- 1GFZ German Research Centre for Geosciences, Potsdam, Germany
- 2Tectonics Research Group, Department of Earth Sciences, Utrecht University, Utrecht, Netherlands
- 3Department of Earth Sciences, ETH Zürich, Zürich, Switzerland
- 4Department of Earth and Environmental Sciences, University of Milano-Bicocca, Milan, Italy
- 5Institute of Meteorology, Freie Universität Berlin, Berlin, Germany
The formation of new subduction zones is a key element of plate tectonics and the Wilson cycle, and many different controlling mechanisms have been proposed to initiate subduction. Here, we provide a brief overview of the known scenarios of subduction initiation in intra-oceanic and ocean-continent tectonic settings. Intra-oceanic subduction is most commonly associated with mechanical heterogeneities within the oceanic lithosphere, such as pre-existing fracture zones, spreading ridges, and transform faults. Numerous and well-recognized examples of new active subduction zones formed in intra-oceanic environments during the Cenozoic, suggesting that the initiation of ocean-ocean subduction must be a routine process that occurs “easily and frequently” in the mode of plate tectonics currently operating on Earth. On the contrary, the most traditional mechanisms for the establishment of classic self-sustaining ocean-continent subduction—passive margin collapse and subduction transference—are surprisingly rare in observations and difficult to reproduce in numerical models. Two alternative scenarios—polarity reversal and lateral propagation-induced subduction initiation—are in contrast much better documented in nature and experimentally. However, switching of subduction polarity due to arc-continent collision and lateral transmission of subducting plate boundaries are both inextricably linked to pre-existing intra-oceanic convergence. We, therefore, conclude that the onset of classic ocean-continent subduction zones is possible only through the transition from a former intra-oceanic subduction system. This transition is likely facilitated by the ductile damage accumulation and stress concentration across the aging continental margin. From this perspective, the future closure of the Atlantic Ocean can be viewed as an archetypal example of the role of transitional process between intra-oceanic subduction (Lesser Antilles) and the development of a new subduction zone at a passive continental margin (eastern North America).
Introduction
Subduction is a key element of terrestrial plate tectonics (Stern, 2002). Although the periodic events of opening and closure of oceanic basins (Wilson, 1966) suggest that subduction must initiate frequently in geologic history, the underlying mechanisms that trigger subduction are not fully understood (Stern, 2004; Cloetingh et al., 2021; Yang, 2022). Difficulties in identifying and characterizing subduction initiation in nature are related to poor accessibility of data, lack of a priori knowledge about where subduction begins, incomplete understanding of the geologic, petrologic, and geochemical signatures, as well as the physical parameters required for the emergence of new subduction zones (Gerya, 2011; Stern and Gerya, 2018; Gerya, 2022). A further complication is that the conditions and dynamics of subduction initiation at mature continental margins (Nikolaeva et al., 2010, 2011; Marques et al., 2013, 2014) differ significantly from those in intra-oceanic environments (Nikolaeva et al., 2008; Zhu et al., 2009; Dymkova and Gerya, 2013).
Traditionally, subduction initiation scenarios are divided into two main types (Stern, 2004): 1) “spontaneous”, caused by internal gravitational instability (i.e., local, vertically-oriented forces originating in situ); and 2) “induced”, triggered by on-going plate convergence (i.e., horizontally-oriented far-field tectonic forces). However, recent studies show that it can be challenging to distinguish between these end-members (Zhang and Leng, 2021; Zhou and Wada, 2021). For example, oceanic transform faults that have been considered as potential sites for purely spontaneous, gravity-driven collapse (Karig, 1982; Matsumoto and Tomoda, 1983; Dymkova and Gerya, 2013; Maunder et al., 2020) can hardly evolve into a subduction zone without either a horizontal or vertical external forcing (Leng et al., 2012; Arcay et al., 2020). Collapse of passive continental margins, another traditional example of the spontaneous mechanism (Vlaar and Wortel, 1976; Cloetingh et al., 1982; Nikolaeva et al., 2010), may also require significant additional forces (Auzemery et al., 2021; Candioti et al., 2022) originating from topographic gradients (Marques et al., 2013) and/or mantle suction induced by adjacent subduction-related flow (Baes and Sobolev, 2017). In this regard, recent systematic reviews of well-documented subduction initiations in the Late Mesozoic and Cenozoic fundamentally question the feasibility of spontaneous mechanisms, suggesting that horizontally forced scenarios predominate (Crameri et al., 2020; Lallemand and Arcay, 2021). However, it is noteworthy that the observed temporal transitions in the stress regime during the onset of subduction may indicate a multistage evolution in which early horizontal forces dominate before subduction becomes self-sustaining, and vertical forces take over with time as the sinking slab begins to pull the subducting plate independently (Shuck et al., 2022). This suggests that the current system for classifying subduction zones based on whether horizontal or vertical forces are responsible for their formation is oversimplified (Crameri, 2022).
A particularly relevant aspect of the debate over “induced” vs “spontaneous” modes of subduction initiation regards the type of overriding plate (oceanic or continental), upon which we focus our review of the mechanisms controlling the formation of new subduction zones. We first consider models of subduction initiation typical of intra-oceanic environments, and then provide an overview of scenarios which are more appropriate for the onset of classic ocean-continent subduction. We argue that the most viable mechanisms for establishing self-sustaining subduction of the oceanic plate beneath the continent are intrinsically connected with the preceding intra-oceanic subduction, the triggering of which, in turn, is a very common process that occurred frequently during the Cenozoic (Gurnis et al., 2004; Crameri et al., 2020; Lallemand and Arcay, 2021). Following this line of reasoning, future closure of the Atlantic Ocean is unlikely to occur by gravitational collapse of mature continental margins, but rather by cascading subduction initiations in the Caribbean region, where on-going subduction of Atlantic lithosphere beneath the Lesser Antilles is prone to lateral transfer toward the western Atlantic passive margin along eastern North America (Zhou et al., 2020).
