- 1Alfred Wegener Institute, Permafrost Research, Helmholtz Centre for Polar and Marine Research, Potsdam, Germany
- 2Eurasia Institute of Earth Sciences, Istanbul Technical University, Istanbul, Turkey
- 3Alfred Wegener Institute, Atmospheric Physics, Helmholtz Centre for Polar and Marine Research, Potsdam, Germany
- 4Melnikov Permafrost Institute, Yakutsk, Russia
We studied heavy and light mineral associations from two grain-size fractions (63–125 μm, 125–250 µm) from 18 permafrost sites in the northern Siberian Arctic in order to differentiate local versus regional source areas of permafrost aggradation on the late Quaternary time scale. The stratigraphic context of the studied profiles spans about 200 ka covering the Marine Isotope Stage (MIS) 7 to MIS 1. Heavy and light mineral grains are mostly angular, subangular or slightly rounded in the studied permafrost sediments. Only grains from sediments with significantly longer transport distances show higher degrees of rounding. Differences in the varying heavy and light mineral associations represent varying sediment sources, frost weathering processes, transport mechanisms, and post-sedimentary soil formation processes of the deposits of distinct cryostratigraphic units. We summarized the results of 1141 microscopic mineral analyses of 486 samples in mean values for the respective cryostratigraphic units. We compared the mineral associations of all 18 sites along the Laptev Sea coast, in the Lena Delta, and on the New Siberian Archipelago to each other and used analysis of variance and cluster analysis to characterize the differences and similarities among mineral associations. The mineral associations of distinct cryostratigraphic units within several studied profiles differ significantly, while others do not. Significant differences between sites as well as between single cryostratigraphic units at an individual site exist in mineral associations, heavy mineral contents, and mineral coefficients. Thus, each study site shows individual, location-specific mineral association. The mineral records originate from multiple locations covering a large spatial range and show that ratios of heavy and light mineral loads remained rather stable over time, including glacial and interglacial periods. This suggests mostly local sediment sources and highlights the importance of sediment reworking under periglacial regimes through time, including for example the formation of MIS 1 thermokarst and thermo-erosional deposits based on remobilized MIS 3 and 2 Yedoma Ice Complex deposits. Based on the diverse mineralogical results our study supports the viewpoint that Yedoma Ice Complex deposits are mainly results of local and polygenetic formations (including local aeolian relocation) superimposed by cryogenic weathering and varying climate conditions rather than exclusive long distance aeolian transport of loess, which would have highly homogenized the deposits across large regions.
Introduction
Periglacial landscapes are widespread in northern high latitudes and are affected by specific cold-region physical weathering, sediment production, transport and deposition (Murton, 2021). Understanding periglacial deposition and associated landscape and permafrost dynamics on long and short time-scales is important for the interpretation of currently ongoing rapid changes in permafrost regions. In particular, changes in air temperatures and precipitation regimes (Box et al., 2019), warming and thawing of permafrost (Biskaborn et al., 2019), and rapid hydrological and geomorphological change (Nitzbon et al., 2020) do all affect cryogenic weathering, sediment transport, and deposition processes. While the cryostratigraphy of permafrost deposits (French & Shur, 2010), and cryolithology (Popov, 1953; Katasonov, 1955; Popov, 1967) have been used for decades to decipher indicators of past and modern periglacial landscape changes, the analysis of mineral associations is still rarely applied for the investigation of sedimentary dynamics of periglacial landscapes and the role of cryogenic weathering (Konishchev and Rogov, 1993).
However, heavy and light mineral associations of sediments are widely used in present-day non-permafrost environments to reconstruct source (provenance) areas and their changes and to identify modifications of sediments after their deposition (e.g., Morton and Hallsworth, 1999; Woronko et al., 2013; Pisarska-Jamroży et al., 2015). Therefore, identifying linkages of mineral associations in sediments to local bedrock, fluvial sources, or far-ranging sources for, e.g., the potential aeolian transport are useful to shed light on permafrost formation and landscape changes in the late Quaternary past.
The origin and genesis of periglacial deposits such as late Pleistocene Yedoma Ice Complex that has been formed during Marine Isotope Stage (MIS) 3 to MIS 2 have been under discussion for decades (for overview see Astakhov, 2013; Murton et al., 2015; Schirrmeister et al., 2013). To foster the understanding of permafrost aggradation and degradation on late Quaternary timescale, we conducted comprehensive paleo-environmental and paleo-landscape reconstructions using the permafrost deposits as archives for a total of 20 study sites in the framework of joint Russian-German research projects in the northeast Siberian Arctic since 1998. The main research foci for the fieldwork were paleoecology, sedimentology, stable water and carbon isotope geochemistry, geophysics, biogeochemistry, and geochronology. Site-specific information and resulting publications are summarized in Supplementary Table S1. In the present paper, we report on the heavy and light mineral associations from 18 study sites and discuss their use for reconstructing the sources of permafrost deposits. Grain-size data from most of these sites are available in Schirrmeister et al. (2002b); Schirrmeister et al. (2003a); Schirrmeister et al. (2008); Schirrmeister et al. (2010); Schirrmeister et al. (2011a); Schirrmeister et al. (2011b); Schirrmeister et al. (2013); Schirrmeister et al. (2020).
Our approach is based on the mineralogical composition of periglacial deposits that is affected by the respective source material (bed rocks or older sediments), the local periglacial settings during deposition in, e.g., floodplains, polygonal ponds, thermokarst lakes and at the foot of slopes, and the influence of frost weathering and soil formation after deposition.
Beyond Yedoma Ice Complex, we investigated its stratigraphic context below and above as well as deposits in thermokarst basins and thermo-erosional valleys and further regionally exposed deposits. Therefore, a wide spectrum of middle and late Quaternary periglacial deposition in Northeast Siberia has been comprehensively captured. To disentangle different source rocks and areas, transport pathways, final deposition and freezing, and post-sedimentary processes, we used the associations of heavy and light minerals in fine (63–125 µm) and coarse (125–250 µm) grain-size fractions as indicators. Fine sand heavy mineral associations of the Laptev Sea margin have been studied already elsewhere and can be used for comparison (Peregovich et al., 1999; Schwamborn et al., 2002; Siegert et al., 2002). With the present study we aim 1) to differentiate mineralogical properties of permafrost deposits from various sites, 2) to characterize the mineralogical variability of different cryostratigraphic units at distinct sites over time, and 3) to detect post-sedimentary changes in mineral compositions. Our study sheds light on late Quaternary periglacial depositional processes and their variations over time and contributes to the understanding of permafrost formation in Northeast Siberia.
Study Region
The study region covers the coastal lowlands of the western and the eastern Laptev Sea, the Lena Delta, the coasts of the Dmitry Laptev Strait connecting the Laptev and the East Siberian seas, and several coastal sites of the New Siberian Archipelago (Figure 1; Supplementary Figure S1). Small mountain ridges of 200–500 m height such as the Pronchishchev Ridge, the Chekanovky Ridge and the Kharaulakh Range frame the coastal lowlands in the western part of the study region (study sites 9–18; Figure 1). In the eastern part of the study region (study sites 1–8; Figure 1), only isolated basement elevations of granite domes exist such as those on Bol’shoy Lyakhovsky Island and in the hinterland of the Oyogos Yar coast, while on Stolbovoy, Belkovsky, and Kotel’ny islands denudated fold ranges are present. The small mountains and heights are often surrounded by multilevel cryopalanation terraces reaching altitudes between 20 and 150 m above sea level (asl). Many of the sites are located at the distal lowland ends of hillslope foreland plains such as in Bykovsky Peninsula.
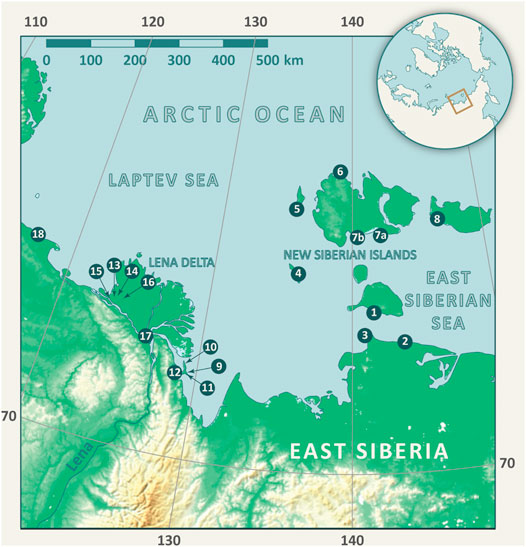
FIGURE 1. Position of the study region in the Laptev Sea area and the positions of the study sites. 1—Bol’shoy Lyakhovsky Island; 2—Oyogos Yar coast; 3—Cape Syatoy Nos; 4—Stolbovoy Island; 5—Bel’kovsky Island; 6—Cape Anisii, Kotel’ny Island; 7—Bunge Land (a—higher terrace, b—lower terrace); 8—Novaya Sibir’ Island. Bykhovsky Peninsula: 9—Mamontovy Khayata; 10—Bykovsky North; 11—Ivashkina Lagoon; 12—Khorogor Valley. Lena Delta: 13—eastern Ebe Basyn Island; 14—Turakh Island; 15—Nagym, southern Ebe Basyn Island; 16—Khardang Island; 17—Kurungnakh Island; 18—Cape Mamontov Klyk (maps compiled by S. Laboor, AWI Potsdam).
The stratigraphic classification of the middle Pleistocene to the Holocene in the East Siberian Arctic lowlands is based mostly on palynological (e.g., Gitermann et al., 1982; Kaplina and Lozhkin, 1984), palaeozoological (e.g., Sher, 1971; Vangenheim, 1977), and cryolithological (e.g., Kaplina, 1981; Kaplina, 1989; Tumskoy, 2012) and geochronological data (e.g., Schirrmeister et al., 2002a; Schirrmeister et al., 2003a; Schirrmeister et al., 2008; Schirrmeister et al., 2011b; Andreev et al., 2004; Andreev et al., 2009; Wetterich et al., 2009; Wetterich et al., 2011; Wetterich et al., 2014; Wetterich et al., 2016; Wetterich et al., 2019; Wetterich et al., 2020; Wetterich et al., 2021; Zimmermann et al., 2017). The study of local profiles resulted in numerous separate stratigraphic schemes, often with individual terms for single suites, layers, or horizons, which were correlated for further chronostratigraphic classification. However, for some periods, the stratotype, the palaeoenvironmental interpretation, and the absolute age, such as of the Kargin stratum, are still under discussion (Astakhov and Mangerud, 2005; Astakhov, 2013). Because of the inconsistent regional Quaternary stratigraphy of northern East Siberia, we present the Russian classification of the studied period in correlation with the Marine Isotope Stage (MIS) (Table 1).
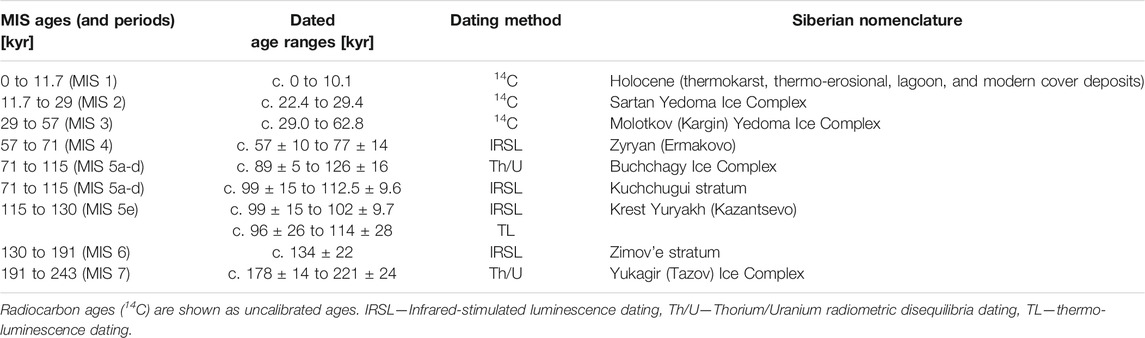
TABLE 1. The regional Quaternary stratigraphic schemes and the corresponding Marine Isotope Stage (MIS; Lisiecki and Raymo, 2005) used in this work according to Andreev et al. (2004, 2009), Wetterich et al. (2019, 2021) and Zimmermann et al. (2017) and references cited therein.
Materials and Methods
Field Work
Our research is based on detailed field work during spring and summer seasons between 1998 and 2007 (see Supplementary Table S1). Permafrost coastal cliffs and riverbank exposures up to 40 m asl were studied. In places, drilling up to 10 m depth below land or ice surface (bs) was carried out. At permafrost outcrops, it often was necessary to study accessible subsections and extrapolate their characteristics across the entire section. Steep bluffs and extensive mudflows from melting ground ice limited the accessibility at some sites. Subsections consisted of sediment sequences deposited in fossil ice-wedge polygon centers exposed between melting ice wedges on steep bluffs or in still-frozen, undisturbed thermokarst mounds (baidzherakhs) on thermo-terraces in front of bluffs. The geometry and thickness of such exposures were surveyed using a laser tachymeter and measuring tapes, accompanied by an initial stratigraphic assessment. We described the frozen sediments in the field by color, organic remains, sediment type, and cryostructures according to own experiences and based on commonly acknowledged terminology (for overview see French and Shur, 2010). General exposure profiles were created by combining and stacking sub-sections up to 5 m thick. Depending on exposure conditions and the accessibility of steep cliffs such sequences were studied in overlapping subsections or in full vertical profiles. We cleaned outcrop walls and drill core segments with shovels, scrapers, and knifes and described the visible cryolithology. Each subsection was sampled at 0.2–1.0 m intervals with hammer and axe. Collected single frozen sediment samples weighed from 0.5 to 1.0 kg and were sealed in plastic bags for transport to the lab.
Analytical Studies
In total, 486 samples were studied for heavy mineral associations of the fine fraction (63–125 µm) and in 282 of these samples the coarse fraction (125–250 µm) was additionally analyzed. Furthermore, light mineral associations were studied in 242 samples of the fine fraction and 131 samples of the coarse fraction (Table 2).
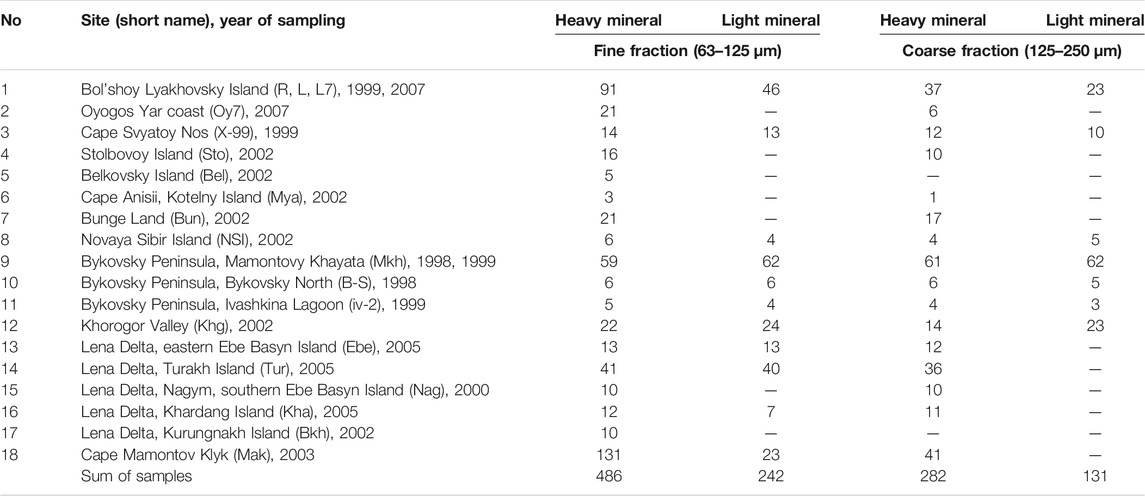
TABLE 2. Number of analyzed samples per site and fractions (heavy and light minerals and grain-size fractions).
