- Laboratory of Mechanics on Disaster and Environment in Western China, Ministry of Education, School of Civil Engineering and Mechanics, Lanzhou University, Lanzhou, China
The type and structure of clay minerals are among the main factors affecting the physical and mechanical properties of loess, and the salt content plays a dominant role in many factors affecting aggregation. In this study, the grain size analysis, Atterberg limits tests, scanning electron microscopy (SEM), and energy spectrum analysis (EDS) were used to explore the microstructure and physicochemical mechanisms of the aggregation of clay particles. Finally, the unconfined compressive test, direct shear test, and elastic wave velocity test were conducted to explore the effect of sulfate agglomeration on loess properties. The results show that the salt-washing process leads to the loss of the original soluble salt, dispersion of the original aggregates, and increase of fine particles, such as fine silt and clay. With the increase of sulfate content, the content of fine silt and clay decreases, the liquid limit and plasticity index decrease, the plasticity limit remains unchanged, the aggregate content of fine silt and clay increases, the adsorption capacity weakens, and the diffusion layer compresses and thins. The mechanical test results show that when the sulfate content is less than 3%, the sulfate is almost completely dissolved and the aggregate is less, which increases compressive strength and the cohesion; when the sulfate content is more than 3%, too much sulfate cannot be dissolved and precipitated; hence, the aggregate increases. However, the existence of large volume mirabilite crystals reduces the cementation, which reduces the compressive strength, increases the internal friction angle, and weakens the cohesion.
Introduction
There have been many studies on the interaction between salt and clay minerals, and these interactions are mainly manifested in the macroscopic effect on the Atterberg limits. Some scholars have tested bentonite, kaolin, beidellite, montmorillonite, and artificial bentonite kaolin mixtures (Di Maio et al., 2004), and others have tested the consistency limits liquid limit, plastic limit, plasticity index, sediment volume, and squeezing properties) of 10 soil types with distilled water and natural seawater (Yukselen-Aksoy et al., 2008), and all of the results have indicated that salt has a greater influence on the plastic limit of montmorillonite but less on kaolinite and illite. Based on a large number of experiments, the proportion, optimum water content, liquid limit, and plastic limit of the saline soil prepared from the Luoyang loess were obtained, which decreased with the increase of chloride content, with a linear variation law; the maximum dry density of the chloride saline soil increased with the increase of salt content when the salt content was less than 8% and decreased with the increase of salt content when the salt content was more than 8% (Wang et al., 2009). (Bjerrum and Rosenqvist, 1956a) found that the shear strength of clay deposited in fresh water would be two to three times of that deposited in brine. Using the clay consistency limits of four different salt solutions (ammonium chloride, potassium chloride, copper sulfate, and ferrous sulfate) at eight different concentrations, it was proven that the liquid limit increased with the increase of the concentration of the salt solution containing chloride ions and decreased with the increase of the concentration of the salt solution containing sulfate ions. In addition, whether it was saline soil containing chloride or sulfate ions, the plastic limit of clay decreased at a low salt concentration and increased at a high salt concentration (Arasan and Yetimoglu, 2008). By studying the effects of three different inorganic salt solutions on the mixing characteristics, liquid limits, free expansion, consolidation characteristics, and water conductivity of two clay–bentonite mixtures, it was found that the salt content of the salt solution increased the maximum dry density of the mixture and lowered the optimum moisture content and liquid limit of the mixture. The main reason for this effect was that the diffusion layer of the clay particles changed (Karimpour-Fard et al., 2011), which is consistent with the results of the study conducted by (Mishra et al., 2005). Some studies illustrated that the liquid limit of montmorillonite was significantly reduced by the influence of the salt solution (Di Maio, 1996; Gleason et al., 1997). The thickness of the diffusion layer was mainly caused by the concentration of sodium ions in pore water (Rao et al., 1993).
