- 1Chinese Academy of Geological Sciences, Beijing, China
- 2Technology Innovation Center of Geothermal and Hot Dry Rock Exploration and Development, Ministry of Natural Resources, Beijing, China
- 3Shandong Provincial Bureau of Geology and Mineral Resources, Jinan, China
- 4School of Water Resources and Environment, China University of Geosciences (Beijing), Beijing, China
Studying the flow path of geothermal water in geothermal fields is an important basis for the scientific development and management of geothermal resources. However, due to the influence of engineering volume, economic benefits and demand, it is very difficult to fully grasp the flow path of deep geothermal water in large areas. The use of hydrogeochemical tracers to determine the flow path of geothermal water is a scientific and effective research method. In order to accurately describe the flow path of carbonate geothermal water in the Xiong’an New Area and its surrounding areas, this study systematically analyzed the 14C, 18O, and 2H data of carbonate and Cenozoic sandstone geothermal water, Quaternary groundwater, as well as mountain spring water of the study area. Based on these results, we can conclude that 1) The 14C, d, and 18O values are favourable clues for indicating the circulating runoff conditions and runoff paths of geothermal water. 2) The salinity of the Xushui-Wenan and Lilan transfer zone are <3 g/l, the 14C values are lower than 25000 years, the d value is higher, and the phenomenon of “18O drift” is weak, all these indicating the dominant flow path of carbonate geothermal water, featured by relative lower temperature. 3) The salinity of karst geothermal water in the southwest of Gaoyang low uplift, Rongcheng uplift and Niutuozhen uplift is > 3 g/l, the 14C values are higher than 40,000 years, the d value is lower, and the phenomenon of “18O drift” is obvious, all these indicating that the tectonic sites are the convergence areas of regional karst geothermal water, featured by relative high temperature. 4) The hydrochemical indicators combined with previous carbonate groundwater flow field indicate that the recharge of carbonate geothermal water in the Xiong’an New Area may not only drive from the western Taihang Mountains, but also along the Xushui-Wenan transfer zone and the Lilan fault, with the recharge of carbonate groundwater from the Cangxian uplift from east to west. By further identifying and defining the dominant flow path and supply direction of carbonate geothermal water in the Xiong’an New Area and surrounding areas, it provides strong support for the scientific development of regional carbonate geothermal water.
Introduction
At present, the studies on flow path of underground water are mainly focused on shallow depth by tracer agent and/or hydrogeochemistry (Hu et al., 2019; Wang et al., 2020). Based on these methods, the recharge, runoff, and discharge of underground water are revealed (Solder et al., 2020; Smerdon and Gardner 2022). Meanwhile, determining the flow path of geothermal water is also important for revealing the genesis of geothermal fields. It is a prerequisite for the scientific development and management of geothermal resources. However, it is difficult for a deep thermal reservoir to establish a large-scale systematic pressure monitoring network to obtained the flow path. Carbonate geothermal reservoir is a main type for that of North China (Chen, 1988). The geothermal water in the carbonate reservoir of North China are charactered by obvious hydrogeochemical zoning, which has positive response characteristics and important indicators for the dynamic zoning of geothermal water (Zhang et al., 2015). Hence, it is reasonable to use the difference in hydrogeochemical characteristics of geothermal water with the help of geological conditions such as the documented flow field of geothermal water and geotectonics to accurately determine the flow path of geothermal water. This helps to clarify the origin and formation mechanism of groundwater. Since Craig (1961) first determined the hydrogen and oxygen isotope standard and proposed the δD-18O linear equation for atmospheric precipitation to study the origin and formation of groundwater, researchers have used hydrogen and oxygen isotopes to study groundwater (Rets et al., 2017), the origin of geothermal water and the influencing factors of its formation (Ma et al., 2008; Zhang et al., 2010; Chatterjee et al., 2019). Great progress has been made using the main ions and stable isotopes of geothermal water to evaluate its flow path (Sun et al., 2016; Avşar and Altuntaş, 2017; Mcgibbon et al., 2018) and the potential of geothermal resources (Luo et al., 2017). 14C is suitable for studying the migration mechanism of deep geothermal water as it has a half-life of 5730 ± 40 a (Wang and Zhu, 2001). It has provided great application effects in terms of age, composition, and flow direction of geothermal water (Wang et al., 2013; Hao et al., 2020).
The Niutuozhen and Rongcheng large-scale geothermal fields in Xiong’an New Area have a long history of development due to the shallow thermal reservoir depth of carbonate rocks (Chen et al., 1982). These are well-known carbonate geothermal fields in China. Previous studies on the detection of deep bedrock thermal reservoirs and new formations in this area have made great progress (Wang et al., 2018; Wu et al., 2018). In particular, the Chinese Academy of Geological Sciences has made progress in exploration of deep carbonate geothermal resources in the Gaoyang low uplift of Xiong’an New Area. In 2020, it discovered the highest temperature geothermal well in the North China Basin with a wellhead temperature of 123.4°C (Wang et al., 2021). With regard to the mechanism of the bedrock uplift (buried hill type) geothermal field in the North China Basin, it is thought that the geothermal anomaly is a result of the redistribution of the relatively uniform heat flow from the deep crust to the surface during the upward conduction process (Chen, 1988; Chen, 1992). Pang et al. (2017) established a “dual heat accumulation” model of the Niutuozhen geothermal field, and noted that the abundant geothermal resources in Xiong’an New Area are formed under the dual mechanism of “rock thermal conductivity” and “basin-scale groundwater circulation.” Wang et al. (2021) found that the carbonate geothermal water flow field influences the buried hill geothermal field in the Xiong’an New Area. Previous studies have concluded that carbonate geothermal water in Xiongan New Area drives from the western Taihang Mountains. After infiltration of these water, it migrated from west to east along the deep carbonate strata. Then after heated by deep circulation, the water upwelled along the deep and large faults and discharged into the Niutuozhen uplift and Rongcheng uplift in Xiong’an New Area. For areas besides the Taihang Mountains, there is no clear understanding of whether the carbonate geothermal water in the Xiong’an New Area comes from other sources. This need established through the research on the flow path of the carbonate geothermal water in the Xiongan New Area and surrounding areas.
In this study, we tested and collected 14C dating and δD-δ18O isotopic data in and around Xiong’an New Area. We also acquired other data such as the regional geological structure and carbonate geothermal water flow field. These data allowed us to clarify the Xiong’an New Area and its surrounding carbonate geothermal water flow path. This research provides geological support for the scientific and rational development and utilization of regional carbonate geothermal water.
