- 1CNOOC Research Institute Ltd, Beijing, China
- 2Bohai-Rim Energy Research Institute, Northeast Petroleum University, Qinhuangdao, China
- 3Institute of Chongqing Unconventional Oil and Gas Development, Chongqing University of Science and Technology, Chongqing, China
Natural fractures are regarded as important reservoir spaces and effective seepage channels at metamorphic buried hills. Continuous networks associated with multi-scale fractures with good connectivity is critical for high-quality reservoirs as well as high and stable production in the tight metamorphic rocks. The multi-scale fractures in Bozhong 19–6 metamorphic buried hills were well characterized through integrating image logs, cores, thin-sections, and scanning electron microscope, etc. After that, power-law distribution of multi-scale fractures was established to understand contribution of fractures to reservoir quality and figure out structure models of fracture networks as well as their impact on production. Results show that parameters of fracture systems vary regularly with fracture scales. Fracture development degree, e.g., cumulative areal density, increases as a power law function with decreasing fracture size from macro to micro (e.g., aperture and/or length), where storage space associated with micro fractures is also increased. Reversely, fracture connectivity and permeability follow a significant decreasing trend. Five structure models of fracture network were established based on combination pattern of multi-scale fractures: multi-scale fracture network with high-density and multi-sets, large-scale fracture network with medium-density and multi-sets, small-scale fracture network with high-density and multi-sets, large-scale fracture network with low-density and multi-sets, and small-scale fracture network with low-density and single-set. The former two fracture networks can be widely developed into high-quality reservoirs, contributing greatly to high and stable yields. Fracturing is required for the third and the fourth fracture networks to obtain stable production, while it is difficult for the fifth fracture network to obtain industrial oil and gas flow.
Introduction
Buried hills have been regarded as important oil and gas exploration targets for a long time. Currently a large number of theories have been developed to understand buried hill oil-gas accumulations (Ye et al., 2021; Zhou et al., 2022). Exploration practice has confirmed that diverse lithology were developed at buried hill reservoirs, e.g., volcanic rocks, carbonate rocks, clastic rocks and metamorphic rocks, where metamorphic reservoirs have the largest exploration potential (Hou et al., 2013; Wang et al., 2015; Zhou et al., 2022). Major breakthroughs have been recently made in Archaean metamorphic buried hills in the Bohai Bay Basin, China, e.g., Bozhong 19–6 metamorphic buried hill, Xinglongtai buried hills and Jinzhou 25–1 metamorphic buried hills at Liaohe Basin, which have obtained hundred million tons of reserves (Hu et al., 2017; Wang et al., 2019; Xu et al., 2020; Ye et al., 2021). Long-period weathering, diverse lithology, and multiple tectonic stress brought complex reservoir structure to Archaean metamorphic buried hills. Hence, how to effectively evaluate buried hills has always been a challenge for researchers (Xu et al., 2020). Previous researchers on metamorphic reservoirs mainly focused on characterization of fractured reservoirs at buried hills, fracture genesis and its impact on reservoir quality (Wang et al., 2022). Multiple reservoirs have been found at metamorphic buried hills, e.g., weathering crust reservoirs on top and fractured reservoirs within buried hills. Scholars have described fracture development phases and filling behaviors, and reported that fracture development in buried hills were closely related to multiple tectonic activities. Researchers show that natural fractures are not only storage space for metamorphic buried hills, but also effective seepage channels (Gao et al., 2015; Gong et al., 2016; Fan et al., 2021; Zeng et al., 2022a). Natural fractures not only connect various pores and enhance seepage capacity, but also are key factors governing high yield of metamorphic buried hills (Huang et al., 2016; Hu et al., 2017; Xue et al., 2020). However, increasing exploration and development activities at buried hill reservoirs gradually expose our poor understanding of multi-scale fracture development and its spatial configuration at buried hills (Cao et al., 2021; Li et al., 2022a), that is, fracture development degree may not be the only factor determining high and stable production of single wells. Fractures of multiple scales are varied in lengths and apertures, resulting in different connectivity and storage capacity. Therefore, understanding configuration mode and spatial distribution of multi-scale fractures is important to guide drilling and development scheme of buried hill reservoirs (Gong et al., 2017). Taking Bozhong 19–6 metamorphic buried hill as an example, this paper uses image logs, core samples, thin sections, scanning electron microscope, etc., to characterize genesis and distribution of multi-scale fractures, clarify fracture distribution modes and their contributions to storage capacity, establish structure model of fracture networks under various configurations, and discuss development characteristics and their impact on productivity.
Geological setting
Bozhong 19–6 buried hill is located in the southwest of Bozhong Sag, Bohai Bay Basin, China (Figures 1A,B). It is a nearly NS extending structural ridge bounded by the Southwest Sag, the central Sag, the South Sag and the Huanghekou Sag. It is a faulted anticline complicated by three strike-slip faults and their derivatives (Figure 1C) (Gong et al., 2019a; Xu et al., 2020; Xue et al., 2020). Four sets of faults, i.e., N-S trending, NNE-SSW trending, NEE-SWW trending and E-W trending, were developed at the top of Bozhong 19–6 buried hills. It is dominated by Archean metamorphic rocks, which is overlaid by Paleogene Kongdian Formation, Shahejie Formation, Dongying Formation, Neogene Guantao Formation, Minghuazhen Formation and Quaternary Pingyuan Formation (Li et al., 2019). Dark mudstone at the third Member and the first Member of Shahejie Formation, and the third Member of Dongying Formation are the primary source rocks in the study area. It is a typical tight reservoirs, with weak overpressure and normal pressure. The Archean metamorphic rocks are mainly granite-gneiss and plagioclase gneiss, while postintrusive dykes, e.g., diorite-porphyrite, etc., are also developed (Zeng et al., 2016; Hou et al., 2019; Xu et al., 2019; Ye et al., 2021). Metamorphic rocks are minerally dominated by light minerals, e.g., quartz, plagioclase and K-feldspar, and dark minerals, e.g., biotite and amphibole, with proportion of light minerals >90%. Controlled by structure, weathering and lithology, Bozhong 19–6 buried hill can be vertically divided into weathering zone and inner zone (Luo et al., 2013; Luo et al., 2015; Luo et al., 2016). Reservoirs at weathering zone are composed of structural fractures, weathering fractures, dissolved pores, etc., where well-developed fractures result in continuous bed-like reservoirs. Reservoirs at inner zone is dominated by structural fractures, which is distributed in belt pattern controlled by high-angle faults.
