- 1College of Earth Science and Engineering, Shandong University of Science and Technology, Qingdao, China
- 2Weihai Earthquake Monitoring Center, Weihai, China
- 3Yantai Earthquake Monitoring Center Station, Shandong Earthquake Agency, Yantai, China
- 4National Institute of Natural Hazards, Ministry of Emergency Management of China, Beijing, China
- 5Jiangsu Earthquake Agency, Nanjing, China
Water temperatures of hot springs close to tectonic fault zones often show some variations before earthquakes, and analyses of earthquake precursors in hot springs have significant referential meaning for earthquake monitoring and forecasting. This study measured the concentration of major ions in water from the Hongshuilantang Hot Spring in 2017 and 2020. The ion composition was classified by hydrochemistry into the HCO3·SO4-Na chemical type. The composition of hydrogen and oxygen isotopes in the Hongshuilantang Hot Spring were located near the global meteoric water line (GMWL), indicating that the recharge source of the hot spring was meteoric water. The δD and δ18O values were not plotted on the Glogal Meteroric Water Line (GMWL), and there were some deviations, which suggested that hot spring water underwent water–rock interactions. Deep circulation water played an important role during the evolution process of thermal water. Water temperature showed a decreasing trend from October 2013 to June 2015 during the Rushan earthquake swarm in eastern China. Because of the occurrence of the earthquake swarm, we inferred that regional stress in this area began to be released, allowing continuous rebalancing. Free surface water appeared in some aquifers, and the seepage of low-temperature underground water into the upper aquifer led to a drop in water temperature in the hot spring. The Hongshuilantang Hot Spring and the epicenter of the Rushan earthquake swarm were located on the Muping–Jimo seismological fault zone, with the same seismotectonic system and some genesis relationships.
Introduction
Geothermal resources have significant economic and social value and are clean and recyclable (Tiwari et al., 2020; Lai et al., 2021; Verma et al., 2021). The study of hot springs is important and indispensable for the development and utilization of geothermal resources (Luan and Li, 1993; Wang et al., 2011). In recent years, hot springs have been widely used for seismic monitoring and forecasting, including the observation and analysis of ion concentrations and isotope compositions in hot springs that are close to fault zones (Gao et al., 2015; Che et al., 2016; Zhang et al., 2021). Seismic precursors, co-seismic responses, and the tidal effects of water temperatures have been recorded in a number of hot springs and geothermal wells (Mogi et al., 1989; Che et al., 2014; Liu et al., 2015; Ma et al., 2015; Miyakoshi et al., 2020). Anomalies in the water temperature of thermal water can be critical and especially useful earthquake precursors (Ma, 2016). For seismic fluid geochemistry, the determination of hydrogeochemical characteristics and measurement of stable hydrogen and oxygen isotopes is a promising and important method for earthquake monitoring and forecasting (Favara et al., 2001; Claesson et al., 2004; Pope et al., 2014; Skelton et al., 2014; Li et al., 2019; Miyakoshi et al., 2020). A large amount of useful information on the origins and migrations of thermal water can be obtained from hydrochemical compositions and environmental isotopes, which provides the basis for determining geochemical characteristics, such as hydrochemical type, genesis, and stage of water–rock reactions (Tiwari et al., 2020; Lai et al., 2021; Sasaki et al., 2021; Song et al., 2021; Su et al., 2021; Verma et al., 2021; Zhang et al., 2021; Duan et al., 2022).
The Jiaodong Peninsula, Shandong Province, eastern China, is located in the eastern part of the Penglai–Weihai fault zone with extensive distributions of metamorphic rocks and granites that have relatively high terrestrial heat flow values. Hot springs are widely distributed in the Jiaodong Peninsula (Jin et al., 2000). The Hongshuilantang Hot Spring is located in the eastern part of the Jiaodong Peninsula, which is a continental margin region of the Middle-Cenozoic crustal tectonic activity zone (Luan and Li, 1993; Jin et al., 2000; Zhou et al., 2002). A great number of moderate-to-strong earthquake swarms have occurred in the vicinity of these areas (Chen et al., 2006; Gu et al., 2020); therefore, Hongshuilantang Hot Spring has been explored as a potential seismic monitoring well by the local earthquake administration in order to capture valuable information related to earthquake precursors. A total of 7420 earthquakes with magnitude >1.0 occurred during the Rushan earthquake swarm from 1 October 2013 to 30 June 2015; the strongest earthquake was ML 5.0 on 22 May 2015. Earthquakes caused cracks in some houses in local rural areas and had a great impact on the residents of Rushan city. During the Rushan earthquake swarm, the water temperature of Hongshuilantang Hot Spring appeared to decrease, so it is very important to study the relationship between earthquake swarm and water temperature. Due to the relatively abundant hot springs and frequent seismic activities in this region, it is an ideal site for research on the relationship between deep geofluids and earthquakes. Much research on the geochemistry of hot springs for the purposes of geothermal development has been carried out in the study area and its surroundings (Luan and Li, 1993; Shangguang et al., 1998; Jin et al., 2000; Wang et al., 2011). However, few studies on hot spring geochemistry have been carried out on seismic monitoring and forecasting. In this paper, the anomalies in water temperatures in the hot spring during the period of the Rushan earthquake swarm were analyzed, and possible relationships between temperature changes in the Hongshuilantang Hot Spring and the Rushan earthquake swarm were discussed together with background information on the geological structure. Combined with hydrochemical compositions, environmental isotopes, and geological and geomorphological investigations, a model map of the genesis of the hot spring was built. The results of this paper provide an important reference for exploring the genesis and earthquake precursor anomalies of geothermal wells and hot springs in other areas.