To remove organic matter samples were treated three times a week over several weeks with 100 ml of 3% H2O2 in a horizontal shaker. Approximately 3 g of an organic-free sample was separated into the two fractions (63–125 μm and 125–250 µm) by wet sieving on the sieve tower. According to Zadkova (1973), and Boenigk (1983), the 63–125 µm fraction contains the richest heavy mineral association, less affected by transport processes. Therefore, this fraction is the most suitable for subdividing sediment sequences by mineralogical composition. The 125–250 µm fraction is used complementarily. The mineral preparation was performed according to standard procedures (Boenigk, 1983; Mange and Maurer, 1992) using sodium metatungstate solution (Na6(H2W12O40) × H2O; density 2.89 g/cm3). After 20 min centrifugation, the heavy fraction was frozen in liquid nitrogen (Fessenden, 1959; Scull, 1960) and the heavy and light fractions were separated.
The mineralogical composition was analyzed by O. Babiy and I. Klimova at the Melnikov Permafrost Institute in Yakutsk (Russia) under the stereo microscope TECHNIVAL 2 including mineral determination, grain rounding, shape, weathering degree, intergrowths. Additionally, a polarization microscope (POLAM L-213M) was used for quantitative analysis on slides applying immersion liquids with n = 1.53 and n = 1.63 for the light and heavy fraction, respectively. For each fraction mostly 300 to 400 grains per sample were counted in the coarser fraction. Sometimes fewer grains could be counted while the numbers of counted grains are always indicated in the analysis data. The mineralogical composition was calculated in grain percentages. In addition, rock fragments were counted. For the main minerals specific color appearance, grain shape, and signs of weathering on grain surfaces were noted. Grain shapes were observed visually during microscopic analysis. The terminology follows Moorhouse (1959).
The heavy mineral association includes ilmenite, epidote, clinopyroxene (monocline), orthopyroxene (rhombic), amphibole, garnet, zircon, apatite, tourmaline, sphene, rutile, anatase, leucoxene, disthene, staurolite, andalusite, chloritoide, spodumene, corundite, orthite, sillimanite, chromite, spinelle, pyrite, biotite, chlorite, muscovite, and weathered mica. The light mineral association includes quartz, feldspar, zeolithe, carbonate, muscovite, biotite, chlorite, broken mica, and rock debris and aggregates.
Since the micas can occur in heavy and light mineral fractions according to their marginal density, they were listed in both fractions and used for comparisons within these fractions. They are shown graphically as biotite, muscovite and weathered mica individually. Thereby also weathered muscovite (Fe accumulations) can be found in the heavy mineral fraction, and vice versa biotite can be present the light mineral fraction after removal of Fe during chemical weathering. The heavy mineral content was calculated for each sample and average values were determined for the respective stratigraphic units.
To characterize the separate profiles, different mineral coefficients were used. The zircon + tourmaline + rutile (ZTR) index indicates the chemical and mechanical alteration of a sediment composition (Hubert, 1962). The zircon + tourmaline + rutile/pyroxenes + amphibole (ZTR/Py + Am) coefficient estimates the weathering intensity following soil formation (modified from Marcinkowski and Mycielska-Dowgiałło, 2013). For example, a ZTR/Py + Am value of 0.003 indicates low weathering; the unstable minerals (pyroxene, amphibole) dominate the stable minerals (zircon, tourmaline, rutile). In contrast, a ZTR/Py + Am value of 0.46 indicates more intense weathering, as the stable minerals dominate over the unstable minerals. The noticeable differences between the pyroxenes/amphiboles (Py/Am) coefficient and the quartz/feldspar (Q/Fs) coefficient point to changes in the source areas (Nesbitt et al., 1996). The role of quartz during frost weathering (Konishchev, 1982) gives another indication of the intensity of this process.
Statistics
In a first step of statistical analysis, we performed an analysis of variance (ANOVA), using a Tukey Honest Significant Differences Test (Tukey, 1949) for multiple comparison of mineral content means averaged according to site and statistical units of sample mineral associations. The test was performed using R’s inbuilt TukeyHSD functionality (R core team, 2021), with the default 95% family-wise confidence level. This test allows minerals to be identified that contribute significantly to a distinction related to the statistical unit of a sample at a specific sample site. We consider separation between stratigraphic units to be prominent when at least five minerals show statistically significant separation.
In order to further explore differences and commonalities between mineral associations from different ages and different sites, we performed an agglomerative hierarchical cluster analysis on means of mineral associations according to age and sample site. Since the mineral associations are determined as grain counts, we used chi-squared distances in the analysis, and the clustering method was average. We assessed the statistical significance of determined clusters using a bootstrapping approach following Suzuki and Shimodaira (2006). The analysis was performed using R’s pvclust package (Suzuki et al., 2019).
Results
The Coasts of the Dmitry Laptev Strait
The study sites on Bol’shoy Lyakhovsky Island are located on the northern shore of the Dmitry Laptev Strait (Figure 1; study site 1). The heavy mineral content of the fine fraction was highest in the MIS 5d (MIS 5a-d) (0.9%) and MIS 1 (1.5%) sediments. The heavy mineral content of the coarse fraction was highest in MIS 5e (3.6%) and MIS 1 (2.1%). In the other units the heavy mineral content was between 0.2 and 0.7 in both fractions. Grains of both fractions from this sample set (91 samples) are mostly subangular and angular. Detailed grain descriptions for each stratigraphic unit are summarized in Supplementary Table S2. In the heavy mineral fractions, ilmenite, sphene, and anatase are partly leucoxenized. Epidote shows irregular greenish fragments. Zoisite occurs in small amounts. Rhombic pyroxenes are represented by hypersthene and enstatite. Enstatite occurs as fragments of prismatic crystals with distinct cleavage cracks, slightly greenish and colorless. Amphiboles are mostly dark green, rarely brown or colorless. Pyroxenes and amphiboles are sometimes chloritized. Tourmalines are columnar, blue, pink, brown, and green with strong pleochroism. Zircons and anatase are prismatic bipyramidal. Garnet (almandine) is pink, sometimes corroded, with numerous ore inclusions. The light mineral fractions consist of organo-mineral aggregates. Quartz and feldspar are contaminated with iron-humus stains and often occur in intergrowths. Feldspars are partly chloritized. Calcite is colorless or brown (ferruginous) and micro-oolitic.
Regarding the heavy mineral composition (Figure 2A) of the fine fraction, more ilmenite and garnet are present in the MIS 7 and 6 sediments, while epidote is increased in MIS 2 deposits. Amphibole dominates the spectrum and is most abundant in MIS 3 and 1 deposits. Leucoxene content is higher in MIS 7, 6, and 2 deposits. Mica and chlorite contents are significantly increased in deposits of MIS 5e, 5a–d, and 4 ages (36, 54, and 40% respectively). The coarse fraction shows comparable heavy mineral associations. The light mineral fractions contain little quartz (especially in MIS 5e, 5a–d, 4, and 3) and are dominated by feldspar (34–62%, Figure 2A). The second most dominant components are rock fragments (debris) and aggregates. Mica occurs at elevated levels in MIS 5e, 5a–d, and 2 (in the fine fraction: 16, 20, and 13% respectively).
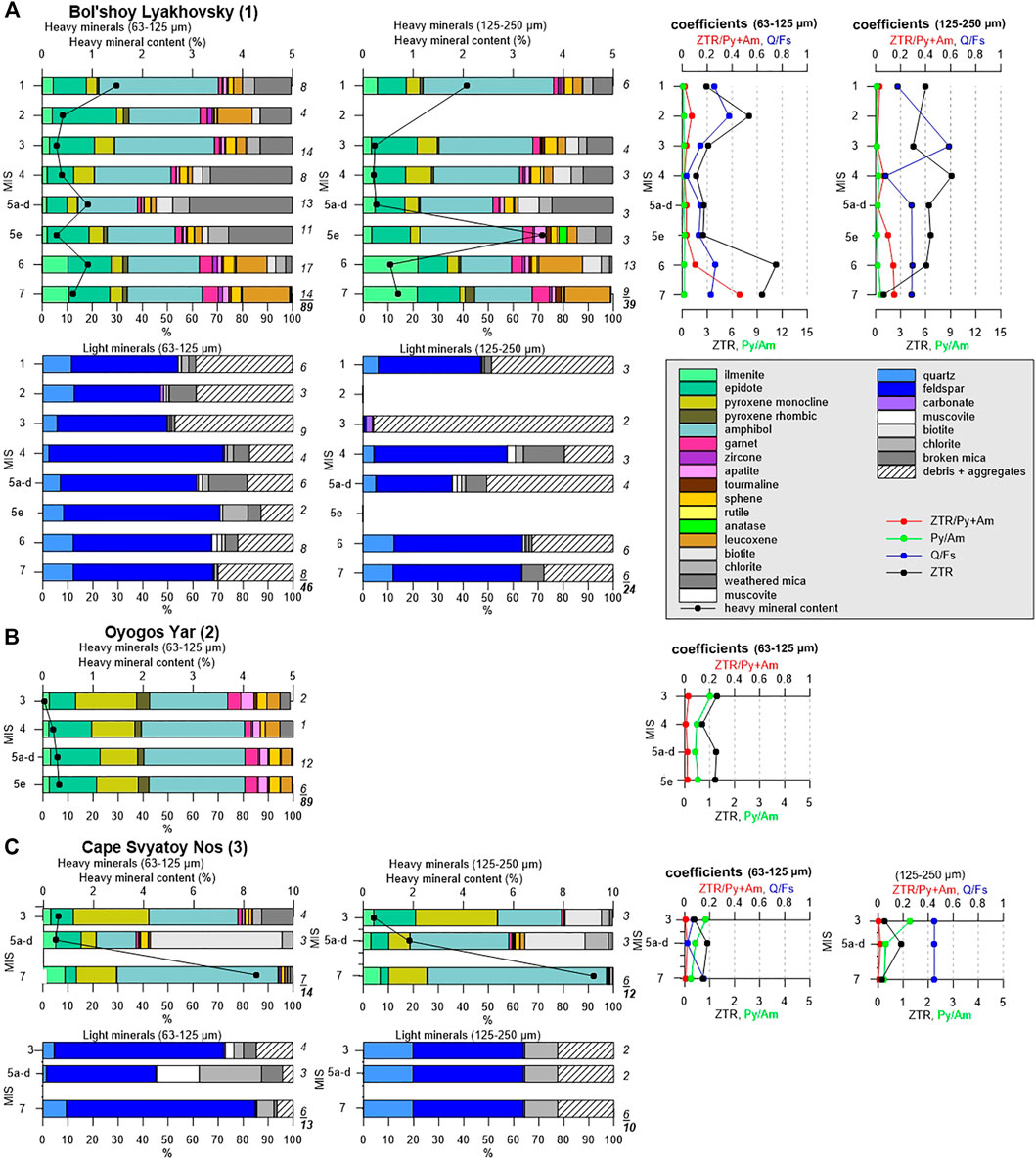
FIGURE 2. Heavy and light mineral associations of the fine and coarse fractions, the heavy mineral contents, and the corresponding mineral coefficients from (A) Bol’shoy Lyakhovsky Island (1), (B) the Oyogos Yar coast (2), and (C) Cape Svyatoy Nos (3). The number of samples is in italics to the left of each diagram (Note the different scales for heavy mineral contents and the mineral coefficients).
With respect to the studied mineral coefficients of the fine fraction, the MIS 7, 6, and 2 sediments indicate stronger weathering, as can be seen from the ZTR (9.6, 11.3, and 8.0 respectively) and ZTR/Py + AM (0.46, 0.10, and 0.07 respectively) values. The Py/Am and Q/Fs quotients show no clear distinction within the stratigraphic profile. This implies that the source area of the sediments did not change significantly over time. For the coarse fraction, the ZTR/Py + Am coefficients for MIS 7, 6, and 5e are increased (0.15, 0.14, and 0.10 respectively). The ZTR values are increased for MIS 7, 6, 4, and 1 units. The Py/AM ratios vary between 0.63 (MIS 7) and 0.14 (MIS 1). The Q/Fs quotients are similar in MIS 7, 6, and 5a–d sediments (0.29) and highest in the MIS 3 deposits (0.59).
The study area of the Oyogos Yar coast is located on the mainland shore of the Dmitry Laptev Strait (Figure 1; study site 2), about 100 km southeast of the Bol’shoy Lyakhovsky Island site. From this sample set (21 samples) only the fine heavy mineral fractions from MIS 5e, 5a–d, 4, and 3 were analyzed. The heavy mineral content was quite low (0.3, 0.3, 0.2, and 0.02% respectively). Most of the grains are subangular and angular. Epidote is of variable preservation, with small amounts of zoisite. Monoclinic pyroxene is represented mainly by diopside. Rhombic pyroxene (hypersthene) is rare. Amphiboles are mostly dark green, more rarely brown and light green. Garnet, epidote, and ilmenite are often intergrown with feldspars. Titaniferous minerals are partially leucoxenized. In general, the associations in the four sedimentary units studied here are quite similar (Figure 2B). There is little ilmenite (2–3%), relatively high epidote (10–20%), monoclinic pyroxene (15–23%), and amphibole (30–40%). Rhombic pyroxene, garnet, zircon, apatite, and tourmalines are few (1–5%). Leucoxene also occurs (4–6%). Micas and chlorite are completely absent. The ZTR/Py + Am (0.01–0.03) and ZTR (0.70–1.28) coefficients are quite low and similar for the four sedimentary units. The Py/Am quotient (0.44–1.02) is much higher than on the opposite Bol’shoy Lyakhov Island site.
Cape Svyatoy Nos is also located on the mainland side of the Dmitry Laptev Strait about 100 km west of the Oyogos Yar site and about 50 km south of the Bol’shoy Lyakhovsky Island site (Figure 1, site 3). The upper profile section between 9–25 m asl was dated as MIS 3 (Schirrmeister et al., 2011b). The middle and the lower parts of the exposure were assigned to MIS 5a–d and MIS 7 (Table 1), respectively (Gilichinsky et al., 2007; Blinov et al., 2009). The heavy mineral content of these three units clearly differs. The MIS 7 deposits have a very high heavy mineral load in the fine and coarse fractions (8.2 and 9.2% respectively). The other samples at Cape Svyatoy Nos range between 1.8 and 0.4%. The grains of this sample set (14 samples) are angular and often consist of mineral aggregates. Pyroxene and amphibole are partially chloritized and iron-bearing (Supplementary Table S2). Monocline pyroxene is diopside. Amphibole mostly predominates the heavy mineral associations. In the MIS 5a–d unit mica and chlorite are enriched. In light mineral fractions angular and fresh plagioclase predominates. Carbonate occurs as micro-oolith. The mineral compositions show clear differences between the three stratigraphic units studied here, in both heavy mineral fractions and the fine light mineral fraction. The coarse fraction of the light mineral association shows a similar composition for all three units. The heavy mineral associations are similar for each unit in both fractions (Figure 2C). Epidote, pyroxene, and amphibole dominate the MIS 3 and MIS 7 sediments, whereas amphibole in the MIS 7 deposits makes up more than half of the composition. The MIS 5a–d sediments are characterized by a high concentration of mica and chlorite in both the heavy and the fine light mineral fraction. This corresponds to similar observations on Bol’shoy Lyakhov Island (Figure 2A). The ZTR, Q/Fs, and ZTR/Py + AM mineral coefficients differ only slightly between the fine and coarse fractions. Only Py/Am shows a significant increase from MIS 7 to MIS 3 (from 0.2 to 1.28).