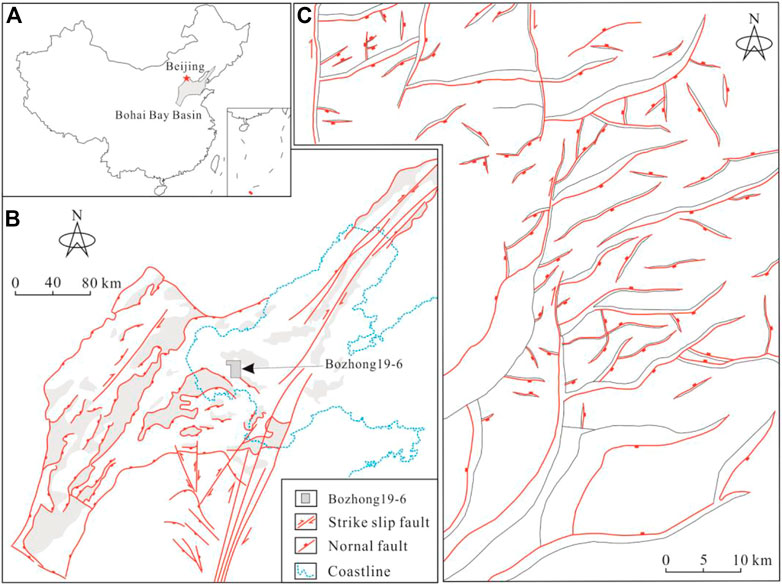
FIGURE 1. Location and structure outline of Bozhong 19–6 metamorphic buried hill. (A) Location of the Bohai Bay Basin. (B) Location of the Bozhong 19–6. (C) structure outline of Bozhong 19–6
Multi-scale fracture development
Fracture development evidenced by image logs
Conductive fractures, resistive fractures, small faults, induced fractures, etc., have been identified from image logs of 18 wells (Figure 2A), while induced fractures as artificial fractures are not in our research category. Conductive fractures are the most popular ones among these three natural fracture types, accounting for 99% of the total fractures number (Figure 3A). It is a good indicator of effective fracture networks in the study area. However, fillings identified from cores and thin-sections confirm (Figures 3B,C) that 59 %–68% of natural fractures are completely filled by minerals, e.g., quartz or calcite. Such a low resistive fracture number can be explained by that filling minerals are similar with that of parent rocks, making it difficult to distinguish them on image logs. In other words, image logs can only identify effective fractures.
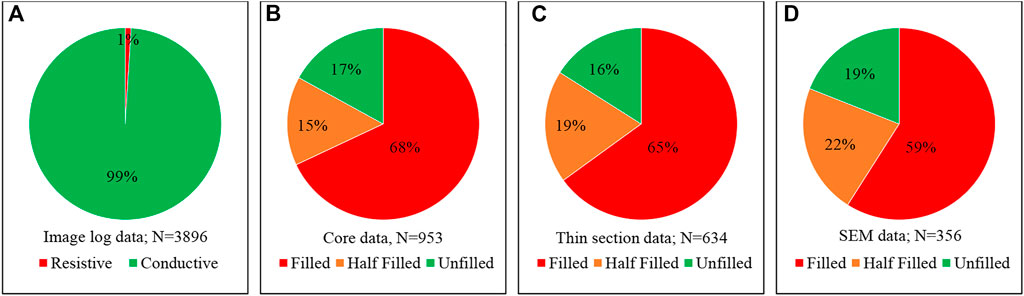
FIGURE 3. Effectiveness of fractures with multiple scales. (A) Effectiveness of fractures from image log data; (B) effectiveness of fractures from core data; (C) effectiveness of fractures from thin section data; (D) effectiveness of fractures from SEM data.
The metamorphic rock reservoirs in the study area have complex fracture orientations, especially at the weathering zone on the upper part of the buried hill, where fractures are developed in almost all directions (Figure 4A). It can be attributed to a large number of unsystematic weathering fractures. Fractures at the inner zone commonly follow four orientations, e.g., NEE-SWW trending, near E-W trending, near N-S trending and NW-SE trending (Figure 4B). The fracture dip angles at weathering zone are mainly distributed at 30°–60°, which is dominated by oblique fractures, with low-angle fractures and nearly vertical fractures of secondary importance (Figure 4C). Medium to high dip angle at the inner zone suggests that it is dominated by structural fractures (Figure 4D). The conductive fractures identified on image logs have large size, with fracture aperture of 50–250 μm (average value: 120.7 μm) (Figure 5A). The fracture areal density is about 1–5 m/m2, with an average of 2.4 m/m2 (Figure 5B). The density in the weathering zone is higher than that in the inner zone. Fracture porosity detected by image logs is relatively low, about 0.02%–0.2%, with an average of 0.10% (Figure 5C). Fracture permeability detected by image logs is about 3–10 mD, with an average of 8.35 mD (Figure 5D), effectively improving reservoir seepage capacity.
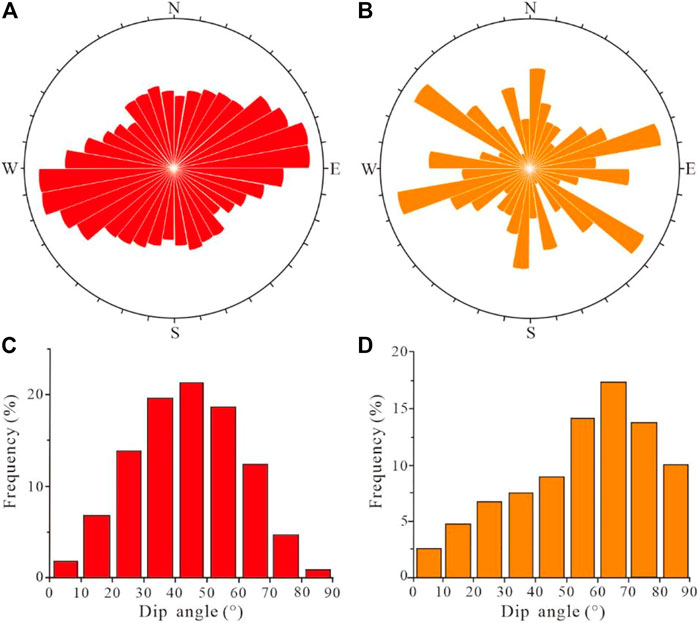
FIGURE 4. Fracture occurrence at weathering zone and inner zone. Rose diagrams showing fracture strikes at weathering zone (A) and at inner zone (B). Fracture dip angle at weathering zone (C) and at inner zone (D).