Settings
Geological settings
The Jiaodong Peninsula, with its many northeast-trending tectonic faults, is located in the northeastern part of the North China Block. It is not only an area with frequent moderate-to-strong earthquakes in the North China Earthquake Zone but also a continental margin zone exhibiting Middle-Cenozoic crustal tectonic activities (Figure 1). The Tanlu fault zone, a NE-trending deep fault zone running through the western part of the Jiaodong Peninsula in eastern China, crosses the Bohai Sea in the north, connects to the lower Liaohe fault zone, and ends at the Yangtze River in the south. The main tectonic evolution pattern of the Tanlu fault zone in recent years has largely comprised compressional activity with right-sided strike–slip characteristics (Pan et al., 2015; Qu et al., 2021). The largest earthquake along the Tanlu fault zone was the 1668 Tancheng 8.5 earthquake. The Penglai–Weihai fault zone, an NW-trending deep fault, is located in the northern part of the Jiaodong Peninsula and controls the distribution of seismic activities (Chen et al., 2006; Zhu G.et al., 2018; Gu et al., 2020). The western segment of the Penglai–Weihai fault zone was active in the late Pleistocene, and the eastern part of it was active in the mid-Pleistocene with no late-Pleistocene offset found. It is considered that the intersection of the Penglai–Weihai fault zone and the NE-striking branch faults of the Tanlu fault zone is the epicenter for medium-to-strong earthquakes, such as the Weihai M6 and the Bohai M7 (Figure 2B, Wang et al., 2006). The NE-trending fault zone in the study area includes the Wulian–Yantai fault zone, a northern boundary of the Sulu orogenic belt, and the Muping–Jimo fault zone, an important NE-oriented fracture (Zhou et al., 2002).
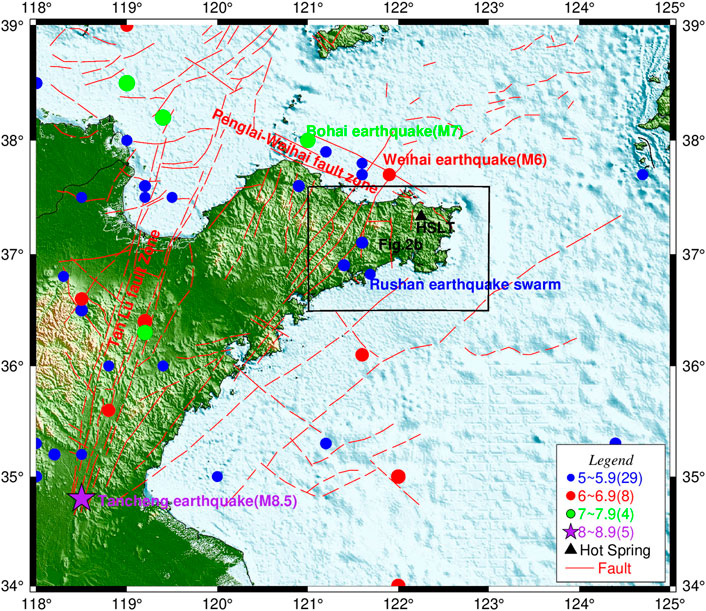
FIGURE 1. Seismotectonic settings of the Hongshuilantang Hot Spring in Jiaodong Peninsula, China. (Data of faults and earthquakes are from Chao et al., 1997).
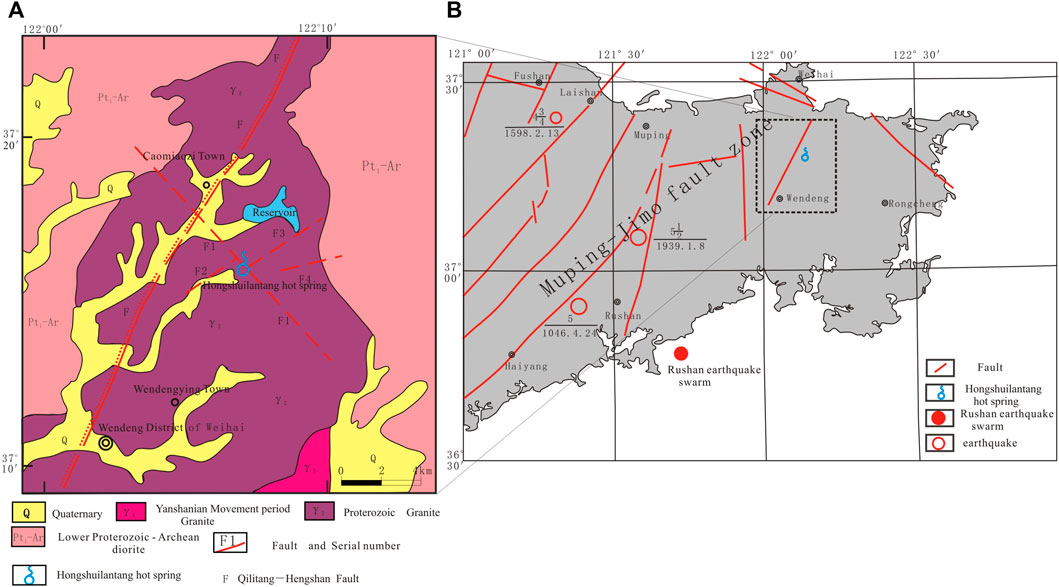
FIGURE 2. Geological settings and regional location of Hongshuilantang Hot Spring. (A) Geological settings; (B) regional location.