Geothermal Geological Background
Geological Structure
The Xiong’an New Area is located in the central and western part of the Jizhong Depression in the Bohai Bay Basin. The Jizhong Depression is mainly composed of secondary tilting blocks, skip-shaped depressions, and buried hill structural units formed by a series of normal faults or reverse normal faults under Mesozoic extrusion and Cenozoic extension. The Rongcheng Uplift, Niutuozhen Uplift, Gaoyang Low Uplift, and Lixian Slope involved in Xiong’an New Area are located in the central uplift belt of the Jizhong Depression, while the Xushui Depression and Baoding Depression are located in the western depression belt, and the Bazhou Depression and Raoyang Depression are located in the eastern depression belt (Figure 1). In addition, there is the NWW-SEE-trending Xushui-Wenan structural transformation belt in the middle of the Jizhong Depression, which is the first-level structural transformation belt in the Jizhong Depression. This structural transformation belt divides the Jizhong Depression into a southern area and a northern area, and hence, the adjacent depressions on both sides are different in structural style and deformation strength.
Stratum and Major Thermal Reservoir
From bottom to top, the main strata in the study area are Archean, Great Wall System, Jixian System, Neogene, Quaternary, and Paleogene in depressions and low uplifts. The main thermal reservoir in the study area is the Wumishan Formation of the Jixian System, followed by the Gaozhuang Formation, and between them is a thin layer of Yangzhuang Formation.
(1) Geothermal resources in the Wumishan formation of Jixian system
The geothermal resources of the Wuzhishan Formation are mainly composed of dolomite and are widely distributed in Xiong’an New Area. The buried depth of the Wuzhishan Formation in the Rongcheng Uplift ranges from 600 to 2400 m, and from 530 to 2400 m in the Niutuozhen Uplift ranges. At the top of the Gaoyang Low Uplift and along the Yanling Buried Hills in the northern part of the Lixian Slope, the top buried depth ranges from 3000 to 3500 m and the deep top buried depth ranges from 3500 to 4000 m or deeper. The thickness of the geothermal resources of the Wuzhishan Formation is approximately 1000–1400 m. That is slightly thinner at the axis of the Rongcheng Uplift and relatively thicker around it, with an overall relatively uniform distribution and an average value of 19% for the reservoir-thickness ratio. The porosity of the rocks is generally less than 6.0%, with a maximum porosity of 22.4% and an average porosity of 3.39%. The permeability distribution ranges from 0.01 to 1000 mD and above, is mainly between 0.01 and 100 mD (87.8%) (Dai et al., 2019).
(2) The geothermal resources in the Gaoyuzhuang formation of Jixian system
Surrounding the study area, a few wells have been drilled into the Gaoyuzhuang Formation of the Jixian System. The top depth of the exposed geothermal resources of the Gaoxiangzhuang Formation ranges from 1425 to 3600 m, with a thickness generally greater than 1000 m. The lithology consists mainly of grey dolomite intercalated with muddy dolomite and siliceous dolomite, containing flint agglomerates or bands. The porosity is 2–6% and the permeability is 0.1–160 mD. The formation temperature is generally 75–95°C. The water yield is approximately 45–80 m3/h and TDS is approximately 3000 mg/L.
The burial depth of the Jixian System in the subduction zone of the Xushui-Wenan tectonic transformation belt in the study area is 2500–3500 m. The burial depth of the bedrock in the depressions is mostly above 4000 m and can reach approximately 5000 m outside the study area. The maximum burial depth of the bedrock in the Raoyang Depression is over 6500 m, and the maximum burial depth of the bedrock in the Baxian Depression is over 10000 m. The Xushui-Wen’an tectonic transformation belt is an indicator of fluid (oil and gas) transport in the Raoyang and Baxian Depressions on both sides and contributes to fluid (hydrocarbon) accumulation.
Sample Collection and Testing
Sample Collection
In this study, 92 samples of 14C, D (deuterium), and 18O isotopes were taken in and around Xiong’an New Area (Figure 2), including 33 carbonate geothermal waters in the Jixian System, 52 sandstone geothermal waters in the Neogene, 3 bedrock spring waters in Taihang Mountain, and 4 samples of Quaternary shallow groundwater. In addition, 30 previous test results of 14C, D, and 18O isotopes were collected, including 6 geothermal waters from Neogene sandstone and 24 geothermal waters from carbonate rock.
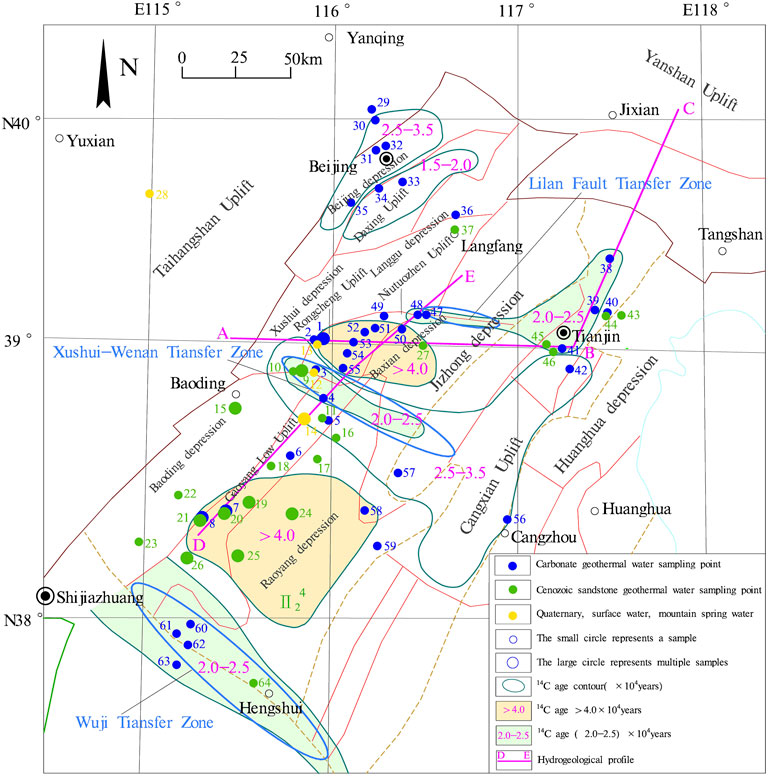
FIGURE 2. Samples of geothermal water isotopes and isotope dating contours of carbonate geothermal water in the study area.
Test Method for Samples
The isotope test analysis was done by Beta Analytic Inc. of the United States, and the results were obtained on Dissolved Inorganic Carbon (DIC). The DIC extraction process was to inject sample water into an acid bath attached to the evacuated collection line. This procedure reduced the pH to <1, and the evolved CO2 was dried with methanol slush and collected in liquid nitrogen. CO2 was then graphitized on the cobalt as a target for Accelerator Mass Spectrometry (AMS) manufacturing in a hydrogen atmosphere. The results were obtained on the DIC and are reported both as a fraction of modern (F14C). Reported radiocarbon results are relative to NIST SRM-4990C. Reported deuterium and oxygen isotopes (δD and δ18O) are reported relative to VSMOW. Measurements were performed using gas-bench Isotope Ratio Mass Spectrometry (IRMS).