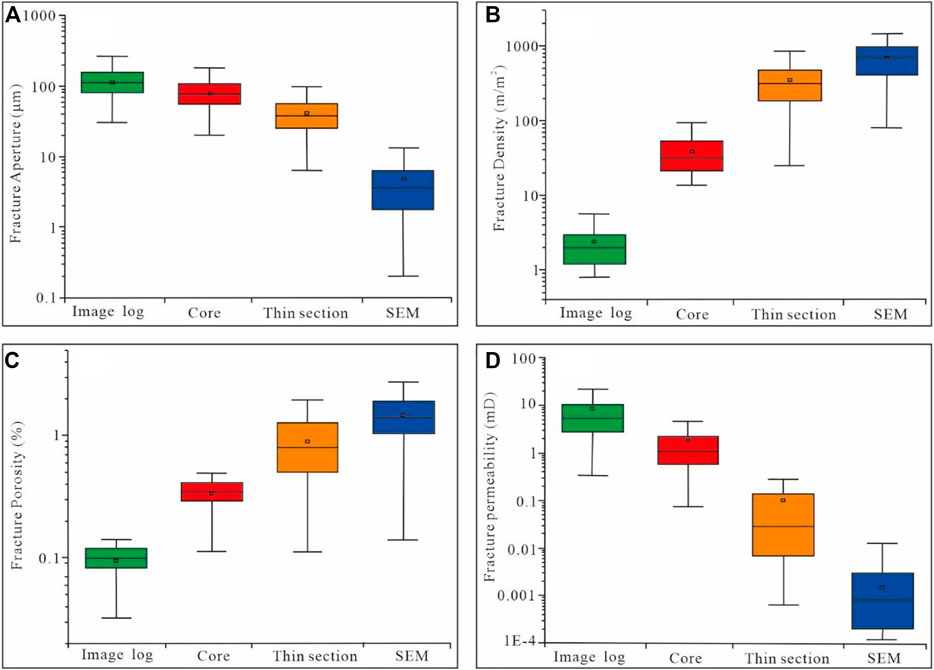
FIGURE 5. Parameters distribution of fractures at multiple scales. (A) Fracture aperture. (B) Fracture areal density. (C) Fracture porosity. (D) Fracture permeability.
Fracture development on cores
Fractures on cores can be genetically divided into structural fractures and weathering fractures based on geological origin. Structural fractures are the most popular type in metamorphic reservoirs in the study area, with multi-stages, multi-directions (multi-sets), uneven development, difference in filling behaviors (Figures 6A,B). Structural fractures have medium to high dip angles, ranging from 60° to 90°. They have large length on cores (diameter of 3 inches), generally larger than 10 cm (mainly 12–20 cm), with an average of 17.09 cm. Their areal density is mainly 10–30 m/m2, with an average of 20.1 m/m2. Calcite and quartz filling can be widely observed, with full-filled fractures of 72%, half-filled fractures of 13% and unfilled fractures of 15%, 28% of them are effective fractures.
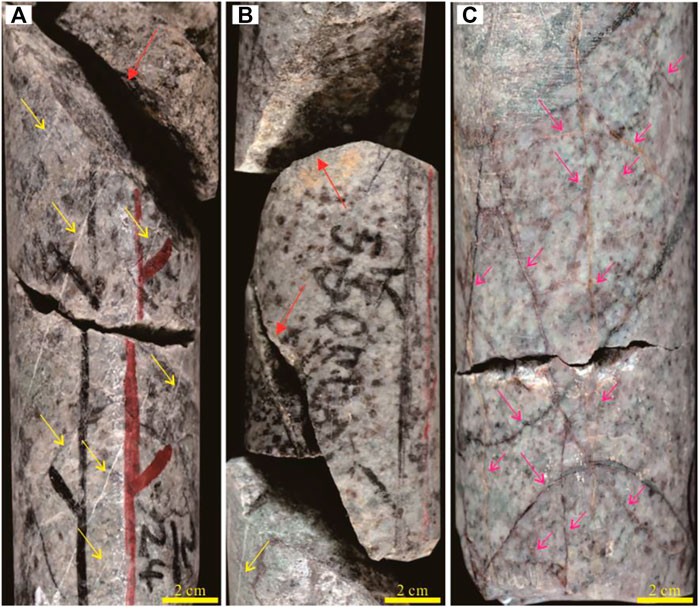
FIGURE 6. Fracture types and development characteristics on cores. Red arrows represent unfilled structural fractures, yellow arrows are calcite-filled structural fractures, purple arrows in Figure 6. (A) Calcite-filled and unfilled structural fractures. (B) Unfilled and calcite-filled structural fractures. (C) Weathering fractures.
Weathering fractures are mainly developed at weathering zone in honeycomb pattern or network pattern (Figure 6C). They are poorly oriented, with curved or arc-shaped surface and unstable occurrence. They are developed in all directions, with dip angles from 0° to 90°. They are short in length, in a range of 4–7 cm, with an average of 5.81 cm, while some can be decimeter-scale. Weathering fractures are well developed, with areal density of 30–70 m/m2 (average value: 53.0 m/m2, and up to 93 m/m2 locally). They are often filled with argillaceous or clay minerals, or are disseminated with iron (oxidized to red) (Figure 3C). They are slightly filled compared with structural fractures, with full-filled ones of 68%, half-filled ones of 15% and unfilled ones of 17%, 32% of them are effective fractures.