The aggregation of clay particles is the main microscopic mechanism of the influence of salt on Atterberg limits. From a quiescent sedimentation test of montmorillonite with different salt content, it was found that the aggregation process presented different speeds with the change of salt content, and the speed was the fastest close to 19% salt content and the slowest close to 1% salt content (Lin et al., 2013). SEM and FT-IR were applied to analyze the microstructure of aggregates, and it was found that the strength and particle size of aggregates increased with increasing salt content. The role of salt was to replenish the electrolyte, where the electric double layer adhered to form a dense aggregate (Liu et al., 2007a). In addition, a 2.25 m settling column was used to study the effect of the salt content on the aggregation velocity of fine particles, indicating that the aggregation velocity increased with the increase of salt content and that the aggregation velocity in brine was greater than that of fresh water (Portela et al., 2013). Some scholars measured the particle aggregation rate of three types of clays in the same salt solution and found that the probability of montmorillonite aggregation was higher than that of kaolin and illite, and montmorillonite was most easily aggregated (Edzwald and O’melia, 1975). However, it was found that the aggregation characteristics of different clay minerals (montmorillonite, illite, and kaolin) in still water affected by the salt content of water were different: illite and kaolin were both aggregated at a salt content of 2–3%, while the aggregation of montmorillonite did not change significantly with salt content (Whitehouse et al., 1958a).
The type and structure of clay minerals are among the main influencing factors affecting the physical and mechanical properties of the loess. There is a special soil layer called the loess salted soil distributed in Northwest China, which has the characteristics of Malan loess and saline soil. Because of the interaction of salt and clay minerals, the physical and mechanical properties of loess salted soil are complex. At present, studies on sulfate saline soil mostly focused on exploring the influence of salt change on the internal structure of single-clay minerals such as highly active bentonite and the influence of salt change on the macroscopic properties of soil (Liu et al., 2007b). Macroscopically, it is mainly manifested in the study of limit water content (Aksoy et al., 2008). Sivchantra explored the shear strength of saline soil with different water content and salt content at different temperatures. The results show that the shear strength decreases with the increase of salt content at room temperature, has the highest shear strength at zero, and 0 and 0.5% saline soil have the highest shear strength at zero (Chantra, 2019). Di Mai et al. (2004) showed that salt has a great impact on the liquid plastic limit of montmorillonite but has little impact on kaolinite and illite (Aksoy et al., 2008). However, there are few studies on the influence of salt change on the physical and mechanical properties of loess clay agglomeration.
Therefore, sulfate saline soil with different mass ratios (0, 0.3, 0.5, 3, 5.0, and 8.0%) was prepared from the soil after salt washing. In this study, based on the grain size analysis and Atterberg limits test, the aggregation of clay particles by sulfate was studied. Scanning electron microscopy (SEM) and energy spectrum analysis (EDS) were used to study the microstructure and physicochemical mechanisms of the aggregation of clay particles. Finally, through the unconfined compressive test, direct shear test and elastic wave velocity test were conducted to explore the effect of sulfate agglomeration on loess engineering properties.
Materials and Experimental Methods
Materials
The original loess used in the experiment is Malan loess in Lanzhou. The natural water content is 5.89%. The basic physical parameters are shown in Table 1.
Anhydrous sodium sulfate is a kind of white crystalline particle or powder, the content of which is not less than 99%. The specifications are shown in Table 2.
Sample Preparation
To explore the nature of the fine particles and avoid the influence of larger particles on the test results, the salted soil was crushed and passed through a 2-mm sieve to remove impurities such as organic compounds or large particles. Then, the sieved soil sample and distilled water were mixed according to a volume ratio of 1:5, and the salt was sufficiently dissolved by stirring. After resting the solution until clarified, the supernatant was sucked out by a straw to leave behind a soil sample. After repeated artificial desalination of the soil samples, the desalted loess was obtained, and the process is defined as salt washing. It is necessary to test the conductivity of each soil suspension with a conductivity meter to test the desalting effect of soil samples. In addition, the temperature was controlled at 20°C during the test. Conductivity test results of original loess are shown in Table 3.
According to the preparation gradient, a certain amount of anhydrous sodium sulfate in powder was weighed to form the sulfate saline soil with 0, 0.3, 0.5, 3, 5, and 8% salt content. The prepared saline soil was placed for 7 days to obtain the maximum exchange adsorption of salt in the soil sample. To make the salt completely displace in the soil, the soil sample after adsorption should be added with sufficient pure water, immersed in pure water for 2 days, and then dried in a natural state so that the salt can completely displace in the soil. The air-dried samples were reground, mixed, and passed through a 2-mm sieve.