Correction of 14C Age for Geothermal Water
14C dating is one of the most important and reliable methods for groundwater dating, and is widely used for groundwater with age changed from 1 to 35 ka. Since geothermal water contain dissolved inorganic carbon and deep inorganic “dead carbon”, showing totally different features with groundwater. It is necessary to correct the apparent 14C age obtained by test. Based on previous experience in 14C age correction of groundwater in North China, methods of chemical dilution correction model based on chemical balance (Tarmers model) and an isotope mixing model based on 14C mass balance (Pearson model) were applied in this study (Ingerson and Person, 1964; Tarmers, 1967). According to the corrected results, the result of “Pearson model” has a larger error, while that of the “Tarmers model” is close to the apparent age of 14C. Therefore, the 14C age calculated by the “Tarmers model” are adopted (Supplementary Table S1). The 14C apparent age is directly used for the mountain spring water and shallow groundwater. As previous geothermal water, if there are measured data of δ13C and 14C, use the “Pearson model” to recalibrate, and then use statistical methods, and use the original “Pearson model” to correct the results without measured data. The average proportional relationship between the results of geothermal water “Tarmers model” and “Pearson model” of Niutuozhen geothermal water, divided by “Pearson model” correction result by 0.77 as “Pearson model” correction age; if it cannot be corrected, 14C apparent age is directly used.
Result
Flow Conditions of Geothermal Water
Figure 2 and Supplementary Table S1 show that the area of the carbonate geothermal water with great 14C age in the Jizhong Depression and its surroundings is located in the center of Gaoyang Low Uplift, Rongcheng Uplift, and segment of Niutuozhen uplift. There areas are featured with the 14C age>40000 years. This may be caused by the relatively closed hydrodynamic conditions insides the bugle as the Rongcheng uplift and the Niutuozhen uplift are surrounded by the thicker depressions in the Cenozoic, while the long Gaoyang and low uplifts are bounded by the Baoding and Raoyang depressions. The 14C age of carbonate geothermal water in Cangxian uplift is mostly lower than 25000 years, and as the distance from the Yanshan Uplift increases from north to south, the 14C age of carbonate geothermal water gradually increases. This is because although the Cangxian uplift is closer to the interior of the Bohai Bay Basin, there is no deep Cenozoic sag between the north of the Cangxian uplift and the Yanshan groundwater recharge area, so that the groundwater in the Yanshan recharge area can follow the karst-developed Cangxian uplift. The long drive from north to south makes the 14C age of carbonate geothermal water in the Cangxian uplift at a relatively low level as a whole. Although the Daxing Uplift in Beijing is farther from the mountainous area than the Beijing Depression, its 14C age of carbonate geothermal water (approximately 20000 years) is smaller than that of the Beijing Depression (>30000 years). This shows that the recharge conditions of carbonate geothermal water in the uplift area are better than those in the depression area. The 14C age of the carbonate geothermal water in the Fengheying Low Uplift in Beijing is 33300 years (sample No. 36), indicating that the low uplift carbonate geothermal water with a relatively sealed hydrogeological environment is also poorly recharged.
Regarding the uplift area, the geothermal water age of carbonate rocks near the deep and large faults of the edge of the uplift is generally younger than that of the interior segment. For example, the 14C age of the carbonate geothermal water at point 40 near the fault on the eastern margin of the Cangxian uplift is 8000 years, which is much less than the 14C age (24000 years) at point 39, that is farther from the marginal fault. The geothermal water circular and alternate conditions in the deep fault belt appear to be better than those in the inner part of the uplift where faults are not developed.
As showed in Figure 2, the 14C values of carbonate geothermal water in Xushui-wenan, Lilan, and Wuji transfer zones are relatively lower (20000–25000 years). The 14C ages of the carbonate geothermal water at point 47 and 48 near the Lilan transfer zone in the northeastern edge of Niutuozhen Uplift range from 16200 to 22400 years, which is significantly less than that of the carbonate geothermal water in the southwestern Niutuozhen Uplift and Rongcheng Uplift (>40000 years). The 14C age of geothermal water from northeast to southwest increases gradually, indicating that carbonate geothermal water may flow from northeast to southwest. The 14C age of carbonate geothermal water in the Xushui-Wenan structural transformation belt between the Rongcheng Uplift-Niutuozhen Uplift and the Gaoyang Low Uplift is relatively low (23800–24800), obvious lower than the uplift segment of north and south, indicating that the circular and alternate conditions of geothermal water in the Wenan structural transformation belt with developed faulted structures are relatively great. It also shows that it may be the dominant flow channel for regional karst geothermal water. The 14C age of carbonate geothermal water in the Wuji structural transformation zone is 15700–22900 years, which is significantly lower than the that in the northern Gaoyang low uplift. This shows that the fault structure in the structural transformation zone in the region is dense, the fractured karst is relatively developed, and the circulation and alternating conditions of geothermal water are relatively good, which is the dominant channel for the flow of regional karst geothermal water, so the groundwater age is relatively low. Compared with the age of the geothermal water in the upper Cenozoic, carbonate geothermal water presents, different characteristics are showed in different structural parts. For example, the Neogene Guantao Formation geothermal water in the Xushui-Anxin structural belt is generally 30000–40000 years old, which is 0.5–15000 years older than the carbonate geothermal water in the lower part. The carbonate rock geothermal water at point 41, located in the southwest of Tianjin, is 6000 to 15000 years younger than the Neogene Guantao Formation geothermal water above it. The carbonate rock geothermal water in the Gaoyang low uplift and the Neogene Guantao Formation geothermal water in the upper part are featured by the same age, about 40000 years old. This is also in the area with relatively good circulation conditions, which is the dominant channel for the flow of regional karst geothermal water.
D-18O Isotopic Characteristics and Indication for Geothermal Water Flow Conditions
Figure 3 shows the δD and δ18O plots of regional surface water, Quaternary shallow groundwater, and Taihang Mountain spring water. Obviously, all points located near the global meteoric water line (δD = 8δ18O + 10; Craig, 1961), Tianjin meteoric water line (δD = 7.7δ18O + 8.7; Deng et al., 2012), and northern Shandong meteoric water line (δD = 7.5δ18O + 5.4; Yang et al., 2009), and slightly drifting to the right. This indicates that the recharge source of regional shallow groundwater is meteoric water and is affected by evaporation to a certain extent.