Northern New Siberian Islands
We studied coastal outcrops of Stolbovoy, Bel’kovky, and Novaya Sibir islands and on Bunge Land as well as a thermokarst mound near Cape Anisii on northern Kotel’ny Island (Figure 1; study sites 4–8) during a ship expedition in 2002 (Supplementary Table S1). No descriptions of grain shapes and mineral properties (except for Bunge Land) are available for most of these sites.
From Stolbovoy Island there are heavy mineral analyses of fine and coarse fractions (16 samples). Heavy mineral contents are high for both fractions and almost all units (1.1–7.8%). The composition is characterized by a predominance of mica and chlorite in the MIS 4 and 3 units of the fine fraction and even greater enrichment in all three units of the coarse fraction (Figure 3A). In particular, we did not find muscovite in any of the heavy mineral compositions. A single Holocene (MIS 1) sample is dominated by ilmenite, epidote, pyroxene, and amphibole. The mineral coefficients are relatively low and do not show strong differences between the three studied units.
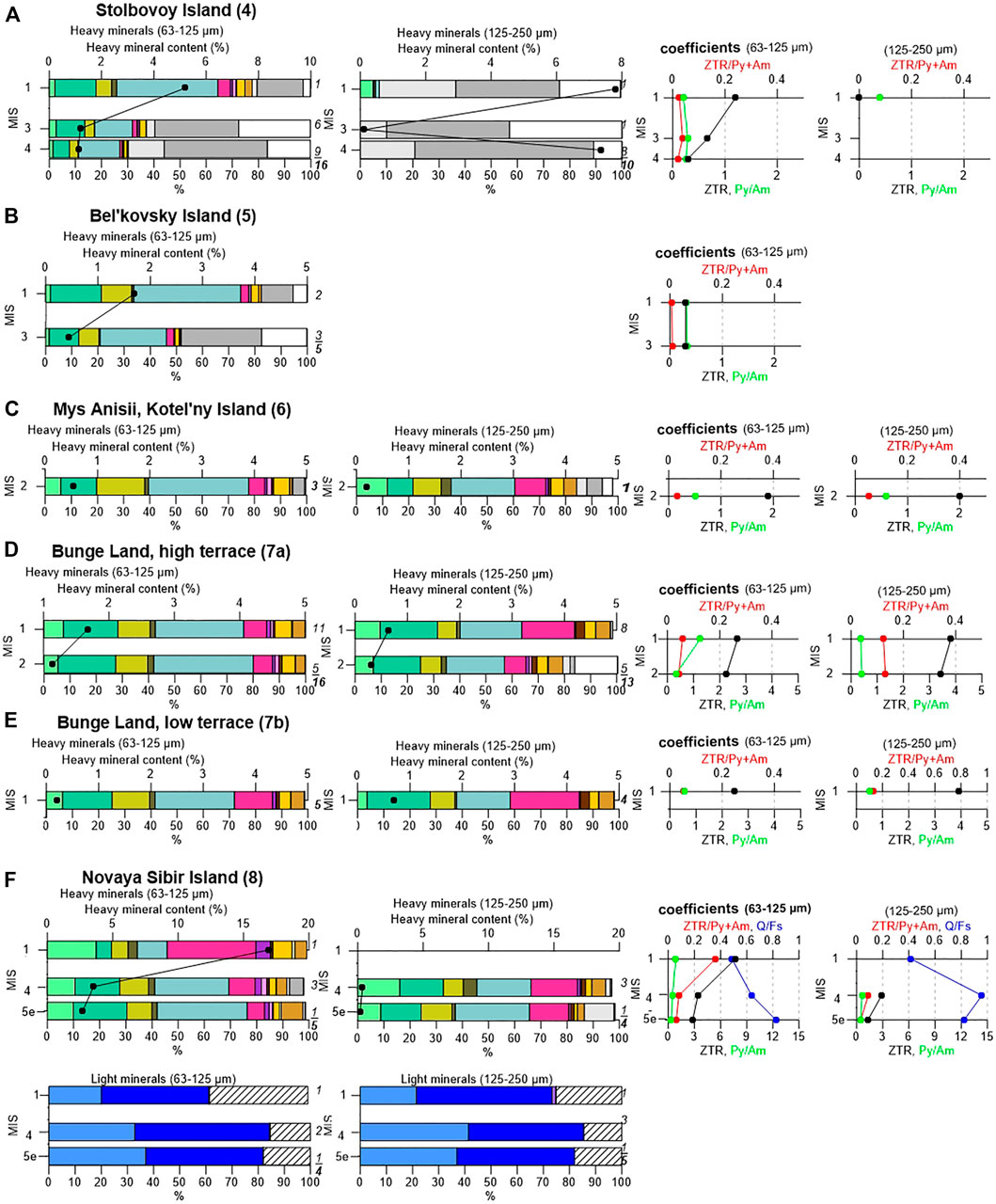
FIGURE 3. Heavy and light mineral associations of the fine and coarse fractions, the heavy mineral contents, and the corresponding mineral coefficients from the northern New Siberian Islands: (A) Stolbovoy Island (4), (B) Bel’kovsky Island (5), (C) Mys Anisii, Kotel’ny Island (6), (D) Bunge Land, high terrace (7a), (E) Bunge Land, low terrace (7b), and (F) Novaya Sibir Island (8). The number of samples is in italics to the left of each diagram (note the different scales for the heavy mineral contents and mineral coefficients). For the legend, see Figure 2.
From Bel’kovsky Island, we analyzed the fine heavy mineral fraction of five samples from a 1.5 m profile. The heavy mineral contents average between 0.4 and 1.7%. In the MIS 3 unit ilmenite, epidote, pyroxenes, and amphibole are approximately equally represented compared to biotite and muscovite, while they dominate the composition in the MIS 1 unit (Figure 3B). The ZTR, ZTR/Py + Am, and Py/Am coefficient are small and do not differ between the two units.
From Cape (Mys) Anisii in the north of Kotel’ny Island, we studied three samples from a 1.5 m profile (MIS 2). The heavy mineral content is low (0.2–0.5%) but from the deepest sample both heavy mineral fractions could be analyzed. The mineral association in both fractions is similar (Figure 3C). Ilmenite, epidote, pyroxene, and amphibole dominate the spectra. Relatively high garnet contents in these two fractions of 6 and 12% are remarkable. The ZTR, ZTR/Py + AM, and Py/Am mineral coefficients are 1.9–2.0, 0.03–0.05, 0.5–0.6 respectively.
From Bunge Land, a 70 × 70 km wide sandy plain between the Kotel’ny and Faddeyevsky islands (Figure 1), two sites (with 21 samples) have been studied (Schirrmeister et al., 2010). One is the remnant of a Lateglacial (upper MIS 2) to Holocene (MIS 1) higher terrace (ca. 15 m asl). The other site, the lower terrace, is located near the shore on a 2–5 m high wide sandy area. The sediment is of late Holocene age (MIS 1). Heavy mineral contents are high in the fine fractions (1.1–4.1%) and small in the coarse fractions (0.3–0.7%). The grains of both heavy fractions are well rounded and well preserved (without clear marks of weathering). Ilmenite is often leucoxenized and intergrown with feldspars (Supplementary Table S2). Epidotes are fragments of irregular shape, transparent to cloudy. Monocline pyroxenes consist of colorless and light green diopside, more rarely of augite. The amphibole belongs to ordinary hornblende and is mostly a dark green, almost black color; brown and colorless varieties rarely occur. Mineral compositions in the fine fraction of the higher terrace are similar for both units (Figures 3D,E). Mica and chlorite occur only in the coarse fraction of the MIS 2 unit. The high garnet content in all samples (8–26%) is remarkable. The relatively low heavy mineral coefficients (ZTR/Py + Am 0.05–0.13, ZTR 2.4–3.9; Py/Am 0.4–0.5) confirm the microscope observations of fresh, unaltered mineral grains.
On Novaya Sibir Island, a profile was investigated that was assigned to the MIS 4 (four samples). In addition, one sample was defined as marine MIS 5e and one surface sample belongs to the MIS 1. The heavy mineral contents are high (0.9–3.5%). The surface sample is very rich in heavy minerals with 16.9%. The MIS 5e and 4 deposits have comparable heavy and light mineral compositions (Figure 3F). Remarkable are the relatively high garnet contents, which increase up to 34% in the fine fraction of the MIS 1 surface sample. The mineral coefficients are relatively similar for the MIS 5e and MIS 4 deposits (ZTR/Py + Am 0.03–0.09, ZTR 1.3–3.4, Py/Am 0.3–0.6, Q/Fs 0.4–1.0). The MIS 1 surface sediment is characterized by increased ZTR/Py + Am (0.36), ZTR (7.7), and Py/Am (0.9) values, and similar Q/Fs value (0.5).
Bykovsky Peninsula and the Adjacent Khorogor Valley
The main study site on Bykovsky Peninsula (Figure 1; study sites 9–12) was the Yedoma cliff of Mamontovy Khayata and the adjacent thermokarst basin (61 samples). In addition, we included the Bykovsky North site (six samples) about 10 km to the north and the Ivashkina Lagoon (five samples) about 4 km to the south. Most mineral grains from the Bykovsky Peninsula are slightly rounded, but angular. Quartz grains occur predominantly as fresh quartz in the light mineral fraction. The heavy mineral composition is dominated by pyroxene and amphibole. Pyroxene consists mainly of greyish brown augite with rare occurrence of diopsite and hypersthene (Supplementary Table S2). Amphibole is dominated by ordinary green colored hornblende. Ilmenite, leucoxene, and epidote are present, and garnet, apatite, and sphene occur in smaller amounts. Zircon and tourmaline are observed in minor amounts. Chlorite occurs in most samples. Weathered mica occurs less frequently. Small amounts of rutile, disthen, chloritoid, staurolith, and andalusite were counted. Angular intergrowths of pyroxene, amphibole, and opaque minerals occur. Aggregations with feldspars were also observed. Augite and hornblende are mainly unchanged. Only some grains show signs of weathering. Feldspars, predominantly potassium feldspar, are often cloudy.
The profiles of the Mamontovy Khayata outcrop include sediments from MIS 3 to MIS 1. The heavy mineral content is relatively high in the fine fraction (0.9–1.9%) and lower in the coarse fraction (0.4–1.2%). The heavy and light mineral associations in both fractions are similar in the three stratigraphic units (Figure 4A). This is also reflected in the similar values of the ZTR (1.9–2.4), ZTR/Py + Am (0.03–0.05), and Q/Fs (0.16–0.19) mineral coefficients of the fine fraction. Only the Py/Am ratio changes more between the units. The coarse fraction also has coefficients quite close to each other, but they are higher for ZTR and Py/Am and lower for the other two coefficients. The light mineral association is dominated by mineral debris and aggregates.
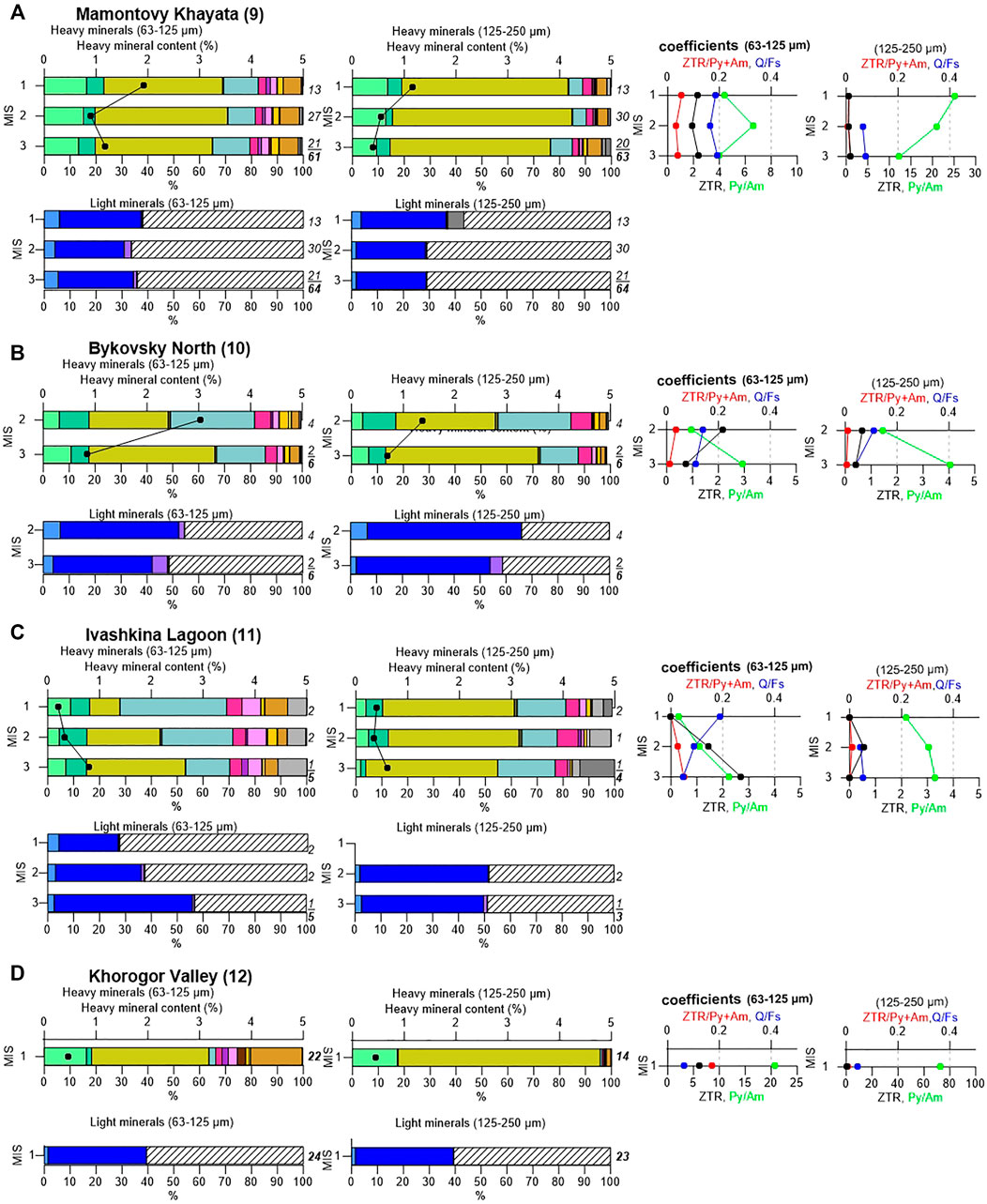
FIGURE 4. Heavy and light mineral associations of the fine and coarse fractions, the heavy mineral contents, and the corresponding mineral coefficients from Bykovsky Peninsula at study sites (A) Mamontovy Khayata (9), (B) Bykovsky North (10), (C) Ivashkina Lagoon (11), and (D) the Khorogor Valley (12). The number of samples is in italics to the left of each diagram (Note the partly different scales for the mineral coefficients). For the legend, see Figure 2.
