- 1Chinese Academy of Geological Sciences, Beijing, China
- 2School of Earth Sciences and Engineering, Nanjing University, Nanjing, China
High-elevation and high-relief topography is the most prominent geomorphological features of the eastern margin of the Tibetan Plateau. This paper proposes that the interaction of the endogenic and exogenic forces jointly determines the formation of such high and steep landform. Eastward propagation of the Tibetan Plateau has been portioned by NW-striking, large-scaled sinistral strike-slip faults due to resistance of rigid Yangtze craton to the east of the eastern margin of the Tibetan Plateau. The transpressional stress has emerged in eastern margin of the Tibetan Plateau and has resulted in several large-scale active faults. The transpressional behavior has changed the flowing direction of the rivers from NW-SE to nearly N-S. The transport capacity of these southward-flowing rivers decreases correspondingly. Since the late Cenozoic, intensive seismic events have occurred on the active faults of the eastern Tibetan Plateau which resulted in geohazards such as slope failures, landslides along these southward-flowing rivers. This resulted in the formation of a large number of dammed lakes in the eastern margin of the Tibetan Plateau. To a certain degree, these dammed lakes play an important role in lowering the upstream erosion rate and in accelerating downstream river incision which yields gravity unloading and uplift of the bedrock. The frequently and widely distributed damming events, therefore, forms an important supplementary factor with respect to the formation of high and steep landforms.
Introduction
Rapid uplift around eastern margin of the Tibetan Plateau generated a high-elevation and high-relief topography in the formation of NE-trending mountain chains (Kirby et al., 2002), including Minshan Mountain, Longmenshan Mountain, and Gonggarshan Mountain. The elevation drops nearly 4,000 m from the Bayan Har block to the Yangtze craton over a distance of 30–50 km, which marks the geomorphological boundary in the East Tibetan Plateau (Liu-Zeng et al., 2008). However, there are still many controversies about the formation and preservation of such high and steep landforms.
Previous studies have proposed at least two typical models to explain the formation of high and steep landforms. One is the crustal shortening model. Hubbard and Shaw (2009) report that crustal shortening across the high and steep landscape varies from around 2–40%, which dramatically increases upon approaching and crossing Longmenshan Mountain. Tian et al., 2016 shows the similar evidence of late Cenozoic thrusting along major Longmenshan faults. However, a comparative analysis was conducted and the results showed that the crustal-shortening strain in eastern Tibetan Plateau is much lower than that in the northeastern Tibetan Plateau, where crustal shortening can reach up to 46% (Gao et al., 2013) and varies from 20% to >60% across the southern Qilian Shan–Nan Shan thrust belt and northern Qaidam Basin (Yin et al., 2008). In fact, the eastern margin of the Tibetan Plateau is much higher and steeper than northeastern Tibetan Plateau. Thus, The shortening of the crust is not the only determinant of the formation of high and steep landforms. Another model is the lower crustal flow model. The model proposes that lower crustal material flows around the strong crust of the Sichuan Basin and “piles up” the material above to create narrow and steep margins (Clark and Royden, 2000; Royden et al., 2008). However, recent seismological results indicate that lower crustal flow does not universally exist, with the deformation of Eastern Tibetan Plateau also being influenced by oblique thrusting of major NW-striking faults (Gao et al., 2013; Liu et al., 2015). In addition, some studies have assumed that climate-induced surface erosion is a predominant factor that determines the formation of high and steep landforms in eastern margin of the Tibetan Plateau (England and Molnar, 1990; Molnar and England, 1990). However, the premise of this assumption is that there should be a pre-existing high elevation difference in the terrain.
Therefore, while previous studies have provided us with possible causes, no single process offers a satisfactory interpretation to the formation and preservation of such terrain, which means the responsible dynamic mechanisms remain unclear.
Deep Earth Forces and Landform Changes
A Continental Transpressional Zone in Eastern Margin of the Tibetan Plateau
The formation of landforms comes from the interactions between deep Earth forces and surface processes (Cloetingh et al., 2013; Cloetingh and Willett, 2013). During the Indo-Asian collision, strong south-north shortening in the Tibetan Plateau occurred (Yin and Harrison, 2000; Tapponnier et al., 2001; Wang et al., 2002). However, slight shortening occurred on the western side of the Yangtze craton, which is adjacent to the eastern Tibetan Plateau. Such great differences in deformation will inevitably lead to a tectonic transition zone which will show special neotectonic characteristics between Tibetan Plateau and the Yangtze craton. Some tectonic processes show the formation of this transition zone.
On one hand, the eastern Tibetan Plateau is cut a series of relatively independent blocks by a series of NW-striking faults (Figure 1). GPS velocity data shows that material of the Tibetan Plateau is extruding from west to east (Shen et al., 2005; Gan et al., 2007). Due to the resistance of the stable Yangtze craton on the eastern side of the Tibetan Plateau, crustal material accumulated and created a broad and steep margin in eastern Tibetan Plateau (Clark and Royden, 2000). On the other hand, the eastward extrusion was approximately orthogonal to the convergence vector and extended along several major sinistral strike-slip fault zones on the eastern Tibetan Plateau (Tapponnier et al., 2001). The regional principal compressive stress is not perpendicular to the western boundary of the Yangtze craton, which promotes the formation of an oblique convergence between the two blocks. Under the common restriction of the two aspects mentioned above, there formed a comparatively restricted transperessional zone, showing a macroscale NE-trending. along eastern margin of the Tibetan Plateau (as seen in the white rectangle of Figure 2). In the transperessional zone, there are two large, arc-shaped fault systems named as the Xianshuihe-Xiaojiang fault zone and the East Kunlun-Minjiang/Huya fault zone. Both of them show N-NW striking trends in the interior of the plateau and then near NS-striking along eastern margin of the Tibetan Plateau (Figure 1).
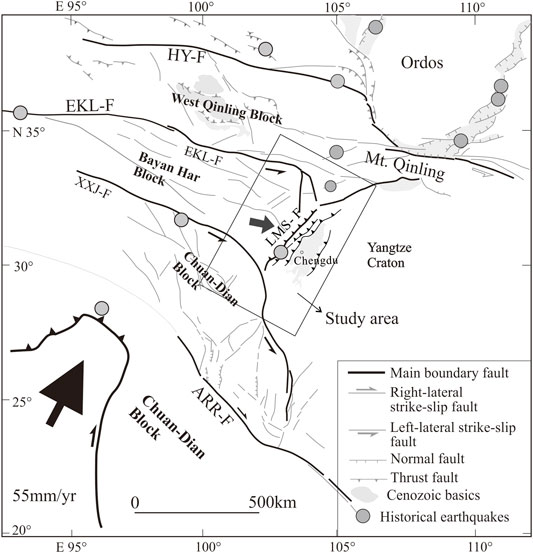
FIGURE 1. Regional tectonic map of the eastern Tibetan Plateau. EKL-F, east kunlun fault; HY-F, Haiyuan fault; QC-F, qingchuan fault; LMS-F, longmenshan fault; XXJ-F, Xianshuihe-Xiaojiang Fault; ARR-F, Ailao shan Red river fault.
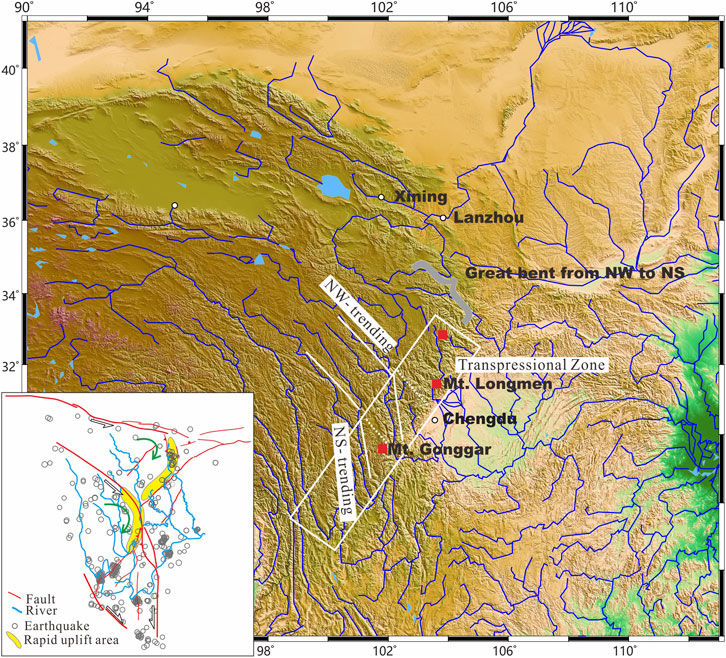
FIGURE 2. Characteristics of the continental transpressional zone in the eastern margin of the Tibetan Plateau. White dots denote the main cities in this region. The insert map in the lower-left corner shows the fault systems, river systems, and past earthquakes of eastern margin of the Tibetan Plateau.
