figure 6B, it can again be seen that the permeability of class II and III altered rocks (Figure 6 near the data points marked near 8% porosity) is about 0.03 mD lower than the yellow points in the figures.
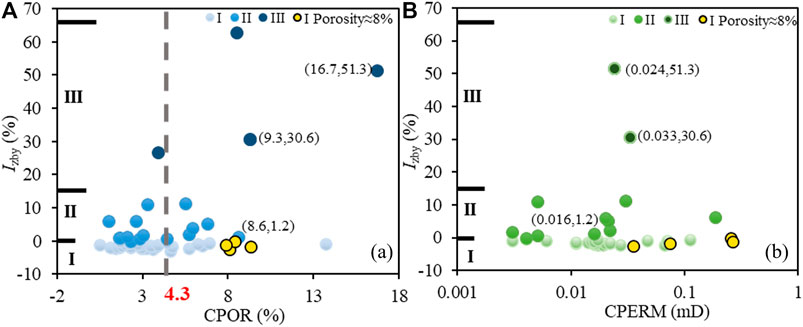
FIGURE 6. Correlation between physical properties and the alteration degree Izby. (A) Core porosity (CPOR) and Izby; (B) Core permeability (CPERM) and Izby. The different colored dots represent the class I, II, and III altered rocks, in which the yellow dots stand for the class I altered rocks with the porosity of 8%.
From the alteration degree of the rock (Figure 5A), alteration grade of the reservoir (Table 2), reservoir physical properties (Figure 6A), and electrical imaging logging characteristics, it is recognized that the lithology of the grade III alteration degree reservoir is a common volcanic rock and intrusive rock, and the reservoir space is mostly dissolution pores, with strong heterogeneity and poor reservoir capacity. Gneiss is common in the lithology of the reservoir with grade II alteration degree, and the reservoir space is mostly pore-fracture type, fracture type, and pore type, with strong heterogeneity. Gneiss is common in the lithology of class I undisturbed reservoirs, with relatively good reservoir homogeneity and easy to develop fractures.
4.2 Lithofacies Division Based on Hydrothermal Alteration Characteristics
Rock clay alteration is the main controlling factor of hydrothermal alteration. Therefore, the clay content of the whole rock X-ray diffraction (Figures 7A–C) with the rock named is selected, and it is found that the granitic gneiss is less affected by the alteration; plagioclase gneiss, monzonite gneiss, and cataclastic rock may be subject to alteration; and andesite and diorite porphyry are subject to strong alteration. In general, it can be seen that the alteration degree of gneiss is smaller than that of igneous rocks (Figures 7A–C).
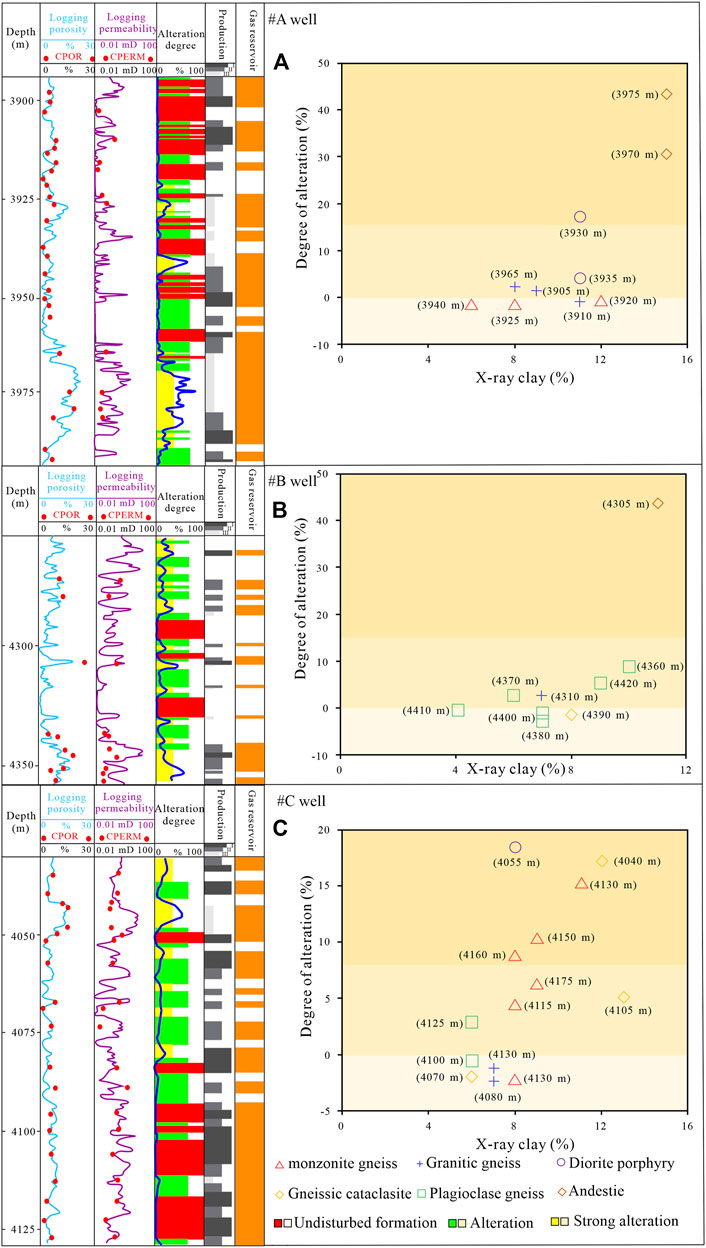
FIGURE 7. Relationship between clay minerals, physical properties, and the degree of rock alteration. (A) Archean buried hill of well Bozhong 19-6-A; (B) Archean buried hill of well Bozhong 19-6-B; (C) Archean buried hill of well Bozhong 19-6-C.