Experimental Methods
The Atterberg limits of soil reflect the difficulty with which the soil changes from one state to another. The greater the plasticity index of the soil, the stronger the plasticity is. An LP-100D liquid-plastic limit tester was used to measure the depth of the cone at different water contents. A Mastersizer 2,000 laser particle size analyzer was used to test the particle size and determine the mass fraction of each particle group. A Thermoscientific Apreos scanning electron microscope was used to characterize the micromorphology, and the chemical element analysis was analyzed by an INCA energy-dispersive X-ray spectroscopy (EDS) system. Therefore, on the basis of the aforementioned tests, the unconfined compressive strength and elastic wave velocity were studied by a CSS-WAW300 universal material testing machine and RSM-SY5 (T) non-metallic ultrasonic testing analyzer. A ZJ strain–controlled direct shear apparatus was used to explore the relationship between cohesion, internal friction angle, and salt content. The test instrument information is shown in Table 4.
Results and Analyses
Physical Index
The Results of the Grain Size Analysis Test
Figure 1 is the grain size analysis graph of loess samples before and after salt washing. The loess before salt washing is defined as the loess without desalination, and the loess after salt washing is defined as saline soil with 0% salt content. It can be seen from the figure that the particle composition of the loess without desalination and saline soil with 0% salt content is mainly powder, the content of which is more than 80%. The content of fine particles in the soil with 0% salt content increased, and the amount of fine silt and clay (<0.01 mm) increased by 6%. The results show that the salt washing process makes the larger particles finer so that the cumulative percentage content of each particle size increases compared with the loess without desalination.
Figure 2 shows the grain size analysis graph of different sulfate contents in saline soil. It can be seen that the fine silt and clay of saline soil display obvious changes. When the particle size is greater than 0.075 mm (mainly sand), the change in the particle size distribution graph is small with the change of the sulfate content, indicating that sulfate content has no effect on the particle size; when the particle size is less than 0.075 mm, there is a significant difference in the grain size analysis graph between the saline soil and desalted soil, with a maximum difference of 7.48%. With the addition of sulfate, the particle state changes, and the content of fine particles decreases.
According to the particle analysis curve, different grading parameters of all soil samples can be obtained. The results are shown in Table 5. It can be seen that the non-uniformity coefficient of all the samples is greater than 5, and the curvature coefficient is 1–3. Therefore, the existence of sulfate has no effect on soil gradation.
The grain composition of soil includes the particle size and proportion of each particle size in the soil, among which particle size is the main factor affecting soil properties. With the increase in sulfate concentration, the ion content increases gradually, and the thickness of the colloidal diffusion layer gradually decreases, which breaks the balance of the electrostatic force in the soil. In addition, the decrease of fine particles in soil leads to the decrease in the ability of the soil to adsorb weakly bound water and enhancing the aggregation of fine particles and clay particles. The addition of sulfate as an electrolyte reduces the proportion of fine particles and clay because of aggregation, which leads to a decrease of wet water of the cohesive soil and capillary water rising height and affects the water-physical properties of the soil, such as permeability, disintegration, and grain composition.
The Results of the Atterberg Limits Test
To ensure the reliability of the test results, three parallel tests are conducted for each sample, and the test parameters within the allowable error range are selected as the final results. That is, the double-logarithm curve of water content–cone penetration depth shall ensure that the two straight lines formed by the high water content point and the other two water content points and the difference of water content at the subsidence of 2 mm shall be less than 2%.
Table 6 shows the Atterberg limits graph of all samples. It can be seen from the table that the loss of soluble sulfate and scattering of agglomerated particles in the process of salt washing leads to the increase of clay particles, resulting in the increase of liquid limit, plastic limit, and plastic index of the test loess after salt washing. In addition, when the sulfate content is less than 3%, the liquid limit, plastic limit, and plasticity index fluctuation value fluctuate greatly; when the sulfate content is more than 3%, the liquid limit, plastic limit, and plastic index are stable. In general, with the increase of sulfate content, the liquid limit and plastic index decrease, and the plastic limit changes slightly but has a certain increasing trend.