The Great Bent Rivers in the Eastern Margin of the Tibetan Plateau
The morphological characteristics and evolutionary history of the fluvial systems are also controlled by surface uplift, especially in actively deforming regions (Clark et al., 2004). The upstream of the Yangtze River is composed of different branches. From east to west, they include the Tuojiang, Minjiang, Qingyijiang, Daduhe, Yalongjiang, and Jingshajiang Rivers. These rivers are neither perpendicular to the NE-trending mountain chain in the eastern margin of the Tibetan Plateau nor parallel to it. On the contrary, they generally show the characteristics of strong curve in their flow direction (Figure 2). In particular, the remarkable bend from a southeastward to near southwards flow direction geographically, Clark et al. (2004) calls it the superposition of eastward and southward draining rivers. The sudden changing of river flow from NW-flowing to nearly NS-flowing caused by fault zone in the east edge of the rivers exists in a universal way. Such as in Ya-lung River and in Jinsha River (Figure 2). This paper focuses more on the macro hydrographic distribution characteristics. However, such characteristics can’t always be observed in a river, such as in the upstream of Min-Jiang River. We prone to consider the feature of NW-to-NS is the primary form of antecedent stream as the latest study by Zhao et al. (2021). In the process of landform uplifting, the hydrographic system of original Tibet Plateau showed NW-to-NS flowing and endured spatial transformation lately (Zhao et al., 2021). These south-flowing rivers coincides with the active faults in the eastern Tibetan Plateau and affected by active fault (Li et al., 2010; Yan and Lin, 2015), showing similar features as those of the two large arc-shaped fault zone aforementioned (Figures 1, 2).
Assuming that the migration of the rivers would only be controlled generally by gravity, the flow direction of these rivers should be perpendicular to the NE trending gravity gradient zone, hence flowing NW, rather than a uniform macro bend from NW to near NS. The universal drainage pattern in eastern margin of the Tibetan Plateau reflects the general and macro-control of the transpressional stress during the Indo-Asian collision (Brookfield, 1998). A simple comparison shows that the length and tortuosity of these bent rivers may increase by 20–30% under the existence of tectonic forces. Since the length and tortuosity of a river defines the associated hydrodynamic forces, the slope, current speed, and transport capacity of these rivers decreased.
Active Fault and Landslide Dam
Landslide dam or dammed lake caused by earthquake are widely developed within intraplate orogenic belts, active belts, and active continental margins, such as the southern Himalayas (Hewitt, 1998; Srivastava et al., 2013; Draganits et al., 2014), Taiwan Island (Lin et al., 2008; Hsu and Hsu, 2009; Hsieh et al., 2012), the Cordillera orogenic belt (García-García et al., 2011), New Zealand (Adams, 1981; Korup, 2004), and the West Bank of Argentina (Bookhagen et al., 2001; Hermanns and Strecker, 1999; Trauth and Strecker, 1999). The eastern margin of the Tibetan Plateau belongs to the NS-trending Earthquake Belt in China, where many N-S striking active faults have developed (Deng et al., 2002; Deng et al., 2003). In some occasions, NW-striking high-slipping active faults overprint the N-S fault belts. Both of these have hosted many large to moderate earthquakes in the eastern margin of the Tibetan Plateau (Ma et al., 1989; Deng, 2008; Zhang, 2008; Zhang et al., 2009). For example, more than 10 strong earthquakes with magnitudes greater than 7 have occurred on the Xianshuihe- Xiaojiang fault over the past 150 years (Ma et al., 1989; Deng, 2008; Zhang, 2008; Zhang et al., 2009), which have caused a series of landslides and dammed lakes. On 1 June 1786, an earthquake of M 7.75 occurred in the Xianshuihe fault zone, forming a large, dammed lake in the Daduhe River, Sichuan Province, China. The subsequent failure of the dammed lakecaused great damage, with around 100,000 people lost (Dai et al., 2005; Korup and Tweed, 2007). The M 7.5 Diexi earthquake, 25 August 1933, also lead to a dammed lake, whereabout 45 days later, the landslide dam, with a height of around 160 m, collapsed due to aftershocks and rainstorms, causing thousands of casualties (Tang et al., 1983; Wang et al., 2005; Wang et al., 2007). Strong seismic events around Longmenshan Mountain brought about mass movements, such as slope failure, landslides, and damming events (Guo et al., 2016a). The Ms 7.9 Wenchuan earthquake provides us with a vivid example, in which approximately 400 million m3 of loose sediments were deposited during the earthquake, with dammed lakes diffused throughout the southward-flowing rivers (Huang and Fan, 2013; Guo et al., 2016b).
The formation of earthquake-induced landside dams is the most important component for the landform (Chai et al., 2000; Huang, 2007). The eastern margin of the Tibetan Plateau is one of the most seismically active areas in the world. In addition to the large elevation difference, this area is also have a high stress state (An et al., 2010) and a significant gravity imbalance within the deep crust. It is undoubtedly one of the steepest terrains in the world, with the most complex surface geometries of all orogenic belts. Such remarkable topographic gradients provide the most favorable locations for the occurrence of landslides, with about 80% of the large-scale landslides in China occurring around eastern margin of the Tibetan Plateau (Huang, 2007). In short, the strong interweaving and transformation of the endogenic and exogenic forces in this region promote the development of large landslides and dammed lakes (Huang, 2007).
Damming Preservation and Landform Evolution
Dammed lakes are usually sustained for a short time: about 27% break down within 1 day, 41% within 1 week, more than half within 10 days, 80% within half a year, and 93% within 1 year (Schuster and Costa, 1986). Those dammed lakes that survive for more than 100 years are termed steady-state dammed lakes (Nie et al., 2004). This is because the dam-body mainly consists of loose and unconsolidated rock and soils that are rapidly deposited and accumulated in the river. Thus, the dam may be damaged rapidly due to overflow, piping, seepage, or overtopping (Costa and Schuster, 1988; Casagli et al., 2003; Nie et al., 2004; Shi et al., 2010). However, at the upstream of the Yangtze River, there are still many dammed lakes that have survived for tens of thousands or even for millions of years. What impact they have on river evolution is therefore an important scientific issue worthy of attention. The following two examples are presented to clarify the overlooked impact of such features.
The Diexi Dammed Lake
The Diexi dammed lake developed in the upstream of the Minjiang River (Figures 4). The maximum thickness of the lacustrine strata is around 200 m. Field distribution characteristics of the lacustrine strata and remote sensing analysis show that the dammed lake is around 20 km in length (Li et al., 2010). Geochronological dating of the lake deposits show that it formed between around 30 to 74 ka. BP (Wang et al., 2005; Li et al., 2010; Wang et al., 2011a). In the interior of the dammed lake, the secondary dam-body formed by landslide can also be found (Figure 4C). On the northern side of the dam, the detachment or the slip plane can be observed in the bedrock (Figures 3, 4D). Meanwhile, the inclined bedrock is almost parallel to the slope, showing the form of typical bedding-controlled landslides. Interior of the dam show bedding of giant gravels which maintained the overall shape of the bedrock structure (Figure 4E). This suggests that these gravels come from a great landslide of bedrock from the east (Figure 4D), which was probably caused by a large earthquake. Lacustrine strata showing approximately horizontal and parallel bedding are deposited above these gravels (Figure 4F), which implies that the area was once submerged by the dammed lake.
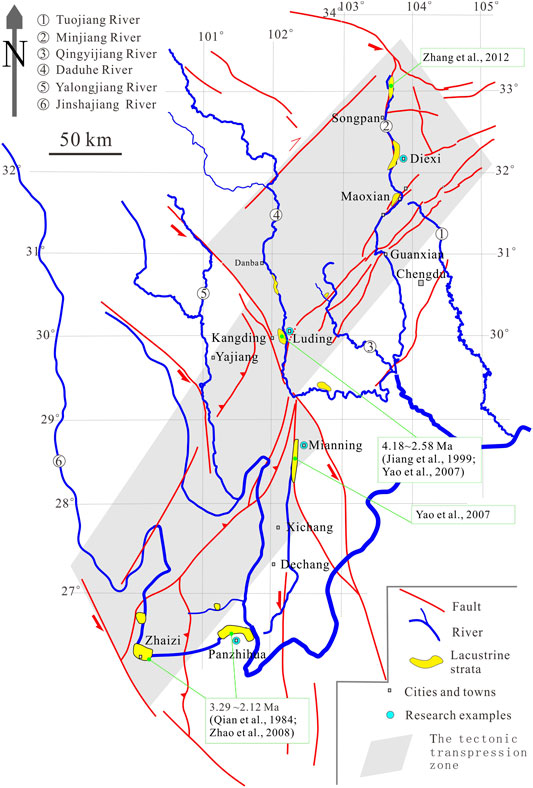
FIGURE 3. River system and its associated dammed lakes in eastern margin of the Tibetan Plateau (modified from Liu et al., 2015). The shaded part is the approximate range of the tectonic transpression zone.