Hongshuilantang Hot Spring, on the east side of the Qilitang–Hengshan fault and close to the Muping–Jimo fault zone, is located in the eastern part of the Jiaodong Peninsula (Luan and Li, 1993; Jin et al., 2000; Zhou et al., 2002). (Figure 2A). The NW-trending Qilitang–Hengshan fault (F1) and the three inferred faults (F2, F3, and F4) may control the development of the Hongshuilantang Hot Spring.
Climatic settings
The hot spring explored in this study is located in the northeast of Shandong Province in northern China (Figure 1). It has a marine monsoon climate, with an average annual precipitation of 740 mm and an average annual temperature of about 12.0°C. Because of the influence of its land and sea location, topography, and other factors, there is more precipitation in summer. As it is surrounded by the sea on three sides and traversed by mountains from east to west, there is a large amount of strong convective weather and heavy snowfall in winter. The amount of precipitation in spring (from March to May), summer (from June to August), autumn (from September to November), and winter (from December to February) accounts for 15%, 53%, 25%, and 7% of total annual precipitation, respectively (Liu, 2021).
Methods and data
Hydrochemical characteristics and δD and δ18O stable isotopes
To determine the genesis of water temperature anomalies, water samples were collected from the Hongshuilantang Hot Spring in March 2017 and June 2020. Chemical ion concentrations and δD and δ18O stable isotope compositions of water samples were measured simultaneously. High-density polyethylene bottles with a 50 ml capacity were used as sample containers. Before sampling, the bottles were rinsed three times with the water samples, and at least three bottles of each water sample were collected for analysis. The bottles were filled with water samples, making sure there were no air bubbles, and the bottle mouths were sealed with a sealing film as quickly as possible. The chemical ion concentrations and δD and δ18O stable isotope compositions were measured in the Underground Fluid Dynamics Laboratory Unit, Key Laboratory of Crustal Dynamics of the China Earthquake Administration. Volumetric methods and CIC-200 ion chromatography were used for hydrochemistry analysis. δD and δ18O stable isotopes were measured with an LGR 912-0008 hydrogen–oxygen stable isotope analyzer calibrated with water standards GBW04458, GBW04459, and GBW04460.
Long-term observation of water temperature
Before seismological observations were begun, the Hongshuilantang Hot Spring was an artesian well. The hot spring cannot flow on its own because of the decrease in the water table. However, when pumped, the hot spring can flow on its own. At present, water from the hot spring is mainly used for freshwater aquaculture by local residents. The Hongshuilantang Hot Spring is a seismic observation well belonging to the Weihai Earthquake Monitoring Center. The station observer uses a thermometer to measure and record the water temperature of the hot spring once a day. Temperature observations began in 2001, meaning we have data covering 21 years.
Thermometry
In this study, Na/K, K/Mg, and SiO2 thermometries were selected to calculate the temperature of thermal reservoirs (Jiang et al., 2022).
Na/K thermometry used the following formula (Tian, 2012; Shi et al., 2019; Jiang et al., 2022):
K/Mg thermometry used the following formula (Tian, 2012; Jiang et al., 2022):
Silica thermometry used the following formula (Tian, 2012; Jiang et al., 2022; Shi et al., 2022):
where C is the concentration of SiO2 in thermal waters, C1 is the concentration of Na+, C2 is the concentration of K+, and C3 is the concentration of Mg2+.
Estimation of circulation depth
The circulation depth of the Hongshuilantang Hot Spring is given by the following formula (Jin et al., 2000; Tian, 2012):
where H is the circulation depth of hot spring water (m), r is the geothermal gradient, t is the temperature of the reservoir, t0 is the average annual temperature of the recharge area, and h is the depth of the local normal temperature zone over a period of many years.
Results
The hydrochemical characteristics and the δD and δ18O stable isotope compositions are shown in Table 1 and Table 2. To determine the genesis of the Hongshuilantang Hot Spring, we used data from other published studies (Jin et al., 2000; Wang et al., 2011; Tian, 2012; Tian, 2015).
Chemical type of hot spring water
Based on data measured in this paper and collected from published studies (Jin et al., 2000; Tian, 2012), a Piper diagram was plotted (Figure 3). The spring water was classified as an HCO3·SO4-Na type. The content of HCO3− in hot springs is relatively high; therefore, we hypothesized that the recharge source may be from surface water. The concentrations of Cl− and SO42− also have implied meanings for the depth of groundwater circulation, retention time, and recharge path.
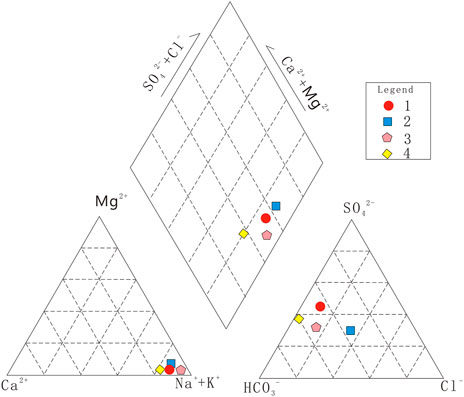
FIGURE 3. Piper diagram of waters from Hongshuilantang Hot Spring. The numbers in the figure correspond to those in Table 1.