On the one hand, the sulfate solution in soil pores gradually reaches saturation, while the excess sulfate exists in the form of crystals between the soil particles (Chen et al., 2015). The existence of sulfate crystals has a certain effect of plugging and cementation on the soil pores, which makes the pore diameter of the soil reduce, the soil more dense, and the plasticity reduce. On the other hand, as an electrolyte, sulfate changes the concentration of water and the content ratio of ions in the soil, aggregating the fine silt and clay so that the proportion is relatively increased, and the electric double-layer on the surface of the soil particles is affected. The diffusion layer is compressed, the thickness of the hydrated film is thinned, the repulsion between the soil particles is weakened, the attraction is enhanced, the spacing of the particles is reduced, and the amount of water that can be adsorbed by the particles is increasingly less (Lei et al., 2001). In general, the liquid limit and plasticity index of the soil decrease.
Microscopic Test
SEM
To clearly observe the flocculated structure of “soil particles,” 1,000× electron microscopy magnification was chosen. SEM was labeled and is shown in Figure 4. Figures 3A–F show that with the increasing sulfate content, sulfate as an electrolyte has an aggregation effect on some fine particles. According to the results of the granule analysis test, the aggregation consists of fine silt and clay. With the increase of sulfate content, the number and volume of irregular aggregates on the soil surface increase, that is, sulfate has a certain degree of elutriation on soil particles. When the sulfate content reaches 8%, a large number of sodium sulfate crystals are precipitated.
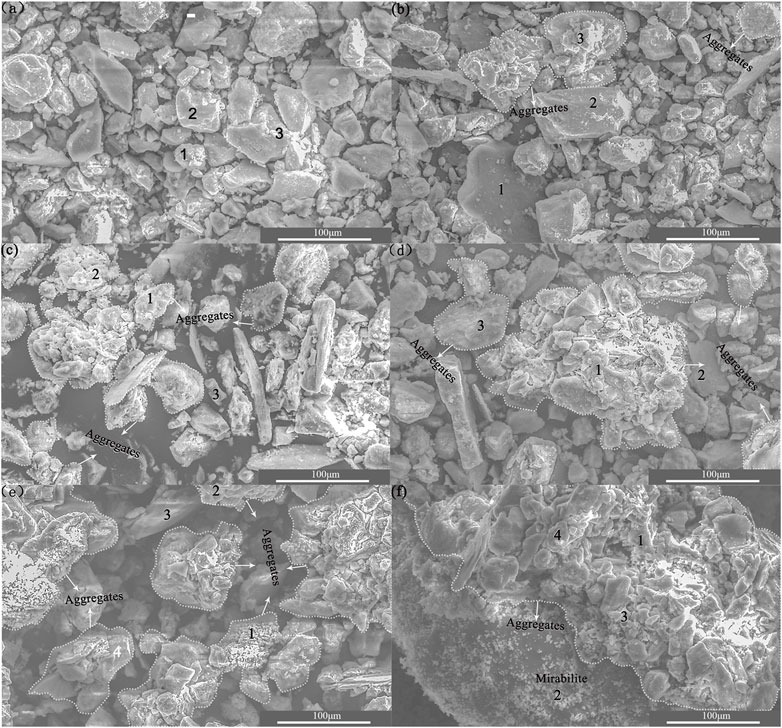
FIGURE 3. SEM graph of different sulfate content in saline soil (A): 0% salt content, (B): 0.3% salt content, (C): 0.5% salt content, (D): 3.0% salt content, (E): 5.0% salt content, and (F): 8.0% salt content.
EDS
Table 7 shows the element weight percentage statistics of different sulfate contents in saline soil according to EDS. It can be seen from Table 5 that the test soils with different sulfate contents generally comprise C, O, Na, Al, Si, etc., of which the most abundant element is O, and the content of Si is second. The composition elements and proportion of saline soil with different sulfate contents are approximately the same. For the same clay mineral, the Si/Al ratio decreases with increasing sulfate content.
Mechanical Property
The results of basic physical tests and microscopic test show that the existence of sulfate makes the clay particles agglomerate in the loess, and the change of the internal structure of loess particles will inevitably have a certain impact on the mechanical properties of the loess.
Compaction Test
Figure 4 shows the compaction curve of saline soil with different sulfate content. It can be seen from the figure that the curve change trend of different sulfate content of sulfate soil is similar. On the dry side of the compaction curve (the moisture content is lower than the optimal moisture content), the dry density increases with the increase of the moisture content and vice versa. But the peak point of the compaction curve is different. With the increase of sulfate content, the peak point shifts to the lower right, that is, with the increase of sulfate content, the optimum moisture content increases, and the maximum dry density decreases.