Intra-oceanic subduction initiation: “Easy and frequently”
The onset of subduction within the oceanic lithosphere has traditionally been associated with abrupt lateral heterogeneities in its structure (Figures 1A-C). Collapse of oceanic transform faults is the most typical mechanism for triggering intra-oceanic subduction (Uyeda and Ben-Avraham, 1972; Karig, 1982; Whattam and Stern, 2011). In this case, subduction nucleates along the active transform boundary, separating younger (less-dense) lithosphere from older (more-dense) lithosphere (Stern and Bloomer, 1992). Such lateral buoyancy contrasts may also be enhanced by pre-existing or fluid-induced rheological weakening of the plate interface (Dymkova and Gerya, 2013; Faccenna et al., 2018) and/or by accompanying external tectonic forces (Arcay et al., 2020). This mechanism has been extensively explored in numerous numerical (Hall et al., 2003; Gerya et al., 2008; Nikolaeva et al., 2008; Zhu et al., 2009; Zhou et al., 2018) and analog (Boutelier and Beckett, 2018) modelling studies. A new subduction zone can also form by inversion of an oceanic spreading ridge (Duretz et al., 2016; Beaussier et al., 2019) possibly assisted by inherited oceanic detachment faults (Toth and Gurnis, 1998; Maffione et al., 2015; Gülcher et al., 2019). When these structures are close to the passive margin, the process of subduction initiation is quickly followed by the process of obduction, during which regional-scale fragments of oceanic lithosphere (ophiolites) are emplaced over the adjacent continental lithosphere (Van Hinsbergen, et al., 2015; Agard et al., 2016). An alternative mechanism that does not rely on pre-defined heterogeneities and weaknesses in the lithosphere is plume-induced subduction initiation (Figure 1D; see also review by Baes et al. (2021) and references therein). In this scenario, a sufficiently large and hot plume head ruptures the overlying plate, leading to self-sustaining sinking of the adjacent portion of the lithosphere (Ueda et al., 2008; Gerya et al., 2015; Baes et al., 2016, 2020).
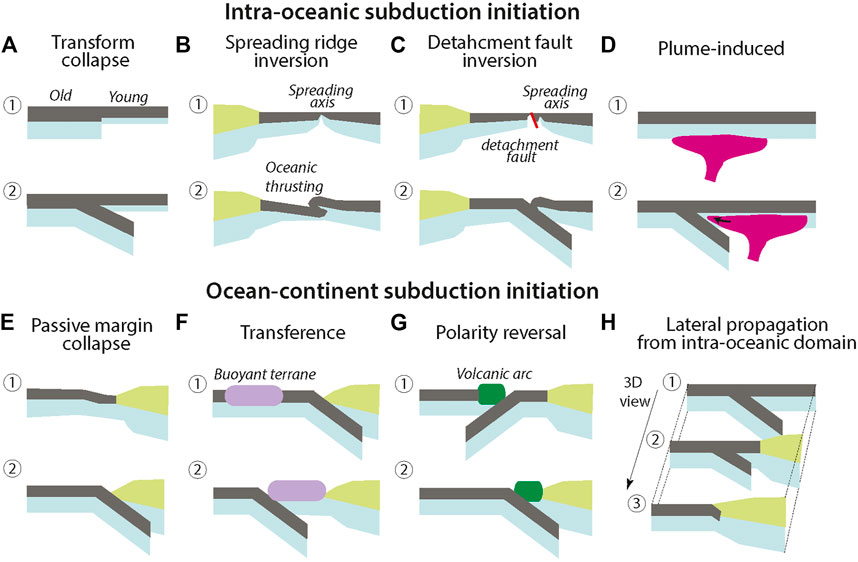
FIGURE 1. Different scenarios proposed (see text for references) for subduction initiation in (A–D) intra-oceanic and (E–H) ocean-continent tectonic settings: (A) collapse of an oceanic transform; (B) inversion of a spreading ridge; (C) inversion of a detachment fault; (D) plume-induced subduction initiation; (E) collapse of a passive continental margin; (F) subduction zone transference; (G) subduction polarity reversal; (H) lateral propagation from a pre-existing intra-oceanic subduction zone.
All the above mechanisms for intra-ocean subduction initiation are viable in terms of both observational records and reproducibility in experiments. This is also consistent with the notion that most Tertiary and earlier subduction zones are intra-oceanic, with relatively simple scenarios for their triggering (Gurnis et al., 2004; Crameri et al., 2020; Lallemand and Arcay, 2021).
Passive margin collapse and subduction transference: Traditional, but rarely working
Since the inception of the plate-tectonic theory (Wilson, 1966; Dewey et al., 1973), it has been assumed that the primary mechanism of subduction initiation is collapse of the passive continental margin (Figure 1E) due to increasing negative buoyancy of the aging lithosphere in an opening ocean (Vlaar and Wortel, 1976; Davies, 1999). However, simultaneously with this gravitational instability, the strength of the oceanic plate is rapidly increasing, posing a serious obstacle to continental margin collapse (Cloetingh et al., 1982, 1989), even in the presence of sedimentary loading (Cloetingh et al., 1982, 1984) and/or additional forcing due to topographic gradients (Marques et al., 2013). Recently, the ever-growing strength of an aging passive continental margin has been challenged by theoretical arguments showing that the accumulation of ductile damage in the margin can critically lower its strength with time (Bercovici and Mulukova, 2021).
Although tectonic inversion of passive continental margins has been widely reported (Lundin and Doré, 2002; Lundin et al., 2013), none of these Atlantic-type margins has yet evolved into a subduction zone. Nevertheless, numerical modelling studies have continued to search for potential sites for future subduction at present-day passive margins, such as the mechanically weak southern Brazilian margin (Nikolaeva et al., 2011; Marques et al., 2013), the Argentine Basin and the U.S. East Coast, which are likely subject to plate suction from adjacent active, eastward-dipping subduction zones (Baes and Sobolev, 2017), as well as the East African margin (Gerya, 2011) thermally weakened by the African superplume (Mulibo and Nyblade, 2013; Koptev et al., 2015, 2018). However, despite numerical (Nikolaeva et al., 2010; Candioti et al., 2022) and laboratory (Faccenna et al., 1999; Auzemery et al., 2021) model inferences, no irrefutable natural example of initiated self-sustained subduction at a passive margin has yet been documented.