The profile of Bykovky North covers six samples from MIS 3 and 2. The heavy mineral contents for both fractions are relatively high (0.7–3.1%). The mineral associations are slightly different from the Mamontovy Khayata outcrop (Figure 4B). First, there is significantly more amphibole (15–33%), which is also reflected in a lower Py/Am ratio. Second, there are stronger differences between the two MIS 2 and 3 investigated units in ilmenite, epidote, monocline pyroxene, and amphibole contents. Carbonate (2–6%) is present in both units as well as in both fractions.
All five samples from a drill core from the Ivashkina Lagoon are characterized by a quite low heavy mineral content (0.2–0.8%), subangular grains, insignificant ore mineral content or lack thereof, and uniform mineralogical composition. Monoclinic pyroxenes are represented by gray-brown augite; a minor amount of light green diopside is present. Amphiboles are represented by dark green hornblende. Pyroxene and amphibole are partially fractured and ferruginous. The light fraction is represented by feldspars, clasts of chlorite schists, sedimentary rock fragments, and aggregate grains. Reddish brown ferruginous carbonate grains occur in dense aggregation (probably micro concretions). The mineral associations from Ivashkina Lagoon show clear differentiations between the units in the fine fraction. From MIS 3 to MIS 1 the pyroxene content decreases and the amphibole content increases (Figure 4C). For the light minerals, the feldspar content decreases. In contrast, mineral associations and coefficients are quite similar in the coarse fraction.
The Kharaulakh Range including the Khorogor Valley is considered the source area for the late Pleistocene deposits of Bykovsky Peninsula (Schirrmeister et al., 2002a; Siegert et al., 2002; Slagoda, 2004; Grosse et al., 2007). Modern (MIS 1) samples (24) from different surface sites, like frost boils or river sediments, were taken during field work around Tiksi in 2002 (Grosse et al., 2003). The samples have mostly the same mineralogical composition of terrigenous material, but they differ in quantity. The average heavy mineral content is about 0.5%. They contain rock fragments - predominantly fragments of chloritic shales, which occur as outcrops in the valley slopes. In addition, there are small amounts of sedimentary and metamorphic rock fragments. All mineral grains are angular, but in fluvial sediments some grains are rounded to subrounded. Very often they occur as clusters of several minerals (ilmenite and augite, leucoxene and sphene, feldspars with minerals of the heavy fraction, etc.). This is typical for a close source area. Predominant heavy minerals are pyroxene (19–72%) and titaniferous minerals like ilmenite, leucoxene, sphene, and rutile (15–53%). Feldspar, which predominates in the light fractions, is mostly altered and turbid. Samples rich in humus and iron hydroxide show brownish colored grains with stained surfaces and fillings in cleavage cracks. The studies of the modern sediments from the Khorogor Valley show a strong dominance of pyroxene and underrepresented amphibole in both fractions (Figure 4D). Therefore, the Py/Am coefficients of 21 and 73 in these two fractions are significantly higher than in many other results shown here.
The Lena Delta
We collected samples from riverbank outcrops from Kurungnakh, Khardang, and Ebe Basyn islands in the western Lena Delta, and two bank outcrops and a 11.4 m deep adjacent borehole from Turakh Island also in the western Lena Delta (Figure 1, study sites 13–17). Further details on fieldwork and results can be found in references of Supplementary Table S1.
For the eastern part of Ebe Basyn Island, we analyzed seven samples from three 1–2 m deep trenches and five samples from a 4 m deep shore profile. These were fluvial sediments deposited during MIS 2 and MIS 1 periods. The average heavy mineral contents are high, with 9–12% for fine and about 2.5% for the coarse fractions. Grains are mostly angular or subangular. Most epidote grains are dark and occur as fresh mineral fragments (Supplementary Table S2). Some epidote grains are intergrown with hornblende. Zoisite is rare. Ilmenite is partially leucoxenized. Rhombic pyroxenes are represented by hypersthene. Enstatite is rare. Monoclinic pyroxenes are represented by diopside and augite. Zircons have an oval-elongated pomegranate shape and are bipyramidal. The heavy mineral association of the fine fraction is similar for both units (Figure 5A). The coarse fraction is characterized by higher epidote, pyroxene, and garnet contents and lower amphibole contents than the finer fraction. There is more pyroxene (14–27%) and less amphibole (30–43%) compared to the Nagym site. The garnet content (6–12%) is quite similar. The light mineral fraction is dominated by feldspars (approx. 60%). At 13–17%, quartz is represented as frequently as on Khardang Island. The mineral coefficients have ranges similar to those of the previous sites.
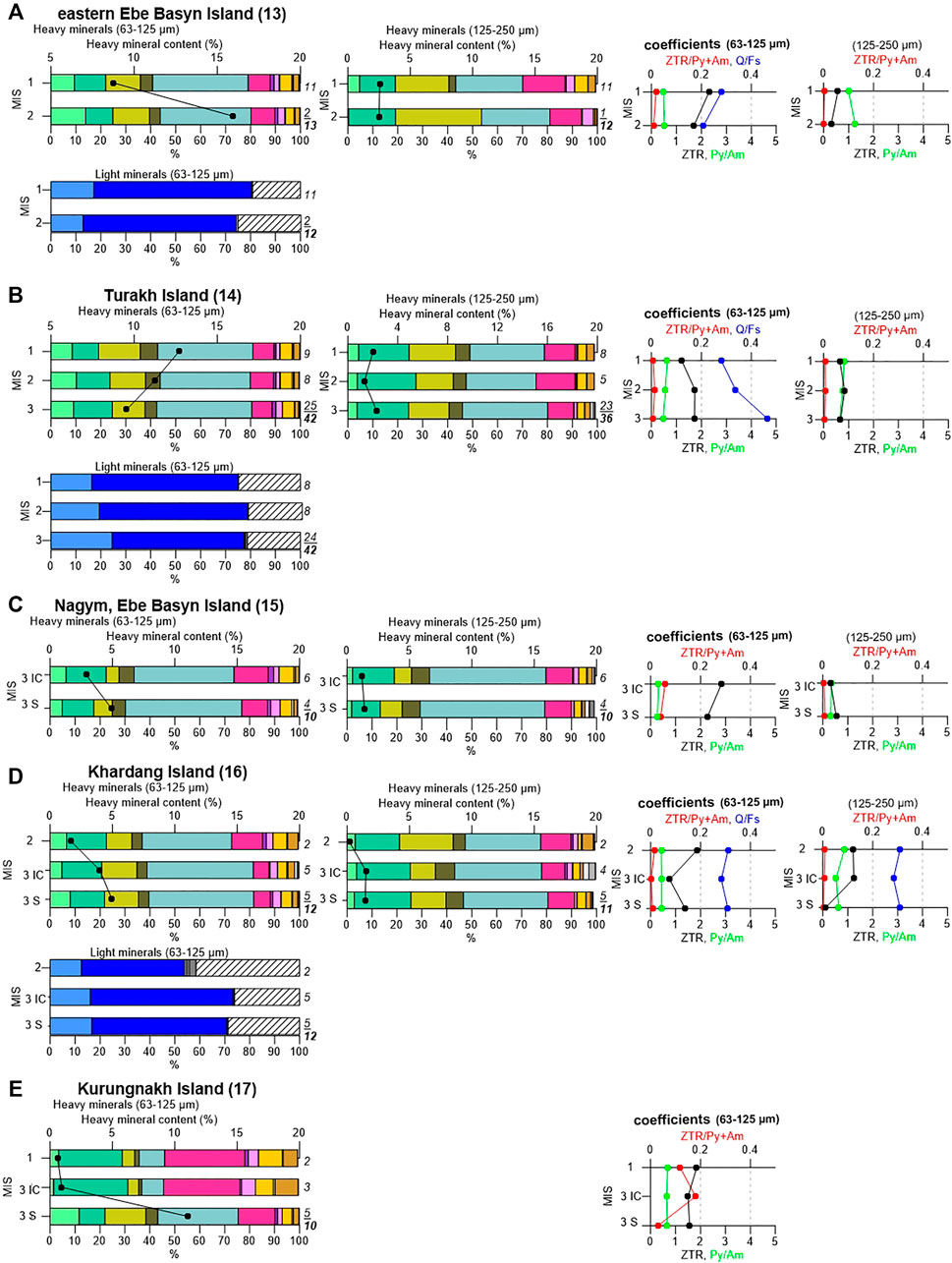
FIGURE 5. Heavy and light mineral associations of the fine and coarse fractions, the heavy mineral contents, and the corresponding mineral coefficients from the Lena Delta sites on (A) eastern Ebe Basyn Island (13), (B) Turakh Island (14), (C) Nagym, southern Ebe Basyn Island (15), (D) Khardang Island (16), and (E) Kurungnakh Island (17). The number of samples is in italics to the left of each diagram (Note the mineral coefficients have smaller scales than in previous figures). For the legend, see Figure 2.
Turakh Island belongs to the Arga Complex, a large sandy plain with many oriented lakes, which forms the second terrace of the Lena Delta (Schwamborn et al., 2002). We analyzed a total of 41 samples from two outcrops (6 and 2 m high) and one 11.4 m long drill core. This approximately 17 m long sand profile was formed between MIS 3 and 1. The average heavy mineral contents are very high for the fine fraction (10–13%) and also high for the coarse fraction (1.3–2.3%). Most grains are angular or subangular. Titanium minerals (ilmenite, sphene) are often leucoxenitized (Supplementary Table S2). Epidote is more often opaque with secondary alteration products. Aggregations of several minerals often occur: e.g., of pyroxene with feldspars, zircon with garnet, epidote with chlorite, garnet with epidote, sphene with rutile, ilmenite with feldspars, and others. Rhombic pyroxenes consist of hypersthene. Monoclinic diopsites have colors of different intensity; augite occurs rarely. Garnet is completely angular. Iron bearing carbonates are considered as new formations (probably siderite). Colorless calcite crystals are rare. Fe hydroxides occur in the form of rounded relocation products. All three units (MIS 3 to 1) show similar heavy and light mineral associations. In comparison, the coarse fraction contains more epidote (20–24%) and garnet (10–16%), but less ilmenite (about 4%), while the pyroxene (18–24%) and amphibole (28–38%) contents are relatively similar. (Figure 5B). The mineral coefficients are quite similar for both fractions.
The set of 10 samples from the Nagym site in the southern part of Ebe Basyn Island includes the lower fluvial sands (MIS 3S) and the overlying Yedoma Ice Complex (MIS 3 IC). The average heavy mineral contents are quite high in both units and fractions (1.2–4.9%). Mineral grains have different roundness. Some minerals are fissured with black and brown parts. Ilmenite is often leucoxenized. Epidote is clear and dark; zoisite and intergrowths with hornblende and feldspar occur (Supplementary Table S2). Pyroxenes (diopside and augite) are colorless or brown and are present in aggregates with feldspar, sometimes ferruginous. Enstatite is present among the rhombic pyroxenes. Amphibole is clear and dark with a fractured surface and is sometimes contaminated with clayey material. Garnets are colorless, pink, and brown; grains are angular and occur in aggregates with hornblende and epidote. Zircon is colorless and brownish. Staurolite occurs with carbonaceous inclusions and chlorite is intergrown with ilmenite interspersed with rutile needles. The heavy mineral associations are not significantly different between the lower fluvial sand and the Yedoma Ice Complex deposits and between the fine and coarse fractions (Figure 5C). Amphibole (40–50%) dominates, epidote (11–17%) and pyroxenes (13–16%) are not very frequent, while garnet (10–14%) is quite strongly represented. The ZTR coefficients of the fine fractions of the sands and the Yedoma Ice Complex are 2.3 and 2.8 respectively, which are much higher than in the coarse fraction (0.3 and 0.5 respectively).
The Yedoma Ice Complex sediments from Khardang Island were formed during MIS 3 (10 samples) and MIS 2 (2 samples). In addition, the MIS 3 sequence is divided into lower fluvial sands (MIS 3 S) and the overlying Yedoma Ice Complex (MIS 3 IC). However, there are no clear differentiations between these two units regarding heavy mineral contents and mineral associations. Ilmenite grains are leucoxenized (Supplementary Table S2). The light fraction is calcified and weakly rounded. Quartz grains are intergrown with rutile. Feldspars are contaminated and have black spotty inclusions. Carbonate microaggregates exist as parts of organo-mineral aggregates. The mineral contents in the sand and the Yedoma units and in both fractions are quite similar (Figure 5D). Garnet also occurs frequently (6.5–12%) but not as frequently as on Kurungnakh Island. Traces of carbonate minerals (0.2%) are present, but low. This, however, differs from all other Lena Delta sites, where carbonate minerals are lacking. The mineral coefficients are quite similar (ZTR/PY + Am 0.002–0.015, ZTR 0.1–1.9, Py/Am 0.4–0.9, Q/Fs 0.3) and do not reflect either changes in the source areas or strong weathering and soil formation processes.
On Kurungnakh Island, we analyzed the lower fluvial sands (MIS 3S, 4 samples), the overlying Yedoma Ice Complex (MIS 3 IC, 3 samples), and the Holocene cover (MIS 1, 2 samples). From these studies, no mineral grain descriptions are available. The average heavy mineral content of the fine fraction differs clearly between the MIS 3 sand (11%) and the MIS 3 Yedoma Ice Complex (0.9%) units. The MIS 1 unit is similar to the Yedoma Ice Complex unit (0.6%). Pyroxene and amphibole contents are significantly higher (21% and 32% respectively) in the MIS 3 sand (Figure 5E). The MIS 3 Yedoma Ice Complex and MIS 1 sediments have higher epidote (27–30%) and garnet (31–32%) contents. Mineral coefficients reflect low weathering intensities for the MIS 3 sands (ZTR/Py + Am = 0.02) relative to the other two units (0.10–0.12). The Py/Am coefficient shows no significant differences (0.62–0.95) and thus no significant changes in the source area.