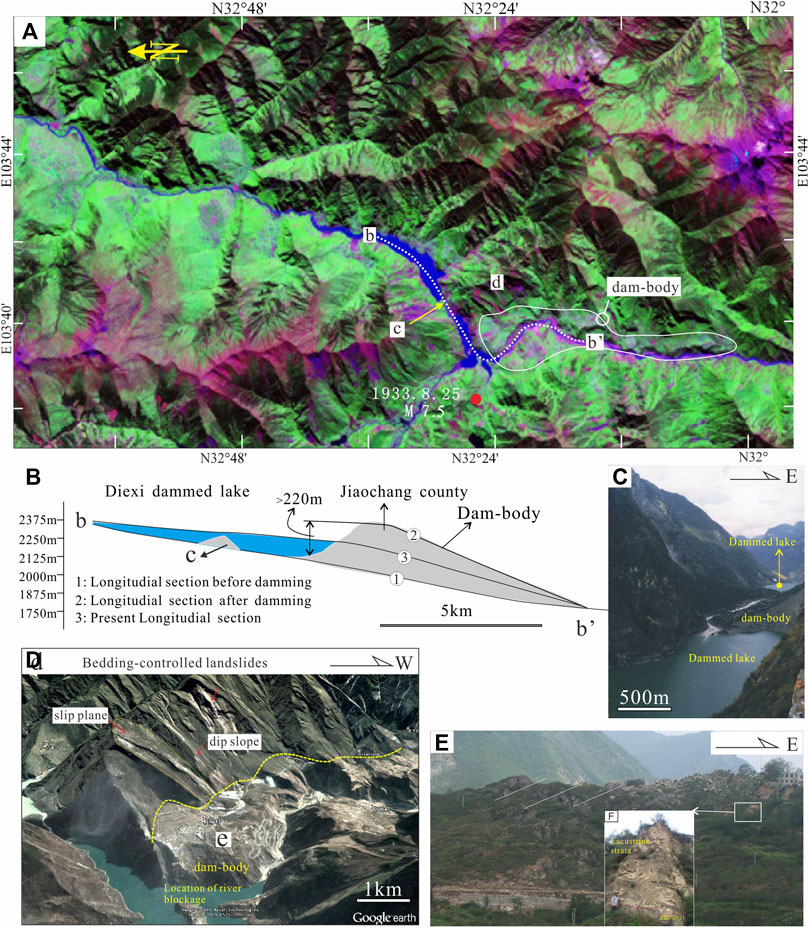
FIGURE 4. (A) Satellite image and sections of the Diexi dammed lake along the Minjiang River (modified from Li et al., 2010). (B) The conceptual longitudinal section across the Diexi dammed lake (modified from Li et al., 2014). (C) Field photos of the Diexi dammed lake. (D) Landslide surface on the north side of the dammed lake. (E,F) Examples of the huge gravel in the debris that consisting the dam.
When a dammed lake is full, it will form a waterfall at the facade and then incision occurs. In terms of the erosion rate, the average vertical incision rate on the downstream of the Diexi dammed lake is around 1810 m/Ma (Zhang et al., 2005). However, until now, the Minjiang River has not completely cut through the lacustrine strata. On the contrary the dammed lake is now being cut. This means that the incision rate of the upstream near the dammed lake is nearly zero after around 30 ka (if we regarded 30 ka as the formation time of the dam).
From the interior of these lacustrine strata, some evidences have been obtained to show the existence of paleo-earthquakes in the region, with each of the disturbance layers in the lacustrine strata representing a paleo-earthquake event that disturbed the unconsolidated strata (Wang et al., 2011b). It needs to be noted that the epicenters of these paleo-earthquakes may not just be in Diexi Country. In 1933, an Ms. 7.5 earthquake occurred and formed a new dammed lake superimposed on the ancient dammed lake (Ren et al., 2018). This makes the dammed lake bigger and the crest stronger. As a result, it will continue to affect the river’s geomorphology.
The Xigeda Dammed Lake
The Xigeda formation is a set of lacustrine strata with considerable thickness widely distributed in the upstream of the Yangtze River in eastern Tibetan Plateau. It is also considered to have formed as a dammed lake (Chen et al., 2004; Xu, 2011; Xu and Liu, 2011; Deng et al., 2021).
The Xigeda Dammed Lake in Dadu River
The age and thickness of the Xigeda formation vary across different reaches. For example, the Xigeda formation is 930 m higher than the current riverbed around the Dadu River which is a tributary of the upper reaches of the Yangtze River. The thickness of the Xigeda formation in this area is more than 400 m. Paleomagnetism studies show that the formation age is between 4.18 and 2.58 Ma (Jiang et al., 1999; Yao et al., 2007). It can be inferred from the data that the duration of the former dammed lake is up to 1.6 Ma. From the point of view of hydrodynamics, the upstream channel of a dammed lake is in an aggradation state during the life span of the lake. After around 2.58 Ma, when the dammed lake broke, the river began to down-cut. Here, this paper draws a conceptual model about the changes between river profile and river longitudinal profile after the formation of a dammed lake (Figure 5). Assuming that the incision rate was constant and that the unconsolidated lacustrine strata will be cut through a very short period, the minimum incision rate of the Dadu river should be 930 m/2.58 Ma = 360 /Ma, while the maximum incision rate should be equal to (930 m + 400 m)/2.58 Ma = 515 m/Ma. The maximum time the river needs to cut through the dam should be equal to 400 m/(515 m/Ma) = 0.78 Ma.
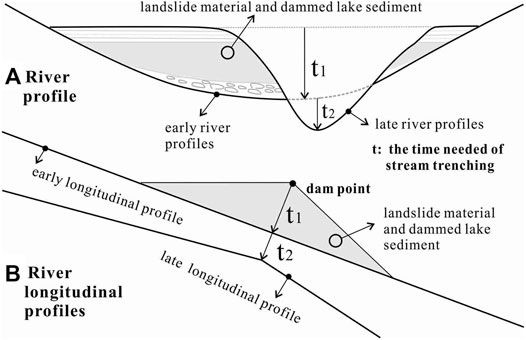
FIGURE 5. A conceptual model about the changes between river profile and river longitudinal profile after the formation of a dammed lake (Assuming that the unconsolidated lacustrine strata will be cut through a very short period, t1 should be a short time during the stage of dam-break).
Calculated in this way, it can be determined that the incision rate is between 360 m/Ma and 515 m/Ma after 2.58 Ma. It can also be estimated that a single-loop process of the dammed lake, from emerging to breaking down completely, takes a time which equal to (4.18–2.58 + 0.78) Ma = 2.38 Ma. Thus, the impact time of the lake is between ∼1.6 Ma to ∼2.38 Ma under conservative estimates.
The Xigeda Dammed Lake in the Jinshajiang River
Xigeda dammed lake near Panzhihua City is also found along the main stream of the Jinshajiang River, a branch of the Yangtze River. The maximum thickness of the Xigeda lacustrine strata here is around 300 m. Paleomagnetic studies show that the dammed lake formed between 3.29 Ma to 2.12 Ma (Qian et al., 1984). At present, the height difference between the riverbed and the bottom of the lacustrine layer is around 100 m (Zhao et al., 2008). The incision rate following the formation of the dammed lake is (300 m + 10 0 m)/2.12 Ma ≈ 188 m/Ma. It can be inferred that the maximum value of influence derived from the dammed lake on the river’s evolution can reach about (3.29–2.12) Ma+ 2.12 Ma* 300 m/(300 m + 100 m) = 2.76 Ma. The real impact time is therefore between around 1.07 Ma to 2.76 Ma.
The Xigeda Dammed Lake in the Others River
Since the formation of a dammed lake, there has been both erosion and sedimentation in the lake. We have also found Xigeda formationin Mianning City along the Anninghe River (Figure 3). The measurable thickness of the Xigeda formation is more than 300 m (Yao et al., 2007). However, the bottom of the lacustrine stratum is lower than current riverbed in several sites. This shows the Xigeda formation has not been exposed completely. Another example in the upper reaches of the Minjiang River, where a borehole has revealed that the thickness of the Xigeda lacustrine strata is more than 100 m in the current river channel (Zhang et al., 2012).