Subduction zone transference is a composite dynamic process that invokes both the termination of subduction by terrane collision/accretion and the onset of subduction on the backside of the colliding block (Figure 1F). When a buoyant block of lithosphere corresponding to an oceanic plateau or (micro)-continent collides with the trench, subduction comes to a local halt. However, convergence continues due to the new subduction zone with the same dip direction at the adjacent passive margin (Stern, 2004). As for the proposed scenario of passive margin collapse, subduction zone transference seems to carry a paradox in view of the temporal geotectonic evolution of the Earth. On the one hand, multiple ribbon-like (micro)-continents were repeatedly drifting away from Gondwana and subsequently accreted to the Eurasian continent with the re-establishment of an active subduction zone at their backside during the evolution of Tethyan systems since the Paleozoic (Yang, 2022). On the other hand, not a single ocean-continent subduction zone was triggered by subduction zone transference in Cenozoic time. The archetypal example of such a “failed” subduction zone transference is the collision between India and Eurasia, on-going since ∼50 Ma, which did not give rise to a new subduction zone along the southern Indian margin (Van Hinsbergen et al., 2019). Thus, subduction zone transference inferred from Paleozoic plate-tectonic reconstructions is surprisingly frequent (e.g., Torsvik, 2019; Wan et al., 2019), considering the lack of confirmed cases for this mechanism in the Cenozoic, which includes the best preserved geodynamic record (Yang, 2022). This process is also difficult to reproduce in numerical models, which generally show oceanward trench jumping over continued subduction (Tetreault and Buiter, 2012; Vogt and Gerya, 2014), rather than generating true subduction zone transference. Only for extreme conditions adopted in the experiments, requiring continued forced convergence instead of free subduction combined with a pre-defined weakness of the backward passive margin of the collided block, subduction can be initiated by subduction zone transference (Zhong and Li, 2020). In the case of the continental collision between India and Eurasia, it was the lack of sufficient weakness that prevented subduction initiation at the passive margin of India (Zhong and Li, 2022).
It appears, therefore, that the two most popular scenarios for triggering ocean-continent subduction face first-order challenges in terms of consistency with plate-tectonic reconstructions and reproducibility in numerical simulations.
Polarity reversal and lateral propagation: Viable alternatives
Subduction polarity reversal is triggered by the arrival of buoyant lithosphere of an adjacent continent (or a thick oceanic plateau) into the trench. As a result, the subducting and overriding plates exchange roles (Stern, 2004) and the initially overlying lithosphere starts to subduct (Figure 1G). Subduction polarity reversal has been demonstrated to be a viable mechanism to explain the possible formation of new subduction zones during the Cenozoic and earlier (see review by Yang (2022) and references therein). The Taiwan and Kamchatka orogens are classic and well-documented examples of the role of this process in the formation of new zones of ocean-continent subduction. The Taiwan orogen formed as a consequence of the oblique Miocene arc-continent collision between the Eurasian plate and the Philippine Sea plate, where the break-off of the originally southward-dipping Eurasian oceanic plate was followed by a change in subduction polarity that resulted in northward-dipping subduction of the Philippine Sea plate beneath the Eurasian continent (Teng et al., 2000; Clift et al., 2003; Ustaszewski et al., 2012). In the Kamchatka region, during the Paleocene, the oceanic part of the Eurasian plate subducted south-eastward under the Pacific Ocean. Subsequently, during the Eocene, the volcanic arc collided with the Eurasian continental margin, causing initiation of north-westward subduction of the oceanic Pacific lithosphere (Hourigan et al., 2009; Konstantinovskaya et al., 2011). It is important to note that the onset of subduction by polarity reversal may not be as common as the initiation of intra-oceanic subduction because of the different timescales of these processes: the time interval required to trigger intra-oceanic subduction by fault collapse or inversion is on the order of only 5 Myr (Nikolaeva et al., 2008; Duretz et al., 2016; Lallemand and Arcay, 2021), whereas it can take up to several tens of Myr for oceanic lithosphere to be consumed before proceeding to subduction beneath a continent (or oceanic plateau) through a change in subduction polarity.
Recently, several successful 2D (Crameri and Tackley, 2015; Sun et al., 2021; Almeida et al., 2022b) and 3D (Almeida et al., 2022a) numerical models have been developed that are capable of mimicking the key elements of subduction polarity reversal in intra-oceanic environments, inspired by the archetypal example of the Miocene collision of the Ontong Java Plateau with the Solomon Arc in the south-western Pacific Ocean (Cooper and Taylor, 1985; Petterson et al., 1999). In addition, a completely spontaneous reversal of subduction polarity in response to an arc-continent collision has been reproduced in a pioneering modelling study by Zhang and Leng (2021).
Another important advance in subduction initiation modelling was made by Zhou et al. (2020), who showed that a pre-existing intra-oceanic subduction zone can propagate laterally toward adjacent weak continental margins (Figure 1H). According to this study, a new subduction zone is expected to develop along the Northern American Atlantic coast, north of the Lesser Antilles. Possible passive margin weakening with its aging (Bercovici and Mulukova, 2021) may play critical role for enabling this scenario. Subduction propagation has also been inferred from tectonic reconstructions in various regions of the world, including the Indonesian subduction zone in the Banda arc region (Hall, 2012) and the subduction of the Nazca plate along the Andes (Chen et al., 2019). Subduction initiation along the western European margin may also arise from future westward migration of the Gibraltar slab into the east-central Atlantic (Duarte et al., 2013).
In view of the above, and differently from passive margin collapse and subduction transference, subduction polarity reversal and lateral propagation can be considered as viable modes of subduction initiation in ocean-continental tectonic settings.