Water–rock reaction characteristics
The Na–K–Mg diagram shows that water samples from the studied hot spring are near the Mg-end (Figure 4) and the equilibrium line, which indicates that the hot spring waters can be classified as immature. The water–rock interactions have not yet reached the state of ion equilibrium, indicating that the water is from a shallow, cold pool.
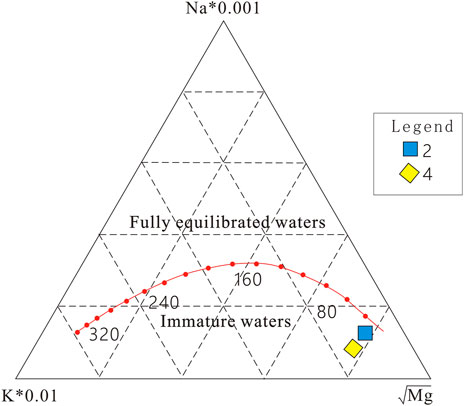
FIGURE 4. Na–K–Mg diagram of the Hongshuilantang Hot Spring. The numbers in the figure correspond to those in Table 1.
δD and δ18O stable isotope characteristics
Environmental isotope analysis has been widely used in hydrogeological research. Measuring hydrogen and oxygen isotope levels is an effective method for ascertaining the origin and migration of water and other fluids circulating within the Earth (Tiwari et al., 2020; Li et al., 2021; Sasaki et al., 2021; Duan et al., 2022). Evaporation and precipitation can result in significant discrepancies in the isotopic composition of the mass of water molecules (Li et al., 2021; Duan et al., 2022). The δD and δ18O values of freshwater and seawater in Weihai vary significantly from place to place, while the isotope levels in the river and thermal water were similar (Table 2).
Based on data obtained in this study and from other published work by Tian (2012) and Tian (2015), the values of the stable isotopes, δD and δ18O, in the hot spring water fell into the GMWL, and a rightward drift occurred to some extent (Figure 5). The GMWL could reflect the hydrogen and oxygen isotopic composition of precipitation in many marine monsoon climate areas. The nearer the origin is to the coastal areas, the closer its δD and δ18O stable isotope values are to the GMWL; therefore, the GMWL was used as a reference for the δD and δ18O stable isotope characteristics in the study (Craig, 1961; Li et al., 2011). There may be two reasons for the rightward drift of δD and δ18O stable isotope compositions seen in the δD and δ18O value graph. One is that Rayleigh fractionation occurs in arid and semi-arid areas of low precipitation and strong evaporation, which leads to an increase in δ18O values. The other is that water–rock reactions between groundwater and siliceous or carbonate rocks cause oxygen drift and equilibrium exchange between hydrogen and oxygen isotopes. Combined climatic and geological conditions around the studied hot spring revealed that water–rock interactions in deep circulation systems may be the main reason for the appearance of δ18O drift. To sum up, the main recharge resources of the Hongshuilantang Hot Springs are meteoric water, surface water, and groundwater.
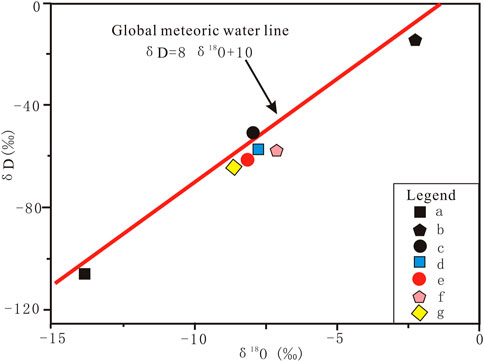
FIGURE 5. Relationships between δD and δ18O values of the hot spring and the global meteoric water line (GMWL). The numbers in the diagram correspond to those in Table 2 and the GMWL is from Craig (1961).
Circulation depth of hot spring water
Na/K thermometries of thermal waters from the hot spring were 193°C and 185°C. K/Mg thermometries were 66°C and 68°C. Silica thermometry was 135°C (Shi et al., 2019; Shi et al., 2022). Since Na+ and K+ ions do not reach equilibrium easily and water–rock interactions were still in process, the temperatures calculated by the Na/K temperature scale were higher than the true values; therefore, silica thermometry was used to estimate the thermal reservoir temperatures, which should be closer to the actual value (Shi et al., 2019; Shi et al., 2022). In this study, formula (4) was used to estimate the circulation depth in the Hongshuilantang Hot Spring (Jin et al., 2000; Tian, 2012). In the equation, r is the geothermal gradient (4.4°C/100 m close to Huangxian basin in Jiaodong Peninsula), t is the selected silica thermometry of 135°C, and t0 is the annual average temperature in the recharge areas; adopting 12.1°C, h is the depth of the local normal temperature zone for multiple years, which is equal to 25 m. Based on the aforementioned assumptions, the circulation depth was calculated to be about 2793 m.
Response to earthquakes
The water temperatures in the hot spring dropped from 76 to 70°C between October 2013 and June 2015, representing a maximum decrease of 6°C. After June 2015, the water temperature gradually began to increase, returning to September 2013 values by November 2015, when the whole water temperature oscillation process was over (Figure 6). The Rushan earthquake swarm was accompanied by continuous aftershocks from the ML 3.2 earthquake on 1 October 2013. As of 30 June 2015, Rushan had experienced 5997 earthquakes with magnitudes of 0.0 ≤ ML ≤ 0.9, 1216 earthquakes with a magnitude of 1.0 ≤ ML ≤ 1.9, 182 earthquakes with a magnitude of 2.0 ≤ ML ≤ 2.9, 21 earthquakes with a magnitude of 3.0 ≤ ML ≤ 3.9, three earthquakes with a magnitude of 4.0 ≤ML ≤ 4.9, and one earthquake with a magnitude of ML ≥ 5.0. The ML 5.0 earthquake hit Rushan on 22 May 2015.