The reliability of the reservoir identification results was verified using Bozhong 19-6-A, Bozhong 19-6-B, and Bozhong 19-6-C. The variation of clay minerals, physical properties, and gas production in relation to the degree of rock alteration can be seen for well Bozhong 19-6-A (Figure 7A), well Bozhong 19-6-B (Figure 7B), and well Bozhong 19-6-C (Figure 7C). The clay mineral contents of wells A, B, and C have a positive correlation with the degree of alteration. The more intense the degree of alteration, the more obvious is the relationship. In the strongly altered formations, the pore space in each well increased, but the seepage ability did not improve.
According to the relationship between productivity classification (Figures 7A–C) obtained from the reservoir condensate gas test and alteration degree, it can be found that the reservoir productivity under strong alteration is the lowest; the reservoir productivity under alteration is good; the reservoir productivity under undisturbed formation is the highest.
Based on the rock properties, alteration degree, productivity, and clay content, the Bozhong 19-6 Archean buried hill reservoirs are divided into: 1) weakly altered metamorphic facies dominated by granitic gneiss; 2) altered metamorphic facies dominated by plagioclase gneiss; and 3) strongly altered igneous facies dominated by volcanic rocks (andesite) (Figure 8).
1) The rocks of weakly altered metamorphic rock facies are basically not altered. In rock analysis, it is found that granitic gneiss is the most stable. Generally, a small area of feldspar and mica is sericitized in thin sections. Under the electron microscope, the rock surface is dense and covered with a small amount of clay particles (Figure 8). 2) The rocks of altered metamorphic facies are partially altered. Monzonite gneiss and plagioclase gneiss are common in rock analysis. Generally, feldspar and mica can be seen in thin sections, and some are replaced by sericitization, chloritization, and ankerite. Under the electron microscope, there are few dissolution holes, and some of them are covered by clay particles on the rock surface (Figure 8). 3) Strong alteration—the rock alteration of igneous facies is serious. In the rock analysis, it is found that the thermal fluid alteration of volcanic rocks is the strongest, followed by intrusive rocks. Generally, it can be seen in the thin section that feldspar is basically replaced by mudding and carbonate, and the lithology is basically changed. Under the electron microscope, there are obvious dissolution pores, and they are covered by clay particles on the rock surface (Figure 8).
Based on the division of the formation alteration degrees of the three wells in Figure 7, reservoirs with weakly altered metamorphic facies occur in the undisturbed formation. This type of reservoir has developed fractures and high gas production capacity, which can be regarded as a high-quality reservoir with less distribution in the whole formation. Reservoirs with strongly altered igneous facies occur in strongly altered formations. This type of reservoir generally develops dissolution pores, which improves the reservoir space, but the alteration products fill the pore channels, which reduces the effectiveness of the reservoir. The reservoir productivity is low, and it can be regarded as a poor reservoir. The most distributed reservoir in the formation is the reservoir with alteration metamorphic facies. This results in various types of reservoirs, and the reservoir quality is between that of the aforementioned two reservoirs.