Cape Mamontov Klyk, Western Laptev Sea
The outcrops of Cape Mamontov Klyk are located on the coast of the western Laptev Sea, about 200 km west of the Lena Delta (Figure 1; study site 18). These are fluvial sands (MIS 3 S, 10 samples) overlain by Yedoma Ice Complex deposits (MIS 3 IC, 33 samples; MIS 2, 65 samples) and Holocene (MIS 1, 22 samples) cover sediments and deposits from a thermokarst basin and a thermoerosional valley. The heavy mineral contents are high for the fine fraction (2.3–5.5%) and quite small (0.4–0.8%) for the coarse fractions (Figure 5). The mineral grains are mainly weakly rounded and are well preserved, except for epidote and micas. The predominant minerals of the heavy fraction are epidote, pyroxene, and amphibole. Ilmenite is rounded and weakly rounded, occurs in assemblages with sphene, and is sometimes leucoxenized (Supplementary Table S2). Epidote occurs in the form of irregularly shaped fragments and is clouded by secondary alteration products. Less common are well preserved prismatic crystals. Sometimes epidote grains are found in aggregates with other minerals (e.g., zoisite). Monoclinic pyroxenes are represented by colorless and light green diopside grains. Augites are present as a minor admixture; sometimes the ratio of augites and diopside changes. Rhombic pyroxenes are represented by hypersthene. Amphiboles are represented by mostly dark green, rarely brown hornblende. Garnets are irregularly shaped, weakly rounded, colorless to pink (almandine). Brown, orange, and bright yellow varieties are rare. Zircon is found as prismatic-bipyramidal minerals and their fragments. Tourmaline occurs as prismatic crystals and their fragments. Their coloration is brown (magnesian variety) or more rarely blue (ferruginous variety). Sphene occurs in the form of prisms and envelope-like flattened crystals, but more often as irregular fragments and aggregate grains, often leucoxenized. The coloration of the grains varies from light brown to intense brown, reddish, with strong pleochroism. Rutile is partially leucoxenized. Mica is ferruginous and is fissured. Hydrous iron oxides are often found as spherical formations. They are probably ferruginous marcasite. Carbonates (calcite) are colorless, irregularly shaped fragments; more rarely prismatic crystals, micro-oolites, and rosettes are found.
Although the general distribution of mineral associations in the fine and coarse fractions is similar, there are minor differences between MIS 1 + 2 and MIS 3 IC + S sediments. This concerns especially the mean contents of epidote, monoclinic pyroxene, garnet, and quartz (Figure 6). The predominant minerals of both heavy fractions are epidote, pyroxene, and amphibole (about 80%). Mica and chlorite rarely occur in the heavy mineral fractions, but they are present in the light mineral fraction of the MIS 2 and MIS 1 samples. The similarities of the main components are supported by the quite similar mineral coefficients of ZTR = 0.7–1.5, ZTR/Py + Am = 0.01–0.02, Py/Am = 0.5–0.8, and Q/Fs = 0.3–0.5.
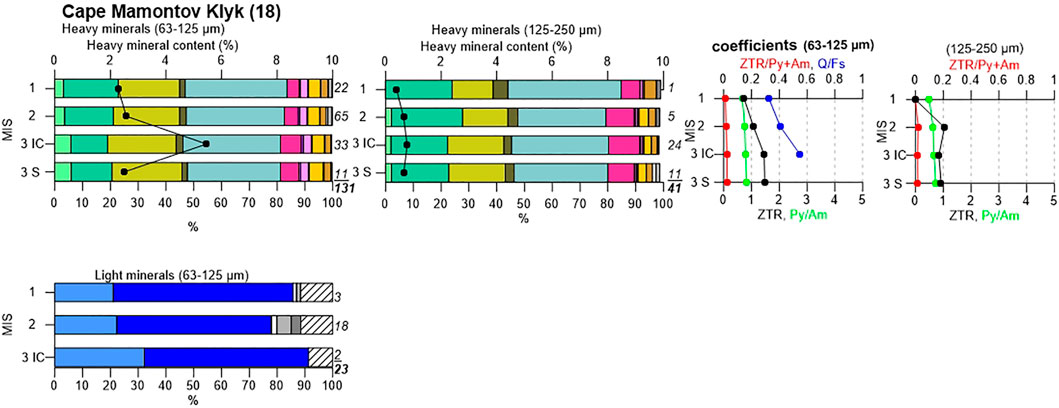
FIGURE 6. Heavy and light mineral associations of the 63–125 μm and 125–250 µm fraction, the heavy mineral contents, and the corresponding mineral coefficients from Cape Mamontov Klyk (18). The number of samples is in italics to the left of each diagram (Note the larger scales for heavy mineral contents and some mineral coefficients than in previous figures). For the legend, see Figure 2.
Discussion
Differences and Similarities—Site Specific and Between Different Sites
The profiles from Bol’shoy Lyakhovsky Island cover the stratigraphically longest period of all sites presented here. High mica and chlorite contents in the heavy (10–54%) and the light mineral association (3–20%) indicates that eolian activities could not have played the major role in the formation of these sediments. According to Reineck and Singh (1980) wind activities would result in destruction of unstable minerals, especially of micas. Only the lowermost exposed unit (MIS 7) has relatively low mica and chlorite contents (1–9%) that might indicate a higher share of aeolian transport in the formation of the unit. Throughout the stratigraphic profile, the MIS 5e interglacial deposits as well as the MIS 5a–d floodplain deposits and the MIS 4 Ice Complex sediments are characterized by high mica and chlorite contents (Figure 2A). The heavy mineral compositions of the MIS 3 and 2 Yedoma Ice Complex deposits and the MIS 1 Holocene sediments are very similar in both fractions. This indicates that the mineral sources and transport pathways did not change significantly during the MIS 3 interstadial and MIS 2 stadial periods and that the mineral content of Holocene sediments mainly derived from thawed and re-deposited late Pleistocene Yedoma Ice Complex deposits. The relatively stable Py/Am (0.14–0.36) and Q/Fs (0.04–0.6) mineral coefficients indicate that the sediment supply area did not change significantly over the studied period. Increased ZTR values of the MIS 7, 6, 4, and 1 units reflect more intense weathering during these periods. Comparing the light and coarse heavy mineral fractions, there are similar trends in mineral contents and associations. Anatase occurs only in the coarse fraction in MIS 5a–d and 5e. The light mineral coarse fraction is dominated by rock debris and aggregates, while the fine fraction is dominated by feldspars. The organo-mineral aggregates in the light fraction are typical of intense soil formation or post-sedimentary alteration under subaquatic conditions. The most probable mineral source area for Bol’shoy Lakhovsky Island are Permian sandstones and phyllitic slates of the nearby Khaptagai-Tas hill and rocks of Permian quartzitic sandstone, slates, basalt, granites, quartz-pebbles, and porphyry found as debris or as solid rocks on the beach (Volnov et al., 1998; Schirrmeister et al., 2000; Kunitsky et al., 2002).
On the Oyogos Yar coast the stratigraphic units of MIS 5e, 5a–d, 4, and 3 ages show very similar heavy mineral associations in the fine fraction. This is also reflected in the similar mineral coefficients within the profile. In contrast to Bol’shoy Lyakhovsky Island, mica and chlorite play only a minor role. The pyroxene content of 17–30% is significantly higher than that found on Bol’shoy Lyakhovsky Island (5–8%). The low ZTR and ZTR/Py + Am coefficients indicate a uniform and relatively low degree of weathering. This means that although Bol’shoy Lyakhovsky Island is close and certainly formed an accumulation area with the Oyogos Yar site in the past, the latter had a different mineral source area that might be Tertiary sediments in the bedrock of the hinterland, according to the regional geological map (Volnov et al., 1998).
The deposits of Cape Svatoy Nos have significantly high heavy mineral content in the fine and coarse fractionsof the MIS 7 unit. In the stratigraphic profile, each unit has a different heavy mineral composition in both fractions. Low ZTR and ZTR/Py + Am coefficients indicate an relatively low weathering degree. Although the associations are quite different in some cases, the mineral coefficients are close to each other, which indicate an relatively low weathering degree for ZTR and ZTR/Py + Am. We assumed the chain of Cretaceous granite domes (Volnov et al., 1998) that dominates the landscape of Cape Svyatoy Nos as source rock.
The MIS 4 and 3 samples from Stolbovoy Island show the highest mica and chlorite contents (fine fraction 22–70%) of all the studied sites. The pyroxene content is lower than 10%. The fine and coarse fractions have distinctly different compositions. The coarse fraction consists almost entirely of mica and chlorite. The low and similar ZTR and ZTR/PY + Am coefficients indicates a low weathering degree and uniform source rocks of Jurassic and Cretaceous sedimentary rocks, exposed at the coast and in higher hill positions (Volnov et al., 1998; Schirrmeister et al., 2003b).
The heavy mineral association of Bel’kovsky Island, located about 140 km to the north, differs by slightly higher pyroxene and amphibole contents (8–13% and 25–40% respectively), and lower but still high mica and chlorite contents (18–48%). The coarse fraction of the heavy minerals contains more ilmenite, garnet, leucoxene, and mica than the fine fraction but less epidote, pyroxene, and amphibole. The small mineral coefficients reflect low weathering degrees and no changes in source rocks. Bedrock consisting of strongly folded Paleozoic sediments (Volnov et al., 1998) and outcrops at the study site (Schirrmeister et al., 2003b) are considered as source rocks.
The composition of the small sample set from Cape Anisii is again different from the two previous sample sets from the northern New Siberian Islands. Mica and chlorite play a minor role (5–14%), while pyroxene is much more represented (15–20%). Mineral coefficients are slightly higher than at the other two sites on the northern New Siberian Islands. Palaeozoic basement of Ordovician and Silurian limestone (Volnov et al., 1998), which is exposed at the beach, is assumed as source rock.
The sediments origin on the higher and lower terraces of Bunge Land differs from the other sites studied on the New Siberian Archipelago. The good rounding of the grains indicates longer transport processes According to Schirrmeister et al. (2010) the higher terrace was accumulated under periglacial flood plain conditions during the Lateglacial to Holocene and the lower terrace has been affected by frequent modern marine inundation. However, the compositions are not much different from those of the neighboring Cape Anisi site, located about 150 km to the north, and the Novaya Sibir Island site located about 150 km to the east; this might point to the same mineral source area, although these sediments are from different time periods and have a different genesis. The northwestern area of strongly folded Kotel’ny Island Paleozoic rocks is considered as source area. The lower terrace is furthermore characterized by high garnet contents. This can be explained by marine relocations in the beach area.
The MIS 1 sample of fine heavy mineral fraction from Novaya Sibir Island is characterized by very high garnet, low amphibole, and very high heavy mineral content. Preliminary, this might be explained with locally scaled aeolian or hydrodynamic sorting in a surface sample. The heavy mineral contents of the other samples are also high. The light mineral data of both fractions are quite similar. The mineral coefficients show significantly different values only for the Holocene cover sediment. This supports a greater amount of alteration of grains and different source rocks for the Holocene cover sediment if compared to any older deposits here. According to the geological map (Volnov et al., 1998), the bedrock consists of Paleogene and Oligocene sandy sediments. These may have originated from the folded ridges on Kotel’ny Island. Therefore, similar heavy mineral associations are found at sites that are 100–200 km away from each other.
The goals for the mineralogical studies on the Bykovsky Peninsula and the adjacent Khorogor Valley was to determine how Yedoma Ice Complex sediments were transported from the Kharaulakh Range to the foreland. Most of the heavy mineral fractions studied here (except for MIS 3 and MIS 2 fine fractions from Ivashkina Lagoon) are characterized by high clinopyroxene contents (31–78%) and quite low amphibole contents (5–13%). Also remarkable are the comparatively low quartz contents of 2–7%. This distinguishes these sediments from most of the other sites studied in the New Siberian Archipelago and at the coasts of the Dmitry Laptev Strait. The high pyroxene and higher titaniferous mineral contents in the Khorogor Valley and in the two Yedoma Ice Complex sites (Mamontovy Khayata and Bykovsky North) indicate that the sediment source for the late Pleistocene sediments were permo-carboniferous sandstones and slates from the Kharaulakh Range (Siegert et al., 2002; Slagoda, 2004). However, there are higher amphibole contents in the Yedoma Ice Complex sediments than in the Khorogor Valley deposits, which may indicate input from an additional sediment source. In the Ivashkina Lagoon prove different mineral associations and coefficients of the fine fraction different sources of the parent material and distinct changes during the different stages of formation (Yedoma deposition, thermokarst formation, lagoon formation).
In the Lena Delta, the MIS 3 Sand-Yedoma Ice Complex profile from Kurungnakh Island differs significantly within the profile as well as from the other two more western sites (Khardang, Nagym) regarding ilmenite, pyroxene, amphibole, and garnet contents (Figure 5). On the other hand, the sites in the west of the delta differ little within their profiles or from each other despite their different genesis. Except for the MIS 3 Yedoma Ice Complex and the Holocene thermokarst deposits from Kurungnakh Island, all other sites show mineral associations strongly reminiscent of Lena River deposits (Schwamborn et al., 2002; Schirrmeister et al., 2003a). However, the composition of the MIS 3 Yedoma Ice Complex and the Holocene thermokarst deposits from Kurungnakh Island are similar to the sediments originating from the southern Chekanovsky Ridge, mainly because of the high garnet content (Schirrmeister et al., 2003a). The Chekanovsky Ridge consists of Mesozoic sandstones and slates in a sequence of overthrusted imbricate synclines (Mikulenko, 1996).
At the Mamontovy Klyk site in the west of the study region, the mineral associations and coefficients are dominated by epidote, pyroxene, and amphibole and are relatively uniform within the profile as well as in the fine and the coarse fractions (Figure 6). However, there are minor differences between the MIS 3, the MIS 2 and the MIS 1 sediments. The weathering and soil formation processes and the source areas did not change significantly during the deposition of the different units. The source area, the Pronchshishev Ridge, is located to the south and is composed of Mesozoic sandstones and siltstones. We assume that the source area has not changed significantly over time, but it is possible that transport processes and pathways changed somewhat. In addition, the Holocene units resemble mainly MIS 2 Yedoma Ice Complex deposits from which thawed and re-located built the Holocene sequences.
Many of the sections do not differ clearly in their mineral coefficients; values remain in a narrow range. Small scale variations might be explained by hydrodynamic sorting rather than indicating source rock change. The Bol’shoy Lyakhovsky section is the site with the largest number of samples and the longest record; stretching over two glacial-interglacial cycles, exemplarily demonstrating the coefficient range in the study area. Even though the coefficient span, e.g., in the ZTR, is greatest here among all sites, the mineral spectrum does not change over time and sediments in the area have likely been remobilized in varying depositional environments (i.e., floodplain, aggrading polygonal tundra soils, thermokarst lakes). We assume that this is also the case with other sites around the Laptev Sea margin.
In general, Q/Fs coefficients are <1 for all studied sites. This agrees with experimental studies of Konishchev and Rogov (1993) showing a quartz enrichment in silt when compared with feldspar and which is interpreted to be typical for permafrost terrain with frequent freeze/thaw cycling.
Our results show that local heavy and light mineral spectra are relatively constant over time in the region, although the mineral proportions individually vary from site to site. This finding strongly suggest that local bedrock properties drive the composition of deposits as opposed to long-range transported contributions.
General Overview From the Statistics
We performed an ANOVA on the association of heavy minerals in the sediment samples to allow quantification of the differences in sample sets with regard to the stratigraphic units. If the stratigraphy can be clearly distinguished at a site, we assume that sedimentary conditions were different or that sediments were altered after sedimentation by different processes.
Tukey Honest Significant Differences Test (Tukey, 1949) was used for multiple comparisons of mineral content means averaged according to site and stratigraphy of sample mineral associations. Figure 7 summarizes the comparison of stratigraphic-wise means of the mineral associations of the fine fraction, where each block table refers to one sample site. Minerals with significantly different means among the compared stratigraphic units are shown with different background coloring in the table.