Fracture development on thin-sections
Fractures can be divided into intergranular ones, intragranular ones and grain-edge ones based on relationship between micro fractures and mineral grains (Zeng et al., 2013; Gong et al., 2019b). Fractures observation on 265 thin-sections shows that intergranular fractures are popular in the study area (Figures 7A,B). These fractures cut through multiple mineral grains, and even develop throughout the whole thin section. These microfractures are also highly filled, with full filling accounting for 70%, semi-filling accounting for 14% and unfilled ones accounting for 16%, while filling at weathering zone is slightly lower than that at inner zone. 14 filling mineral types can be identified, e.g., carbonate minerals (dolomite, calcite, ankerite and ferroan calcite), felsic matrix, quartz, argillaceous (ferrous), authigenic clay minerals (chlorite, kaolinite) and pyrite and siderite, etc. Fracture aperture is mainly distributed in 10–150 um, with peak value at 20–50 um (Figure 5A). Filled fractures are 40–150 um in aperture (peak value at 50–80 um), while unfilled fractures are 10–60 um in aperture (peak value at 20–40 um).
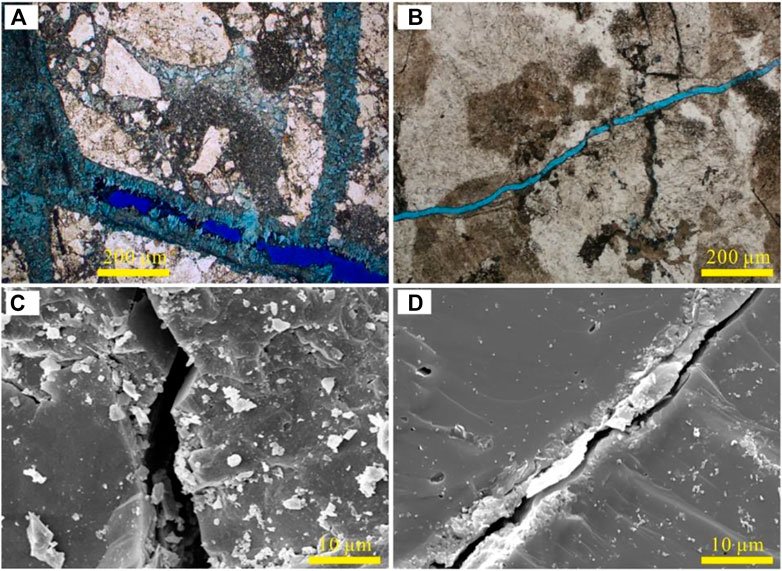
FIGURE 7. Development characteristics of micro fractures. (A) Intergranular fracture, full to half filled; (B) intergranular fracture, unfilled; (C) intragranular fracture, unfilled; (D) intragranular fracture, half filled.
The intergranular fractures are well developed, with areal density of 200–600 m/m2 (an average value of 345.4 m/m2) (Figure 5B). The porosity of micro fractures on thin-sections is mainly 0.5%–1.2%, with an average of 0.88% (Figure 5C), while permeability is between 0.01 mD and 1 mD, with an average of 0.13 mD (Figure 5D).
Fracture development on SEM
Micro fractures under scanning electron microscope are mainly developed within mineral grains as intragranular fractures (e.g., cleavage cracks in feldspar, mica, calcite and quartz cracks) and grain-edge fractures. They have small apertures, ranging from 2 μm to 8 μm, with an average value of 5.8 μm (Figure 5A). The aperture of full-filled fractures is mainly distributed between 10 μm and 30 μm, averaging of 21.8 μm. Although aperture is small, intragranular fractures are developed in almost all mineral grains, while multi-phase fractures can be observed at some grains. The areal density of intragranular fractures is mainly 400–1000 m/m2, with peak at 600–800 m/m2 and average value of 700.5 m/m2 (Figure 5B). Porosity of intragranular fractures is about 1.0%–2.5%, with an average of 1.47% (Figure 5C), while permeability is about 0.0001–0.01 mD, with an average of 0.0087 mD (Figure 5D).
Discussions
Multi-scale fracture classification and its contribution to reservoir
Multi-scale fracture development description shows that fracture size is negatively correlated with fracture development degree (Figure 5B). Investigations on multi-scale fractures from outcrops, cores, thin-sections, etc., show that length and aperture are generally distributed at power-law pattern (Fu et al., 2007; Gong et al., 2012), while specific parameters of power law distribution vary with fracture size and development degree (Bonnet et al., 2001; Maerten et al., 2006; Ortega et al., 2006; Li et al., 2012; Strijker et al., 2012; Johri et al., 2014; Gong et al., 2018; Pan et al., 2019; Gong et al., 2021; Zhu et al., 2021; Li et al., 2022b; Zhu et al., 2022). 3D geological modeling of multi-scale fractures can be performed based on the power law pattern of multi-scale fractures (Zeng et al., 2020; Lyu et al., 2021; Zeng et al., 2022b). The power law distribution of fractures with different scales (apertures) was studied in this study, based on which, structure models of fracture networks were established to classify fracture scales and analyze contribution of multi-scale fractures to storage capacity and their roles in oil field development.
Power-law distribution of (Figure 8B) multi-scale fractures in weathering zone is established based on their areal density distribution (Figure 8A), which can be used to determine certain fracture development intensity (the lateral axis represents fracture scale (aperture), and the vertical axis represents development intensity of fractures with size larger than certain value). Meanwhile, porosity and permeability distribution models were established for fractures with various scales (Figures 9, 10). Figures 9, 10 show that storage capacity of fractures varies greatly with their scales. Consequently, natural fracture system in the study area can be divided into two types and four sub-types based on identify methods, fracture scales and their contributions to reservoirs. Specifically, the two types are macro fractures and micro fractures, and the four sub-types are fractures of image log scale, fractures of core scale, fractures of thin-section scale, and fractures of SEM scale (Figure 8C and Table 1).
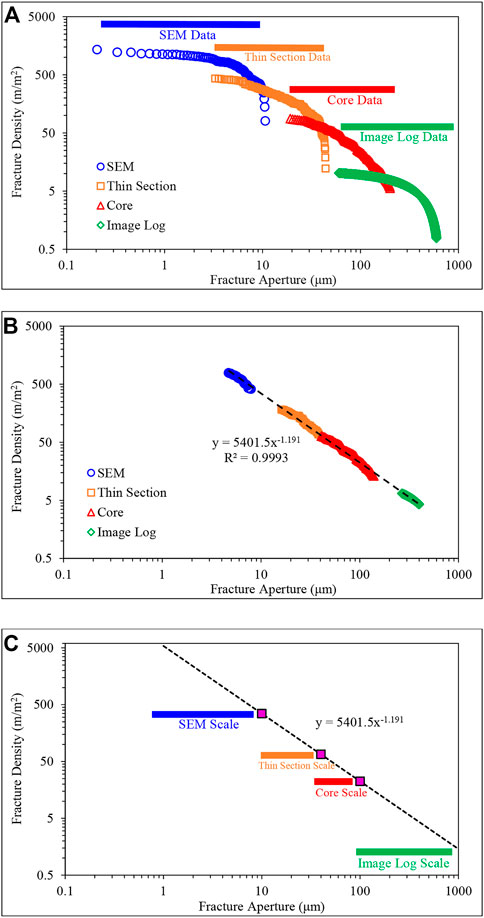
FIGURE 8. Density distribution of multi-scale fractures. (A) Density distribution of multi-scale fractures from statistical data. Density of certain fracture follows lognormal distribution. (B) Power-law distribution of fracture density based on multi-scale fractures. (C) Power-law distribution model of fractures and fracture classification in the study area.