Archetypal examples from the past and toward the future: how Farallon plate subduction closes the Atlantic Ocean
The Caribbean region provides a well-documented natural laboratory to serve as a test-bed for exploring the different scenarios of subduction initiation reviewed above (Figure 2). In Cretaceous time, north-eastward subduction of the Farallon plate (Figure 2A) was followed by collision of the Caribbean plateau—the thickened part of the oceanic lithosphere (Kerr et al., 2003) formed by magmatism associated with the Galapagos hotspot ∼90 Ma (Thompson et al., 2004)—with the Greater Antilles Arc and north-western South America at ∼80 Ma (Neil et al., 2011; Buchs et al., 2018). In the Greater Antilles, this collision caused subduction polarity reversal, resulting in the formation of a new subduction dipping in south-western direction below the Caribbean plateau (Figure 2B). A subsequent subduction transference (Figure 2C) formed the Central American Arc behind the oceanic plateau (Yang, 2022). The south-westward-dipping subduction of the Atlantic oceanic lithosphere is marked by a pronounced trench retreat (Munch et al., 2020), which allowed the insertion of the Caribbean plateau between the North and South American continents (Hoernle et al., 2002). As mentioned above, this narrow segment of intra-oceanic subduction in the central Atlantic may in the future extend northward (Figure 2D) toward the present passive margin of North America (Zhou et al., 2020). Such cascading subduction onsets, which took and might take place in different modes, shows how subduction on one side of the continent can effectively transform the passive margin along the opposite side of the same continent into an active margin. Global changes in the balance of plate-tectonic forces and plate motions triggered by passive to active margin transitions may eventually lead to the closure of entire oceanic basins such as the present-day Atlantic.
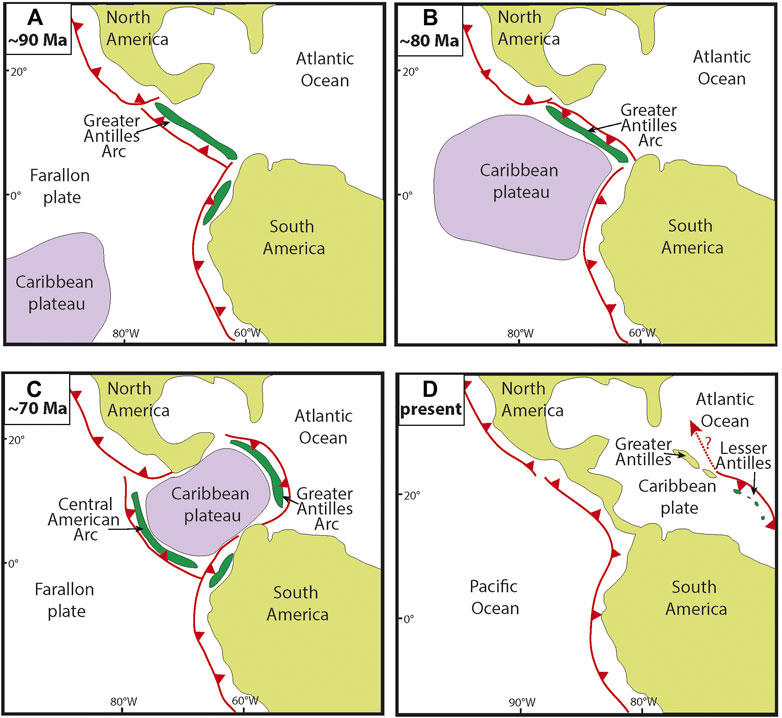
FIGURE 2. From subduction of the Farallon plate to closure of the Atlantic Ocean by a cascade of subduction initiations. (A–C) Geodynamic model for the evolution of the Caribbean region in Late Cretaceous: (A) north-eastward subduction of the Farallon plate; (B) collision of the Caribbean plateau accompanied by subduction polarity reversal; (C) subduction transference with formation of the Central American Arc behind the Caribbean plateau (from Yang, 2022). (D) Predicted development of a future ocean-continent subduction zone (eastern North America) by lateral propagation of the currently on-going Lesser Antilles subduction (Zhou et al., 2020).
As pointed out in our review, the initiation of a classic ocean-continent subduction zone is intrinsically linked to the precursor intra-oceanic subduction. At the same time, the triggering of intra-oceanic subduction seems to be a routine geodynamic process on Earth. We, therefore, conclude that intra-oceanic subduction plays a crucial role not only in plate-tectonic reorganizations in the oceans, but also in the initiation of ocean-continent subduction, key in the closure of oceanic basins and the entire Wilson cycle.
Author contributions
AK developed the conceptual idea and took the lead in writing the manuscript. Preparation of the manuscript was done by AK and SC with contributions from all co-authors. All authors discussed and commented on the manuscript at all stages.
Funding
We gratefully acknowledge support from the International Lithosphere Program (ILP) and the funding programme “Open Access Publikationskosten” Deutsche Forschungsgemeinschaft (DFG, German Research Foundation), project number 491075472. SC was supported by a research award from the Alexander von Humboldt Foundation.
Conflict of interest
The authors declare that the research was conducted in the absence of any commercial or financial relationships that could be construed as a potential conflict of interest.
Publisher’s note
All claims expressed in this article are solely those of the authors and do not necessarily represent those of their affiliated organizations, or those of the publisher, the editors and the reviewers. Any product that may be evaluated in this article, or claim that may be made by its manufacturer, is not guaranteed or endorsed by the publisher.