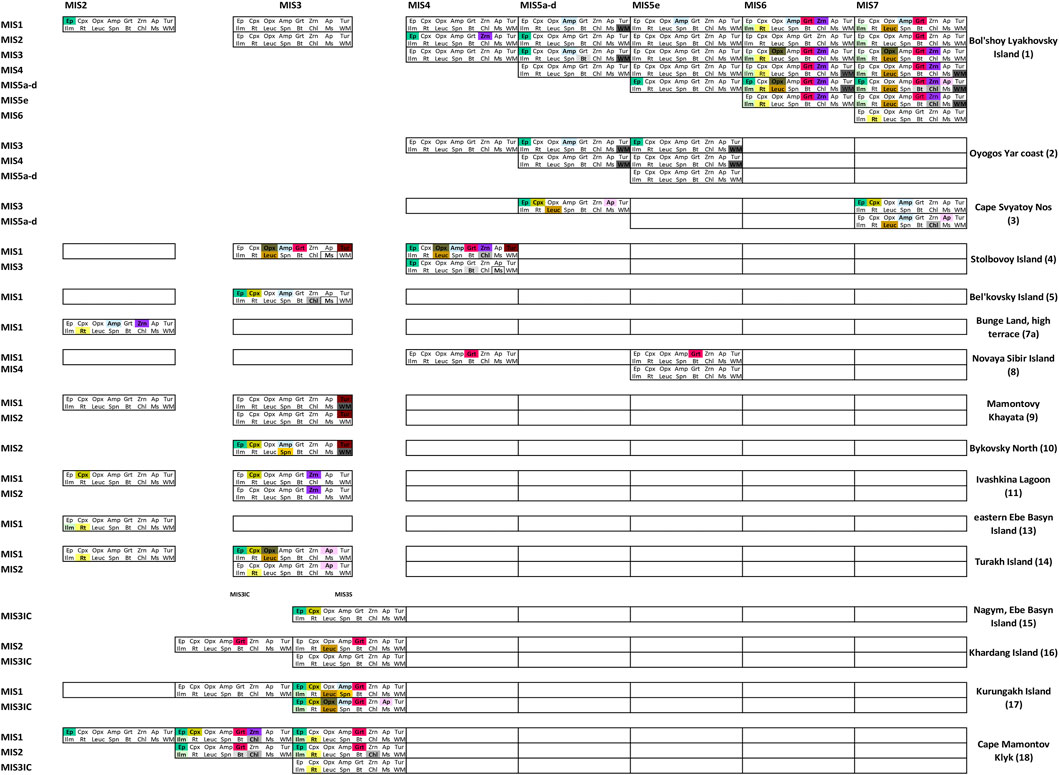
FIGURE 7. Comparison of stratigraphic-wise mean values of mineral associations of the fine fraction from sites (site numbers according to Figure 2) with sufficient number of samples. using the Tukey Honest Significant Differences Test (Tukey, 1949). The background coloring shows minerals with significantly different means among the compared stratigraphic units. (Ep—epidote, Cpx—clinopyroxene, Opx—orthopyroxene, Amp—amphibole, Grt—garnet, Zrn—zircon, Ap—apatite, Tur—tourmaline, Ilm—ilmenite, Rt—rutile, Leuc—leucoxene, Spn—sphene, Bt—biotite, Chl—chlorite, Ms—muscovite, WM—weathered mica).
For the Oyogos Yar coast, Bunge Land high terrace, the Novaya Siber Island, Mamontovy Khayata, Ivashkina Lagoon, Eastern Ebe Basyn Island, Nagym (southern Ebe Basyn Island), and Khardang Island, the ANOVA analysis shows that no clear separation of the stratigraphy by heavy mineral content is possible. This implies that sedimentary conditions during the different ages and source material were somewhat similar is at these sites.
For Bol’shoy Lyakhovsky Island, the ANOVA analysis demonstrates that both MIS 6 and MIS 7 are significantly different from all other stratigraphic units, but cannot be distinguished from each other. The other stratigraphic units also do not show strong distinctions from each other. In contrast, at Cape Svyatoy Nos, all studied stratigraphic units (MIS 3, MIS 5a, MIS 7) are distinguishable from each other. At Stolbovoy Island, the MIS 1 mineral composition is clearly different from those of MIS 3 and MIS 4, while MIS 3 and MIS 4 are similar to each other. For Bel’kovsky Island and Bykovsky North, MIS 2 and MIS 3 mineral associations can be differentiated. For Turakh Island, MIS 1 has no clear distinction from MIS 2, and MIS 2 in turn has no clear distinction from MIS 3. MIS 1 and MIS 3, however, are clearly distinguished from each other. The Kurungakh Island sediments from MIS 3 S can be distinguished from MIS 3 IC and MIS 1 sediments through their fine heavy mineral content, while there is no apparent difference between MIS 1 and MIS 3 IC indicating that MIS 1 deposits here formed from re-deposited MIS 3 deposits. For Cape Mamontov Klyk, MIS 1 and MIS 2 are indistinguishable, similar to MIS 3 IC and MIS 3S. However, both MIS 1and MIS 2 are clearly different from MIS 3 IC and MIS 3S.
In general, ANOVA shows that the heavy mineral contents contributing to a statistically significant separation of stratigraphic units are mainly epidote, garnet, ilmenite, zircon, and weathered mica. Weathered mica content seems to gain importance with increasing age of the samples.
In order to assess the implications of differences between stratigraphic units and sites, a cluster analysis was performed on age means of mineral associations for the heavy and light minerals in the fine fraction. Clusters containing one sample site each would indicate that the geographical location dominates the similarities between mineral associations. Clusters containing one stratigraphic unit each would indicate that the stratigraphic unit of the sediments dominated the mineral association and in turn the sedimentary conditions. Eight clusters were extracted from the dendrogram in Supplementary Figure S3 for the fine fraction heavy mineral associations, using a combination of cluster significance and height.
The clusters do not show a clear differentiation by stratigraphic units for the fine heavy mineral associations, but they highlight site-specific relationships and differences.
The three stratigraphic units from Cape Svyatoy Nos (MIS 3, MIS 5a-d, MIS 7) could not be assigned to any cluster.
The Stolbovoy Island MIS 3 and MIS 4 deposits form cluster 1, along with Bel’kovsky Island MIS 3. Cluster 4 contains MIS 1 from both Bel’kovsky Island and Stolbovoy Island.
Cluster 2 consists of all stratigraphic units from Bunge Land (Bunge Land, low terrace MIS 1, Bunge Land, high terrace MIS 1 and MIS 2), Turakh Island (MIS 1, MIS 2, and MIS 3), eastern Ebe Basyn Island (MIS 1, MIS 2) and Nagym, Ebe Basyn Island (MIS 3S, MIS 3 IC). Additionally, this cluster contains Novaya Sibir Island (MIS 5e), Khardang Island (MIS 2 and MIS 3 S), and Kurungnakh Island (MIS 3 S). Both other stratigraphic units of Kurungnakh Island (MIS 1, MIS 3 IC) form cluster 8, while two other units from Novaya Sibir Island (MIS 4, MIS 1) cannot be assigned to any cluster. The third stratigraphic unit from Khardang Island (MIS 3 IC) is assigned to cluster 3.
In addition, cluster 3 contains all stratigraphic units from Cape Mamontov Klyk (MIS 1, MIS 2, MIS 3 IC, MIS 3 S), Mys Anisii, Kotel’ny Island (MIS 2), Oyogos Yar (MIS 5a–d and MIS 5e) and Bykovsky North (MIS 2). Oyogos Yar MIS 3 and MIS 4 cannot be assigned to a cluster. Bykovsky North MIS 3 is contained in cluster 6.
Cluster 5 contains Ivashkina Lagoon MIS 2 and MIS 3 deposits, while MIS 1 could not be assigned to any cluster. Cluster 6 contains all stratigraphic units from Mamontovy Khayata (MIS 1, MIS 2, and MIS 3) together with Bykovsky North (MIS 3).
Cluster 7 contains five stratigraphic units from Bol’shoy Lyakhovsky (MIS 1, MIS 3, MIS 4, MIS 5a–d, MIS 5e). The other three stratigraphic units from Bol’shoy Lyakhovsky (MIS 2, MIS 6, MIS 7) could not be assigned.
Aside from Mamontov Klyk, where ANOVA suggests a separation between MIS 1 + MIS 2 and MIS 3 IC + MIS 3 S that is not reflected in the cluster analysis, all separations shown in the ANOVA are also present in the cluster analysis, sorting the different units into different clusters. Additionally, units from Novaya Sibir Island and Ivashkina Lagoon are sorted into different clusters, reflecting the differences apparent from the means shown in Supplementary Figures S3 and S4 that ANOVA cannot detect due to too few samples.
The fine light mineral associations can be separated into four clusters (Supplementary Figure S4). Ten stratigraphic units from different sites could not be assigned. Cluster 1 contains Cape Svyatoy Nos MIS 3 and Bol’shoy Lyakhovsky MIS 4 associations. Bol’shoy Lyakhovsky MIS 5a–d, MIS 5e, and MIS 6 associations could not be assigned to a cluster, while MIS 1, MIS 2, and MIS 3 associations are contained in cluster 4. Cape Svyatoy Nos MIS 5a–d and MIS 7 associations could not be assigned to any cluster. Novaya Sibir Island MIS 4 and MIS 5a–d associations form cluster 2 along with Turakh Island MIS 3. Turakh Island MIS 1 and MIS 2 associations are contained in cluster 3 along with eastern Ebe Basyn Island MIS 1 and MIS 2, Khardang Island MIS 3c and MIS 3 IC, and Bol’shoy Lyakhovsky MIS 7 associations. In addition to the stratigraphic units from Bol’shoy Lyakhovsky listed above, cluster 4 contains all stratigraphic units from Ivshkina Lagoon (MIS 1, MIS 2, and MIS 3), Khorogor Valley MIS 1, both Bykovsky North MIS 2 and MIS 3, all stratigraphic units from Mamontovy Khayata (MIS 1, MIS 2, and MIS 3), and Khardang Island MIS 2. All three stratigraphic units from Cape Mamontov Klyk with light mineral records (MIS 1, MIS 2, and MIS 3 IC) are outside of all clusters. Novaya Sibir Island MIS 1 could also not be assigned to a cluster.
Cluster associations from the fine light minerals fraction are distinctly different from those of the fine heavy minerals fraction. However, they also indicate that there is no clear differentiation by stratigraphic units at certain sites or by geographic location of the study sites.
The cluster analyses of the fine heavy and light mineral associations contain clusters that explain a local genetic association with the same source rocks (Bykovsky Peninsula, Lena Delta). There are also clusters where the site units are several hundred kilometers distant from each other. Furthermore, close correlations between the MIS 3 Yedoma units and the MIS 1 thermokarst units were often evident. This supports the hypothesis that the mineral composition of Holocene deposits in most cases derives from thawed and redeposited Yedoma Ice Complex deposits.
Generally, the mineral compositions are results of several process factors. These include the source rock, the sedimentation conditions, the duration of frost weathering after deposition in the active layer, and the impact of post-sedimentary soil formation processes.
Regional and Methodical Context
Finally, we have compared the best studied heavy mineral fraction (63–125 µm) in the most widespread stratigraphic units (MIS 3, 2 and 1) (Figure 8). In general, this regional comparison demonstrates that heavy mineral contents are specific to the sample sites within similar sedimentary context, which indicates that local processes determine the heavy mineral content. The heavy mineral associations of some neighboring sites are similar within limits for certain stratigraphic units.
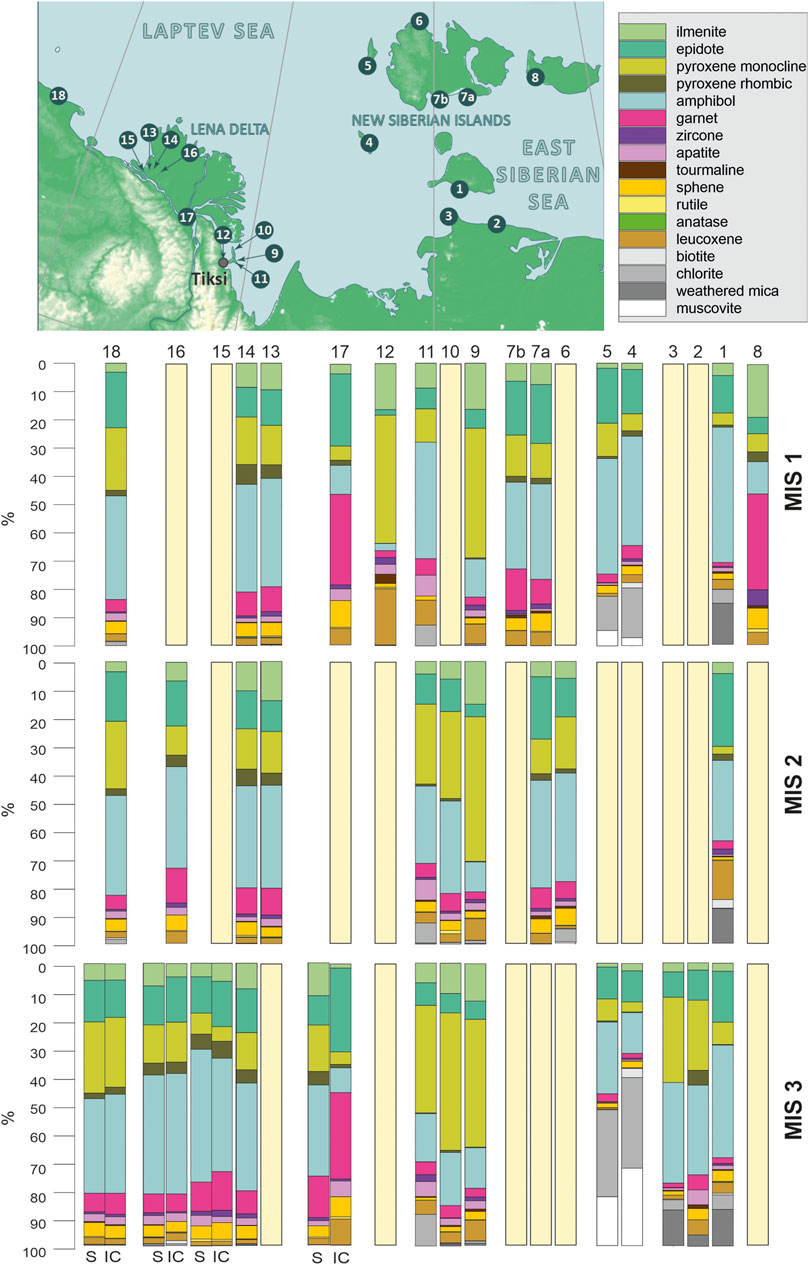
FIGURE 8. Regional comparison of the best studied heavy minerals association (63–125 µm) in the most widespread stratigraphic units (MIS 3, 2 and 1). For the light-yellow boxes are no data available. The map is a cutout from the map of Figure 1. (1—Bol’shoy Lyakhovsky Island; 2—Oyogos Yar coast; 3—Cape Syatoy Nos; 4—Stolbovoy Island; 5—Bel’kovsky Island; 6—Cape Anisii, Kotel’ny Island; 7—Bunge Land (a—higher terrace, b—lower terrace); 8—Novaya Sibir’ Island. Bykhovsky Peninsula: 9—Mamontovy Khayata; 10—Bykovsky North; 11—Ivashkina Lagoon; 12—Khorogor Valley. Lena Delta: 13—eastern Ebe Basyn Island; 14—Turakh Island; 15—Nagym, southern Ebe Basyn Island; 16—Khardang Island; 17—Kurungnakh Island; 18—Cape Mamontov Klyk).