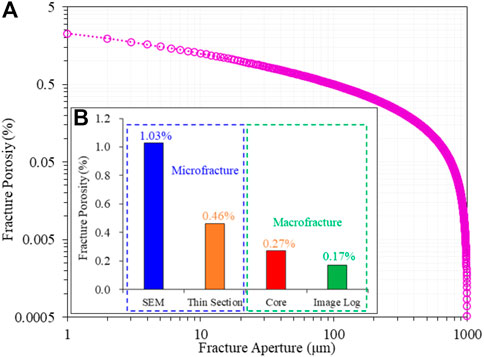
FIGURE 9. Porosity of fractures with multiple scales at BZ 19–6 metamorphic rock reservoir. (A) Contribution of fractures with different apertures to porosity. (B) Contribution of fractures of different scales to porosity.
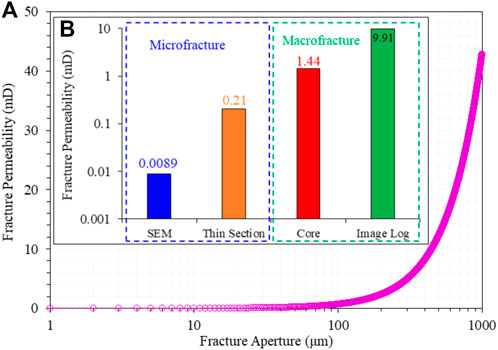
FIGURE 10. Permeability of fractures with multiple scales at BZ 19–6 metamorphic rock reservoir. (A) Contribution of fractures with different apertures to permeability. (B) Contribution of fractures of different scales to permeability.
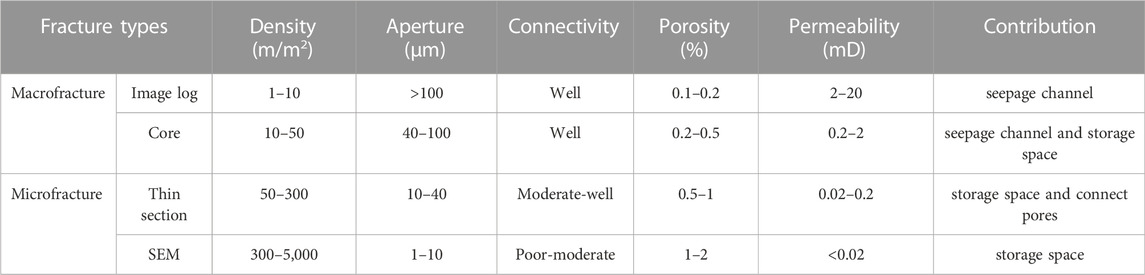
TABLE 1. Fracture types at BZ 19–6 metamorphic rock reservoir and its contribution to reservoir property.
Fractures of image log scale are defined as those can be identified on image logs with aperture >100 μm. Their density at the weathering zone is mainly 1–10 m/m2, contributing 0.1%–0.2% to porosity and 2–20 mD to permeability. They are well connected as primary seepage channels, which contributes greatly to high production at early development stage.
Fractures of core-scale can be directly observed with eye from cores, with apertures of 40–100 μm. Their density at the weathering zone of the study area is mainly 10–50 m/m2, which can increase porosity by 0.2%–0.5% and increase permeability by 0.2–2 mD. They are not only well connected, but also can provide storage space. Therefore, they are both important seepage channels and storage spaces, which is important for stable production at early development.
Fractures of thin-section scale are those can only be observed under optical microscope, commonly intergranular fracture with an aperture of 10–40 μm. Their density is mainly 50–300 m/m2, which can provide porosity of 0.5%–1.0% and permeability of 0.02–0.2 mD. These fractures can both increase porosity and connect matrix pores and micro fractures, which plays an important role in stable production at middle development period.
Fractures of SEM scale are those can only be observed under scanning electron microscope, which are typical intergranular fractures and grain-edge fractures with aperture of 1–10 μm. They are mainly developed within mineral grains. Their density at the weathering zone is about 300–5,000 m/m2, whose porosity is about 1.0%–2.0% with permeability <0.02 mD. Although they are small in scale and aperture, high density can increase porosity greatly, enabling them to be reservoirs. They can provide recoverable reserves at later development stage, depending greatly on their connectivity with fractures of thin-section scale.
Structure models of multi-scale fracture network
Exploration activities show that well productivity or reserves is not only related to reservoir quality and fracture density, but also comprehensively controlled by factors, e.g., fracture sets, fracture scale (length), fracture connectivity, and multi-scale fracture configuration, etc., since sometimes high and stable production cannot be obtained from reservoirs with high porosity. Therefore, five fracture configurations and network structures were constructed based on fracture density, fracture sets, fracture scale as well as fault distribution: multi-scale fracture network with high-density and multi-sets, large-scale fracture network with medium-density and multi-sets, small-scale fracture network with high-density and multi-sets, large-scale fracture network with low-density and multi-sets, and small-scale fracture network with low-density and single-set.
The multi-scale fracture networks with high-density and multi-sets are mainly developed at structures with multi-stage active faults and long-term active faults (in parallel or intersected pattern) (Figure 11). This fracture network pattern can develop multi-scale fractures (i.e., large faults, small faults, marco-fractures and micro-fractures) with high fracture density and good connectivity. The small faults with large apertures and length of hundreds meters to kilometers of meters can effectively communicate multi-scale fracture systems and further connect with matrix pore system, thus forming large-scale and well-connected reservoirs. High and stable oil and gas production can be obtained from this kind of fracture systems.