Recently, it has been reported that different age ranges have been obtained for the Xigeda formation, such as 1.34 to 1.58 Ma (Kong et al., 2009) and 200 to 700 ka (Wang et al., 2011a). Such a dispersed range of ages is, on the one hand, due to the limitations of current dating technology, especially for late Cenozoic sediments, while also reflecting the complexity of these lacustrine strata.
Similar to the Diexi dammed lake, these dammed-lake deposits for a multi-stage dam-break loop circulation process until it completely disappears. From the perspective of river evolution, Zhao et al. (2008) pointed out that the gravel layer at the bottom of the Xigeda formation was found close to the bottom of the Jinshajiang River. This indicates that the river had been down-cut to a depth that was close to the bottom of the current valley several million years ago, which requires the pre-existing channel was filled and downcutted again. While these rivers are still experiencing ongoing incision, over the long term, namely, from the emergence to the final disappearance of the dammed lake, the incision rate was close to zero. These data, therefore, provide us with a fact that the amount of incision in the eastern margin of the Tibet Plateau is quite small. However, many thermochronological studies suggest ∼10 Ma initiation of the last phase of rock uplift on the eastern margin of the Tibetan Plateau (much strongly uplifted since around 5 to 3 Ma), and shows the relatively deep exhumation of rocks along its eastern margin, by about 8–10 km (Kirby et al., 2002; Wang et al., 2012; Yang et al., 2020).
The strong uplift should have resulted in relatively rapid erosion or incision due to the river and deeply incise the bedrock in the high and steep eastern margin of the Tibetan Plateau. This is in contradiction with the previously introduced long-term preserved dammed lakes. To better understand this conundrum, a simple experiment is conducted. We present two terms: Unloading Capacity and Unloading Ratio. The former refers to the carrying capacity which can be measured by the amount of unloading of a river. The latter refers to the ratio between carrying capacity and loose sediment. A lot of loose sediments, rocks, debris, and landslides, which are derived from the strong earthquakes and weathering in the region, are accumulated and fill up these rivers’ branches. However, these rivers could not unload this added material immediately or quickly. After the Wenchuan earthquake, Parker et al. (2011) estimated that coseismic landslides produced amounts of erodible material which were greater than the net volume added to the orogen by coseismic rock uplift, even if only a fraction of landslide debris is removed from the orogen over the earthquake recurrence period. Although the unloading amount is very large in the eastern Tibetan Plateau, the unloading ratio is not high enough. This indicates that frequent damming behavior and strong unloading can cause river accretion, which can counteract a river’s incision because of the rock uplift. Beyond our intuitive knowledge, the unloading ratios of these rivers were not as strong as expected. To a certain degree, eastern margin of the Tibetan Plateau plays a role as a large-scaled damming or blocking system, over the last around 4 Ma.
Landscape Deformation and Energy Conversion
To illustrate the energy conversion process of the dammed lakes, we should trace back to the beginning when a dammed lake formed. The formation of a dammed lake is accomplished within a short time. Earthquakes are one form of the internal energy released by the deep Earth. Driven or triggered by seismic energy, landslides, rock collapses, and debris flows are other forms of potential energy release. After the formation of the dammed lake, the river water level rises and the potential energy increases. Hence, the formation and destruction of earthquake-induced dammed lakes reflect instantaneous energy transformation in nature.
In the process of river evolution, the formation of knickpoints could raise the erosion basis and slow down the erosion rate of the upstream channel. Earthquakes can create dammed lakes and then form a knickpoint in the long profile whenever a damming event occurs on a river, which caused the ups and downs of the river in the long profile and can reduce the kinetic energy of rivers (Figure 5). This phenomenon is similar to the “step-pool system” (Figure 5) (Xu and Wang, 2004; Wang et al., 2006; Zhang et al., 2011). As shown in Figure 5, the energy dissipation ratio k of the reach ABC is given by:
where
The Diexi dammed lake, with a height of ∼200 m (Figure 4) and an impact time of >40 to 70 ka, will continue to affect the river landforms for a long time. The height of the dammed lake in Jinsha River is ∼300 m, and the duration of its impaction is greater than 1.16 Ma. The Xigeda dammed lake on the Dadu River, with a height of around 400 m, has an impact time of more than 1.6 Ma. During these periods, it can be considered that the river cutting rate is near zero for the upstream areas of a dammed lake, at least within the range of tens or even near a hundred kilometer from it. Macroscopically speaking, the cutting rate during these periods will also be very low. From the point of view of river incision ratios, during this period of around 4 to 3 Ma, there must be a sharp decrease or a gap in the average incision rate. This had been discovered by the research on the Dadu River (Ouimet et al., 2007; Ma et al., 2020). These examples also reflect the basic fact that once a large-scale dammed lake has formed, it will play a continuous role in the evolution of river geomorphology over long timescales. It is therefore definitely the case that damming behaviors associated with frequent earthquake events are closely related to processes of mountain building.
Discussion
How should we understand the formation of high and steep landform in the eastern margin of the Tibetan Plateau? We thought that the process was similar to building a skyscraper, adding materials bit by bit. From this point of view, the primary reason for the formation of high and steep landforms is the accumulation of materials within the body and to reduce material erosion on its surface. There are four ways for material accumulation.
The first way is the overlapping of crustal materials from horizontal contraction deformation, such as the thrust caused by the extrusion of the eastern Tibetan Plateau. The second way is the upwelling of deep materials in the vertical direction. The third way is the weakening of erosion in terms of external dynamics, and the fourth way is the strengthening of the aforementioned three ways by the coupling of internal and external dynamics. These aspects will now be discussed.
First, compression deformation is always the essential cause of geomorphic uplift, because it is the most effective means of material accumulation, not only in the eastern margin of the Tibetan Plateau but in all orogenic belts around the world. The structural pattern is an important factor in the process of material accumulation. Compression stress in the eastern Tibetan Plateau has shown the characteristics of transpressional deformation since the middle to late Cenozoic. As a result, the convergence deformation in the upper crust has seen dramatic shortening, for example, up to 40% for Longmenshan Mountain (Hubbard and Shaw, 2009) while also resulting in the characteristics of the strike-slip and oblique thrust of the Longmenshan fault zone. Li et al. (2008) showed that oblique thrust is the most effective way of controlling the rapid rise of the mountains through a large number of case studies, such as the Altun fault, the East Kunlun fault, and the Longmenshan fault.
Secondly, deep material upwelling also plays a role in material accumulation. Our previous findings have shown that the youngest magma (14.4–3.6 Ma) in the Tibetan Plateau is emplaced under Mt. Gonggar (unpublished data), where unroofing is dated from 11 to 1 Ma by low-temperature thermochronological dating (Xu and Kamp, 2000; Lai et al., 2007). We are inclined to believe that transpressional behavior plays a vital role in the ascent and emplacement of magma (or deep-earth materials) and provides the space occupied by the up-welling of the youngest magma. This space is eventually transformed by the uplift of the landscape, such as lower crustal flow (Figures 6, 7).
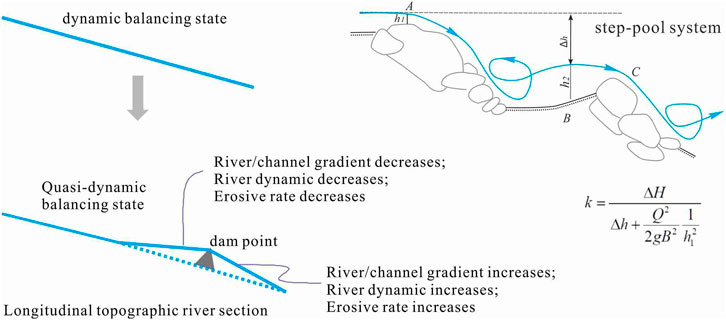
FIGURE 6. Energy dissipation in a dammed river. Energy dissipation k = ΔH/(Δh + Q2/2gB2h12), where Δh is the difference between two surface elevations from point A to point C, Q is the water flow, B is the width of the river, and h2 is the depth of the water (Xu and Wang, 2004).