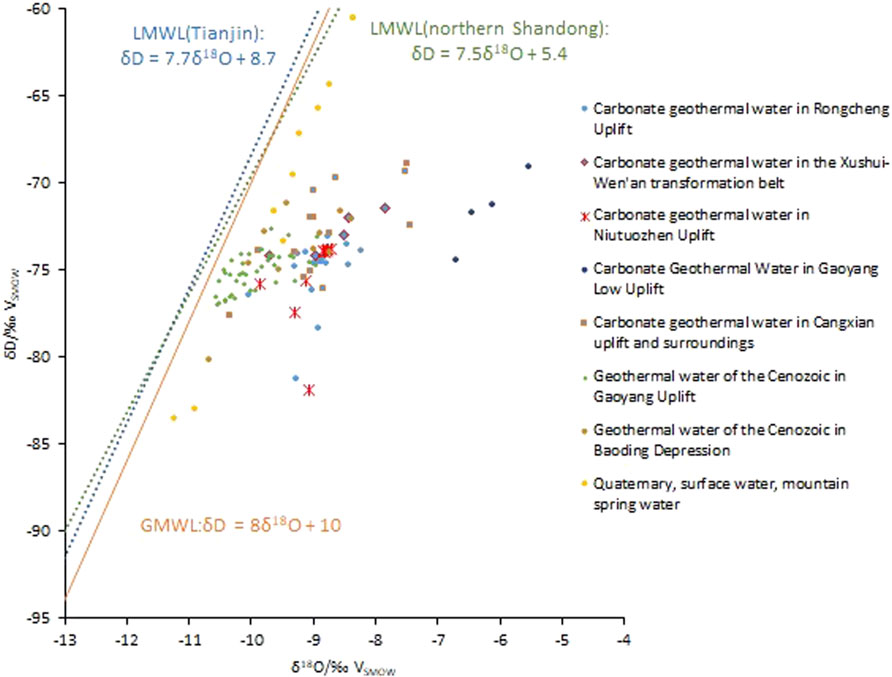
FIGURE 3. Characteristics and relationship of δD-δ18O of geothermal water in Xiong’an New Area and its surroundings.
The Cenozoic geothermal water in the Gaoyang Low Uplift is relatively close to the meteoric water line because its thermal reservoir is mainly the shallow buried Neogene Guantao Formation. The Cenozoic geothermal water in the Baoding Depression, which close to the Taihang Mountain recharge area, is farther away from the meteoric water line. This is because the thermal reservoir temperature of the Guantao Formation in Baoding Depression is relatively low, and part of the deep thermal reservoir of Paleogene Dongying Formation and Shahejie Formation is used. This also indicates that the deeper the geothermal water is, the older it is.
As a result of water–rock interaction, the values of 18O in water often increases, which is called “18O drift” The degree of 18O drift can reflect the residence time and metamorphic degree of water in the aquifer. As shown in Figure 3, compared with Cenozoic geothermal water, the “18O drift” phenomenon of carbonate geothermal water is more obvious. The temperature of carbonate rock geothermal reservoir in the study area is lower than 200°C, and no obvious oxygen isotope fractionation can be produced. This may be caused by isotopic exchange between carbonate geothermal water and oxygen-bearing host rocks (carbonate or silicate) over a long geological history. The 18O drift phenomenon of carbonate geothermal water in Rongcheng, Niutuozhen, and Gaoyang Uplift is more obvious than that in Cangxian Uplift, while the 18O drift phenomenon of carbonate geothermal water in the northern Cangxian Uplift is more obvious than that in the southern Cangxian Uplift. This indicates that the farther away from the recharge area, the worse the circular and alternate conditions and the more obvious the 18O drift phenomenon. There is no deep depression in the northern Cangxian Uplift, which can directly receive the mountainous recharge, and the circular and alternate conditions are great. Therefore, the phenomenon of 18O drift of the carbonate geothermal water in the northern Cangxian Uplift is not obvious. Similarly, the “18O drift” phenomenon of carbonate geothermal water in the Xushui-Wenan transfer zone is obviously weaker than that in the Niutuozhen uplift and the Gaoyang low uplift, indicating that the carbonate thermal storage occurs in this structural transformation zone. The circulation and alternating conditions of geothermal water are better.
To facilitate the comparison of the difference in meteoric water in different regions, Dansgaard (1964) proposed the concept of the deuterium excess parameter (d) and defined it as d = δD − 8δ18O. The d values are equivalent to the intercept values when the slope of meteoric water (δD/δ18O) in the area is 8. An important feature of the d parameter is that it is not affected by seasons, altitudes, and other factors in the same area, and is only directly related to the length of its residence time in the aquifer (Yin et al., 2001). It is therefore suitable for studying the circular and alternate conditions and deterioration degree of geothermal water. Parameter d can be used as a measure of the degree of 18O isotope exchange in the water-rock interactions. The smaller the values of d, the more sealed the hydrogeological environment, the slower the groundwater runoff speed, the longer the water residence time in the aquifer, and the higher the degree of water metamorphism.
The d values of carbonate geothermal water in the southern Cangxian Uplift and Xushui-wenan transfer zone are higher, whereas those of Rongcheng and Niutuozhen Uplift are relatively low (0 to -10), except the higher d values of carbonate geothermal water in the northern part of Cangxian uplift (Figure 4). In addition, the phenomenon of 18O drift is relatively apparent, indicating that the carbonate geothermal water in these areas has poor circular and alternate conditions, long residence time in the aquifer, and a strong metamorphic degree. The parameter d of the Gaoyang Uplift is the smallest and the 18O drift is the most distinct, indicating that the geothermal water in the Gaoyang Uplift has circular and alternate conditions, long residence time in the aquifer, and a strong metamorphic degree. At the same time, the 18O drift is distinct due to the large buried depth of the carbonate reservoir, high temperature of the water, and obvious 18O exchange between water and rock under the high-temperature conditions in the Gaoyang Uplift (Craig, 1966). This is consistent with the occurrence of geothermal water indicated by the 14C age and the δD-δ18O characteristics of geothermal water. That is the high d values of geothermal water in the northern Cangxian uplift and the Xushui-Wenan transition zone are the structural parts with better conditions for the alternation of regional carbonate geothermal water circulation.
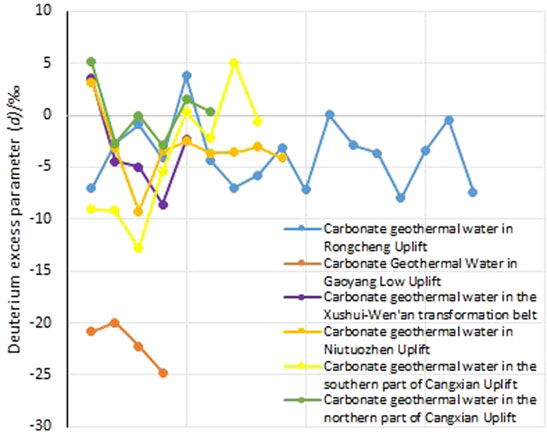
FIGURE 4. Characteristics of deuterium excess parameter (d) in Xiong’an New Area and surrounding carbonate geothermal water.