The large-scale fracture networks with medium-density and multi-sets are mainly developed at the middle and lower part of weathering zone, where many faults are developed at single stage (in parallel or intersected pattern) (Figure 12). They are dominated by large-scale structural fractures, followed by a small group of weathering fractures. However, they are well-connected to form continuous and well-connected reservoirs.
The small-scale fracture networks with high-density and multi-sets are commonly have high fracture density. However, small aperture and length as well as poor connectivity make it difficult to form widely-connected reservoirs (Figure 13). They are mainly developed at the upper part of weathering zone and strata with altered brittleness and ductility. High oil and gas production can be obtained from it at initial stage, where fracturing is required to improve and stable long-term oil and gas production.
The large-scale fracture networks with low-density and multi-sets are mainly developed in the inner zone, with large structural fractures, low fracture density and fair connectivity. Fractures are commonly well developed at the edge of the faults and brittle strata, where industrial oil and gas flow can also be obtained. Small-scale fracture network with low-density and single-set is mainly developed at basements with poor connectivity, which is generally not regarded as reservoir.
Conclusion
Multi-scale fracture systems were developed at the Bozhong 19–6 metamorphic rock reservoir, where system parameters vary regularly with fracture scales. Macro fractures with large length and aperture can contribute greatly to reservoir permeability (2-20 mD), however, their low density can only provide limited porosity (0.1%–0.2%) to reservoirs. The micro fractures with high density are important reservoirs with porosity of 1%–2%. However, small aperture and length can only communicate matrix pores, contributing minor to reservoir permeability (<0.02 mD). Density of single-scale fractures follows lognormal distribution pattern, while multi-scale fractures follow power-law distribution pattern. Hence, fracture density of certain scale can be accurately predicted with the power-law distribution.
Five structure models of fracture networks are established based on fracture development at different structures of the buried hills. The multi-scale fracture networks with high-density and multi-sets are mainly developed at structures with multi-stage faults and long-term faults. They can form widely-distributed reservoirs with good connectivity, contributing to high and stable oil and gas production. The small-scale fracture networks with high-density and multi-sets are developed at the upper part of weathering zone. However, small aperture and length as well as poor connectivity limits the development of widely-connected reservoirs, where fracturing is required to stable long-term oil and gas production. The large-scale fracture networks with medium-density and multi-sets are mainly developed at the inner zone, mainly composed of large-scale structural fractures, with low density and fair connectivity. Industrial oil and gas flow can also be obtained from this network via fracturing. The small-scale fracture networks with low-density and single-set are mainly developed at basements with poor connectivity, which is generally not regarded as reservoir.
Data availability statement
The original contributions presented in the study are included in the article/Supplementary Material, further inquiries can be directed to the corresponding author.
Author contributions
Conceptualization, HF and TN; Data curation, LM and XS; Formal analysis, JL; Investigation, TF; Methodology, LG; Writing—review & editing, LG, YS, and XS.
Funding
This study is financially supported by National Natural Science Foundation of China (Grant No. 42072155), Natural Science Foundation of Heilongjiang Province (Grant No. YQ 2022D006), Young Innovative Talents Training Program for Universities in Heilongjiang Province (grant no. UNPYSCT-2020147) and Postdoctoral Research Foundation of Heilongjiang Province (Grant No. LBH-Q21001).
Conflict of interest
The authors LM, HF, TF, TN, and JL were employed by CNOOC Research Institute Ltd, China.
The remaining authors declare that the research was conducted in the absence of any commercial or financial relationships that could be construed as a potential conflict of interest.
Publisher’s note
All claims expressed in this article are solely those of the authors and do not necessarily represent those of their affiliated organizations, or those of the publisher, the editors and the reviewers. Any product that may be evaluated in this article, or claim that may be made by its manufacturer, is not guaranteed or endorsed by the publisher.
References
Bonnet, E., Bour, O., Odling, N. E., Davy, P., Main, I., Cowie, P., et al. (2001). Scaling of fracture systems in geological media. Rev. Geophys. 39 (3), 347–383. doi:10.1029/1999RG000074
Cao, D. S., Zeng, L. B., Lvy, W. Y., Xu, X., and Tian, H. (2021). Progress in brittleness evaluation and prediction methods in unconventional reservoirs. Petroleum Sci. Bull. 01, 31–45. doi:10.3969/j.issn.2096-1693.2021.01.003
Fan, T. E., Niu, T., Fan, H. J., Wang, S., Xiao, K., and Luo, J. H. (2021). Geological model and development strategy of Archean buried hill reservoirin BZ19-6 condensate field. China Offshore Oil Gas 33 (03), 85–92. doi:10.11935/j.issn.1673-1506.2021.03.009
Fu, X. F., Su, Y. P., Lv, Y. F., Zhang, Y. F., and Fu, G. (2007). Fractal characteristic and geological meaning of fault and fracture. Earth Science-Journal China Univ. Geosciences 32 (2), 227–234. doi:10.3321/j.issn:1000-2383.2007.02.011