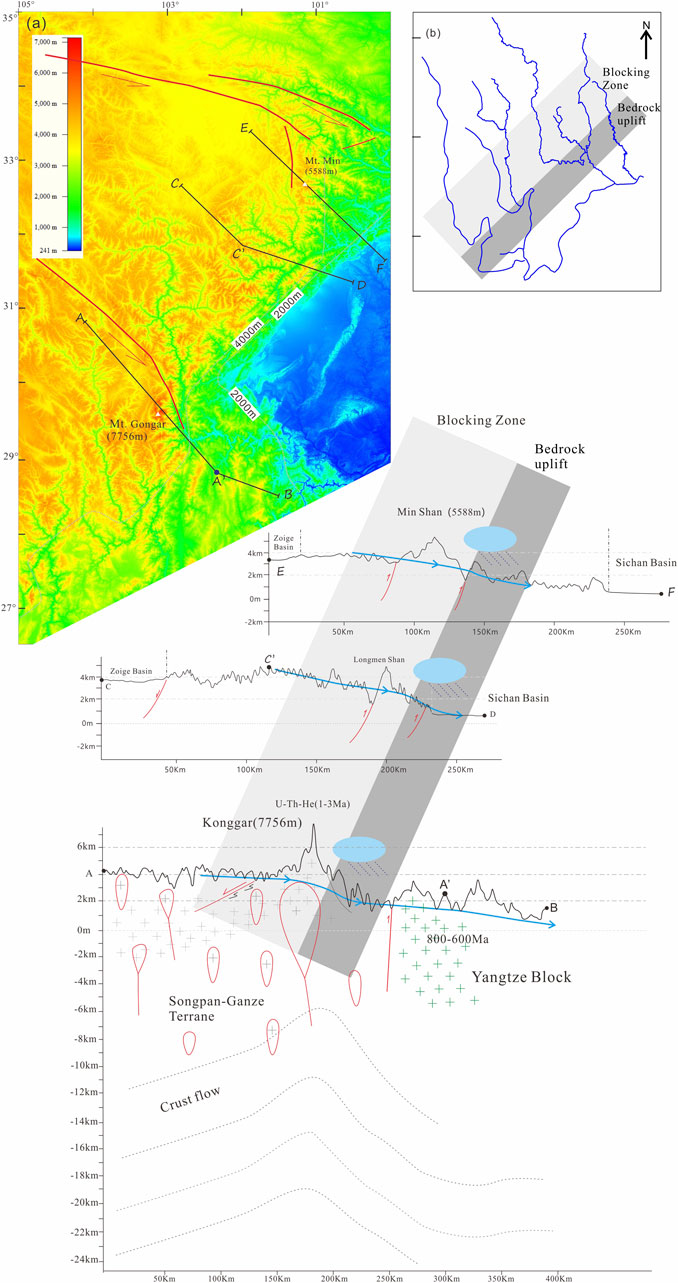
FIGURE 7. Landform analysis of the eastern Tibetan Plateau (The map summarizes the causes of the high and steep landform on the East Tibet. The approximate spatial position of the blocking area is marked in the map. From the perspective of exogenic forces, heavy rainfall frequently occurs in the piedmont of the eastern margin of Tibet Plateau, which speeds up the denudation rate and makes an obvious contribution to high and steep landform. From the perspective of endogenic forces, three topographical sections are provided here. Profile A-B passes through the Gonggar mountain. Scholars believe that there is lower crustal flow in its deep part (Clark and Royden, 2000; Zhang 2008; Liu et al., 2015)., which is the influence of deep structure on landform. Profile C-D passes through Longmenshan mountain, and the compressive uplift of thrust fault zone is the main controlling factor of geomorphic uplift (Tian et al., 2016; Gao et al., 2013). Profile E-F passes through the Minshan mountain, one of the highest peaks in the northeast margin of Tibet Plateau, and is controlled by two boundary faults (the Minjiang and Huya fault). The data of this figure comes from the following websites: http://dds.cr.usgs.gov/srtm/and http://glcf.umiacs.umd.edu/data.
In this paper, we noted that the highest mountain peaks, for example, Mt. Gonggar (7,756 m) and Mt. Min (5,588 m), as well as the fastest-growing places in eastern margin of the Tibetan Plateau, are all located at the bend of the two big arc-shaped faults aforementioned (Figure 7). Also, analog experiments (Mazzarini et al., 2010) and a large number of field examples indicate that strike–slip deformation is favorable for magma emplacement (Cao and Neubauer, 2016).
Thirdly, there can be no doubt that the formation of high and steep landforms is the result of the influence of internal and external dynamics. Molnar and England (1990) first noted the frequent rainfall around Longmenshan Mountain. Under the action of gravity equilibrium, the crustal material rebounds and causes the uplift of the landscape (Molnar and England, 1990; Zhang et al., 1994). In subsequent research, Korup and Montgomery (2008) showed that moraine-dammed lakes not only inhibit bedrock incision during their lifetime but also promote the upstream aggradation and burial of bedrock valley floors under glaciofluvial sediment long after the dam’s formation. Reviewing these mechanisms (Korup and Montgomery, 2008) and combining them with the results of this paper, we conclude several key points that can help us to understand the high and steep landforms in the eastern margin of the Tibetan Plateau.
1) When a dammed lake is formed, it can be considered that the river changes from a dynamic balancing state to a quasi-dynamic balancing state (Figure 6). Taking the dammed lake as a knickpoint, the trunk can be divided into upstream and downstream sections where the hydrodynamic processes change between the ups and downs correspondingly. This is closely related to the erosional or incisional processes and represents the mechanism of the step-pool system (Figure 6).
2) In longitudinal sections, there should be many big dammed lakes in a river system. Therefore, the decrease in hydrodynamic forces has a cumulative effect.
3) With regards to the evolution of landforms, the impact of one single damming event is insignificant, yet, frequent and continuous damming events can result in the sustained effect of external forces which can be observed in the eastern margin of the Tibetan Plateau, where frequent seismic events occur.
4) The long-term retention of loose materials can protect the riverbed and lead to weakening denudation. However, this is an extremely complex proposition. We have presented in-depth discussions on this issue (Li et al., 2010; Li and Zhang, 2015), and the conclusion can be summarized into two points, namely that the transpressional structures increase the bending of these rivers and that deep valleys result from rapid crustal uplift.
Finally, comparing the earthquake-induced damming behavior with that resulting from glacial dam in the Yarlung River (Korup and Tweed, 2007; Korup and Montgomery, 2008; Owen, 2008), this paper emphasizes that the effect of these events are similar to river morphology and the hydrodynamic environment. Meanwhile, this paper describes how transpressional stress causes overlapping in crustal materials, as well as spatial adjustment and provides a channel or space for the upwelling of material from the deep Earth, both of which eventually transform into landform uplift. Transpressional behavior therefore strongly affects and bridges surface and deep geological processes. Hence, it provides a new insight into a logical link between the endogenetic and exogenetic forces on the Earth’s surface, which results in the high elevation and steep relief in the eastern margin of the Tibetan Plateau.
Conclusion
1) Geological coupling between endogenetic and exogenetic forces has resulted in the formation and maintenance of the high-elevation and high-relief topography of eastern margin of the Tibetan Plateau.
2) The continental transpressional stress between the Tibetan Plateau and the Yangtze block, showing contraction deformation and oblique thrusting, strongly affects and bridges surface and deep geological processes.
3) Frequent seismic activity has resulted in widespread large-scale damming events, offering an important insight into the evolution of landscape.
Data Availability Statement
The original contributions presented in the study are included in the article/Supplementary Material, further inquiries can be directed to the corresponding authors.
Author Contributions
All authors listed have made a substantial, direct, and intellectual contribution to the work and approved it for publication.
Funding
This study was jointly supported by the National Natural Science Foundation of China (grant number 41872223, 41874114), and the Fundamental Research Funds for the Chinese Academy of Geological Sciences.
Conflict of Interest
The authors declare that the research was conducted in the absence of any commercial or financial relationships that could be construed as a potential conflict of interest.
Publisher’s Note
All claims expressed in this article are solely those of the authors and do not necessarily represent those of their affiliated organizations, or those of the publisher, the editors and the reviewers. Any product that may be evaluated in this article, or claim that may be made by its manufacturer, is not guaranteed or endorsed by the publisher.
Acknowledgments
We thank the Editors and the reviewers for their constructive reviews and encouragement. We hereby to express our sincere gratitude toward Dr. Hongdan Deng, professor Yue Zhao and professor Yuntao Tian.