For MIS 3, the Laptev Strait sites (Figure 8, study sites 1–3), the Stolbovoy and Belkovsky islands (study sites 4 and 5), Bykovsky Island (study sites 9–11); and the Kurungnakh sands and all other sites in the Lena Delta and Mamontov Klyk (study sites 17S, 13–18) are relatively similar.
Not as many data sets are available for MIS 2. Here the sites of Cape Anissi on Kotel’ny Island and from the higher terrace of Bunge Land (study sites 6 and 7a), on the Bykovsky Peninsula the sites Bykovsky North and Ivashkina Laguna (study sites 10 and 11) and in the Lena Delta the sites eastern Ebe Basyn Island, Tuarakh Island and Khardang Island as well as further west Cape Mamontov Klyk are similar.
For MIS 1, there are relative similarities at Stolbovoy and Belkovsky Islands (study sites 4, 5), at the two sites on Bunge Land (study sites 7a, 7b), at Tuarakh Island and eastern Ebe Sise Island in the Lena Delta. The sites at Novaya Sibir' Island and Kurungnakh Island (MIS 1 and MIS 3 Ice Complex) stand out primarily because of their high garnet contents. In the Lena Delta, the MIS 3 sand and Yedoma Ice Complex deposits of study sites 13–16 have a similar heavy mineralogical signature (Figure 8). It is similar to the sediments of the Lena River (Schwamborn et al., 2002; Schirrmeister et al., 2011a). While the MIS 3 Ice Complex deposits from Kurungnakh Island (study site 17) were formed by proluvial sediments from Chekanovsky Ridge which accumulated on a flat foreland plain in front of the mountain range (Schirrmeister et al., 2011a). The MIS 1 deposits from this site are similar to the MIS 3 sediments as they were formed from the redeposited Yedoma Ice Complex sediments.
Stolbovoy and Belkovsky islands (Figure 8, study sites 4 and 5) are characterized by high mica content compared to all other sites. This could be caused by changes in heavy mineral content dominated by mica weathering which leads to higher percentage of all other heavy minerals in MIS1 compared to MIS3. On Bykovsky Peninsula the development of Mamontovy Khayata (study site 9) from MIS3 to MIS1 show very small changes, while for the Ivashkina Lagoon (study site 11), MIS3 and MIS2 are similar, but MIS1 looks very differently. This is due the Holocene lagoon impact.
Over many decades there has been a debate on how to interpret the origin of the Yedoma deposits. Transport via aeolian drift over long distances has been proposed to explain the silty to sandy sediment load that has built up the several meters to tens of meters high Yedoma uplands in the otherwise flat Northern Siberian lowland (e.g., Tomirdiaro, 1996; Murton et al., 2015). In contrast, other studies favored local sediment sources and dynamics delivering the detritus (e.g., Kunitsky et al., 2002; Schirrmeister et al., 2020). While a number of studies have described heavy mineral associations of Arctic sediments both from the Laptev Sea region (Siegert et al., 2002; Siegert et al., 2009; Schwamborn et al., 2002; Schirrmeister et al., 2003a; Schirrmeister et al., 2008; Schirrmeister et al., 2010; Schirrmeister et al., 2011b) and the neighboring shelf sea areas (Behrends et al., 1999; Peregovich et al., 1999; Kaparulina et al., 2018), our study presents the first comprehensive analysis of heavy mineral associations as a proxy for provenance. This analysis is restricted methodically to sand fractions, due to weathering processes that alter the silt fractions and the fact that in the silt fractions, the minerals cannot be identified anymore. Therefore, this analysis allows no direct conclusions on the origins of the Yedoma sediment silt fraction, where a homogeneous heavy mineral association would fit the long-range aeolian transport hypothesis, while heterogeneous associations would be consistent with local and polygenetic origin of the sediments. In accordance with the grain size distribution analysis presented in Schirrmeister et al., 2020, our results demonstrate a polygenetic origin of coarse grain Yedoma sediments, which make up 5–50% of the Yedoma deposits.
Perhaps in the future, mineralogical studies of the finer fractions of the Yedoma deposits could help to further clarify the genesis of this Arctic phenomenon.
Conclusion
At most of our 18 study sites in Northeast Siberia (except on Bunge Land) the studied heavy and light mineral grains of more than 450 samples from a wide range of Late Quaternary permafrost deposits are weakly rounded, rounded to subangular, and angular. For the fine sand fraction this finding indicates that the sediments did not experience long transport distances.
Within single outcrop profiles we found clear differences, but also relatively similar mineral associations in stratigraphic units of different ages. We suggest two possible scenarios resulting in this:
(1) the mineral source areas and/or transport mechanisms and distances changed over time for a distinct site, resulting in clear differences in the mineral composition of stratigraphic units of different ages (such as on Bol’shoy Lyakhovsky Island); and
(2) the mineral source area did not change over time and the main transport mechanism and distance remained the same resulting in rather similar mineral associations at distinct sites (such as on Bykovsky Peninsula). In addition, there are distinct regional differences in the heavy mineral associations that mainly reflect differences in provenance.
Mineral associations of the same age differ significantly between different sites, not in their spectra but in their mineral proportions. This means that different sites had different source rocks. However, there are also some sites with similar mineral associations that are sometimes dozens or hundreds of kilometers away from each other, but that had clearly different formation periods and conditions. In these cases, the source rocks, like sandstones or slates, were somewhat similar in composition.
Cluster associations of the fine light mineral fraction are distinctly different from those of the fine heavy mineral fraction. The light minerals are much more subject to frost weathering and are therefore more likely to indicate the intensity of these processes. The heavy minerals, on the other hand, provide information on the provenance areas.
Our study of heavy and light mineral associations from permafrost deposits helps disentangling periglacial sediment dynamics in Northeast Siberia. In particular for the late Pleistocene Yedoma Ice Complex deposits, our findings suggest a strong local sediment source for individual sites, in agreement with dominance of periglacial weathering and local transport processes (including local aeolian relocation) instead of solely far-ranging transport and mixing during aeolian loess deposition.
Data Availability Statement
Several datasets presented in this study can be found in online repositories. The names of the repository/repositories and accession number(s) can be found below: https://doi.pangaea.de/10.1594/PANGAEA.879589 and https://doi.org/10.1594/PANGAEA.879592. Further inquiries can be directed to the corresponding author.
Author Contributions
LS and CS have designed the long-term studies. LS, CS, GG, SW, and VK participated in the field work and performed the cryolithological and stratigraphical investigations. IK has done large parts of microscopic analysis. HM conducted the statistical analyses. All authors contributed in writing and editing the manuscript.
Funding
This work has been supported by the Deutsche Forschungsgemeinschaft (DFG grants No. WE4390/7-1 to SW).
Conflict of Interest
The authors declare that the research was conducted in the absence of any commercial or financial relationships that could be construed as a potential conflict of interest.
The handling editor is currently organizing a Research Topic with one of the authors LS.
Publisher’s Note
All claims expressed in this article are solely those of the authors and do not necessarily represent those of their affiliated organizations, or those of the publisher, the editors and the reviewers. Any product that may be evaluated in this article, or claim that may be made by its manufacturer, is not guaranteed or endorsed by the publisher.
Acknowledgments
First and foremost, we thank Olga Babiy of the Melnikov Permafrost Institute for conducting the analyses in the very early years. We thank our colleagues from the Hydrobase Tiksi for help with fieldwork from 1998 to 2007, which was supported by the joint Russian-German System Laptev Sea projects. Sample preparations were done in the AWI Potsdam Sediment Laboratory. We thank Ute Bastian/Kuschel and Dyke Scheidemann for their support. The manuscript benefited from English language corrections by Candace O’Connor (Fairbanks, Alaska).
Supplementary Material
The Supplementary Material for this article can be found online at: https://www.frontiersin.org/articles/10.3389/feart.2022.741932/full#supplementary-material
References
Andreev, A. A., Grosse, G., Schirrmeister, L., Kuznetsova, T. V., Kuzmina, S. A., Bobrov, A. A., et al. (2009). Weichselian and Holocene Palaeoenvironmental History of the Bol'shoy Lyakhovsky Island, New Siberian Archipelago, Arctic Siberia. Boreas 38 (1), 72–110. doi:10.1111/j.1502-3885.2008.00039.x
Andreev, A., Grosse, G., Schirrmeister, L., Kuzmina, S., Novenko, E., Bobrov, A., et al. (2004). Late Saalian and Eemian Palaeoenvironmental History of the Bol'shoy Lyakhovsky Island (Laptev Sea Region, Arctic Siberia). Boreas 33 (4), 319–348. doi:10.1080/03009480410001974
Astakhov, V. I. (2013). Pleistocene Glaciations of Northern Russia - a Modern View. Boreas 42, 1–24. doi:10.1111/j.1502-3885.2012.00269.x
Astakhov, V., and Mangerud, J. (2005). The Age of the Karginsky Interglacial Strata on the Lower Yenisei. Doklady Earth Sci. 403, 673–676.
Behrends, M., Hoops, E., and Peregovich, B. (1999). “Distribution Patterns of Heavy Minerals in Siberian Rivers, the Laptev Sea and the Eastern Arctic Ocean: An Approach to Identify Sources, Transport and Pathways of Terrigenous Matter,” in Land-Ocean Systems in the Siberian Arctic. Editor H. H. Kassens (Berlin, Heidelberg: Springer), 265–286. doi:10.1007/978-3-642-60134-7_24
Biskaborn, B. K., Smith, S. L., Noetzli, J., Matthes, H., Vieira, G., Streletskiy, D. A., et al. (2019). Permafrost Is Warming at a Global Scale. Nat. Commun. 10, 264. doi:10.1038/s41467-018-08240-4
Blinov, A., Alfimov, V., Beer, J., Gilichinsky, D., Schirrmeister, L., Kholodov, A., et al. (2009). Ratio of36Cl/Cl in Ground Ice of East Siberia and its Application for Chronometry. Geochem. Geophys. Geosyst. 10 (1), a–n. doi:10.1029/2009GC002548
Boenigk, W. (1983). Schwermineralanalyse. Stuttgart, Germany: Ferdinand Enke Publishers, 158. in German.
Box, J. E., Colgan, W. T., Christensen, T. R., Schmidt, N. M., Lund, M., Parmentier, F.-J. W., et al. (2019). Key Indicators of Arctic Climate Change: 1971-2017. Environ. Res. Lett. 14 (4), 045010. doi:10.1088/1748-9326/aafc1b
Fessenden, F. W. (1959). Removal of Heavy Liquid Separates from Glass Centrifuge Tubes. J. Sed. Petrol. 29, 269–280. doi:10.2110/jsr.28.621
French, H., and Shur, Y. (2010). The Principles of Cryostratigraphy. Earth-Science Rev. 101, 190–206. doi:10.1016/j.earscirev.2010.04.002
Gilichinsky, D. A., Nolte, E., Basilyan, A. E., Beer, J., Blinov, A. V., Lazarev, V. E., et al. (2007). Dating of Syngenetic Ice Wedges in Permafrost with 36Cl. Quat. Sci. Rev. 26, 1547–1556. doi:10.1016/j.quascirev.2007.04.004
Giterman, R. E., Sher, A. V., and Matthews, J. V. (1982). “Comparison of the Development of Tundra-Steppe Environments in West and East Beringia: Pollen and Macrofossil Evidence from Key Sections,” in Paleoecology of Beringia. Editors D. M. Hopkins, J. V. Matthews, C. E. Schwerger, and S. B. Young (NY, London: Academy Press), 43–73. doi:10.1016/b978-0-12-355860-2.50011-9
Grosse, G., Schirrmeister, L., Kunitsky, V., and Dereviagyn, A. (2003). “Periglacial Features Around Tiksi,” in Russian-German Cooperation System Laptev Sea - the Expedition Lena 2002. Editors M. N. Grigoriev, V. Rachold, D. Bolshiyanov, E. M. Pfeiffer, L. Schirrmeister, D. Wagneret al. (Bremerhaven: Reports on Polar and Marine Research), 137–191. doi:10.2312/BzPM_0466_2003
Grosse, G., Schirrmeister, L., Siegert, C., Kunitsky, V. V., Slagoda, E. A., Andreev, A. A., et al. (2007). Geological and Geomorphological Evolution of a Sedimentary Periglacial Landscape in Northeast Siberia during the Late Quaternary. Geomorphology 86 (1/2), 25–51. doi:10.1016/j.geomorph.2006.08.005
John F. Hubert, J. F. (1962). A Zircon-Tourmaline-Rutile Maturity index and the Interdependence of the Composition of Heavy mineral Assemblages with the Gross Composition and Texture of Sandstones. Sepm Jsr 32 (3), 440–450. doi:10.1306/74D70CE5-2B21-11D7-8648000102C1865D
Kaparulina, E., Junttila, J., Strand, K., and Lunkka, J. P. (2018). Provenance Signatures and Changes of the Southwestern Sector of the Barents Ice Sheet during the Last Deglaciation. Boreas 47, 522–543. doi:10.1111/bor.12293
Kaplina, T. N. (1981). “History of Frozen Ground in Northern Yakutia during the Late Cenozoic,” in History of Permafrost Development in Eurasia. Editors V. V. Baulin, and I. Dubikov (Moscow: Nauka Press), 153–181. in Russian.
Kaplina, T. N. (1989). “Stages of Formation of Geocryological Conditions,” in Geocryology of the USSR, East Siberia and the Far East. Editor E. D. Yershov (Moscow: Nedra), 20–25. in Russian.
Kaplina, T. N., and Lozhkin, A. V. (1984). “Age and History of Accumulation of the "Ice Complex" of the Maritime Lowlands of Yakutiya,” in Late Quaternary Environments of the Soviet Union. Editors A. A. Velichko, H. E. Wright, and C. W. Barnosky (University of Minnesota Press, MN), 147–151.
Katasonov, E. M. (1955). Lithology of Frozen Quaternary Sediments (Cryolithology) of the Yana Coastal lowland. Moscow: Author's abstract of the Candidate's thesis, 25.
Konishchev, V. N. (1982). Characteristics of Cryogenic Weathering in the Permafrost Zone of the European USSR. Arctic Alpine Res. 14 (3), 261–265. doi:10.2307/1551158
Konishchev, V. N., and Rogov, V. V. (1993). Investigations of Cryogenic Weathering in Europe and Northern Asia. Permafrost Periglac. Process. 4, 49–64. doi:10.1002/ppp.3430040105
Kunitsky, V., Schirrmeister, L., Grosse, G., and Kienast, F. (2002). Snow Patches in Nival Landscapes and Their Role for the Ice Complex Formation in the Laptev Sea Coastal Lowlands. Polarforschung 70, 53–67. doi:10.2312/polarforschung.70.53
Lisiecki, L. E., and Raymo, M. E. (2005). A Pliocene-Pleistocene Stack of 57 Globally Distributed Benthic δ18O Records. Paleoceanography 20, a–n. doi:10.1029/2004PA001071
Mange, M. A., and Maurer, H. F. W. (1992). Heavy Minerals in Colour. London: Chapman & Hall, 147. doi:10.1007/978-94-011-2308-2
Marcinkowski, B., and Mycielska-Dowgiałło, E. (2013). Heavy-mineral Analysis in Polish Investigations of Quaternary Deposits: a Review. Geologos 19 (1–2), 5–23. doi:10.2478/logos-2013-0002
Mikulenko, K. I. (1996). “Questions of the Geology of the Arctic Region of West Yakutia,” in Geology, Seismicity and Permafrost Processes of the Arctic Region of West Yakutia (Yakutsk, Russia: Scientific Center SD RAS). in Russian.