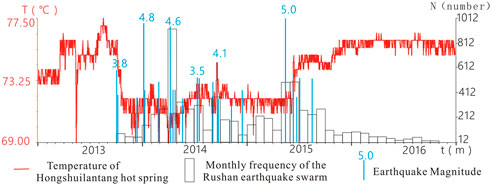
FIGURE 6. Comparison chart of daily temperature variations of the Hongshuilantang Hot Spring and monthly frequency of the Rushan earthquake swarm from 1 Jan 2013 to 31 Dec 2016.
Discussion
Genesis model of the Hongshuilantang Hot Spring
Considering the hydrochemical characteristics, the Na–K–Mg diagram, and the δD and δ18O stable isotope levels, we concluded that the Hongshuilantang Hot Spring possesses properties of both shallow and deep circulation water. The reason for this complex origin is that the chemical composition of hot spring water is closely related to burial conditions, surrounding rock lithology, recharge conditions of the hot spring, etc., (Shangguang et al., 1998; Jin et al., 2000). Combining the results of the geological and geomorphological investigations, a genesis model map of the Hongshuilantang Hot Spring was created (Figure 7), illustrating that it is not only affected by shallow meteoric water but also by deep circulation water.
Relative motion of the blocks in the vicinity
Based on continuous GPS observation data, Zhu et al. (2018) analyzed the relative motion state of the block continuum on both sides of the Tanlu fault zone after the 2011 Mw 9.0 earthquake in Japan through a sliding block model. The study suggested that the occurrence of the Rushan earthquake swarm may be related to the local regional stress adjustment promoted by the relative motion of the blocks on each side of the Tanlu fault zone. The enhanced relative motion from September 2013 to September 2015, which is vertical to the deep fault zone, promoted the release of the concentrated energy by means of the Rushan earthquake swarm. The vertical motion of the blocks on each side of the Tanlu Fault Zone has decelerated since February 2016, and at the same time, the activity of the Rushan earthquake swarm was also significantly weakened (Zhu C.et al., 2018). The epicenter of the Rushan earthquake swarm and the Hongshuilantang Hot Spring are both located in the Muping–Iimo Fault Zone on the eastern side of the Tanlu Fault Zone and belong to the Muping–Iimo Fault Zone, implying that they should be affected by the same stress conditions. Local stress adjustments also contributed to the variations in pore pressure of ground fluids, which affected the water temperature of the Hongshuilantang Hot Spring (Igarashi and Wakita, 1990; Lai et al., 2021).
Relationships between water temperature anomalies and the Rushan earthquake swarm
As shown in Figure 3, the water temperature of the hot spring declined from 76°C to approximately 70°C, with a maximum drop of 7°C after the Rushan ML3.8 earthquake on 1 October 2013. After the Rushan ML 5.0 earthquake on 22 May 2015, the number of earthquakes showed a decreasing trend. The data indicated that the water temperature began to increase from June 2015 and slowly returned to 76°C. As of the end of March 2017, the water temperature had remained stable. There appeared to be a temporal correlation between the Rushan earthquake swarm and the temperature drop in the hot spring. In addition, there was a close relationship between the monthly earthquake frequency during the swarm and the decrease in water temperature. When earthquakes of ML 4.0 or greater magnitude were occurring in the Rushan earthquake swarm, water temperatures dropped significantly. For example, in January and April 2014, water temperatures of Hongshuilantang Hot Spring dropped by 7°C. The ML 4.8 earthquake on 7 January 2014 and the ML 4.6 earthquake on 4 April 2014 occurred during this period, and the highest monthly frequency of 917 earthquakes was recorded during the Rushan earthquake swarm in April 2014. The highest monthly frequency of earthquakes corresponded to the largest drop in water temperature (Figures 6, 8).
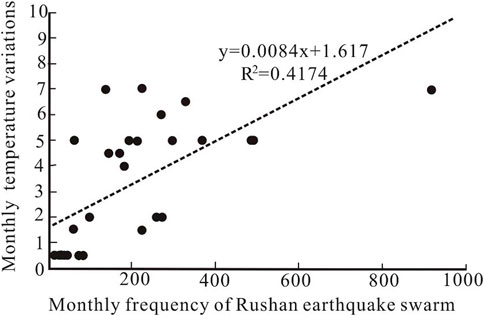
FIGURE 8. Correlation of monthly temperature variations of the Hongshuilantang Hot Spring and monthly frequencies of the Rushan earthquake swarm.
The epicenter of the Rushan earthquake swarm and the Hongshuilantang Hot Spring were located in the Jiaodong shield of the Jiaoliao fault block. According to the spatial distribution of local seismic faults, the Hongshuilantang Hot Spring and the epicenter of the Rushan earthquake swarm were both located in the Muping–Jimo fault zone (Figure 2B), suggesting a certain genesis relationship in seismic structure.