References
Agard, P., Yamato, P., Soret, M., Prigent, C., Guillot, S., Plunder, A., et al. (2016). Plate interface rheological switches during subduction infancy: Control on slab penetration and metamorphic sole formation. Earth Planet. Sci. Lett. 451, 208–220. doi:10.1016/j.epsl.2016.06.054
Almeida, J., Riel, N., Rosas, F. M., Duarte, J. C., and Kaus, B. (2022a). Self-replicating subduction zone initiation by polarity reversal. Commun. Earth Environ. 3, 55–11. doi:10.1038/s43247-022-00380-2
Almeida, J., Riel, N., Rosas, F. M., Duarte, J. C., and Schellart, W. P. (2022b). Polarity-reversal subduction zone initiation triggered by buoyant plateau obstruction. Earth Planet. Sci. Lett. 577, 117195. doi:10.1016/j.epsl.2021.117195
Arcay, D., Lallemand, S., Abecassis, S., and Garel, F. (2020). Can subduction initiation at a transform fault be spontaneous? Solid Earth. 11 (1), 37–62. doi:10.5194/se-11-37-2020
Auzemery, A., Willingshofer, E., Sokoutis, D., Brun, J. P., and Cloetingh, S. A. (2021). Passive margin inversion controlled by stability of the mantle lithosphere. Tectonophysics 817, 229042. doi:10.1016/j.tecto.2021.229042
Baes, M., Gerya, T., and Sobolev, S. V. (2016). 3-D thermo-mechanical modeling of plume-induced subduction initiation. Earth Planet. Sci. Lett. 453, 193–203. doi:10.1016/j.epsl.2016.08.023
Baes, M., Sobolev, S. V., Gerya, T., and Brune, S. (2020). Subduction initiation by plume-plateau interaction: Insights from numerical models. Geochem. Geophys. Geosyst. 21 (8), e2020GC009119. doi:10.1029/2020gc009119
Baes, M., and Sobolev, S. V. (2017). Mantle flow as a trigger for subduction initiation: A missing element of the Wilson cycle concept. Geochem. Geophys. Geosyst. 18 (12), 4469–4486. doi:10.1002/2017gc006962
Baes, M., Stern, R. J., Whattam, S., Gerya, T. V., and Sobolev, S. V. (2021). Plume-induced subduction initiation: Revisiting models and observations. Front. Earth Sci. (Lausanne). 9, 766604. doi:10.3389/feart.2021.766604
Beaussier, S. J., Gerya, T. V., and Burg, J. P. (2019). Near-ridge initiation of intraoceanic subduction: Effects of inheritance in 3D numerical models of the Wilson Cycle. Tectonophysics 763, 1–13. doi:10.1016/j.tecto.2019.04.011
Bercovici, D., and Mulyukova, E. (2021). Evolution and demise of passive margins through grain mixing and damage. Proc. Natl. Acad. Sci. U. S. A. 118 (4), e2011247118. doi:10.1073/pnas.2011247118
Boutelier, D., and Beckett, D. (2018). Initiation of subduction along oceanic transform faults: Insights from three-dimensional analog modeling experiments. Front. Earth Sci. (Lausanne). 6, 204. doi:10.3389/feart.2018.00204
Buchs, D. M., Kerr, A. C., Brims, J. C., Zapata-Villada, J. P., Correa-Restrepo, T., and Rodríguez, G. (2018). Evidence for subaerial development of the Caribbean oceanic plateau in the Late Cretaceous and palaeo-environmental implications. Earth Planet. Sci. Lett. 499, 62–73. doi:10.1016/j.epsl.2018.07.020
Candioti, L. G., Duretz, T., and Schmalholz, S. M. (2022). Horizontal force required for subduction initiation at passive margins with constraints from slab detachment. Front. Earth Sci. (Lausanne). 10, 785418. doi:10.3389/feart.2022.785418
Chen, Y. W., Wu, J., and Suppe, J. (2019). Southward propagation of Nazca subduction along the Andes. Nature 565 (7740), 441–447. doi:10.1038/s41586-018-0860-1
Clift, P. D., Schouten, H., and Draut, A. E. (2003). A general model of arc-continent collision and subduction polarity reversal from Taiwan and the Irish Caledonides. Geol. Soc. Lond. Spec. Publ. 219 (1), 81–98. doi:10.1144/gsl.sp.2003.219.01.04
Cloetingh, S. A. P. L., Wortel, M. J. R., and Vlaar, N. J. (1982). Evolution of passive continental margins and initiation of subduction zones. Nature 297 (5862), 139–142. doi:10.1038/297139a0
Cloetingh, S. A. P. L., Wortel, M. J. R., and Vlaar, N. J. (1984). Passive margin evolution, initiation of subduction and the Wilson cycle. Tectonophysics 109 (1-2), 147–163. doi:10.1016/0040-1951(84)90175-6
Cloetingh, S., Koptev, A., Kovacs, I., Gerya, T., Beniest, A., Willingshofer, E., et al. (2021). Plume-induced sinking of intracontinental lithospheric mantle: An overlooked mechanism of subduction initiation? Geochem. Geophys. Geosyst. 22 (2), e2020GC009482. doi:10.1029/2020gc009482
Cloetingh, S., Wortel, R., and Vlaar, N. J. (1989). On the initiation of subduction zones. Pure Appl. Geophys. 129, 7–25. doi:10.1007/bf00874622
Cooper, P. A., and Taylor, B. (1985). Polarity reversal in the Solomon Islands arc. Nature 314 (6010), 392–430. doi:10.1038/318392a0
Crameri, F. (2022). Breaking subductions’ fourth wall. Nat. Geosci. 15 (2), 95–96. doi:10.1038/s41561-022-00894-6