Discussion
Decades of geothermal water exploitation history and the influence of oilfield fluid exploitation as well as injection activities have caused significant changes in the basement geothermal water hydrodynamic field of the Xiong’an New Area. The current geothermal water hydrodynamic field no longer reflects the bedrock geothermal water recharge, runoff, and drainage conditions present in the natural state. Therefore, this study used the earlier bedrock geothermal water hydrodynamic field characteristics (Yan and Yu, 2000) to illustrate the bedrock geothermal water recharge, runoff, and drainage conditions in the study area.
The bedrock geothermal water in the Jizhong Depression is mainly recharged by Taihang Mountains in the west and Yanshan Mountains in the north (Figure 5). The groundwater level of the bedrock in the Xushui-Wenan structural transformation belt is slightly higher than that of the uplift areas on both of the north and south sides. This may be due to a series of NWW-SEE faults in the Xushui-Wenan structural transformation belt connecting the piedmont faults in the eastern foothills of the Taihang Mountains, making it easier to recharge groundwater from the piedmont of the Taihang Mountains along the Xushui-Wenan structural transformation belt. The Cangxian Uplift is connected to the Yanshan Mountain towards the northeast. The relatively shallow buried carbonate thermal reservoir developed many karst fissures and the recharge conditions are better. As a result, the groundwater level of the bedrock of the Cangxian Uplift is significantly higher than that of the Jizhong Depression on the west, which enables the bedrock groundwater of the Cangxian Uplift to flow westward.
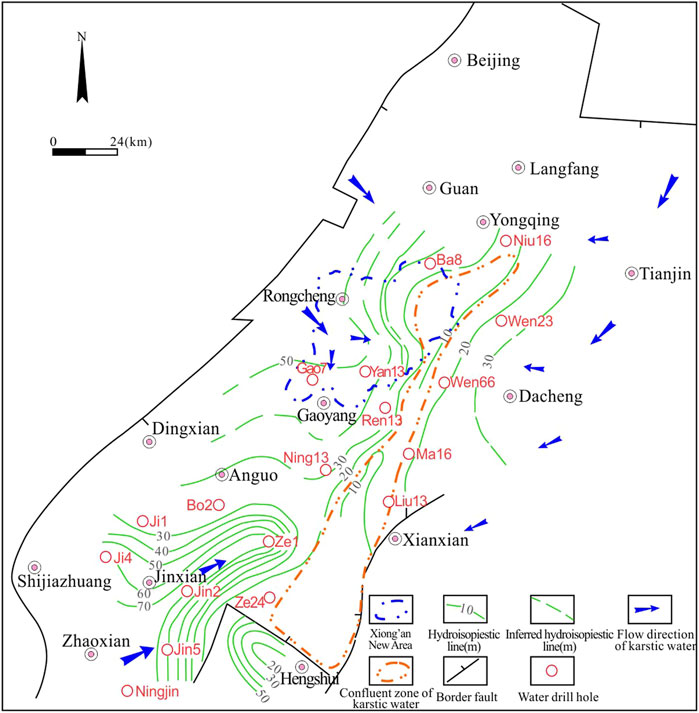
FIGURE 5. Contour map of piezometric level of buried hill in the Jizhong Depression [according to Yan and Yu (2000)].
A large number of 14C, D (deuterium), and 18O isotopes of carbonate geothermal water around Xiong’an New Area were tested. This compensated for the lack of distribution and quantity of carbonate geothermal wells and the unclear groundwater flow field modified by the disturbances caused by human exploitation. However, there are rare understand on the flow path of geothermal water. Local artificial exploitation definitely has not fundamentally changed the flow field morphology of regional karst geothermal water. Using the good indicators of 14C, D, and 18O isotopes, the flow path of karst geothermal water in Xiong’an New Area and its surroundings can be accurately inferred.
The karst geothermal water in Xiong’an New Area and its surroundings is mainly recharged by the Taihang Mountains in the west and the Yanshan Mountains in the north. Figure 5 shows that the recharge mainly comes from the northwest, west, southwest, and northeast. However, since the maximum thickness of the Cenozoic in the Baxian Depression, Raoyang Depression, and Baoding Depression on both sides of the uplift of Niutuozhen, Rongcheng, and Gaoyang can reach 6000–10000 m, it is difficult to charge karst geothermal water in shallow buried uplift areas for carbonate groundwater deeply buried under the great thickness of the Cenozoic. The most likely recharge path is the relatively shallow buried area of the bedrock at the edge of the depression. The Xushui-Wenan structural transformation belt, which is located between The Xushui Depression-Rongcheng Uplift-Niutuozhen Uplift-Baxian Depression-Wen’an Slope and Baoding Depression-Gaoyang Uplift-Raoyang Depression, is characterized by dense structural development and a relatively shallow bedrock burial depth (approximately 4000–5000 m). The buried depth of bedrock is 1000–3000 m, which is shallower than that of the depression on both sides. Karst is still relatively developed in the carbonate strata at this depth, which can form the dominant flow channel of karst geothermal water. The karst hydraulic pressure and deuterium excess parameter d values in the Xushui-Wenan structural transformation belt between Rongcheng and Gaoyang is significantly higher than that of the uplift areas on both sides (Figures 6, 7, Supplementary Table S1). The 14C age and salinity of geothermal water are significantly lower than those of the bulge areas on both sides. According to the carbonate geothermal wells constructed in the past 2 years, the water temperature of the D19 and D21 geothermal wells located in the Xushui-Wenan structural transformation belt is significantly lower than that of the D34 and D35 geothermal wells with similar well depths outside the Xushui-Wenan structural transformation belt (about 30–40°C lower). This indicates that the Xushui-Wenan structural transformation belt is the dominant flow channel of regional karst geothermal water, which is conducive to the recharge of karst water from the Taihang mountains in the west and can receive the recharge from the Cangxian uplift in the east. Therefore, geothermal water has a low degree of metamorphism, low 14C age and salinity, and relatively low temperature.
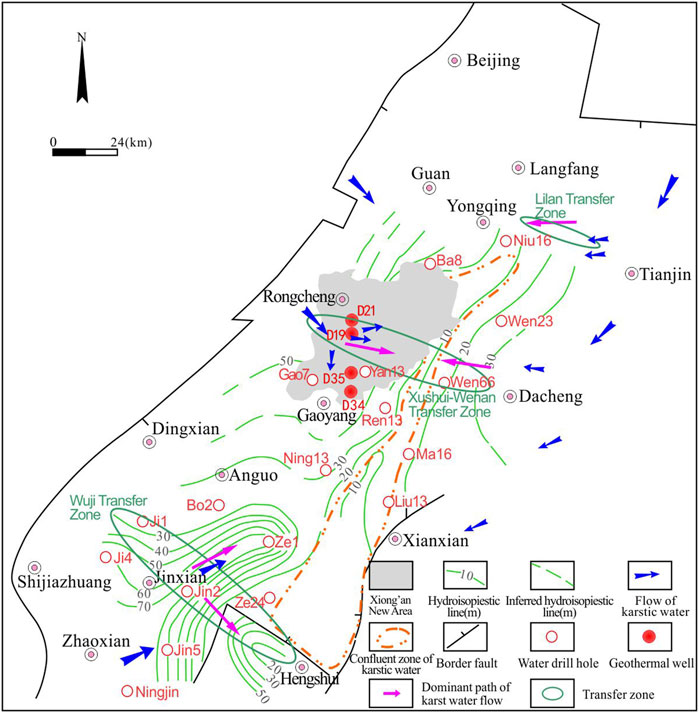
FIGURE 6. The flow path identification diagram of karst geothermal water in Xiong’an New Area and its surroundings.