4.3 Quantitative Evaluation of the Effectiveness of Rock Hydrothermal Alteration Methods
Using the sensitivity of CNL, DEN, AC, and RD to the amplitude change of the altered formation, the quality of the curve is controlled by the ratio method, and finally, the degree of formation alteration is evaluated in the form of an index (Gao et al., 2019). IB uses the rock stability coefficient and rock fracture coefficient in dynamic rock mechanics parameters to indicate the degree of formation alteration by the ratio method, although IB can replace the logging response characteristics to indicate the degree of alteration. However, logging curve identification is relatively more sensitive in altered formations, with greater variation in curve amplitude (Figure 4). If the response of the logging curve is very sensitive to the altered formation, it is the opposite of non-altered undisturbed formation. It is combined with the idea that logging curves are more sensitive (greater variation in curve amplitude) than rock mechanics parameter identification in altered formations. Under the condition that the trends of IA and IB are consistent, IB is greater than or equal to IA in places with small alteration degrees, such as 3,900 m–920 m in depth (Figure 4). The final alteration degree subtraction model is based on the subtraction of IA and IB.
The advantage of this new method is that it can quantitatively evaluate the hydrothermal alteration of rocks and formations and has the characteristics of universality and easy realization. The disadvantage is that there are insufficient experimental data to account for alteration minerals to accurately assess the accuracy of the method. However, the accuracy of the method to identify the degree of hydrothermal alteration in reservoirs by capturing the high porosity and low permeability of altered reservoirs is 76% correct, which needs to be improved. In addition, due to the limitation of data, the evaluation system for the rock alteration degree is built on the previous studies, and there is no experimental analysis of the alteration degree under a certain environment, such as the changes under the conditions of being full of gas and the same temperature and pressure environment.
The relevant data to verify the accuracy of the quantitative evaluation of the degree of rock alteration are in the supplementary information of the study (Supplementary Table S1). Iφ represents the difference between the calculated porosity and core porosity, and IK represents the difference between the calculated permeability and core permeability. This method can also be used to verify the correctness of Eqs 6, 7 and to determine the
where φ is the calculated porosity, %; Cφ is the core porosity, %;
5 Conclusion
In this study, based on rock thin sections, petrophysical properties, electrical imaging, and logging response characteristics, we analyzed the hydrothermal alteration of the Bozhong 19-6 buried hill structural belt in the Bohai Bay Basin, eastern China. It is demonstrated that both the metamorphic rocks and igneous rocks in the buried hill formations were subjected to clay alteration (sericitization and chloritization) and carbonate metasomatism (iron dolomite metasomatism) to varying extents. Rocks are altered by hydrothermal fluids, and there are alteration products such as sericite, chlorite, iron dolomite, and other iron minerals, resulting in very complex lithology and strong heterogeneity of the reservoir. Rock clay alteration is the main factor controlling the degree of rock alteration.
A model for the quantitative evaluation of the degree of formation alteration was established based on neutron logging, density logging, resistivity logging, and acoustic logging with rock stability and rock fracture coefficients. The altered formations of buried hills are classified into class I (undisturbed formations), in which the degree of alteration is ≤0; class II (altered formations), in which the degree of alteration is 0–15; and class III (strongly altered formations), in which the degree of alteration is >15.
The type of altered lithofacies in the study area is further determined according to the degree of formation alteration, which is divided into weakly altered metamorphic lithofacies, altered metamorphic lithofacies, and strongly altered igneous facies. Weakly altered metamorphic facies reservoirs have fractures and high gas productivity and can be regarded as high-quality reservoirs. Strongly altered igneous facies reservoirs have dissolved pores, strong heterogeneity, and low gas productivity and can be regarded as poor reservoirs. Altered metamorphic facies produce various types of reservoirs, which are the most common type of reservoirs in the study area, and the quality of the reservoirs is good.
Data Availability Statement
The original contributions presented in the study are included in the article/Supplementary Material, further inquiries can be directed to the corresponding author.
Author Contributions
BZ designed the project and wrote the main manuscript. RP provided the funding. CZ and ZZ developed the main ideas. WS contributed to revising the manuscript. All coauthors actively contributed to the manuscript with comments, ideas, and suggestions.
Funding
This study was partly funded by the National Natural Science Foundation of China (No. 41472123).
Conflict of Interest
The authors declare that the research was conducted in the absence of any commercial or financial relationships that could be construed as a potential conflict of interest.
Publisher’s Note
All claims expressed in this article are solely those of the authors and do not necessarily represent those of their affiliated organizations, or those of the publisher, the editors, and the reviewers. Any product that may be evaluated in this article, or claim that may be made by its manufacturer, is not guaranteed or endorsed by the publisher.