Crameri, F., Magni, V., Domeier, M., Shephard, G. E., Chotalia, K., Cooper, G., et al. (2020). A transdisciplinary and community-driven database to unravel subduction zone initiation. Nat. Commun. 11, 3750. doi:10.1038/s41467-020-17522-9
Crameri, F., and Tackley, P. J. (2015). Parameters controlling dynamically self-consistent plate tectonics and single-sided subduction in global models of mantle convection. J. Geophys. Res. Solid Earth 120 (5), 3680–3706. doi:10.1002/2014jb011664
Davies, J. H. (1999). The role of hydraulic fractures and intermediate-depth earthquakes in generating subduction-zone magmatism. Nature 398 (6723), 142–145. doi:10.1038/18202
Dewey, J. F., Pitmann, W. C., Ryan, W. B., and Bonnin, J. (1973). Plate tectonics and the evolution of the Alpine system. Geol. Soc. Am. Bull. 84 (10), 3137–3180. doi:10.1130/0016-7606(1973)84<3137:ptateo>2.0.co;2
Duarte, J. C., Rosas, F. M., Terrinha, P., Schellart, W. P., Boutelier, D., Gutscher, M. A., et al. (2013). Are subduction zones invading the Atlantic? Evidence from the southwest Iberia margin. Geology 41 (8), 839–842. doi:10.1130/g34100.1
Duretz, T., Agard, P., Yamato, P., Ducassou, C., Burov, E. B., and Gerya, T. V. (2016). Thermo-mechanical modeling of the obduction process based on the Oman ophiolite case. Gondwana Res. 32, 1–10. doi:10.1016/j.gr.2015.02.002
Dymkova, D., and Gerya, T. (2013). Porous fluid flow enables oceanic subduction initiation on Earth. Geophys. Res. Lett. 40 (21), 5671–5676. doi:10.1002/2013gl057798
Faccenna, C., Giardini, D., Davy, P., and Argentieri, A. (1999). Initiation of subduction at Atlantic-type margins: Insights from laboratory experiments. J. Geophys. Res. 104 (B2), 2749–2766. doi:10.1029/1998jb900072
Faccenna, C., Holt, A. F., Becker, T. W., Lallemand, S., and Royden, L. H. (2018). Dynamics of the Ryukyu/Izu-Bonin-Marianas double subduction system. Tectonophysics 746, 229–238. doi:10.1016/j.tecto.2017.08.011
Gerya, T. (2011). Future directions in subduction modeling. J. Geodyn. 52 (5), 344–378. doi:10.1016/j.jog.2011.06.005
Gerya, T. (2022). Numerical modeling of subduction: State of the art and future directions. Geosphere 18 (2), 503–561. doi:10.1130/ges02416.1
Gerya, T. V., Connolly, J. A., and Yuen, D. A. (2008). Why is terrestrial subduction one-sided? Geology 36 (1), 43–46. doi:10.1130/g24060a.1
Gerya, T. V., Stern, R. J., Baes, M., Sobolev, S. V., and Whattam, S. A. (2015). Plate tectonics on the Earth triggered by plume-induced subduction initiation. Nature 527 (7577), 221–225. doi:10.1038/nature15752
Gülcher, A. J., Beaussier, S. J., and Gerya, T. V. (2019). On the formation of oceanic detachment faults and their influence on intra-oceanic subduction initiation: 3D thermomechanical modeling. Earth Planet. Sci. Lett. 506, 195–208. doi:10.1016/j.epsl.2018.10.042
Gurnis, M., Hall, C., and Lavier, L. (2004). Evolving force balance during incipient subduction. Geochem. Geophys. Geosyst. 5 (7), Q07001. doi:10.1029/2003gc000681
Hall, C. E., Gurnis, M., Sdrolias, M., Lavier, L. L., and Müller, R. D. (2003). Catastrophic initiation of subduction following forced convergence across fracture zones. Earth Planet. Sci. Lett. 212 (1-2), 15–30. doi:10.1016/s0012-821x(03)00242-5
Hall, R. (2012). Late Jurassic–Cenozoic reconstructions of the Indonesian region and the Indian ocean. Tectonophysics 570, 1–41. doi:10.1016/j.tecto.2012.04.021
Hoernle, K., van den Bogaard, P., Werner, R., Lissinna, B., Hauff, F., Alvarado, G., et al. (2002). Missing history (16–71 Ma) of the Galápagos hotspot: Implications for the tectonic and biological evolution of the Americas. Geology 30 (9), 795–798. doi:10.1130/0091-7613(2002)030<0795:mhmotg>2.0.co;2
Hourigan, J. K., Brandon, M. T., Soloviev, A. V., Kirmasov, A. B., Garver, J. I., Stevenson, J., et al. (2009). Eocene arc-continent collision and crustal consolidation in Kamchatka, Russian Far East. Am. J. Sci. 309 (5), 333–396. doi:10.2475/05.2009.01
Karig, D. E. (1982). Initiation of subduction zones: Implications for arc evolution and ophiolite development. Geol. Soc. Lond. Spec. Publ. 10 (1), 563–576. doi:10.1144/gsl.sp.1982.010.01.37
Kerr, A. C., White, R. V., Thompson, P. M. E., Tarney, J., and Saunders, A. D. (2003). “No oceanic plateau—No Caribbean plate? The seminal role of an oceanic plateau in Caribbean plate evolution,” in The circum- gulf of Mexico and Caribbean region, hydrocarbon habitats, basin formation, and plate tectonics: Tulsa. Editors C. Bartolini, R. T. Buffler, and J. F. Blickwede (American Association of Petroleum Geologists Memoir), 79, 126–168. doi:10.1306/M79877C6
Konstantinovskaya, E. (2011). “Early Eocene arc-continent collision in Kamchatka, Russia: Structural evolution and geodynamic model” in Arc-continent collision (Berlin, Heidelberg: Springer), 247–277.