When the sulfate content is low, the sodium sulfate is almost completely dissolved. With the increase of the sulfate content, the precipitated sodium sulfate crystals further absorb water to form larger mirabilite crystals. The existence of crystals will increase the connection strength of soil, resulting in the decrease of the maximum dry density of saline soil. In addition, because of the strong hydration of sodium ions and existence of sodium sulfate decahydrate, the optimum moisture content increases with the increase of sulfate content (Pécsi and Bariss, 1993).
Compression Test
Figure 5 shows the relationship between compressive strength and sulfate content of sulfate saline soil. It can be seen from the figure that with the increase of sulfate content, the compressive strength increases first and then decreases, and the maximum compressive strength is obtained in this process. It shows that a certain amount of sulfate in saline soil can improve the strength of soil, but too much sulfate in saline soil is unfavorable to the soil.
The cementation of sulfate crystals has gradually developed into the main factor affecting the compression test. With the increase of sulfate content, the cementation becomes stronger, and the compressive strength reaches the peak. After the peak point, with the increase of sulfate content, excessive aggregates and larger sulfate crystals lead to the increase of soil porosity and roughness, which lead to the decrease of cementation and compressive strength.
Shear Test
Figure 6 shows the relationship between vertical pressure (100 kPa, 200 kPa, 300 kPa, and 400 kPa) and shear strength of sulfate saline soil with different sulfate content. It can be seen from the figure that with the change of sulfate content, the trend of the curve is similar, but the slope and intercept of the curve have great changes, that is, the existence of sulfate has a great influence on the shear strength of the soil.
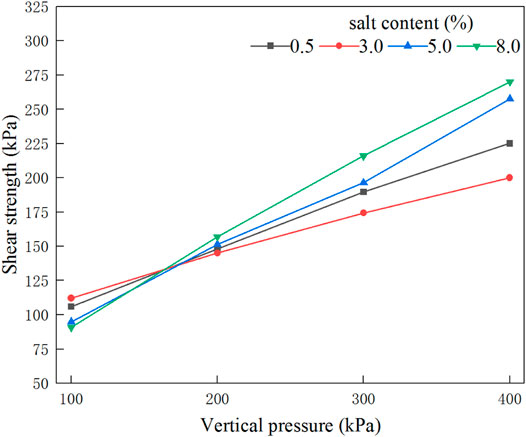
FIGURE 6. Relationship between vertical pressure and shear strength of sulfate saline soil with different sulfate content.
Figure 7 shows the relationship between sulfate content and cohesion and internal friction angle. It can be seen from the Figure 7A that with the increase of sulfate content, the cohesion of sulfate soil increases first and then decreases and changes in the range of 34.22 ∼84.59 kPa. When the sulfate content is less than 3%, the cohesion increases with the increase of sulfate content. When the sulfate content is more than 3%, the cohesion decreases gradually. The change trend of the internal friction angle and cohesion is basically opposite, as shown in Figure 7B.
When the sulfate content of the soil is low, with the increase of the sulfate content, the soluble sulfate content gradually reaches saturation (Williams, 1957), and the excess sulfate in the sample precipitates in the form of sodium sulfate crystals, which act as the skeleton of the soil particles so that the cohesion between the soil particles is enhanced. The fine particles such as fine powder and clay aggregate result in the reduction of sliding friction between the particles. When the sulfate content of soil is high, more fine silt and clay aggregate together, the friction and bite force decrease, the internal friction angle of the soil increases, and cohesion decreases (Handy and Spangler, 2006).
Elastic Wave Velocity Test
Figure 8 shows the wave velocity test results of samples with different sulfate contents. The elastic wave velocity can indirectly reflect the strength of the soil. It can be seen from the figure that the elastic wave velocity of sulfate-bearing samples is generally higher than that of loess samples after salt washing; with the increase of sulfate content, the elastic wave velocity of the samples increases, indicating that the existence of sulfate plays a certain role in the solidification of soil.