References
Adams, J. (1981). Earthquake-dammed Lakes in New Zealand. Geol 9 (5), 215–219. doi:10.1130/0091-7613(1981)9<215:elinz>2.0.co;2
An, M., Feng, M., and Long, C. (2010). Deep Ruptures Around the Hypocenter of the 12 May 2008 Wenchuan Earthquake Deduced from Aftershock Observations. Tectonophysics 491 (1–4), 96–104. doi:10.1016/j.tecto.2009.12.024
Bookhagen, B., Haselton, K., and Trauth, M. H. (2001). Hydrological Modelling of a Pleistocene Landslide-Dammed lake in the Santa Maria Basin, NW Argentina. Palaeogeogr. Palaeoclimatol. Palaeoecol. 169 (1), 113–127. doi:10.1016/s0031-0182(01)00221-8
Brookfield, M. E. (1998). The Evolution of the great river Systems of Southern Asia during the Cenozoic India-Asia Collision: Rivers Draining Southwards. Geomorphology 22 (3), 285–312. doi:10.1016/s0169-555x(97)00082-2
Cao, S., and Neubauer, F. (2016). Deep Crustal Expressions of Exhumed Strike-Slip Fault Systems: Shear Zone Initiation on Rheological Boundaries. Earth-Science Rev. 162, 155–176. doi:10.1016/j.earscirev.2016.09.010
Casagli, N., Ermini, L., and Rosati, G. (2003). Determining Grain Size Distribution of the Material Composing Landslide Dams in the Northern Apennines: Sampling and Processing Methods. Eng. Gelogy 69 (1), 83–97. doi:10.1016/s0013-7952(02)00249-1
Chai, H., Liu, H., and Zhang, Z. (2000). The Temporal-Soatial Distribution of Damming Landslides in China. J. Mountain Sci. 18 (Z1), 51–54. (In Chinese with English Abstract). doi:10.16089/j.cnki.1008-2786.2000.s1.011
Chen, Z., Sun, Z., Royden, L. H., and Zhang, X. (2004). Landslide Blocked lake: Origin of the Xigeda Formation in Luding, Sichuan and its Significance. Quat. Res. 24 (6), 614–620. (In Chinese with English Abstract). doi:10.3901/cjme.2004.04.614
Clark, M. K., Schoenbohm, L. M., Royden, L. H., Whipple, K. X., Burchfiel, B. C., Zhang, X., et al. (2004). Surface Uplift, Tectonics, and Erosion of Eastern Tibet from Large-Scale Drainage Patterns. Tectonics 23 (1), TC1006. doi:10.1029/2002tc001402
Clark, M. K., and Handy Royden, L. (2000). Topographic Ooze: Building the Eastern Margin of Tibet by Lower Crustal Flow. Geology 28 (8), 703–706. doi:10.1130/0091-7613(2000)028<0703:tobtem>2.3.co;2
Cloetingh, S., Burov, E., and Francois, T. (2013). Thermo-mechanical Controls on Intra-plate Deformation and the Role of Plume-Folding Interactions in continental Topography. Gondwana Res. 24 (3-4), 815–837. doi:10.1016/j.gr.2012.11.012
Cloetingh, S., and Willett, S. D. (2013). TOPO-EUROPE: Understanding of the Coupling between the Deep Earth and continental Topography. Tectonophysics 602, 1–14. doi:10.1016/j.tecto.2013.05.023
Costa, J. E., and Schuster, R. L. (1988). The Formation and Failure of Natural Dams. GSA Bull. 100 (7), 1054–1068. doi:10.1130/0016-7606(1988)100<1054:tfafon>2.3.co;2
Dai, F., Lee, C., Deng, J., and Tham, L. (2005). The 1786 Earthquake-Triggered Landslide Dam and Subsequent Dam-Break Flood on the Dadu River, Southwestern China. Geomorphology 73 (3), 277–278. doi:10.1016/j.geomorph.2004.08.011
Deng, Q. (2008). Sone Thoughts on the Ms 8.0 Wenchuan, Earthquake. Seismology Geology. 30 (4), 811–827. (In Chinese with English Abstract).
Deng, Q., Zhang, P. Z., Ran, Y., Yang, X., Min, W., and Chu, Q. (2002). Basic Characteristics of Active Tectonics of China. Sci. China (Series D) 32 (12), 1020–1030. (In Chinese). doi:10.1360/zd2002-32-12-1020
Deng, Q., Zhang, P., Ran, Y., Yang, X., Min, W., and Chen, L. (2003). Active Tectonics and Earthquake Activities in china. Earth Sci. Front. 10 (z1), 66–73. (In Chinese with English Abstract). doi:10.3321/j.issn:1005-2321.2003.z1.012
Deng, B., Chew, D., Mark, C., Liu, S., and Li, J. (2021). Late Cenozoic Drainage Reorganization of the Paleo-Yangtze River Constrained by Multi-Proxy Provenance Analysis of the Paleo-lake Xigeda. Geol. Soc. America Bull. 133 (1-2), 199–211. doi:10.1130/b35579.1
Draganits, E., Grasemann, B., Janda, C., Hager, C., and Preh, A. (2014). 300 MW Baspa II - India's Largest Private Hydroelectric Facility on Top of a Rock Avalanche-Dammed Palaeo-lake (NW Himalaya): Regional Geology, Tectonic Setting and Seismicity. Eng. Geology. 169, 14–29. doi:10.1016/j.enggeo.2013.11.009
England, P., and Molnar, P. (1990). Right-lateral Shear and Rotation as the Explanation for Strike-Slip Faulting in Eastern Tibet. Nature 344 (6262), 140–142. doi:10.1038/344140a0
Gan, W., Zhang, P., Shen, Z. K., Niu, Z. J., Wang, M., Wan, Y. G., et al. (2007). Present-day Crustal Motion within the Tibetan Plateau Inferred from GPS Measurements. J. Geophys. Res. 112 (B8), 582–596. doi:10.1029/2005jb004120
Gao, R., Wang, H., Yin, A., Dong, S., Kuang, Z., Zuza, A. V., et al. (2013). Tectonic Development of the Northeastern Tibetan Plateau as Constrained by High-Resolution Deep Seismic-Reflection Data. Lithosphere 5 (6), 555–574. doi:10.1130/l293.1
García-García, F., Sánchez-Gómez, M., Navarro, V., and Pla, S. (2011). Formation, Infill, and Dissection of a Latest-Pleistocene Landslide-Dammed Reservoir (Betic Cordillera, Southern Spain): Upstream and Downstream Geomorphological and Sedimentological Evidence. Quat. Int. 233 (1), 61–71. doi:10.1016/j.quaint.2010.07.010
Guo, X., Cui, P., Li, Y., Zou, Q., and Kong, Y. (2016a). The Formation and Development of Debris Flows in Large Watersheds after the 2008 Wenchuan Earthquake. Landslides 13 (1), 116–125. doi:10.1007/s10346-014-0541-6
Guo, X., Cui, P., Li, Y., Ma, L., Ge, Y., and Mahoney, W. B. (2016b). Intensity-duration Threshold of Rainfall-Triggered Debris Flows in the Wenchuan Earthquake Affected Area, China. Geomorphology 253, 208–216. doi:10.1016/j.geomorph.2015.10.009
Hermanns, R. L., and Strecker, M. R. (1999). Structural and Lithological Controls on Large Quaternary Rock Avalanches (Sturzstroms) in Arid Northwestern Argentina. GSA Bull. 111 (6), 934–948. doi:10.1130/0016-7606(1999)111<0934:salcol>2.3.co;2
Hewitt, K. (1998). Catastrophic Landslides and Their Effects on the Upper Indus Streams, Karakoram Himalaya, Northern Pakistan. Geomorphology 26 (1), 47–80. doi:10.1016/s0169-555x(98)00051-8
Hsieh, M.-L., Lai, L. S.-H., Lin, C. D.-J., and Shyu, J. B. H. (2012). Late Quaternary Landscape Evolution and Genesis of the 2009 Catastrophic Landslide in the Hsiao-Lin Area, Southwestern Taiwan. Geomorphology 179, 225–239. doi:10.1016/j.geomorph.2012.08.014
Hsu, Y., and Hsu, Y. (2009). Impact of Earthquake-Induced Dammed Lakes on Channel Evolution and Bed Mobility. Case Study of the Tsaoling Landslide Dammed lake. J. Hydrol. 374 (1), 43–55. doi:10.1016/j.jhydrol.2009.05.020
Huang, R., and Fan, X. (2013). The Landslide story. Nat. Geosci 6 (5), 325–326. doi:10.1038/ngeo1806
Huang, R. (2007). Large-scale Landslides and Their Sliding Mechanisms in China since the 20th century. Chin. J. rock Mech. Eng. 26 (3), 433–454. doi:10.3321/j.issn:1000-6915.2007.03.001
Hubbard, J., and Shaw, J. H. (2009). Uplift of the Longmen Shan and Tibetan Plateau, and the 2008 Wenchuan (M = 7.9) Earthquake. Nature 458 (7235), 194–197. doi:10.1038/nature07837
Jiang, F., Wu, X., Xiao, H., and Zhao, Z. (1999). On the Age of the Xigeda Formation in Luding, Sichuan and its Neotectonic Significance. Acta Geol. Sinica 73 (1), 1–6. (In Chinese with English Abstract).