Koptev, A., Calais, E., Burov, E., Leroy, S., and Gerya, T. (2018). Along-axis variations of rift width in a coupled lithosphere-mantle system, Application to East Africa. Geophys. Res. Lett. 45 (11), 5362–5370. doi:10.1029/2018gl077276
Koptev, A., Calais, E., Burov, E., Leroy, S., and Gerya, T. (2015). Dual continental rift systems generated by plume–lithosphere interaction. Nat. Geosci. 8 (5), 388–392. doi:10.1038/ngeo2401
Lallemand, S., and Arcay, D. (2021). Subduction initiation from the earliest stages to self-sustained subduction: Insights from the analysis of 70 Cenozoic sites. Earth-Science Rev. 221, 103779. doi:10.1016/j.earscirev.2021.103779
Leng, W., Gurnis, M., and Asimow, P. (2012). From basalts to boninites: The geodynamics of volcanic expression during induced subduction initiation. Lithosphere 4 (6), 511–523. doi:10.1130/l215.1
Lundin, E., and Doré, A. G. (2002). Mid-Cenozoic post-breakup deformation in the ‘passive’ margins bordering the Norwegian–Greenland Sea. Mar. Petroleum Geol. 19 (1), 79–93. doi:10.1016/s0264-8172(01)00046-0
Lundin, E. R., Doré, A. G., Rønning, K., and Kyrkjebø, R. (2013). Repeated inversion and collapse in the Late Cretaceous-Cenozoic northern Vøring Basin, offshore Norway. Pet. Geosci. 19 (4), 329–341. doi:10.1144/petgeo2012-022
Maffione, M., Thieulot, C., Van Hinsbergen, D. J., Morris, A., Plümper, O., and Spakman, W. (2015). Dynamics of intraoceanic subduction initiation: 1. Oceanic detachment fault inversion and the formation of supra-subduction zone ophiolites. Geochem. Geophys. Geosyst. 16 (6), 1753–1770. doi:10.1002/2015gc005746
Marques, F. O., Cabral, F. R., Gerya, T. V., Zhu, G., and May, D. A. (2014). Subduction initiates at straight passive margins. Geology 42 (4), 331–334. doi:10.1130/g35246.1
Marques, F. O., Nikolaeva, K., Assumpção, M., Gerya, T. V., Bezerra, F. H., do Nascimento, A. F., et al. (2013). Testing the influence of far-field topographic forcing on subduction initiation at a passive margin. Tectonophysics 608, 517–524. doi:10.1016/j.tecto.2013.08.035
Matsumoto, T., and Tomoda, Y. (1983). Numerical simulation of the initiation of subduction at the fracture zone. J. Phys. Earth 31 (3), 183–194. doi:10.4294/jpe1952.31.183
Maunder, B., Prytulak, J., Goes, S., and Reagan, M. (2020). Rapid subduction initiation and magmatism in the Western Pacific driven by internal vertical forces. Nat. Commun. 11, 1874. doi:10.1038/s41467-020-15737-4
Mulibo, G. D., and Nyblade, A. A. (2013). Mantle transition zone thinning beneath eastern Africa: Evidence for a whole-mantle superplume structure. Geophys. Res. Lett. 40 (14), 3562–3566. doi:10.1002/grl.50694
Munch, J., Gerya, T., and Ueda, K. (2020). Oceanic crust recycling controlled by weakening at slab edges. Nat. Commun. 11, 2009. doi:10.1038/s41467-020-15750-7
Neill, I., Kerr, A. C., Hastie, A. R., Stanek, K. P., and Millar, I. L. (2011). Origin of the Aves Ridge and Dutch–Venezuelan Antilles: interaction of the Cretaceous ‘Great Arc' and Caribbean–Colombian Oceanic Plateau? J. Geol. Soc. Lond. 168 (2), 333–348. doi:10.1144/0016-76492010-067
Nikolaeva, K., Gerya, T. V., and Connolly, J. A. (2008). Numerical modelling of crustal growth in intraoceanic volcanic arcs. Phys. Earth Planet. Interiors 171 (1-4), 336–356. doi:10.1016/j.pepi.2008.06.026
Nikolaeva, K., Gerya, T. V., and Marques, F. O. (2011). Numerical analysis of subduction initiation risk along the Atlantic American passive margins. Geology 39 (5), 463–466. doi:10.1130/g31972.1
Nikolaeva, K., Gerya, T. V., and Marques, F. O. (2010). Subduction initiation at passive margins: Numerical modeling. J. Geophys. Res. 115 (3), B03406. doi:10.1029/2009jb006549
Petterson, M. G., Babbs, T., Neal, C., Mahoney, J., Saunders, A., Duncan, R., et al. (1999). Geological–tectonic framework of Solomon islands, SW pacific: Crustal accretion and growth within an intra-oceanic setting. Tectonophysics 301 (1-2), 35–60. doi:10.1016/s0040-1951(98)00214-5
Shuck, B., Gulick, S. P., Van Avendonk, H. J., Gurnis, M., Sutherland, R., Stock, J., et al. (2022). Stress transition from horizontal to vertical forces during subduction initiation. Nat. Geosci. 15 (2), 149–155. doi:10.1038/s41561-021-00880-4
Stern, R. J., and Bloomer, S. H. (1992). Subduction zone infancy: Examples from the Eocene Izu-Bonin-Mariana and Jurassic California arcs. Geol. Soc. Am. Bull. 104 (12), 1621–1636. doi:10.1130/0016-7606(1992)104<1621:szieft>2.3.co;2
Stern, R. J., and Gerya, T. (2018). Subduction initiation in nature and models: A review. Tectonophysics 746, 173–198. doi:10.1016/j.tecto.2017.10.014
Stern, R. J. (2004). Subduction initiation: Spontaneous and induced. Earth Planet. Sci. Lett. 226 (3-4), 275–292. doi:10.1016/s0012-821x(04)00498-4
Sun, B., Kaus, B. J., Yang, J., Lu, G., Wang, X., Wang, K., et al. (2021). Subduction polarity reversal triggered by oceanic plateau accretion: Implications for induced subduction initiation. Geophys. Res. Lett. 48 (24), e2021GL095299. doi:10.1029/2021gl095299
Teng, L. S., Lee, C. T., Tsai, Y. B., and Hsiao, L. Y. (2000). Slab breakoff as a mechanism for flipping of subduction polarity in Taiwan. Geology 28 (2), 155–158. doi:10.1130/0091-7613(2000)28<155:sbaamf>2.0.co;2
Tetreault, J. Á., and Buiter, S. J. H. (2012). Geodynamic models of terrane accretion: Testing the fate of island arcs, oceanic plateaus, and continental fragments in subduction zones. J. Geophys. Res. 117 (8), B08403. doi:10.1029/2012jb009316