The results of the on-site investigation and verification showed no obvious changes in the surrounding observation conditions; therefore, the impact of environmental disturbances on the hot spring water temperature could be ignored. A large number of early Middle Pleistocene and pre-quaternary faults are scattered in the Jiaodong Peninsula. The Weihai M6.0 earthquake, which was the strongest earthquake in the history of the area, occurred in the northwest coastal part in 1948. Earthquakes in the Jiaodong Peninsula and its adjacent areas often appeared in the form of earthquake swarms, and the process of energy release was relatively slow (Zhou et al., 2002). The stress changes in the crustal medium and the water circulation in hot springs and aquifers resulted in water temperature variations due to stress increase or unloading. Thus, fluctuations of groundwater temperature could be caused by seepage from hot spring and aquifer systems (Yu and Che, 1997; Sun and Liu 2006). A study of the Xiangcheng Hot Spring also showed that stress changes could lead to connections between two adjacent hot spring aquifers, resulting in variations of water temperature (Ma, 2016).
Conclusion
1) The analysis of water quality, Na–K–Mg thermometry, and δD and δ18O stable isotope levels shows that Hongshuilantang Hot Spring is not only affected by shallow meteoric water but also by deep circulating water.
2) Both the Hongshuilantang Hot Spring and the epicenter of the Rushan earthquake swarm are located in the Muping–Jimo fault zone, suggesting that they have a certain genesis relationship in seismic structure.
3) The stress increased slowly before the occurrence of the Rushan earthquake swarm, which was a continuously increasing process that changed monotonously. During this process, there were no obvious changes in water temperature caused by the Hongshuilantang Hot Spring; when the Rushan earthquake swarm occurred, the stress began to be released. When a free water surface appeared in the aquifer, the water temperatures of the hot spring decreased owing to the seepage of low-temperature underwater into the upper layer of the aquifer under the action of seismic stress unloading. After stress release, the water temperature gradually returned to normal.
Data availability statement
The original contributions presented in the study are included in the article/Supplementary Material; further inquiries can be directed to the corresponding author.
Author contributions
GD: methodology, data curation, and writing—original draft preparation. SS: methodology, data curation, supervision, conceptualization, writing—review & editing. XC: writing and reviewing. HR: methodology, data curation, validation, writing— review and editing. ZH: writing— review and editing. XZ: editing.
Funding
This study was jointly funded by the National Natural Science Foundation of China (No. 42277196), the National Natural Science Foundation of China (No. 41877299), the National Science Foundation of China (No. 42073063), the Program of Monitoring, Forecasting and Scientific Research, China Earthquake Administration (3JH-202001057), the Basic Scientific Research Program of Shandong Earthquake Agency (No. YB2220), the National Key Research and Development Program of China (No. 2019YFC1509203), and the Basic Science Research Plan of the Institute of Earthquake Science, China Earthquake Administration (No. 2020IEF0704). This work is a contribution to IGCP Project 724.
Acknowledgments
Some figures were made using Generic Mapping Tools (https://forum.genericmapping-tools.org/, last accessed March 2020) (Wessel et al., 2013).
Conflict of interest
The authors declare that the research was conducted in the absence of any commercial or financial relationships that could be construed as a potential conflict of interest.
Publisher’s note
All claims expressed in this article are solely those of the authors and do not necessarily represent those of their affiliated organizations, or those of the publisher, the editors, and the reviewers. Any product that may be evaluated in this article, or claim that may be made by its manufacturer, is not guaranteed or endorsed by the publisher.
References
Chao, H., Wang, Q., and Li, J., 1997. Seismotectonic map of Shandong Province, new tectonic map of Shandong Province and instructions, China, Press, S.M, 100–132.
Che, Y., He, A., and Yu, J. (2014). Mechanisms of water-heat dynamics and earth-heat dynamics of well water temperature micro-behavior. Acta Seismol. sin. 36, 106–117. doi:10.3969/j.issn.0253-3782.2014.01.009
Che, Y., Yu, J., Chen, Q., Deng, Y., and Li, J. (2016). A thought on the establishment of the fault gas monitoring network-Further discussion on searching new approaches to the short/imminent earthquake prediction. Recent Dev. World Seismol. 12. doi:10.3969/j.issn.0235-4975.2016.12.004
Chen, L., Zheng, T., and Xu, W. (2006). A thinned lithospheric image of the Tanlu Fault Zone, eastern China: Constructed from wave equation based receiver function migration. J. Geophys. Res. 111, B09312. doi:10.1029/2005jb003974
Claesson, L., Skelton, A., Graham, C., Dietl, C., Mörth, M., Torssander, P., et al. (2004). Hydrogeochemical changes before and after a major earthquake. Geol. 32, 641–644. doi:10.1130/g20542.1
Craig, H. (1961). Isotopic variations in meteoric waters. Science 133, 1702–1703. doi:10.1126/science.133.3465.1702
Duan, R., Li, P., Wang, L., He, X., and Zhang, L. (2022). Hydrochemical characteristics, hydrochemical processes and recharge sources of the geothermal systems in Lanzhou City, northwestern China. Urban Clim. 43, 101152. doi:10.1016/j.uclim.2022.101152
Favara, R., Grassa, F., Inguaggiato, S., and Valenza, M. (2001). Hydrogeochemistry and stable isotopes of thermal springs: Earthquake-related chemical changes along belice fault (western sicily). Appl. Geochem. 16, 1–17. doi:10.1016/S0883-2927(00)00015-9
Gao, X., Liang, H., Wang, H., Zheng, L., Li, J., Zhao, C., et al. (2015). Origin of the mud volcano in northern Tianshan constrained by geochemical investigation. Seismol. Geol. 4, 1215–1224. doi:10.3969/j.issn.0253-4967.2015.04.021
Gu, Q., Ding, Z., Kang, Q., and Li, D. (2020). Group velocity tomography of Rayleigh wave in the middle-southern segment of the Tan-Lu fault zone and adjacent regions using ambient seismic noise. Chin. J. Geophys. Chin. 63, 1505–1522. doi:10.6038/cjg2020N0117
Igarashi, G., and Wakita, H. (1990). Groundwater radon anomalies associated with earthquakes. Tectonophysics 180, 237–254. doi:10.1016/0040-1951(90)90311-U
Jiang, B., Li, Z., and Yu, C. (2022). Application of geochemical temperature scale in jiyuan wulongkou geothermal area. J. Hebei Geo Univ. 45, 83–88. doi:10.13937/j.cnki.hbdzdxxb.2022.01.013
Jin, B., Zhang, Y., and Luan, G. (2000). Geothermal characteristics of warm spring in Jiaodong peninsula. J. Xi’an Eng. Univ. 1, 54–58. doi:10.3969/j.issn.1672-6561.2000.01.012
Lai, G., Jiang, C., Wang, W., Han, L., and Deng, S. (2021). Correlation between the water temperature and water level data at the Lijiang well in Yunnan, China, and its implication for local earthquake prediction. Eur. Phys. J. Spec. Top. 230, 275–285. doi:10.1140/epjst/e2020-000255-3
Li, Y., Zhang, M., Wang, S., Li, Z., and Wang, F. (2011). Progress of the research of stable isotope in precipitation in China:A review. J. Glaciol. Geocryol. 33, 624–633.