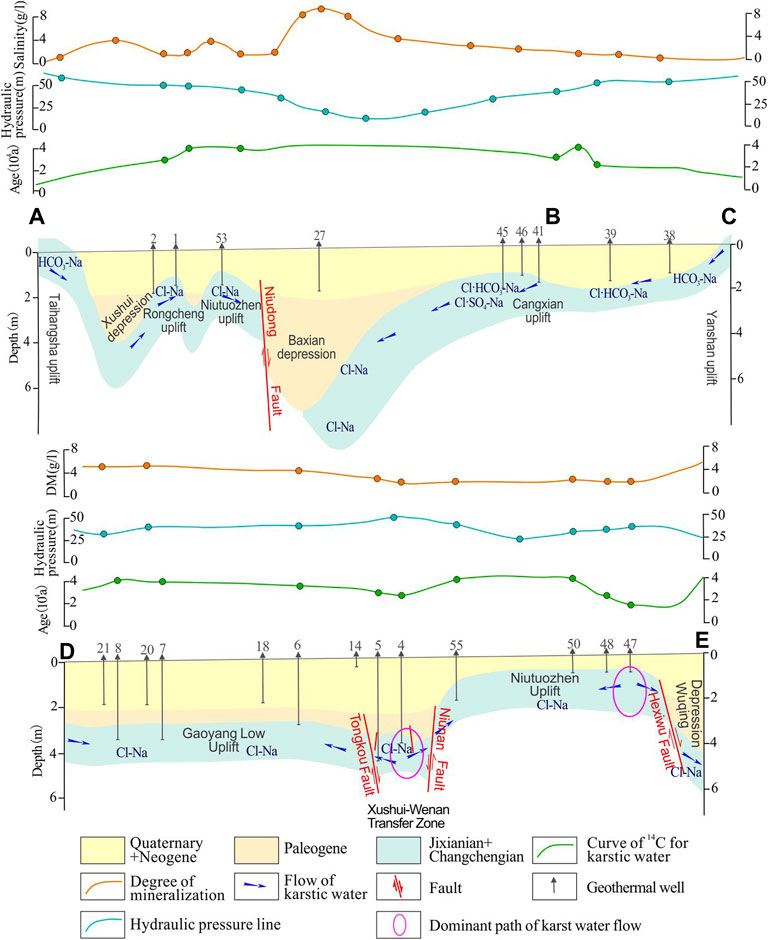
FIGURE 7. Geological cross-section along section line (A–E) on the map of Figure 2 of the study area.
In addition, a small structural transformation belt also developed between the Wuqing Depression-Yangcun Slope and Baxian Depression-Wenan Slope. This structural transformation belt is distributed along the NWW-trending Lilan fault (Yao, 2014), and the bedrock burial depth is relatively shallow (approximately 4000–5000 m) (Wang, 2020). Karst is well developed in the carbonate strata, and it has conditions allowing it to form the dominant flow channel of karst geothermal water. The 14C ages of the carbonate geothermal water at point 47 and 48 in the northeast of Niutuozhen uplift are 16200–22400 years old, which is significantly younger than the 14C ages of the carbonate geothermal water in the southwest of the Niutuozhen Uplift and Rongcheng Uplift (>40000 years). However, the d values are significantly higher than the latter. This indicates that the karst geothermal water may flow from northeast to southwest. The Lilan transfer zone between Wuqing Depression-Yangcun Slope and Baxian Depression-Wenan Slope may be the dominant flow channel of another regional karst geothermal water recharge from Cangxian uplift to the west. The 14C age of carbonate geothermal water in the northern Cangxian uplift is relatively low, the hydrochemical type is Cl·HCO3-Na or Cl·SO4-Na type, and the salinity is low, appearing characteristics of closer to the recharge area (Figure 7).
Conclusion
The 14C age, deuterium excess parameter (d), and the 18O drift phenomenon are good indicators of the circulating runoff conditions and runoff path of geothermal water. Geothermal water flow conditions are good in sections with low 14C age and salinity, high d values, and weak 18O drift phenomenon, corresponding to the dominant recharge channel for carbonate geothermal water.
The 14C dating, 18O, and 2H data of carbonate geothermal water, Cenozoic sandstone geothermal water, quaternary groundwater, and mountain springs in Xiongan New Area and surroundings and the analysis of regional carbonate groundwater flow field and structural conditions, indicated that the dominant flow paths and main recharge channels of regional carbonate geothermal water are as follows. 1) The karst geothermal water in the Xiong’an New Area and its surroundings is mainly recharged by the Taihang Mountains in the west and the Yanshan Mountains in the north. The Xushui-Wenan structural transformation belt is the dominant flow channel for the regional karst geothermal water, which is conducive to the recharge of karst water from the Taihang Mountains in the west and also receives recharge from the Cangxian uplift in the east. 2) The northeastern part of the Niutuozhen Uplift may receive the recharge of carbonate geothermal water from Cangxian uplift in the east through the Lilan transfer zone. 3) For the southwest of Gaoyang low Uplift, Rongcheng and Niutuozhen Uplift, the 14C age >40000, the d is low, and the phenomenon of “18O drift” is obvious, indicating that these structural parts are the convergence areas of regional karst geothermal water.
Through the identification and definition of the dominant flow path and recharge direction of carbonate geothermal water in Xiong’an New Area and its surrounding areas, a clearer basis for discriminating the flow field and temperature of carbonate geothermal water is obtained. All these studies provide geothermal geology for scientific development carbonate geothermal energy.
The 14C values of carbonate geothermal water in the southwest of Gaoyang low uplift, Rongcheng uplift, and Niutuozhen uplift is lower than 0.44 pmc, and the 14C age of carbonate rock geothermal water can only be determined to be > 40000 years old, which cannot be accurately determined. Therefore, when estimating the age of groundwater >40000 years, groundwater dating methods with a large dating range such as 36Cl and 3He-4He should be used.
Data Availability Statement
The original contributions presented in the study are included in the article/Supplementary Material, further inquiries can be directed to the corresponding authors.
Author Contributions
BZ wrote the manuscript with the support of the list authors. All authors have made a substantial, direct, and intellectual contribution to the manuscript.