Thompson, P. M., Kempton, P., White, R., Kerr, A., Tarney, J., Saunders, A., et al. (2004). Hf–Nd isotope constraints on the origin of the Cretaceous Caribbean plateau and its relationship to the Galapagos plume. Earth Planet. Sci. Lett. 217 (1-2), 59–75. doi:10.1016/s0012-821x(03)00542-9
Torsvik, T. H. (2019). Earth history: A journey in time and space from base to top. Tectonophysics 760, 297–313. doi:10.1016/j.tecto.2018.09.009
Toth, J., and Gurnis, M. (1998). Dynamics of subduction initiation at preexisting fault zones. J. Geophys. Res. 103 (B8), 18053–18067. doi:10.1029/98jb01076
Ueda, K., Gerya, T., and Sobolev, S. V. (2008). Subduction initiation by thermal–chemical plumes: Numerical studies. Phys. Earth Planet. Interiors 171 (1-4), 296–312. doi:10.1016/j.pepi.2008.06.032
Ustaszewski, K., Wu, Y. M., Suppe, J., Huang, H. H., Chang, C. H., and Carena, S. (2012). Crust–mantle boundaries in the Taiwan–Luzon arc-continent collision system determined from local earthquake tomography and 1D models: Implications for the mode of subduction polarity reversal. Tectonophysics 578, 31–49. doi:10.1016/j.tecto.2011.12.029
Uyeda, S., and Ben-Avraham, Z. (1972). Origin and development of the Philippine Sea. Nat. Phys. Sci. 240 (104), 176–178. doi:10.1038/physci240176a0
Van Hinsbergen, D. J., Lippert, P. C., Li, S., Huang, W., Advokaat, E. L., and Spakman, W. (2019). Reconstructing Greater India: Paleogeographic, kinematic, and geodynamic perspectives. Tectonophysics 760, 69–94. doi:10.1016/j.tecto.2018.04.006
Van Hinsbergen, D. J., Peters, K., Maffione, M., Spakman, W., Guilmette, C., Thieulot, C., et al. (2015). Dynamics of intraoceanic subduction initiation: 2. Suprasubduction zone ophiolite formation and metamorphic sole exhumation in context of absolute plate motions. Geochem. Geophys. Geosyst. 16 (6), 1771–1785. doi:10.1002/2015gc005745
Vlaar, N. J., and Wortel, M. J. R. (1976). Lithospheric aging, instability and subduction. Tectonophysics 32 (3-4), 331–351. doi:10.1016/0040-1951(76)90068-8
Vogt, K., and Gerya, T. V. (2014). From oceanic plateaus to allochthonous terranes: Numerical modelling. Gondwana Res. 25 (2), 494–508. doi:10.1016/j.gr.2012.11.002
Wan, B., Wu, F., Chen, L., Zhao, L., Liang, X., Xiao, W., et al. (2019). Cyclical one-way continental rupture-drift in the Tethyan evolution: Subduction-driven plate tectonics. Sci. China Earth Sci. 62 (12), 2005–2016. doi:10.1007/s11430-019-9393-4
Whattam, S. A., and Stern, R. J. (2011). The ‘subduction initiation rule’: A key for linking ophiolites, intra-oceanic forearcs, and subduction initiation. Contrib. Mineral. Pet. 162 (5), 1031–1045. doi:10.1007/s00410-011-0638-z
Wilson, J. T. (1966). Did the Atlantic close and then re-open? Nature 211, 676–681. doi:10.1038/211676a0
Yang, G. (2022). Subduction initiation triggered by collision: A review based on examples and models. Earth-Science Rev. 232, 104129. doi:10.1016/j.earscirev.2022.104129
Zhang, S., and Leng, W. (2021). Subduction polarity reversal: Induced or spontaneous? Geophys. Res. Lett. 48 (11), e2021GL093201. doi:10.1029/2021gl093201
Zhong, X., and Li, Z. H. (2020). Subduction initiation during collision-induced subduction transference: Numerical modeling and implications for the Tethyan evolution. J. Geophys. Res. Solid Earth 125 (2), e2019JB019288. doi:10.1029/2019jb019288
Zhong, X., and Li, Z. H. (2022). Wedge-shaped southern Indian continental margin without proper weakness hinders subduction initiation. Geochem. Geophys. Geosyst. 23 (2), e2021GC009998. doi:10.1029/2021gc009998
Zhou, X., Li, Z. H., Gerya, T. V., and Stern, R. J. (2020). Lateral propagation–induced subduction initiation at passive continental margins controlled by preexisting lithospheric weakness. Sci. Adv. 6 (10), eaaz1048. doi:10.1126/sciadv.aaz1048
Zhou, X., Li, Z. H., Gerya, T. V., Stern, R. J., Xu, Z., and Zhang, J. (2018). Subduction initiation dynamics along a transform fault control trench curvature and ophiolite ages. Geology 46 (7), 607–610. doi:10.1130/g40154.1
Zhou, X., and Wada, I. (2021). Differentiating induced versus spontaneous subduction initiation using thermomechanical models and metamorphic soles. Nat. Commun. 12, 4632. doi:10.1038/s41467-021-24896-x
Keywords: subduction initiation, transform fault collapse, passive margin collapse, subduction transference, polarity reversal, lateral propagation, Caribbean plateau, Atlantic Ocean
Citation: Koptev A, Cloetingh S, Gerya T, Sternai P and Botsyun S (2022) Ocean-continent subduction cannot be initiated without preceding intra-oceanic subduction!. Front. Earth Sci. 10:1097922. doi: 10.3389/feart.2022.1097922
Received: 14 November 2022; Accepted: 07 December 2022;
Published: 21 December 2022.
Edited by:
Roberto Scarpa, University of Salerno, ItalyReviewed by:
Joao C. Duarte, University of Lisbon, PortugalCopyright © 2022 Koptev, Cloetingh, Gerya, Sternai and Botsyun. This is an open-access article distributed under the terms of the Creative Commons Attribution License (CC BY). The use, distribution or reproduction in other forums is permitted, provided the original author(s) and the copyright owner(s) are credited and that the original publication in this journal is cited, in accordance with accepted academic practice. No use, distribution or reproduction is permitted which does not comply with these terms.
*Correspondence: Alexander Koptev, YWxleGFuZGVyLmtvcHRldkBnZnotcG90c2RhbS5kZQ==