Discussion
(1) The results presented herein confirm that the aggregates formed by sulfate change the physical structure of the loess, resulting in changes in the physical and mechanical properties of the loess, but the mineral composition experiences no noticeable change. In the previous studies, scholars mainly explored the influence of the existence of salt on its physical and mechanical properties in the macro aspect of saline soil and did not delve into the relationship between agglomeration and property changes (Bjerrum and Rosenqvist, 1956b; Bjerrum, 1967; Chen and Anadarajah, 1998).Moreover, the research on the microscopic properties of agglomeration of saline soil mostly focuses on a single clay mineral, mainly on the boundary moisture content, and does not involve whether new substances are produced in the agglomeration process (Edzwald and O’melia, 1975; Whitehouse et al., 1958b). However, the cementation between aggregates, that is, more uniform aggregate formation have contributed to the physical structure modification, which is responsible for the changes in physicochemical and index properties (Kong et al., 2018; Kong et al., 2019). Therefore, the change of loess properties with different sulfate content is not a chemical reaction but related to the change of the physical structure caused by the formation of aggregates.
(2) In addition, scholars have studied the agglomeration of single clay minerals and found that the number and volume of aggregates increase with the increase of sulfate, but when the salt content increases to a certain extent, the agglomeration speed decreases (Liu et al., 2007b; Edzwald and O’melia, 1975). At the same time, scholars also found that with the increase of sulfate, the mechanical strength of the loess first increased and then decreased, indicating that a large salt content will inhibit the mechanical strength of the soil (Chatterj and Jensen, 1999; Aksoy et al., 2008). However, it should be noted that after the mechanical properties of the loess reached the maximum, the number of aggregates increased slowly and the volume increased significantly, mainly because the aggregates in the later stage were further agglomerated from the existing aggregates into large particles, which affected the structure of the loess and led to the decline of mechanical strength. But, this conclusion has only been confirmed in a single sulfuric acid saline soil, and other types of saline soil and mixed saline soil still need further research. As a whole, the agglomeration of fine particles of a small amount of saline soil may facilitate improving loess performance, and thus it has potential as a new treatment technique for saline soil improvement so as to improve the resource utilization rate of saline soil in engineering construction.
(3) Finally, this study adopts a special research method of combining macro and microscopic aspects. It is found that the agglomeration in saline soil mainly concerns fine silt and clay. In the early stage, with the increase of salt content, the agglomeration speed is fast, the volume is small, and the mechanical strength of loess increases gradually. In the later stage, with the increase of sulfate, the agglomeration speed is slow, the volume increases, the mechanical strength of loess decreases, and no new substances are produced in the whole process of agglomeration (Figures 2, 3, 5, 7). Thus, sulfate has the potential to be used as an eco-friendly additive in soil improvement.
Conclusion
(1) Salt washing makes the larger particles finer, and the particles which are easy to dissolve and agglomerate are washed away, resulting in the increase of clay particles, liquid limit, plastic limit, and plastic index.
(2) Aggregation occurs in the state of fine silt and clay with lower sulfate content, and as the sulfate content increases, new fine silt and clay aggregations do not occur, but the existing aggregations will further aggregate into large particles. With increasing sulfate content, the number and volume of aggregates increased, and the content of fine silt and clay decreased.
(3) With the increase in sulfate content, the aggregation particles increase, the framework grains change from point-surface contact to plane–plane contact, the adsorption capacity decreases, the compressibility increases, and the hydration film becomes thinner.
(4) When the sulfate content is low (less than 3%), the sulfate is almost completely dissolved and exists in the form of ions. The connection of soil is mainly based on the bound water, which has less influence on the limit water content. In addition, with the increase of sulfate content, the gradual precipitation of sodium sulfate crystals in the soil makes the internal cementation become stronger, the maximum dry density increase, the compressive strength increase, and the cohesion increase. When the sulfate content is large (more than 3%), excess sulfate cannot be dissolved and is precipitated in the form of crystals. The connection of the soil changes into the cementation inosculation of bound water and soluble sulfate, which has a great influence on the limit water content. With the increase of sulfate content, because of the strong hydration of sodium ions, a large number of sodium sulfate crystals are precipitated, and the increase of aggregates leads to the maximum dry density decrease, compressive strength decrease, internal friction angle increase, and cohesion decrease.
Data Availability Statement
The original contributions presented in the study are included in the article/Supplementary Material, further inquiries can be directed to the corresponding author.
Author Contributions
All authors listed have made a substantial, direct, and intellectual contribution to the work and approved it for publication.
Funding
This study was supported by the National Natural Science Foundation of China (No.51878322) and Major scientific and technological projects in Gansu Province (No. 19ZD2FA001).