Funding
This research was financially supported by National Natural Science Foundation of China (Nos 41902310, U1906209, and 42072331), National Key Research and Development Projects of China (2019YFB1504102) and Project of China Geological Survey (Nos DD20189114 and DD20190129).
Conflict of Interest
The authors declare that the research was conducted in the absence of any commercial or financial relationships that could be construed as a potential conflict of interest.
Publisher’s Note
All claims expressed in this article are solely those of the authors and do not necessarily represent those of their affiliated organizations, or those of the publisher, the editors and the reviewers. Any product that may be evaluated in this article, or claim that may be made by its manufacturer, is not guaranteed or endorsed by the publisher.
Acknowledgments
The editor, associate editor, and reviewers are thanked for their constructive comments that greatly improved the paper.
Supplementary Material
The Supplementary Material for this article can be found online at: https://www.frontiersin.org/articles/10.3389/feart.2022.782273/full#supplementary-material
References
Avşar, Ö., and Altuntaş, G. (2017). Hydrogeochemical Evaluation of Umut Geothermal Field (SW Turkey). Environ. Earth Sci. 76, 582. doi:10.1007/s12665-017-6929-6
Chatterjee, S., Sinha, U. K., Biswal, B. P., Jaryal, A., Jain, P. K., Patbhaje, S., et al. (2019). An Integrated Isotope-Geochemical Approach to Characterize a Medium Enthalpy Geothermal System in India. Aquat. Geochem. 25, 63–89. doi:10.1007/s10498-019-09352-z
Chen, M. X. (1992). Advances of Studies of Geothermal Resources in China. Adv. Earth Sci. 7 (3), 9–14. CNKI:SUN:DXJZ.0.1992-03-001
Chen, M. X., Huang, G. S., Zhang, W. R., Zhang, R. Y., and Liu, B. Y. (1982). The Temperature Distributibution Pattern and the Utilization of Geothermalwater at Niutuozhen Basement Protrusion of Central Hebei Province. Scientia Geologica Sinica 3, 239–252. doi:10.1007/BF01033890
Craig, H. (1966). Isotopic Composition and Origin of the Red Sea and Salton Sea Geothermal Brines. Science 154, 1544–1548. doi:10.1126/science.154.3756.1544
Craig, H. (1961). Isotopic Variations in Meteoric Waters. Science 133, 1702–1703. doi:10.1126/science.133.3465.1702
Dai, M. G., Lei, H. F., Hu, J. G., Guo, X. F., Ma, P. P., and Zhang, J. Y. (2019). Evaluation of Recoverable Geothermal Resources and Development Parameters of Mesoproterozoic thermal Reservoir with the Top Surface Depth of 3500 M and Shallow in Xiong’an New Area. Acta Geologica Sinica 93, 2874–2888. 10.1111/1755-6724.13979. doi:10.3969/j.issn.0001-5717.2019.11.012
Dansgaard, W. (1964). Stable Isotopes in Precipitation. Tellus 16, 436–468. doi:10.3402/tellusa.v16i4.8993
Deng, W. P., Yu, X. X., and Jia, G. D. (2012). Sources and Stable Isotope Characteristics of Precipitation in North China. Bull. Mineralogy, Petrology Geochem. 31 (5), 489–493. doi:10.3969/j.issn.1007-2802.2012.05.007
Hao, Y., Pang, Z., Kong, Y., Tian, J., Wang, Y., Liao, D., et al. (2020). Chemical and Isotopic Constraints on the Origin of saline Waters from a Hot spring in the Eastern Coastal Area of China. Hydrogeol J. 28, 2457–2475. doi:10.1007/s10040-020-02199-7
Hu, Y., Ma, R., Wang, Y., Chang, Q., Wang, S., Ge, M., et al. (2019). Using Hydrogeochemical Data to Trace Groundwater Flow Paths in a Cold alpine Catchment. Hydrological Process. 33 (14), 1942–1960. doi:10.1002/hyp.13440
Ingerson, E., and Person, F. J. (1964). “Estimation of Age and Rate of Motion of the Groundwater by the C Method,” in Proceedings of Sugawara Festival on Recentresearches in the fields of Atmosphere, Hydrosphere, and Nuclear Geochemisrtry (Tokyo: Maruzen), 237–266.
Ke, B. L. (2009). Characteristics of Geothermal Geology in the Northwestern Part of the Urban Beijing Geothermal Field. Geoscience 23, 49–56. doi:10.3969/j.issn.1000-8527.2009.01.008
Liu, K., Liu, Y. C., Sun, Y., Liu, J. R., and Wang, S. F. (2015a). Characteristics of Deuterium Excess Parameters of Geothermal Water in Beijing. Geology. China 42, 2029–2035. CNKI:SUN:DIZI.0.2015-06-029
Liu, Y. C., Liu, K., Sun, Y., Liu, J. R., and Guo, G. X. (2015b). Hydrochemical Characteristics and Isotopic Analysis of Geothermal Water in Liangxiang Geothermal Field. South-to-North Water Transfers Water Sci. Technology 13, 963–967. doi:10.13476/j.cnki.nsbdqk.2015.05.030
Luo, J., Pang, Z., Kong, Y., and Wang, Y. (2017). Geothermal Potential Evaluation and Development Prioritization Based on Geochemistry of Geothermal Waters from Kangding Area, Western Sichuan, China. Environ. Earth Sci. 76, 343. doi:10.1007/s12665-017-6659-9
Lv, J. B. (2004). Characteristics of the Geothermal Field in Northern Beijing and the Influence of its Exploitation on Seismic Ground Fluid Behavior. China Earthquake Administration: Doctor's thesis of Institute of Geology.
Ma, Z. Y., Wang, X. G., Su, Y., and Yu, J. (2008). Oxygen and Hydrogen Isotope Exchange and its Controlling Factors in Subsurface Geothermal Waters in the central Guanzhong basin, Shaanxi, China. Geol. Bull. China 27, 888–894. doi:10.1016/S1872-5791(08)60056-1
Mcgibbon, C., Crossey, L. J., Karlstrom, K. E., and Grulke, T. (2018). Carbonic Springs as Distal Manifestations of Geothermal Systems, Highlighting the Importance of Fault Pathways and Hydrochemical Mixing: Example from the Jemez Mountains, New Mexico. Appl. Geochem. 98, 45–57. doi:10.1016/j.apgeochem.2018.08.015
Pang, Z. H., Kong, Y. L., Pang, J. M., Hu, S. B., and Wang, J. Y. (2017). Geothermal Resources and Development in Xiongan New Area. Bull. Chin. Acad. Sci. 32 (11), 1224–1230. doi:10.16418/j.issn.1000-3045.2017.11.007
Rets, E., Chizhova, J., Loshakova, N., Tokarev, I., Kireeva, M., Budantseva, N., et al. (2017). Erratum to: Using Isotope Methods to Study alpine Headwater Regions in the Northern Caucasus and Tien Shan. Front. Earth Sci. 11, 702. doi:10.1007/s11707-017-0674-8
Smerdon, B. D., and Gardner, W. P. (2022). Characterizing Groundwater Flow Paths in an Undeveloped Region through Synoptic River Sampling for Environmental Tracers. Hydrological Process. 36 (1), e14464. doi:10.1002/hyp.14464
Solder, J. E., Beisner, K. R., Anderson, J., and Bills, D. J. (2020). Rethinking Groundwater Flow on the South Rim of the Grand Canyon, USA: Characterizing Recharge Sources and Flow Paths with Environmental Tracers. Hydrogeol J. 28 (5), 1593–1613. doi:10.1007/s10040-020-02193-z
Sun, Z., Ma, R., Wang, Y., Ma, T., and Liu, Y. (2016). Using Isotopic, Hydrogeochemical-Tracer and Temperature Data to Characterize Recharge and Flow Paths in a Complex Karst Groundwater Flow System in Northern China. Hydrogeol J. 24, 1393–1412. doi:10.1007/s10040-016-1390-2
Tarmers, M. A. (1967). “Radiocarbon Ages of Groundwater in an Arid Zone Unconfined Aquifer,” in Stout G E Isotope Techniques in the Hydrologic Cycle, Geophysics (Washington D C: AGU), 143–152.