Supplementary Material
The Supplementary Material for this article can be found online at: https://www.frontiersin.org/articles/10.3389/feart.2022.807659/full#supplementary-material
References
Ameen, M. S., Smart, B. G. D., Somerville, J. M., Hammilton, S., and Naji, N. A. (2009). Predicting Rock Mechanical Properties of Carbonates from Wireline Logs (A Case Study: Arab-D Reservoir, Ghawar Field, Saudi Arabia). Mar. Pet. Geology. 26 (4), 430–444. doi:10.1016/j.marpetgeo.2009.01.017
Chen, H., Lu, Z., Cao, Z., Han, J., and Yun, L. (2015). Hydrothermal Alteration of Ordovician Reservoir in Northeastern Slope of Tazhong Uplift, Tarim Basin. Acta Petrol. Sin. 37 (1), 43–63. (in Chinese with English Abstract). doi:10.7623/syxb201601004
Diao, H. Y. (2013). Rock Mechanical Properties and Brittleness Evaluation of Shale Reservoir. Acta Petrol. Sin. 29 (9), 3300–3306. doi:10.1086/671395
Ding, Q., Hu, X., Gao, Q., Ye, Y., and Zhang, P. (2019). The Hydrothermal Alteration Types and Zoning Features of Ordovician Carbonate in Tarim Basin. J. Zhejiang Univ. Sci. Edi. 46 (5), 600–609. (in Chinese with English Abstract). doi:10.3785/j.issn.1008-9497.2019.05.013
Gao, Y., Hu, T., Chen, G., Cheng, L., Yang, F., and Xiao, H. (2019). Porosity Evaluation Method for Clay-Altered Volcanic Rocks in Zhongguai Uplif. Well Logging Technol. 43 (1), 26–34. (in Chinese with English Abstract). doi:10.16489/j.issn.1004-1338.2019.01.005
He, S., Shi, Y., Xie, N., Zhang, Z., and Li, C. (2008). The Method for Acquirement of Conventional Logging Response-Based Lithomechanical Parameters. Xinjiang Petrol. Geol. 29 (5), 662–664. CNKI:SUN:XJSD.0.2008-05-048 (in Chinese with English Abstract).
Hou, M., Cao, H., Li, H., Chen, A., Wei, A., Chen, Y., et al. (2019). Characteristics and Controlling Factors of Deep Buried-Hill Reservoirs in the BZ19-6 Structural Belt, Bohai Sea Area. Nat. Gas Industry B 6 (1), 305–316. (in Chinese with English Abstract). doi:10.1016/j.ngib.2019.01.011
Jiang, T., Pan, X., Ren, M., Ren, M., Lei, J., and Dong, J. (2019). Analysis of Rock Alteration in A Hydropower Engineering Project, Southeastern China. Arab. J. Geosci. 12 (8), 1–12. doi:10.1007/s12517-019-4428-7
Li, J., Huang, H., and Wang, M. (2017). A Theoretical Derivation of the Dilatancy Equation for Brittle Rocks Based on Maxwell Model. Acta Geophys. 65 (1), 55–64. doi:10.1007/s11600-017-0006-0
Liu, K., Ma, Y., Zhang, Z., and Li, H. (2018). Evaluation Method for Fractured Reservoir in Buried Hill. Oil Drilling Prod. Technol 40, 58–61. (in Chinese with English Abstract). doi:10.13639/j.odpt.2018.S0.016
Liu, Z., Tang, X., Yu, H., and Zhang, B. (2009). Evaluation of Fracture Development in Volcanic Rocks Based on Rock Mechanical Parameters. Nat. Gas Ind. 29 (11), 20–21. (in Chinese with English Abstract). doi:10.3787/j.issn.1000-0976.2009.11.006
Nagano, Y., Lin, W., and Yamamoto, K. (2015). In-Situ Stress Analysis Using the Anelastic Strain Recovery (ASR) Method at the First Offshore Gas Production Test Site in the Eastern Nankai Trough, Japan. Mar. Pet. Geology. 66, 418–424. doi:10.1016/j.marpetgeo.2015.02.027
Nesbitt, H. W., and Young, G. M. (1982). Early Proterozoic Climates and Plate Motions Inferred from Major Element Chemistry of Lutites. Nature 299, 715–717. doi:10.1038/299715a0
Pandarinath, K. (2015). Testing of the Recently Developed Tectonomagmatic Discrimination Diagrams from Hydrothermally Altered Igneous Rocks of 7 Geothermal Fields. Turkish J. Earth Sci. 23 (4), 412–426. doi:10.3906/yer-1401-27
Shen, B., Mao, Z., Fan, H., and Wang, G. (2012). A New Porosity Calculation Method Based on Principal Component Analytical Technology for Altered Formation. Well Logging Technol. 36 (2), 130–134. (in Chinese with English Abstract). doi:10.3969/j.issn.1004-1338.2012.02.005
Shibuya, T., Kitajima, K., Komiya, T., Terabayashi, M., and Maruyama, S. (2007). Middle Archean Ocean Ridge Hydrothermal Metamorphism and Alteration Recorded in the Cleaverville Area, Pilbara Craton, Western Australia. J. Metamorph. Geol. 25 (7), 751–767. doi:10.1111/j.1525-1314.2007.00725.x
Simonen, A. (1953). Stratigraphy and Sedimentation of the Svecofennidic, Early Archean Supracrustal Rocks in Southwestern Finland. B. Geol. Soc. Finland 160, 1–64.