Conflict of Interest
The authors declare that the research was conducted in the absence of any commercial or financial relationships that could be construed as a potential conflict of interest.
Publisher’s Note
All claims expressed in this article are solely those of the authors and do not necessarily represent those of their affiliated organizations, or those of the publisher, the editors, and the reviewers. Any product that may be evaluated in this article, or claim that may be made by its manufacturer, is not guaranteed or endorsed by the publisher.
References
Aksoy, Y. Y., Kaya, A., and Ören, A. H. (2008). Seawater Effect on Consistency Limits and Compressibility Characteristics of Clays[J]. Eng. Geology. 102 (1-2), 54–61. doi:10.1016/j.enggeo.2008.07.005
Arasan, S., and Yetimoglu, T. (2008). Effect of Inorganic Salt Solutions on the Consistency Limits of Two Clays[J]. Turkish J. Eng. Environ. Sci. 32, 107–115.
Bjerrum, L. (1967). Engineering Geology of Norwegian Normally-Consolidated marine Clays as Related to Settlements of Buildings [J]. Geotechnique 17 (02), 83–138. doi:10.1680/geot.1967.17.2.83
Bjerrum, L., and Rosenqvist, I. T. (1956). Some Experiments with Artificially Sedimented Clays. Géotechnique 6, 124–136. doi:10.1680/geot.1956.6.3.124
Bjerrum, L., and Rosenqvist, I. T. (1956). Some Experiments with Artificially Sedimented Clays[J]. Geotechnique 3 (06), 124–136. doi:10.1680/geot.1956.6.3.124
Chantra, S. I. V. (2019). Preliminary Study of Shear Strength of Soil Containing Sodium sulfate[D]. Beijing: Beijing Jiaotong University.
Chatterj, S., and Jensen, A. D. (1999). Efflorescence and Breakdown of Building Materials[J]. Nordic Concrete Res. 8, 56–61.
Chen, J., and Anadarajah, A. (1998). Influence of Pore Fluid Composition on Volume of Sediments in Kaolinite Suspensions [J]. Clays and Clay Minerals 2 (46), 145–152. doi:10.1346/ccmn.1998.0460204
Chen, W. W., Lv, H. M., and Cui, K. (2015). Comparative Study of the Influence of Chlorine and Sulfate Salt on Grain Size Distribution and Limit Moisture Content in Site Soils[J]. J. Lanzhou Univ. (Natural Sciences) 51, 334–338.
Di Maio, C. (1996). Exposure of Bentonite to Salt Solution: Osmotic and Mechanical Effects. Géotechnique 46, 695–707. doi:10.1680/geot.1996.46.4.695
Di Maio, C., Santoli, L., and Schiavone, P. (2004). Volume Change Behaviour of Clays: the Influence of mineral Composition, Pore Fluid Composition and Stress State. Mech. Mater. 36, 435–451. doi:10.1016/s0167-6636(03)00070-x
Di, M. C., Santoli, L., and Schiavone, P. (2004). Volume Change Behavior of Clays: the Influence of mineral Composition, Pore Fluid Composition and Stress State [J]. Mech. Mater. 36 (5-6), 435–451. doi:10.1016/s0167-6636(03)00070-x
Edzwald, J. K., and O'melia, C. R. (1975). Clay Distributions in Recent Estuarine Sediments. Clays and Clay Minerals 23, 39–44. doi:10.1346/ccmn.1975.0230106
Edzwald, J. K., and O’melia, C. R. (1975). Clay Distributions in Recent Estuarine Sediments [J]. Clays and Clay Minerals 23 (01), 39–44. doi:10.1346/ccmn.1975.0230106
Gleason, M. H., Daniel, D. E., and Eykholt, G. R. (1997). Calcium and Sodium Bentonite for Hydraulic Containment Applications. J. Geotechnical Geoenvironmental Eng. 123, 438–445. doi:10.1061/(asce)1090-0241(1997)123:5(438)
Karimpour-Fard, M., Shariatmadari, N., and Salami, M. (2011). Effect of Inorganic Salt Solutions on Some Geotechnical Properties of Soil-Bentonite Mixtures as Barriers[J]. Int. J. Civil Eng. 9, 103–110.