Kirby, E., Reiners, P. W., Krol, M. A., Whipple, K. X., Hodges, K. V., Farley, K. A., et al. (2002). Late Cenozoic Evolution of the Eastern Margin of the Tibetan Plateau: Inferences from 40Ar/39Ar and (U-Th)/He Thermochronology. Tectonics 21 (1), 1001. doi:10.1029/2000tc001246
Kong, P., Granger, D. E., Wu, F., Caffee, M. W., Wang, Y., Zhao, X., et al. (2009). Cosmogenic Nuclide Burial Ages and Provenance of the Xigeda paleo-lake: Implications for Evolution of the Middle Yangtze River. Earth Planet. Sci. Lett. 278 (1–2), 131–141. doi:10.1016/j.epsl.2008.12.003
Korup, O. (2004). Geomorphometric Characteristics of New Zealand Landslide Dams. Eng. Geology. 73 (1), 13–35. doi:10.1016/j.enggeo.2003.11.003
Korup, O., and Montgomery, D. R. (2008). Tibetan Plateau River Incision Inhibited by Glacial Stabilization of the Tsangpo Gorge. Nature 455 (7214), 786–789. doi:10.1038/nature07322
Korup, O., and Tweed, F. (2007). Ice, Moraine, and Landslide Dams in Mountainous Terrain. Quat. Sci. Rev. 26 (25), 3406–3422. doi:10.1016/j.quascirev.2007.10.012
Lai, Q. Z., Ding, L., Wang, H. W., Yue, Y. H., and Cai, F. L. (2007). Constraining the Stepwise Migration of the Eastern Tibetan Plateau Margin by Apatite Fission Track Thermochronology. Sci. China Ser. D 36 (9), 785–796. doi:10.1007/s11430-007-2048-7
Li, H., and Zhang, Y. (2015). Analysis of the Controlling Factors of Landslide Damming and Dam Failure: an Analysis Based on Literature Review and the Study on the Meridional River System of Eastern Tibetan Plateau. Quat. Sci. 35 (1), 71–87. (In Chinese with English Abstract). doi:10.11928/j.issn.1001-7410.2015.01.07
Li, H., Fu, X., Jvan Der Woerd, R. O. M., Si, J., Wang, Z., Hou, L., et al. (2008). Co-seisimic Surface Rupture and Dextral-Slip Oblique Thrusting of the MS 8.0 Wenchuan Earthquake. Acta Geologica Sinica 82 (12), 1623–1643. (In Chinese with English Abstract). doi:10.3321/j.issn:0001-5717.2008.12.002
Li, H., Zhang, Y., and Li, J. (2010). Meridional River Systems and Ancient Dammed Lakes on the East Margin of the Tibetan Plateau. Quat. Sci. 30 (4), 812–824. (In Chinese with English Abstract). doi:10.3969/j.issn.1001-7410.2010.04.16
Lin, W., Lin, C., Tsai, J., and Huang, P. (2008). Eco-environmental Changes Assessment at the Chiufenershan Landslide Area Caused by Catastrophic Earthquake in Central Taiwan. Ecol. Eng. 33 (3), 220–232. doi:10.1016/j.ecoleng.2008.04.002
Liu, Q. Y., Hilst, R. D. V. D., Li, Y., Yao, H. J., Chen, J. H., Guo, B., et al. (2015). Eastward Expansion of the Tibetan Plateau by Crustal Flow and Strain Partitioning across Faults. Nat. Geosci. 7 (5), 361–365. doi:10.1038/ngeo2130
Liu-Zeng, J., Tapponnier, P., Gaudemer, Y., and Ding, L. (2008). Quantifying Landscape Differences across the Tibetan Plateau: Implications for Topographic Relief Evolution. J. Geophys. Res. 113, F04018. doi:10.1029/2007JF000897
Ma, J., Ma, S. L., and Lei, X. L. (1989). Fault Geometry and its Relationship with Seismicity in the Xianshuihe Fault Zone. Phys. Chem. Earth 17, 131–142. doi:10.1016/0079-1946(89)90018-9
Ma, Z., Zhang, H., Wang, Y., Tao, Y., and Li, X. (2020). Inversion of Dadu River Bedrock Channels for the Late Cenozoic Uplift History of the Eastern Tibetan Plateau. Geophys. Res. Lett. 47 (4), e2019GL086882. doi:10.1029/2019gl086882
Mazzarini, F., Musumeci, G., Montanari, D., and Corti, G. (2010). Relations between Deformation and Upper Crustal Magma Emplacement in Laboratory Physical Models. Tectonophysics 484 (1-4), 139–146. doi:10.1016/j.tecto.2009.09.013
Molnar, P., and England, P. (1990). Late Cenozoic Uplift of Mountain Ranges and Global Climate Change: Chicken or Egg. Nature 346 (6279), 29–34. doi:10.1038/346029a0
Nie, G., Gao, J., and Deng, Y. (2004). Preliminary Study on Earthquake-Induced Dammed lake. Quat. Sci. 24 (3), 293–301. (In Chinese with English Abstract). doi:10.3321/j.issn:1001-7410.2004.03.008
Ouimet, W. B., Whipple, K. X., Royden, L. H., Sun, Z., and Chen, Z. (2007). The Influence of Large Landslides on River Incision in a Transient Landscape: Eastern Margin of the Tibetan Plateau (Sichuan, China). Geol. Soc. America Bull. 119, 1462–1476. doi:10.1130/b26136.1
Parker, R. N., Densmore, A. L., Rosser, N. J., de Michele, M., Li, Y., Huang, R., et al. (2011). Mass Wasting Triggered by the 2008 Wenchuan Earthquake Is Greater Than Orogenic Growth. Nat. Geosci 4 (7), 449–452. doi:10.1038/ngeo1154
Qian, F., Xu, S., Chen, F., and Zhao, Y. (1984). Study on the Paleomagnetion of the Xigeda Formation. Mountain Res. 2 (4), 275–282. (In Chinese with English Abstract). doi:10.16089/j.cnki.1008-2786.1984.04.012
Ren, J., Xu, X., Zhang, S., Yeats, R. S., Chen, J., Zhu, A., et al. (2018). Surface Rupture of the 1933 M 7.5 Diexi Earthquake in Eastern Tibet: Implications for Seismogenic Tectonics. Geophys. J. Int. 212 (3), 1627–1644. doi:10.1093/gji/ggx498
Royden, L. H., Burchfiel, B. C., and van der Hilst, R. D. (2008). The Geological Evolution of the Tibetan Plateau. Science 321 (5892), 1054–1058. doi:10.1126/science.1155371
Schuster, R. L., and Costa, J. E., 1986. Effects of Landslide Damming on Hydroelectric Projects, in Proceedings 5th International Association of Engineering Geology Congress, 1295–1307.
Shen, Z., Lü, J., Wang, M., and Bürgmann, R. (2005). Contemporary Crustal Deformation Around the Southeast Borderland of the Tibetan Plateau. J. Geophys. Res. 110, B11. doi:10.1029/2004jb003421
Shi, Z., Li, J., Lu, C., Wang, Y., and Xu, Q. (2010). Research Status and prospect of the Stability of Landslide Dam. J. Eng. Geology. 18 (5), 657–663. (In Chinese with English Abstract).
Srivastava, P., Ray, Y., Phartiyal, B., and Sharma, A. (2013). Late Pleistocene-Holocene Morphosedimentary Architecture, Spiti River, Arid Higher Himalaya. Int. J. Earth Sci. (Geol Rundsch) 102 (7), 1967–1984. doi:10.1007/s00531-013-0871-y
Tang, R., Liu, S., and Jiang, N. (1983). The 1933 Diexi Earthquake: Chengdu. Chengdu: Sichuan Science and Technology Press. (In Chinese).