Smith, L. B., and Davies, G. R. (2006). Structurally Controlled Hydrothermal Alteration of Carbonate Reservoirs: Introduction. AAPG Bull. 90 (11), 1635–1640. doi:10.1306/intro901106
Tong, K., Zhao, C., Lv, Z., Zhang, Y., Zhen, H., Xu, S., et al. (2012). Reservoir Evaluation and Fracture Characterization of the Metamorphic Buried Hill Reservoir in Bohai Bay. Petrol. Explor. Dev. 39 (1), 56–63. CNKI:SUN:SKYK.0.2012-01-007 (in Chinese with English Abstract). doi:10.1016/s1876-3804(12)60015-9
Wang, J., Zhang, L., and Jin, X. (2013). Characteristics and Fluid Identification of Altered Volcanic Reservoir. J. Northeast. Petrol. Univ. 37 (3), 18–23. (in Chinese with English Abstract). doi:10.3969/j.issn.2095-4107.2013.03.003
Wang, M., Wang, Y., Tian, M., Fu, A., Zhu, J., Zhang, S., et al. (2018). Identification of Effective Reservoir and Quantitative Evaluation of Porosity of Altered Igneous Rock: A Case Study of Igneous Rock in the Upper Fourth Member of Shahejie Formation in Binnan Oilfield. Petrol. Geol. Reco. Effic. 25 (4), 26–31. (in Chinese with English Abstract). doi:10.13673/j.cnki.cn37-1359/te.2018.04.004
Wei, X., Gao, Y., Wei, Q., Sun, S., Yin, C., Liu, J., et al. (2017). Controlling of Alteration on Intermediate and Basic Volcanic Reservoirs: A Case Study of Lower Cretaceous Yingcheng Formation of Xujiaweizi Fault Depression, Songliao Basin. Glob. Geol. 36 (2), 541–551. (in Chinese with English Abstract). doi:10.3969/j.issn.1004-5589.2017.02.020
Xu, C., Du, X., Liu, X., Xu, W., and He, Y. (2020). Formation Mechanism of High-Quality Deep Buried-Hill Reservoir of Archaean Metamorphic Rocks and its Significance in Petroleum Exploration in Bohai Sea Area. Oil Gas Geol. 41 (2), 235–249. (in Chinese with English Abstract). doi:10.11743/ogg20200201
Xu, C., Yu, H., Wang, J., and Liu, X. (2019). Formation Conditions and Accumulation Characteristics of Bozhong 19-6 Large Condensate Gas Field in Offshore Bohai Bay Basin. Pet. Exploration Develop. 46 (1), 27–40. doi:10.1016/S1876-3804(19)30003-5
Xue, Y. A., Zhao, M., and Liu, X. (2021). Reservoir Characteristics and Controlling Factors of the Metamorphic Buried Hill of Bozhong Sag, Bohai Bay Basin. J. Earth Sci. 32 (4), 919–926. doi:10.1007/s12583-021-1415-1
Xue, Y., and Lei, H. (2018). Discovery and Geological Significance of Large Condensate Gas Field in Deep Archean Metamorphic Buried Hill in Bohai Sea. China Offshore Oil Gas 30 (3), 1–9. (in Chinese with English Abstract). doi:10.11935/j.issn.1673-1506.2018.03.001
Yin, S., Ding, W., Shan, Y., Zhou, W., Wang, R., Zhou, X., et al. (2016). A New Method for Assessing Young's Modulus and Poisson's Ratio in Tight Interbedded Clastic Reservoirs without A Shear Wave Time Difference. J. Nat. Gas Sci. Eng. 36, 267–279. doi:10.1016/j.jngse.2016.10.033
You, X., Jia, W., Xu, F., and Liu, Y. (2018). Mineralogical Characteristics of Ankerite and Mechanisms of Primary an Secondary Origins. Earth Sci. 43 (11), 246–255. doi:10.3799/dqkx.2018.152
Yuan, G., Cao, Y., Schulz, H.-M., Hao, F., Gluyas, J., Liu, K., et al. (2019). A Review of Feldspar Alteration and its Geological Significance in Sedimentary Basins: From Shallow Aquifers to Deep Hydrocarbon Reservoirs. Earth-Science Rev. 191, 114–140. doi:10.1016/j.earscirev.2019.02.004