Kong, R., Yan, B., Xu, J., Shi, Z., Peng, Q., and Lin, X. (2019). Physical Homogenization and Chemical Stability of Nano-SiO2 Treated Loess. Soil Mech. Found. Eng. 56, 336–339. doi:10.1007/s11204-019-09611-9
Kong, R., Zhang, F., Wang, G., and Peng, J. (2018). Stabilization of Loess Using Nano-SiO2. Materials 11, 1014. doi:10.3390/ma11061014
Lei, H. Y., Zhang, W. S., and Zhang, X. F. (2001). Study on Engineering Property Indexes of Ultracholrine saline Soil[J]. J. Changchun Univ. Sci. Technology 31, 70–73.
Lin, J., Xie, D. H., and Lu, T. L. (2013). Effect of Salinity on the Flocculation of Montmorillonite Mineral[J]. China Water Transport 13, 121–123.
Liu, Q., Li, J., and Dai, Z. (2007). Flocculation Process of fine-grained Sediments by the Combined Effect of Salinity and Humus in the Changjiang Estuary[J]. Acta Oceanologica Sinica 26, 140–149.
Liu, Q. Z., Li, J. F., and Dai, Z. J. (2007). Flocculation Process of fine Grained Sediments by the Combined Effect of Salinity and Humus in the Changjiang Estuary[J]. Acta Oceanologica Sinica 26 (01), 1–10.
Mishra, A. K., Ohtsubo, M., Li, L., and Higashi, T. (2005). Effect of Salt Concentrations on the Permeability and Compressibility of Soil-Bentonite Mixtures. Journal- Fac. Agric. Kyushu Univ. 50, 837–849. doi:10.5109/4692
Pécsi, M., and Bariss, N. (1993). Quaternary and Loess research[M]. Hungarian: Geographical Research Institute, Hungarian Academy of Sciences.
Portela, L. I., Ramos, S., and Teixeira, A. T. (2013). Effect of Salinity on the Settling Velocity of fine Sediments of a Harbour basin. J. Coastal Res. 165, 1188–1193. doi:10.2112/si65-201.1
Rao, S. M., Sridharan, A., and Chandrakaran, S. (1993). Consistency Limits Behavior of Bentonites Exposed to Sea Water. Mar. Georesources Geotechnology 11, 213–227. doi:10.1080/10641199309379919
Wang, L., Dang, J., and Yang, X. (2009). The Research of Soil-Water Characteristic Curves of saline Soil[J]. Geotechnical Invest. Surv. 37, 19–23. doi:10.1109/CLEOE-EQEC.2009.5194697
Whitehouse, U. G., Jeffrey, L. M., and Debbrecht, J. D. (1958). Differential Settling Tendencies of clay Minerals in saline Waters [J]. Clays Clay Mineral. 7 (01), 1–79. doi:10.1346/ccmn.1958.0070102
Whitehouse, U. G., Jeffrey, L. M., and Debbrecht, J. D. (1958). Differential Settling Tendencies of Clay Minerals in Saline Waters1. Clays and Clay Minerals 7, 1–79. doi:10.1346/ccmn.1958.0070102
Williams, A. B. (1957). Studies of Shear Strength and Bearing Capacity of Some Partially Saturated Sands[J]. Int. Soc. Soil Mech. Geotechnical Eng. 2, 453–456.
Keywords: sulfate, aggregation, Atterberg limits, microstructure, macroscopic characterization
Citation: Gu L, Zhang J, Guo L and Lv Q (2022) Effect of Sulfate on the Aggregation of Clay Particles in Loess. Front. Earth Sci. 10:790882. doi: 10.3389/feart.2022.790882
Received: 07 October 2021; Accepted: 14 March 2022;
Published: 26 April 2022.
Edited by:
Gonghui Wang, Kyoto University, JapanReviewed by:
Junhui Zhang, Changsha University of Science and Technology, ChinaXiangjun Pei, Chengdu University of Technology, China
Copyright © 2022 Gu, Zhang, Guo and Lv. This is an open-access article distributed under the terms of the Creative Commons Attribution License (CC BY). The use, distribution or reproduction in other forums is permitted, provided the original author(s) and the copyright owner(s) are credited and that the original publication in this journal is cited, in accordance with accepted academic practice. No use, distribution or reproduction is permitted which does not comply with these terms.
*Correspondence: Qingfeng Lv, lvqf@lzu.edu.cn