Morton, A. C., and Hallsworth, C. R. (1999). Processes Controlling the Composition of Heavy mineral Assemblages in Sandstones. Sediment. Geology. 124, 3–29. doi:10.1016/S0037-0738(98)00118-3
Murton, J. B., Goslar, T., Edwards, M. E., Bateman, M. D., Danilov, P. P., Savvinov, G. N., et al. (2015). Palaeoenvironmental Interpretation of Yedoma silt (Ice Complex) Deposition as Cold-Climate Loess, Duvanny Yar, Northeast Siberia. Permafrost Periglac. Process. 26, 208–288. doi:10.1002/ppp.1843
Murton, J. B. (2021). What and where Are Periglacial Landscapes? Permafrost and Periglac Process 32, 186–212. doi:10.1002/ppp.2102
Nesbitt, H. W., Young, G. M., McLennan, S. M., and Keays, R. R. (1996). Effects of Chemical Weathering and Sorting on the Petrogenesis of Siliciclastic Sediments, with Implications for Provenance Studies. J. Geology. 104 (5), 525–542. doi:10.1086/629850
Nitzbon, J., Westermann, S., Langer, M., Martin, L. C. P., Strauss, J., Laboor, S., et al. (2020). Fast Response of Cold Ice-Rich Permafrost in Northeast Siberia to a Warming Climate. Nat. Commun. 11, 2201. doi:10.1038/s41467-020-15725-8
Peregovich, B., Hoops, E., and Rachold, V. (1999). Sediment Transport to the Laptev Sea (Siberian Arctic) during the Holocene - Evidence from the Heavy mineral Composition of Fluvial and marine Sediments. Boreas 28, 205–214. doi:10.1111/j.1502-3885.1999.tb00215.x
Pisarska-Jamroży, M., van Loon, A. J., and Woronko, B. (2015). Sorting of Heavy Minerals in Sediments Deposited at a High Accumulation Rate, with Examples from Sandurs and an Ice-Marginal valley in NW Poland. GFF 137 (2), 126–140. doi:10.1080/11035897.2015.1009158
Popov, A. I. (1967). Cryolithogenesis as a Process of Lithogenesis. Underground Ice, Issue 3. Moscow: Moscow State University, Geographical Faculty, Department of Polar Lands and Glaciology, 7–25.
Popov, A. I. (1953). Peculiarities of Lithogenesis of Alluvial plains under Harsh Climate Conditions. Bulletin of the Academy of Sciences of the USSR Geographic Series. No 2, 29–41. doi:10.1007/bf01188237
R Core Team (2021). “R: A Language and Environment for Statistical Computing,” in R Foundation for Statistical Computing (Vienna: Springer).
Reineck, H.-E., and Singh, I. B. (1980). Depositional Sedimentary Environments: With Reference to Terrigenous Clastics. Berlin, Heidelberg, New York: Springer, 551.
Schirrmeister, L., Dietze, E., Matthes, H., Grosse, G., Strauss, J., Laboor, S., et al. (2020). The Genesis of Yedoma Ice Complex Permafrost - Grain-Size Endmember Modeling Analysis from Siberia and Alaska. E&g Quat. Sci. J. 69, 33–53. doi:10.5194/egqsj-69-33-2020
Schirrmeister, L., Froese, D., Tumskoy, V., Grosse, G., and Wetterich, S. (2013). “Permafrost and Periglacial Features | Yedoma: Late Pleistocene Ice-Rich Syngenetic Permafrost of Beringia,” in The Encyclopedia of Quaternary Science. Editor S. A. Elias (Amsterdam: Elsevier), 542–552. doi:10.1016/B978-0-444-53643-3.00106-0
Schirrmeister, L., Grosse, G., Kunitsky, V., Magens, D., Meyer, H., Dereviagin, A., et al. (2008). Periglacial Landscape Evolution and Environmental Changes of Arctic lowland Areas for the Last 60 000 Years (Western Laptev Sea Coast, Cape Mamontov Klyk). Polar Res. 27 (2), 249–272. doi:10.1111/j.1751-8369.2008.00067.x
Schirrmeister, L., Grosse, G., Kunitsky, V., Meyer, H., Derivyagin, A., and Kuznetsova, T. (2003b). “Permafrost, Periglacial and Paleo-Environmental Studies on New Siberian Islands,” in Russian-German Cooperation System Laptev Sea - the Expedition Lena 2002 (Bremerhaven: Reports on Polar and Marine Research), 195–265. doi:10.2312/BzPM_0466_2003
Schirrmeister, L., Grosse, G., Kunitsky, V. V., Fuchs, M. C., Krbetschek, M., Andreev, A. A., et al. (2010). The Mystery of Bunge Land (New Siberian Archipelago): Implications for its Formation Based on Palaeoenvironmental Records, Geomorphology, and Remote Sensing. Quat. Sci. Rev. 29, 3598–3614. doi:10.1016/j.quascirev.2009.11.017
Schirrmeister, L., Grosse, G., Schnelle, M., Fuchs, M., Krbetschek, M., Ulrich, M., et al. (2011a). Late Quaternary Paleoenvironmental Records from the Western Lena Delta, Arctic Siberia. Palaeogeogr. Palaeoclimatol. Palaeoecol. 299, 175–196. doi:10.1016/j.quascirev.2009.11.01710.1016/j.palaeo.2010.10.045
Schirrmeister, L., Grosse, G., Schwamborn, G., Andreev, A. A., Meyer, H., Kunitsky, V. V., et al. (2003a). Late Quaternary History of the Accumulation Plain North of the Chekanovsky Ridge (Lena Delta, Russia): A Multidisciplinary Approach. Polar Geogr. 27 (4), 277–319. doi:10.1080/789610225
Schirrmeister, L., Kunitsky, V., Grosse, G., Wetterich, S., Meyer, H., Schwamborn, G., et al. (2011b). Sedimentary Characteristics and Origin of the Late Pleistocene Ice Complex on North-East Siberian Arctic Coastal Lowlands and Islands - a Review. Quat. Int. 241, 3–25. doi:10.1016/j.quaint.2010.04.004
Schirrmeister, L., Kunitsky, V., Grosse, V., Meyer, H., Kuznetsova, T., Kuzmina, S., et al. (2000). Quaternary Deposits of Bol'shoy Lyakhovsky Island. Rep. Polar Res. 354, 113–168. doi:10.2312/BzP_0354_1_2000
Schirrmeister, L., Siegert, C., Kunitzky, V. V., Grootes, P. M., and Erlenkeuser, H. (2002a). Late Quaternary Ice-Rich Permafrost Sequences as a Paleoenvironmental Archive for the Laptev Sea Region in Northern Siberia. Int. J. Earth Sci. 91, 154–167. doi:10.1007/s005310100205
Schirrmeister, L., Siegert, C., Kuznetsova, T., Kuzmina, S., Andreev, A., Kienast, F., et al. (2002b). Paleoenvironmental and Paleoclimatic Records from Permafrost Deposits in the Arctic Region of Northern Siberia. Quat. Int. 89, 97–118. doi:10.1016/S1040-6182(01)00083-0
Schwamborn, G., Rachold, V., and Grigoriev, M. N. (2002). Late Quaternary Sedimentation History of the Lena Delta. Quat. Int. 89, 119–134. doi:10.1016/s1040-6182(01)00084-2
Scull, B. J. (1960). Removal of Heavy Liquid Separates from Glass Centrifuge Tubes--Alternative Method. J. Sediment. Res. 30, 626. doi:10.1306/74d70ad8-2b21-11d7-8648000102c1865d
Sher, A. V. (1971). Mammals and Pleistocene Stratigraphy of the Extreme Northeast of the USSR and North America. Moscow: Nauka Press, 344.
Siegert, C., Kunitsky, V. V., and Schirrmeister, L. (2009). “Ice Complex Deposits - A Data Archive for the Reconstruction of Climate and Ecology at the Laptev Sea Coast during the Late Pleistocene,” in System of the Laptev Sea and the Adjacent Arctic Seas - Modern and Past Environments (Moscow: Moscow University Press), 320–331.
Siegert, C., Schirrmeister, L., and Babiy, O. (2002). The Sedimentological, Mineralogical and Geochemical Composition of Late Pleistocene Deposits from the Ice Complex on the Bykovsky peninsula, Northern Siberia. Polarforschung 70 (2000), 3–11. doi:10.2312/polarforschung.70.3
Slagoda, E. A. (2004). Cryolithogenic Deposits of the Laptev Sea Coastal plain: Lithology and Micromorphology. Tyumen, Publishing and Printing Centre Express, 119.
Suzuki, R., and Shimodaira, H. (2006). Pvclust: an R Package for Assessing the Uncertainty in Hierarchical Clustering. Bioinformatics 22 (12), 1540–1542. doi:10.1093/bioinformatics/btl117
Suzuki, R., Terada, Y., and Shimodaira, H. (2019). Pvclust: Hierarchical Clustering with P-Values via Multiscale Bootstrap Resampling. Kyoto: R package version 2.2-0. http://stat.sys.i.kyoto-u.ac.jp/prog/pvclust/
Tomirdiaro, S. V. (1996). “Palaeogeography of Beringia and Arctida,” in American Beginnings (Chicago: University of Chicago), 58–69.
Tukey, J. W. (1949). Comparing Individual Means in the Analysis of Variance. Biometrics 5 (2), 99–114. doi:10.2307/3001913
Tumskoy, V. E. (2012). Peculiarities of Cryolithogenesis in Northern Yakutia from the Middle Neopleistocene to the Holocene [Особенности Криолитогенеза Oтложений Северной Якутии B Hреднем Kеоплейстоцене – Голоцене]. Earth‘ Cryosphere [Криосфера Земли] 16 (1), 12–21.
Vangenheim, E. A. (1977). Paleontological Substantiation of the Anthropogene Stratigraphy of Northern Asia. Moscow: Nauka Press, 170.
Volnov, D. A., Ivanenko, G. V., Kosko, M. K., and Lopatin, B. G. (1998). Map of Pre Quaternary Formations, S-53-55 (New Siberian Islands) State Geological Map of the Russian Federation, Scale 1: 1 000 000, Ministry of Natural Resources of the Russian Federation. Springer.
Wetterich, S., Kizyakov, A., Fritz, M., Wolter, J., Mollenhauer, G., Meyer, H., et al. (2020). The Cryostratigraphy of the Yedoma Cliff of Sobo-Sise Island (Lena delta) Reveals Permafrost Dynamics in the central Laptev Sea Coastal Region during the Last 52 Kyr. The Cryosphere 14, 4525–4551. doi:10.5194/tc-14-4525-2020
Wetterich, S., Meyer, H., Fritz, M., Mollenhauer, G., Rethemeyer, J., Kizyakov, A., et al. (2021). Northeast Siberian Permafrost Ice‐Wedge Stable Isotopes Depict Pronounced Last Glacial Maximum Winter Cooling. Geophys. Res. Lett. 48, e2020GL092087. doi:10.1029/2020GL092087
Wetterich, S., Rudaya, N., Kuznetsov, V., Maksimov, F., Opel, T., Meyer, H., et al. (2019). Ice Complex Formation on Bol'shoy Lyakhovsky Island (New Siberian Archipelago, East Siberian Arctic) since about 200 Ka. Quat. Res. 92 (2), 530–548. doi:10.1017/qua.2019.6
Wetterich, S., Rudaya, N., Tumskoy, V., Andreev, A. A., Opel, T., Schirrmeister, L., et al. (2011). Last Glacial Maximum Records in Permafrost of the East Siberian Arctic. Quat. Sci. Rev. 30, 3139–3151. doi:10.1016/j.quascirev.2011.07.020
Wetterich, S., Schirrmeister, L., Andreev, A. A., Pudenz, M., Plessen, B., Meyer, H., et al. (2009). Eemian and Late Glacial/Holocene Palaeoenvironmental Records from Permafrost Sequences at the Dmitry Laptev Strait (NE Siberia, Russia). Palaeogeogr. Palaeoclimatol. Palaeoecol. 279, 73–95. doi:10.1016/j.palaeo.2009.05.002
Wetterich, S., Tumskoy, V., Rudaya, N., Andreev, A. A., Opel, T., Meyer, H., et al. (2014). Ice Complex Formation in Arctic East Siberia during the MIS3 Interstadial. Quat. Sci. Rev. 84, 39–55. doi:10.1016/j.quascirev.2013.11.009
Wetterich, S., Tumskoy, V., Rudaya, N., Kuznetsov, V., Maksimov, F., Opel, T., et al. (2016). Ice Complex Permafrost of MIS5 Age in the Dmitry Laptev Strait Coastal Region (East Siberian Arctic). Quat. Sci. Rev. 147, 298–311. doi:10.1016/j.quascirev.2015.11.016
Woronko, B., Rychel, J., Karasiewicz, M. T., Ber, A., Krzywicki, T., Marks, L., et al. (2013). Heavy and Light Minerals as a Tool for Reconstructing Depositional Environments: an Example from the Jałówka Site (Northern Podlasie Region, NE Poland). Geologos 19 (1-2), 47–66. doi:10.2478/logos-2013-0004
Zadkova, I. I. (1973). Composition and Lithological Structure of Deposits. In.: Main Features of the Lithology of Quaternary Sediments in the Interfluve basin of the Lower Irtysh River. Novosibirsk: Nauka, 23–72.
Zimmermann, H., Raschke, E., Epp, L., Stoof-Leichsenring, K., Schirrmeister, L., Schwamborn, G., et al. (2017). The History of Tree and Shrub Taxa on Bol'shoy Lyakhovsky Island (New Siberian Archipelago) since the Last Interglacial Uncovered by Sedimentary Ancient DNA and Pollen Data. Genes 8 (10), 273. doi:10.3390/genes8100273
Keywords: mineral content, permafrost deposits, Quaternary, Yedoma, northeastern Siberia, terrestrial Arctic
Citation: Schirrmeister L, Wetterich S, Schwamborn G, Matthes H, Grosse G, Klimova I, Kunitsky VV and Siegert C (2022) Heavy and Light Mineral Association of Late Quaternary Permafrost Deposits in Northeastern Siberia. Front. Earth Sci. 10:741932. doi: 10.3389/feart.2022.741932
Received: 15 July 2021; Accepted: 14 February 2022;
Published: 09 March 2022.
Edited by:
Alexandra Veremeeva, Institute of Physical-Chemical and Biological Problems in Soil Science (RAS), RussiaReviewed by:
Barbara Woronko, University of Warsaw, PolandDenis Shmelev, Lomonosov Moscow State University, Russia
Copyright © 2022 Schirrmeister, Wetterich, Schwamborn, Matthes, Grosse, Klimova, Kunitsky and Siegert. This is an open-access article distributed under the terms of the Creative Commons Attribution License (CC BY). The use, distribution or reproduction in other forums is permitted, provided the original author(s) and the copyright owner(s) are credited and that the original publication in this journal is cited, in accordance with accepted academic practice. No use, distribution or reproduction is permitted which does not comply with these terms.
*Correspondence: L. Schirrmeister, bHV0ei5zY2hpcnJtZWlzdGVyQGF3aS5kZQ==