Zhan, H., Dong, W., Chen, S., Hu, D., Zhou, H., and Luo, J. (2021). Improved Test Method for Convection Heat Transfer Characteristics of Carbonate Fractures after Acidizing Etching. Adv. Geo-energy Res. 5 (4), 376–385. doi:10.46690/ager.2021.04.04
Zhang, L., Pan, B., and Chan, G. (2012). Conduction Mechanism and Saturation Equation of Altered Igneous Reservoir. J. Jilin Univ. 42 (2), 429–435. (in Chinese with English Abstract). doi:10.13278/j.cnki.jjuese.2012.s2.027
Zhang, Y. Z. (2012). Rock Mechanics Parameters Calculation of the Fractured Rock Based on the Effective Media Theory with Well Logging. Amm 166-169, 2958–2961. doi:10.4028/www.scientific.net/amm.166-169.2958
Zhao, W., Sheng, A., Qiao, Z., Pan, L., Hu, P., and Zhang, J. (2018). Genetic Types and Distinguished Characteristics of Dolomite and the Origin of Dolomite Reservoirs. Petrol. Explor. Dev. 45 (6), 5–17. doi:10.1016/S1876-3804(18)30103-4
Zhao, Y., Wu, J., Ling, H., and Wang, H. (2016). Mineral Chemistry of Biotite and Chlorite in Western Part of Fu Cheng Granite, Southern Jiangxi Province: Implications for Uranium Mineralization. Min. Deposits. 35 (1), 153–168. (in Chinese with English Abstract). doi:10.16111/j.0258-7106.2016.01.010
Zhu, S., Zhu, X., Liu, X., Li, C., Wang, X., Tan, M., et al. (2016). Authigenic Minerals and Diagenetic Evolution in Altered Volcanic Materials and Their Impacts on Hydrocarbon Reservoirs: Evidence from the Lower Permian in the Northwestern Margin of Junggar Basin, China. Arab. J. Geosci. 9 (2), 276–285. doi:10.1007/s12517-015-2201-0
Zhu, X., Zhang, X., Fan, Y., and Deng, S. (2013). Research on the Effectiveness Analysis and Storage Evaluation of the Melaphyre Reservoir. Geophys. Geochem. Explor. 37 (3), 400–405. doi:10.11720/j.issn.1000-8918.2013.3.05
Keywords: metamorphic rock, igneous rock, hydrothermal alteration, well logging geology, petrophysics, reservoir characteristics
Citation: Zhu B, Pan R, Zhang C, Zhang Z and Shi W (2022) Application of Hydrothermal Altered Reservoir Identification Method in Metamorphic Rock Buried Hill of Bozhong sag, Bohai Bay Basin, China. Front. Earth Sci. 10:807659. doi: 10.3389/feart.2022.807659
Received: 02 November 2021; Accepted: 15 March 2022;
Published: 26 May 2022.
Edited by:
Shu Jiang, The University of Utah, United StatesReviewed by:
Yinhui Zuo, Chengdu University of Technology, ChinaRuyue Wang, SINOPEC Petroleum Exploration and Production Research Institute, China
Copyright © 2022 Zhu, Pan, Zhang, Zhang and Shi. This is an open-access article distributed under the terms of the Creative Commons Attribution License (CC BY). The use, distribution or reproduction in other forums is permitted, provided the original author(s) and the copyright owner(s) are credited and that the original publication in this journal is cited, in accordance with accepted academic practice. No use, distribution or reproduction is permitted which does not comply with these terms.
*Correspondence: Chaomo Zhang, emhhbmc3ODAxQDI2My5uZXQ=