Gao, S., Zeng, L. B., Ma, S. Z., He, Y. H., Gong, L., Zhao, X. Y., et al. (2015). Quantitative prediction of fractures with different directions in tight sandstone reservoirs. Nat. Gas. Geosci. 26 (03), 427–434. doi:10.11764/j.issn.1672-1926.2015.03.0427
Gong, L., Fu, X. F., Gao, S., Zhao, P. Q., Luo, Q. Y., Zeng, L. B., et al. (2018). Characterization and prediction of complex natural fractures in the tight conglomerate reservoirs: A fractal method. Energies 11 (9), 2311. doi:10.3390/en11092311
Gong, L., Gao, M. Z., Zeng, L. B., Fu, X. F., Gao, Z. Y., Gao, A., et al. (2017). Controlling factors on fracture development in the tight sandstone reservoirs: A case study of jurassic-neogene in the kuqa foreland basin. Nat. Gas. Geosci. 28 (2), 199–208. doi:10.11764/j.issn.1672-1926.2016.12.003
Gong, L., Gao, S., Liu, B., Yang, J. G., Fu, X. F., Xiao, F., et al. (2021). Quantitative prediction of natural fractures in shale oil reservoirs. Geofluids 2021, 1–15. doi:10.1155/2021/5571855
Gong, L., Liu, B., Fu, X. F., Jabbari, H., Gao, S., Yue, W. T., et al. (2019). Quantitative prediction of sub-seismic faults and their impact on waterflood performance: Bozhong 34 oilfield case study. J. Petroleum Sci. Eng. 172, 60–69. doi:10.1016/j.petrol.2018.09.049
Gong, L., Su, X. C., Gao, S., Fu, X. F., Jabbari, H., Wang, X. X., et al. (2019). Characteristics and formation mechanism of natural fractures in the tight gas sandstones of Jiulongshan gas field, China. J. Petroleum Sci. Eng. 175, 1112–1121. doi:10.1016/j.petrol.2019.01.021
Gong, L., Zeng, L. B., Chen, S. M., Gao, S., Zhang, B. J., Zu, K. W., et al. (2016). Characteristics of micro-fractures and contribution to the compact conglomerate reservoirs. Geotect. Metallogenia 40 (1), 38–46. doi:10.16539/j.ddgzyckx.2016.01.004
Gong, L., Zeng, L. B., Miao, F. B., Wang, Y. S., Wei, Y., Li, J., et al. (2012). Application of fractal geometry on the description of complex fracture systems. J. Hunan Univ. Sci. Technol. Nat. Sci. Ed. 27 (4), 6–10. doi:10.3969/j.issn.0563-5020.2013.01.014
Hou, L. H., Luo, X., Wang, J. H., Yang, F., Zhao, X., and Mao, Z. G. (2013). Weathered volcanic crust and its petroleum geologic significance: A case study of the carboniferous volcanic crust in northern xinjiang. Petroleum Explor. Dev. 40 (3), 257–265. doi:10.11698/PED.2013.03.01
Hou, M. C., Cao, H. Y., Li, H. Y., Chen, A. Q., Wei, A. J., Chen, Y., et al. (2019). Characteristics and controlling factors of deep buried-hill reservoirs in the BZ19-6 structural belt, Bohai Sea area. Geol. Explor. 39 (01), 33–44. doi:10.3787/j.issn.1000-0976.2019.01.004
Hu, Z. W., Xu, C. G., Yang, B., Huang, Z., and Su, W. (2017). Reservoir forming mechanism of Penglai 9-1 granite buried-hills and its oil geology significance in Bohai Sea. Acta Pet. Sin. 38 (03), 274–285. doi:10.7623/syxb201703004
Huang, J. H., Tan, X. F., Cheng, Y. J., Li, Z. M., Ma, L. J., Zhang, H. L., et al. (2016). Structural features of weathering crust of granitic basement rock and its petroleum geological significance a case study of basement weathering crust of Dongping Area in Qaidam Basin. Earth Sci. 41 (12), 2041–2060. doi:10.3799/dqkx.2016.528
Johri, M., Zoback, M. D., and Hennings, P. (2014). A scaling law to characterize fault-damage zones at reservoir depths. AAPG Bull. 98 (10), 2057–2079. doi:10.1306/05061413173
Li, H., Tang, H. M., Qin, Q. R., Zhou, J. L., Qin, Z. J., Fan, C. H., et al. (2019). Characteristics, formation periods and genetic mechanisms of tectonic fractures in the tight gas sandstones reservoir: A case study of xujiahe formation in YB area, sichuan basin, China. J. Petrol. Sci. Eng. 178, 723–735. doi:10.1016/j.petrol.2019.04.007
Li, H., Zhou, J. L., Mou, X. Y., Guo, H. X., Wang, X. X., An, H. Y., et al. (2022). Pore structure and fractal characteristics of the marine shale of the longmaxi formation in the changning area, southern sichuan basin, China. Front. Earth Sci. 10, 1018274. doi:10.3389/feart.2022.1018274
Li, J., Li, H., Yang, C., Wu, Y. J., Gao, Z., and Jiang, S. L. (2022). Geological characteristics and controlling factors of deep shale gas enrichment of the wufeng-longmaxi formation in the southern sichuan basin, China. Lithosphere, 2022, 4737801. doi:10.2113/2022/4737801
Li, Y. G., Gong, L., Zeng, L. B., Ma, H. L., Yang, H., Zhang, B. J., et al. (2012). Characteristics of fractures and their contribution to the deliverability of tight conglomerate reservoirs in the Jiulongshan Structure, Sichuan Basin. Nat. Gas. Ind. 32 (1), 22–26. doi:10.3787/j.issn.1000-0976.2012.01.004
Luo, Q., George, S., Xu, Y., and Zhong, N. (2016). Organic geochemical characteristics of the mesoproterozoic hongshuizhuang formation from northern China: Implications for thermal maturity and biological sources. Org. Geochem. 99, 23–37. doi:10.1016/j.orggeochem.2016.05.004
Luo, Q., Zhong, N., Wang, Y., Ma, L., and Li, M. (2015). Provenance and paleoweathering reconstruction of the mesoproterozoic hongshuizhuang formation (1.4 Ga), northern north China. Int. J. Earth Sci. 104 (7), 1701–1720. doi:10.1007/s00531-015-1163-5
Luo, Q., Zhong, N., Zhu, L., Wang, Y., Qin, J., Qi, L., et al. (2013). Correlation of burial organic carbon and paleoproductivity in the Mesoproterozoic Hongshuizhuang Formation, northern North China. Chin. Sci. Bull. 58, 1299–1309. doi:10.1007/s11434-012-5534-z
Lyu, W. Y., Zeng, L. B., Chen, S. Q., Lyu, P., Dong, S. Q., Hui, C., et al. (2021). Characterization methods of multi-scale natural fractures in tight and low-permeability sandstone reservoirs. Geol. Rev. 67 (02), 543–556. doi:10.16509/j.georeview.2021.02.020