Wang, G. L., Li, J., Wu, A. M., Zhang, W., and Hu, Q. Y. (2018). A Study of the Thermal Storage Characteristics of Gaoyuzhuang Formation, A New Layer System of Thermal Reservoir in Rongcheng Uplift Area, Hebei Province. Acta Geoscientica Sinica 39, 533–541. doi:10.3975/cagsb.2018.071901
Wang, K., and Zhu, J. (2001). A Conceptual Model of the Tianjin Geothermal System Based on Isotopic Studies. Sci. China Ser. E-technol. Sci. 44, 160–164. doi:10.1007/bf02916809
Wang, P., Zhang, F., and Chen, Z. (2020). Characterization of Recharge Processes and Groundwater Flow Paths Using Isotopes in the Arid Santanghu basin, Northwest China. Hydrogeology J. 28 (3), 1–15. doi:10.1007/s10040-020-02119-9
Wang, S., Pang, Z., Liu, J., Lin, P., Liu, S., and Yin, M. (2013). Origin and Evolution Characteristics of Geothermal Water in the Niutuozhen Geothermal Field, North China Plain. J. Earth Sci. 24, 891–902. doi:10.1007/s12583-013-0390-6
Wang, S. Q., Zhang, B. J., Li, Y. Y., Xing, Y. F., Yuan, W. Z., Li, J., et al. (2021). Heat Accumulation Mechanism of Deep Ancient Buried hill in the Northeast of Gaoyang Geothermal Field, Xiong’an New Area. Bull. Geol. Sci. Technology 40, 533–541. doi:10.19509/j.cnki.dzkq.2021.0319
Wang, Y. Z. (2020). Carboniferous-Permian Coal-Derived Gas Resource Potential Analysis and Favorable Area Prediction in the Northeast of Jizhong Depression. Beijing: Doctoral Dissertation of China University of Geosciences.
Wu, A. M., Ma, F., Wang, G. L., Liu, J. X., Hu, Q. Y., and Miao, Q. Z. (2018). A Study of Deep-Seated Karst Geothermal Reservoir Exploration and Huge Capacity Geothermal Well Parameters in Xiongan New Area. Acta Geoscientica Sinica 39, 523–532. doi:10.3975/cagsb.2018.071104
Yan, D. S., and Yu, Y. T. (2000). Evaluation and Utilization of Geothermal Resources in Beijing Tianjin Hebei Oil Region. Wuhan: China University of Geosciences Press, 58–59.
Yang, L. Z., Zhang, G. H., Hu, N. S., Liu, C. H., and Liu, Z. Y. (2009). Recognition of Ground Water Supplying Features for Northern Shandong plain;China Using Environmental Isotope Information. Geol. Bull. China 28, 515–522. doi:10.3969/j.issn.1671-2552.2009.04.013
Yao, J. L. (2014). Analysis of Carboniferous-Permian Tectonic Control of Oil and Gas in the Northeast of Jizhong Depression. Beijing): Master's thesis of China University of Geosciences.
Yao, Z. J. (1995). Paleoclimate Record of Geothermal Water for Last 0.03 Ma in North China. Earth Sci. 20 (4), 383–388. CNKI:SUN:DQKX.0.1995-04-005
Yin, G., Ni, S. J., and Zhang, Q. C. (2001). Euterium Excess Parameter and Geohydrology Significance-Taiking the Geohydrology Researches in Jiuzhaigou and Yele, Sichuan for Example. J. Chengdu Univ. Technology 7 (3), 251–254. doi:10.3969/j.issn.1671-9727.2001.03.006
Zhang, B. J., Gao, Z. J., Zhang, F. Y., Hao, S. H., Liu, F. Y., and Zang, J. J. (2015). Hydrodynamic Condition and Response of Formation Water Chemical fields of Geothermal Water in North China Basin. Earth Sci. Front. 22 (06), 217–226. doi:10.13745/j.esf.2015.06.017
Zhang, B. J., Xu, J. X., Ma, Z. M., Shen, Z. L., and Qi, L. (2010). Analysis on Groundwater Supply Sources Using Hydrogen and Oxygen Isotope Data-A Case Study of Yanggu-Qihe Salient, Northwestern Shandong, China. Geol. Bull. China 29, 603–609. doi:10.3969/j.issn.1671-2552.2010.04.016
Keywords: Xiong’an New Area, carbonate thermal reservoir, 14C dating, D-O isotope, geothermal water flow path, development and utilization
Citation: Zhang B, Wang S, Kang F, Li Y, Zhuo L, Gao J, Yuan W and Xing Y (2022) Flow Path of the Carbonate Geothermal Water in Xiong’an New Area, North China: Constraints From 14C Dating and H-O Isotopes. Front. Earth Sci. 10:782273. doi: 10.3389/feart.2022.782273
Received: 24 September 2021; Accepted: 28 February 2022;
Published: 08 April 2022.
Edited by:
Yinhui Zuo, Chengdu University of Technology, ChinaReviewed by:
Yingchun Wang, Chengdu University of Technology, ChinaYiman Li, Institute of Geology and Geophysics (CAS), China
Copyright © 2022 Zhang, Wang, Kang, Li, Zhuo, Gao, Yuan and Xing. This is an open-access article distributed under the terms of the Creative Commons Attribution License (CC BY). The use, distribution or reproduction in other forums is permitted, provided the original author(s) and the copyright owner(s) are credited and that the original publication in this journal is cited, in accordance with accepted academic practice. No use, distribution or reproduction is permitted which does not comply with these terms.
*Correspondence: Baojian Zhang, zbjsddk@126.com; Siqi Wang, wsiqi17@163.com