Tapponnier, P., Zhiqin, X., Roger, F., Meyer, B., Arnaud, N., Wittlinger, G., et al. (2001). Oblique Stepwise Rise and Growth of the Tibet Plateau. Science 294, 1671–1677. doi:10.1126/science.105978
Tian, Y., Kohn, B. P., Phillips, D., Hu, S., Gleadow, A. J. W., Carter, A., et al. (2016). Late Cretaceous-earliest Paleogene deformation in the Longmen Shan fold-and-thrust belt, eastern Tibetan Plateau margin: Pre-Cenozoic thickened crust?. Tectonics 35 (10), 2293-2312. doi:10.1002/2016TC004182
Trauth, M. H., and Strecker, M. R. (1999). Formation of Landslide-Dammed Lakes during a Wet Period between 40,000 and 25,000 Yr B.P. In Northwestern Argentina. Palaeogeogr. Palaeoclimatol. Palaeoecol. 153 (1), 277–287. doi:10.1016/s0031-0182(99)00078-4
Wang, C., Liu, Z., Yi, H., Liu, S., and Zhao, X. (2002). Tertiary Crustal Shortening and Peneplanation in the Hoh Xil Region: Implications for the Tectonic History of the Northern Tibetan Plateau. J. Asian Earth Sci. 20 (3), 211–223. doi:10.1016/s1367-9120(01)00051-7
Wang, L., Dun, L., Yang, L., and Wang, X. (2005). Discovery of Huge Ancient Dammed lake on Upstream of Minjiang River in Sichuan, China. J. Chengdu Univ. Technol. (science Technol. edition 32 (1), 1–11. (In Chinese with English Abstract). doi:10.3969/j.issn.1671-9727.2005.01.001
Wang, Z., Cheng, D., He, Y., and Wang, H. (2006). A Study of the Ecological Functions of Step-Pool System in Southwest Mountain Streams. Adv. Earth Sci. 21 (4), 409–416. (In Chinese with English Abstract). doi:10.3321/j.issn:1001-8166.2006.04.010
Wang, L., Wang, X., Xu, X., and Cui, J. (2007). What Happened on the Upstream of Minjiang River in Sichuan Province 20 000 Years Ago. Earth Sci. Front. 14 (4), 189–196. (In Chinese with English Abstract). doi:10.1016/s1872-5791(07)60037-2
Wang, P., Li, J., Wang, J., Liu, C., Han, F., and Gao, L. (2011a). Quartz Ti-center in ESR Dating of Xigeda Formation in Sichuan and Contrast with Magnetic Stratigraphic Profiles. Nucl. Tech. 34 (2), 111–115. (In Chinese with English Abstract).
Wang, P., Zhang, B., Qiu, W., and Wang, J. (2011b). Soft-sediment Deformation Structures from the Diexi Paleo-Dammed Lakes in the Upper Reaches of the Minjiang River, East Tibet. J. Asian Earth Sci. 40 (4), 865–872. doi:10.1016/j.jseaes.2010.04.006
Wang, E., Kirby, E., Furlong, K. P., van Soest, M., Xu, G., Shi, X., et al. (2012). Two-phase Growth of High Topography in Eastern Tibet during the Cenozoic. Nat. Geosci 5 (9), 640–645. doi:10.1038/ngeo1538
Xu, G., and Kamp, P. J. J. (2000). Tectonics and Denudation Adjacent to the Xianshuihe Fault, Eastern Tibetan Plateau: Constraints from Fission Track Thermochronology. J. Geophys. Res. 105 (B8), 19231–19251. doi:10.1029/2000jb900159
Xu, J., and Wang, Z. (2004). Formation and Mechanism of Step-Pool System. J. Hydraulic Eng. 35 (10), 48–55. (In Chinese with English Abstract). doi:10.3321/j.issn:0559-9350.2004.10.008
Xu, Z. (2011). Deposits of Zhaizicun Landslide-Dammed Lake along Jinsha River and its Implication for the Genesis of Xigeda Formation. Geol. Rev. 57 (5), 675–686. (In Chinese with English Abstract). doi:10.1007/s10114-011-0511-z
Xu, Z., and Liu, W. (2011). Some Problems in the Study of the Genesis of the Xigeda Formation. Earth Sci. Front. 18 (5), 256–270. (In Chinese with English abstract).
Yan, B., and Lin, A. (2015). Systematic Deflection and Offset of the Yangtze River Drainage System along the Strike-Slip Ganzi-Yushu-Xianshuihe Fault Zone, Tibetan Plateau. J. Geodynamics 87, 13–25. doi:10.1016/j.jog.2015.03.002
Yang, R., Suhail, H. A., Gourbet, L., Willett, S. D., Fellin, M. G., Lin, X., et al. (2020). Early Pleistocene Drainage Pattern Changes in Eastern Tibet: Constraints from Provenance Analysis, Thermochronometry, and Numerical Modeling. Earth Planet. Sci. Lett. 531, 115955. doi:10.1016/j.epsl.2019.115955
Yao, H., Zhao, Z., Qiao, Y., Li, C., Wang, S., Wang, Y., et al. (2007). Magnetostratigraphic Dating of the Xigeda Formation in Mianning. Sichuan and its significance 27 (1), 74–84. (In Chinese with English abstract). doi:10.3321/j.issn:1001-7410.2007.01.009
Yin, A., Dang, Y. Q., Wang, L. C., Jiang, W. M., Zhou, S. P., Chen, X. H., et al. (2008). Cenozoic Tectonic Evolution of Qaidam basin and its Surrounding Regions (Part 1): The Southern Qilian Shan-Nan Shan Thrust belt and Northern Qaidam basin. GSA Bull. 120 (7), 813–846. doi:10.1130/b26180.1
Yin, A., and Harrison, T. M. (2000). Geologic Evolution of the Himalayan-Tibetan Orogen. Annu. Rev. Earth Planet. Sci. 28 (1), 211–280. doi:10.1146/annurev.earth.28.1.211
Zhang, P. (2008). The Present Day's Deformation, Strain Distribution and Deep Dynamic Process on the Western Sichuan, Eastern Tibetan Plateau. Sci. China Ser. D 38 (9), 1041–1056. (In Chinese). doi:10.1360/zd2008-38-9-1041
Zhang, P., Molnar, P., and Downs, W. R. (1994). Increased Sedimentation Rates and Grain Sizes 2–4? Myr Ago Due to the Influence of Climate Change on Erosion Rates. Nature 410 (6831), 891–897. doi:10.1038/35073504
Zhang, Y., Yang, N., and Meng, H. (2005). Deep-incised Valleys along the Minjiang River Upstream and Their Responses to the Uplift of the West Sichuan Plateau, China. J. Chengdu Univ. Tech. (Science Tech. edition 32 (4), 331–339. (In Chinese with English abstract). doi:10.3969/j.issn.1671-9727.2005.04.001
Zhang, Y., Dong, S., and Yang, N. (2009). Active Faulting Pattern, Present-Day Tectonic Stress Field and Block Kinematics in the East Tibetan Plateau. Acta Geologica Sinica 83 (4), 694–712. doi:10.1111/j.1755-6724.2009.00093.x
Zhang, K., Wang, Z., Liu, H., and Yu, G. (2011). Effect of Knickpoint Development in Controlling the Stability of the Landslide Dam. J. Mountain Sci. 29 (4), 474–482. doi:10.3969/j.issn.1008-2786.2011.04.012
Zhang, Y., Li, H., Wu, M., and Liao, C. (2012). Late Cenozoic Thrust and Nappe Structure along the Minjiang Upstream: Evidence from a Drill Hole. Geol. Rev. 58 (2), 215–223. (In Chinese with English abstract). doi:10.3969/j.issn.0371-5736.2012.02.003
Zhao, X., Hu, D., and Zhang, Y. (2008). Genesis and Age of the Gravels Underlying the Xigeda Formation of Panzhihua, Sichuan , China , and Valley Development of the Ancient Jinsha River. Acta Geoscientia Sinica 29 (1), 1–12. (In Chinese with English abstract). doi:10.3321/j.issn:1006-3021.2008.01.001
Keywords: Eastern tibet, continental transpressional zone, Earthquake-induced dammed lakes, step-pool system, high-elevation and high-relief topography
Citation: Li H, Sun Y and Zhang Y (2022) Chain Actions Generated High-Elevation and High-Relief Topography of the Eastern Margin of the Tibetan Plateau: From Deep Earth Forces to Earthquake-Induced Dams. Front. Earth Sci. 10:791264. doi: 10.3389/feart.2022.791264
Received: 08 October 2021; Accepted: 24 January 2022;
Published: 22 February 2022.
Edited by:
Xiaoping Yuan, GFZ German Research Centre for Geosciences, GermanyCopyright © 2022 Li, Sun and Zhang. This is an open-access article distributed under the terms of the Creative Commons Attribution License (CC BY). The use, distribution or reproduction in other forums is permitted, provided the original author(s) and the copyright owner(s) are credited and that the original publication in this journal is cited, in accordance with accepted academic practice. No use, distribution or reproduction is permitted which does not comply with these terms.
*Correspondence: Hailong Li, 13811539443@126.com; Yujun Sun, sunyujunabc@163.com