Maerten, L., Gillespie, P., and Daniel, J. (2006). Three-dimensional geomechanical modeling for constraint of subseismic fault simulation. AAPG Bull. 90 (9), 1337–1358. doi:10.1306/03130605148
Ortega, O. J., Marrett, R. A., and Loubach, S. E. (2006). A scale-independent approach to fracture intensity and average spacing measurement. AAPG Bull. 90 (2), 193–208. doi:10.1306/08250505059
Pan, Z. K., Liu, D. D., Huang, Z. X., Jiang, Z. X., Song, Y., Guo, J., et al. (2019). Paleotemperature and paleopressure of methane inclusions in fracture cements from the Wufeng-Longmaxi shales in the Luzhou area, southern Sichuan Basin. Petroleum Sci. Bull. 03, 242–253. doi:10.3969/j.issn.2096-1693.2019.03.022
Strijker, G., Bertotti, G., and Luthi, S. M. (2012). Multi-scale fracture network analysis from an outcrop analogue: A case study from the cambro-ordovician clastic succession in petra, Jordan. Mar. Petroleum Geol. 38 (1), 104–116. doi:10.1016/j.marpetgeo.2012.07.003
Wang, D. Y., Wang, Q. B., Liu, X. J., Zhao, M., Hao, Y. W., and YiWei, H. (2019). Characteristics and developing patterns of gneiss buried hill weathering crust reservoir in the sea area of the Bohai Bay basin. Acta Petrol. Sin. 35 (4), 1181–1193. doi:10.18654/1000-0569/2019.04.13
Wang, J., Hu, C. G., Pan, Y. L., Huang, Q. J., Yuan, H. Q., Gong, L., et al. (2022). Fracture development characteristics and comprehensive evaluation of buried hill metamorphic reservoir in Jihua 1 area. Chin. J. Geol. 57 (2), 463–477. doi:10.12017/dzkx.2022.027
Wang, X., Zhou, X. H., Xu, G. S., Liu, P. B., Gao, K. S., and Guan, D. Y. (2015). Characteristics and controlling factors of reservoirs in Penglai 9-1 large – scale oilfield in buried granite hills, Bohai Sea. Oil & Gas Geol. 36 (02), 262–270. doi:10.11743/ogg20150211
Xu, C. G., Du, X. F., Liu, X. J., Xu, W., and Hao, Y. W. (2020). Formation mechanism of high-quality deep buried-hill reservoir of Archaean metamorphic rocks and its significance in petroleum exploration in Bohai Sea area. Oil & Gas Geol. 41 (02), 235–247. doi:10.11743/ogg20200201
Xu, C. G., Yu, H. B., Wang, J., and Liu, X. J. (2019). Formation conditions and accumulation characteristics of Bozhong 19-6 large condensate gas field in offshore Bohai Bay Basin. Petroleum Explor. Dev. 46 (01), 27–40. doi:10.1016/s1876-3804(19)30003-5
Xue, Y. A., Wang, Q., Niu, C. M., Miao, Q. Y., Liu, M. X., and Yin, J. (2020). Hydrocarbon charging and accumulation of BZ 19-6 gas condensate field in deep buried hills of Bozhong Depression, Bohai Sea. Oil & Gas Geol. 41 (05), 891–902. doi:10.11743/ogg20200501
Ye, T., Niu, C. M., Wang, Q. B., Dai, L. M., and Li, F. (2021). Characteristics and controlling factors of large bedrock buried-hill reservoirs in the Bohai Bay Basin—A case study of the BZ19-6 condensate field. Acta Geol. Sin. 95 (06), 1889–1902. doi:10.19762/j.cnki.dizhixuebao.2021132
Zeng, L. B., Gong, L., Guan, C., Zhang, B. J., Wang, Q. Q., Zeng, Q., et al. (2022). Natural fractures and their contribution to tight gas conglomerate reservoirs: A case study in the northwestern sichuan basin, China. J. Petroleum Sci. Eng. 210, 110028. doi:10.1016/j.petrol.2021.110028
Zeng, L. B., Lyu, P., Qu, X. F., and Fan, J. M. (2020). Multi-scale fractures in tight sandstone reservoirs with low permeability and geological conditions of their development. Oil & Gas Geol. 41 (3), 449–453. doi:10.11743/ogg20200301
Zeng, L. B., Lyu, W. Y., Xu, X., Tian, H., Lu, S. L., and Zhang, M. Q. (2022). Development characteristics, formation mechanism and hydrocarbon significance of bedding fractures in typical tight sandstone and shale. Acta Pet. Sin. 43 (02), 180–191. doi:10.7623/syxb202202002
Zeng, L. B., Su, H., Tang, X. M., Peng, Y. M., and Gong, L. (2013). Fractured tight sandstone oil and gas reservoirs: A new play type in the dongpu depression, Bohai Bay Basin, China. AAPG Bull. 97 (3), 363–377. doi:10.1306/09121212057
Zeng, L. B., Zhu, R. K., Gao, Z. Y., Gong, L., and Liu, G. P. (2016). Structural diagenesis and its petroleum geological significance. Petroleum Sci. Bull. 02, 191–197. doi:10.3969/j.issn.2096-1693.2016.02.015
Zhou, X. H., Wang, Q. B., Feng, C., Ye, T., Liu, X. J., Hao, Y. W., et al. (2022). Formation conditions and geological significance of large archean buried hill reservoirs in Bohai sea. Earth Sci. 47 (05), 1534–1548. doi:10.3799/dqkx.2021.249
Zhu, H. Y., Song, Y. J., and Tang, X. H. (2021). Research progress on 4-dimensional stress evolution and complex fracture propagation of infill wells in shale gas reservoirs. Petroleum Sci. Bull. 03, 396–416. doi:10.3969/j.issn.2096-1693.2021.03.032
Keywords: metamorphic buried hills, multi-scale fractures, structure models of fracture networks, contribution, power-law distribution
Citation: Mi L, Fan H, Fan T, Gong L, Niu T, Su X, Luo J and Sun Y (2023) Development characteristics of multi-scale fracture network systems in metamorphic buried hills. Front. Earth Sci. 10:1108032. doi: 10.3389/feart.2022.1108032
Received: 25 November 2022; Accepted: 09 December 2022;
Published: 04 January 2023.
Edited by:
Hu Li, Southwest Petroleum University, ChinaReviewed by:
Xiaodong Lan, China University of Geosciences, ChinaJinxiong Shi, Yangtze University, China
Copyright © 2023 Mi, Fan, Fan, Gong, Niu, Su, Luo and Sun. This is an open-access article distributed under the terms of the Creative Commons Attribution License (CC BY). The use, distribution or reproduction in other forums is permitted, provided the original author(s) and the copyright owner(s) are credited and that the original publication in this journal is cited, in accordance with accepted academic practice. No use, distribution or reproduction is permitted which does not comply with these terms.
*Correspondence: Lei Gong, kcgonglei@foxmail.com