Li, B., Shi, Z., Wang, G., and Liu, C. (2019). Earthquake-related hydrochemical changes in thermal springs in the Xianshuihe Fault zone, Western China. J. Hydrology 579, 124175. doi:10.1016/j.jhydrol.2019.124175
Li, Y., Li, P., Cui, X., and He, S. (2021). Groundwater quality, health risk, and major influencing factors in the lower Beiluo River watershed of northwest China. Hum. Ecol. Risk Assess. Int. J. 27, 1987–2013. doi:10.1080/10807039.2021.1940834
Liu, Y., Ren, H., Zhang, L., Fu, H., Sun, X., He, D., et al. (2015). Undergrund fluied anomalies and the precursor mechanisms of the Ludian Ms6.5 earthquake. Seismol. Geol. 1, 307–318. doi:10.3969/j.issn.0253-4967.2015.01.024
Liu, H. (2021). Analysis of hydrogeological conditions in Weihai area of Shandong province. World Non-Ferrous Met. 567, 217–218.
Luan, G., and Li, G. (1993). The geologic geothermal background and spring-controlling structure of Jiaodong warm springs. J. ocean Univ. Qingdao 1, 75–82. doi:10.16441/j.cnki.hdxb.1993.01.006
Ma, Y. (2016). Earthquake-related temperature changes in two neighboring hot springs at Xiangcheng, China. Geofluids 16, 434–439. doi:10.1111/gfl.12161
Ma, Y., Huang, F., Xue, Y., and Wang, B. (2015). Statistical analysis of long-term observation data of water temperature in springs and wells. Technol. Earthq. Disaster Prev. 2, 367–377. doi:10.11899/zzfy20150217
Miyakoshi, A., Taniguchi, M., Ide, K., Kagabu, M., Hosono, T., and Shimada, J. (2020). Identification of changes in subsurface temperature and groundwater flow after the 2016 Kumamoto earthquake using long-term well temperature–depth profiles. J. Hydrology 582, 124530. doi:10.1016/j.jhydrol.2019.124530
Mogi, K., Mochizuki, H., and Kurokawa, Y. (1989). Temperature changes in an artesian spring at Usami in the Izu Peninsula (Japan) and their relation to earthquakes. Tectonophysics 159, 95–108. doi:10.1016/0040-1951(89)90172-8
Pan, S., Wang, F., Zheng, Y., Duan, Y., Liu, L., Deng, X., et al. (2015). Crustal velocity structure beneath Jiaodong Peninsula and its tectonic implications. Chin. J. Geophys. 9, 3251–3263. doi:10.6038/cjg20150920
Pope, E., Bird, D., and Arnórsson, S. (2014). Stable isotopes of hydrothermal minerals as tracers for geothermal fluids in Iceland. Geothermics 49, 99–110. doi:10.1016/j.geothermics.2013.05.005
Qu, J., Gao, S. S., Wang, C., Liu, K. H., Zhou, S., Yang, Y., et al. (2021). Crustal P-wave velocity structure and earthquake distribution in the Jiaodong Peninsula, China. Tectonophysics 814, 228973. doi:10.1016/j.tecto.2021.228973
Sasaki, K., Morita, J., Iwaki, C., and Ueda, A. (2021). Geochemical evaluation of geothermal resources in Toyama Prefecture, Japan, based on the chemical and isotopic characteristics of hot spring waters. Geothermics 93, 102071. doi:10.1016/j.geothermics.2021.102071
Shangguang, Z., Du, J., Zang, W., Wang, J., Kong, L., and Gao, S. (1998). Modern geothermal fluid geochemistry of Tanlu fault zone and Jiaoliao fault block area. Sci. China (series D; earth Sci. 28 (1), 23–29. doi:10.1088/0256-307X/15/11/025
Shi, M., Kang, F., Zhang, J., Gao, S., and Yu, J. (2022). Discussion on the deep heat flow diversion-acculturation between uplift and depression in different tectonic units in the Jiaodong Peninsula. Acta Geol. Sin. 5, 1594–1605. doi:10.19762/j.cnki.dizhixuebao.2020063
Shi, M., Zhang, J., Yin, T., Yang, N., and Jiang, H. (2019). Hydrochemistry characteristic analysis of low-medium temperature convective geothermal resources in Jiaodong Peninsula. Acta Geol. Sin. 1, 138–148. doi:10.19762/j.cnki.dizhixuebao.2019221
Skelton, A., Andrén, M., Kristmannsdóttir, H., Stockmann, G., Mrth, C.-M., Sveinbjrnsdóttir, r., et al. (2014). Changes in groundwater chemistry before two consecutive earthquakes in Iceland. Nat. Geosci. 7, 752–756. doi:10.1038/NGEO2250
Song, D. F., Chen, Y., Wang, T. G., Li, M. J., and Li, P. (2021). Organic geochemical compositions of mesoproterozoic source rocks in the yanliao rift, northern China. Mar. Petroleum Geol. 123, 104740–104820. doi:10.1016/j.marpetgeo.2020.104740
Su, S., Chen, Q., Zou, C., Yan, B., Du, G., and Feng, E. (2021). Hydrogeochemical characteristics and Genesis of seismic observation wells in Shandong Province, China. Arab. J. Geosci. 14, 2398. doi:10.1007/s12517-021-08774-w
Sun, X., and Liu, Y. (2006). Summaries of the micro temperature variations of underground water caused by loading stresses. Recent Dev. world Seismol. 7, 17–26.
Tian, S. (2012). The study on the mechanism of structural geothermal fields of Weihai. China: Jinan university.
Tian, Y. (2015). Features of hydrogen and oxygen isotopes and the make-up source for geothermal water in east Shandong geothermal area. Geol. Sci. Technol. Inf. 34, 182–185.
Tiwari, S., Gupta, A., and Asthana, A. (2020). Evaluating CO2 flux and recharge source in geothermal springs, garhwal himalaya, India: Stable isotope systematics and geochemical proxies. Environ. Sci. Pollut. Res. 27, 14818–14835. doi:10.1007/s11356-020-07922-1
Verma, A., Tiwari, S., Kumar, A., Sain, K., Rai, S., and Kumari, S. (2021). Assessment of water recharge source of geothermal systems in Garhwal Himalaya (India). Arab. J. Geosci. 14, 2341. doi:10.1007/s12517-021-08679-8
Wang, Z., Deng, Q., Chao, H., Du, X., Shi, R., Sun, Z., et al. (2006). Shallow depth sonic reflection profiling studies on the active Penglai Weihai fault zone offshore of the northern Shandong peninsula. Chin. J. Of Geophys. 4, 1092–1101. doi:10.1002/cjg2.920
Wang, Y., Ma, X. X., and Shan, W. (2011). Study on geological characteristics and origin mechanism of Hongshuilantang hot spring in Weihai city. Land Resour. Shandong Prov. 2, 16–19+24.
Wessel, P., Smith, W., Scharroo, R., Luis, J., and Wobbe, F. (2013). Generic mapping tools: Improved version released. Eos Trans. AGU. 45, 409–410. doi:10.1002/2013eo450001
Yu, J., and Che, Y. (1997). Preliminary study on hydrodynamic mechanism of microbehavior of water temperature in well. Earthquake 4, 389–396.
Zhang, L., Guo, L., Zhou, X., Yang, Y., Shi, D., and Liu, Y. (2021). Temporal variations in stable isotopes and synchronous earthquake-related changes in hot springs. J. Hydrology 599, 126316. doi:10.1016/j.jhydrol.2021.126316
Zhou, B., Ran, Y., Huan, W., and Ran, H. (2002). Late Pleistocene surface faulting and the maximum potential earthquake on the Dongshilangou segment of the Haiyang fault, Shandong province. Seismol. Geol. 2, 159–166. doi:10.3969/j.issn.0253-4967.2002.02
Zhu, C., Gan, W., Li, J., Jia, Y., Wang, Q., and Yin, H. (2018). Relative motion between the two blocks on either side of the Yishu fault zone after the 2011 Japan MW9.0 earthquake and its effect on seismic activity. Chin. J. Geophys. (in Chinese) 61, 988–999. doi:10.6038/cjg2018K0687
Keywords: Hongshuilantang Hot spring, anomaly analysis, Rushan earthquake swarm, genesis, hydrogeochemistry
Citation: Du G, Su S, Chang X, Ren H, Huo Z and Zhang X (2023) Hydrogeochemical characteristics and genesis of Hongshuilantang Hot Spring and its water temperature anomalies during the Rushan earthquake swarm in Eastern China. Front. Earth Sci. 10:1088240. doi: 10.3389/feart.2022.1088240
Received: 03 November 2022; Accepted: 14 November 2022;
Published: 17 January 2023.
Edited by:
Qingqiang Meng, SINOPEC Petroleum Exploration and Production Research Institute, ChinaReviewed by:
Rijun Hu, Ocean University of China, ChinaMaosheng Gao, Qingdao Institute of Marine Geology (QIMG), China
Copyright © 2023 Du, Su, Chang, Ren, Huo and Zhang. This is an open-access article distributed under the terms of the Creative Commons Attribution License (CC BY). The use, distribution or reproduction in other forums is permitted, provided the original author(s) and the copyright owner(s) are credited and that the original publication in this journal is cited, in accordance with accepted academic practice. No use, distribution or reproduction is permitted which does not comply with these terms.
*Correspondence: Shujuan Su, shujuan_su@163.com