- 1School of Geological Engineering and Geomatics, Chang’an University, Xi’an, China
- 2Key Laboratory of Western China Mineral Resources and Geological Engineering, Ministry of Education, Xi’an, China
- 3Shanxi Railway Engineering Investigation Co, Ltd, Xi’an, China
- 4School of Civil and Architecture Engineering, Xi’an Technological University, Xi’an, China
- 5College of Civil Engineering, Xijing University, Xi’an, China
Fractures are widespread phenomena on loess slopes in northwestern China. Fractures are of various types and have different distribution patterns, and they are important factors affecting the mechanical properties of loess. In this paper, the effect of different fracture distribution modes (fracture angle, fracture position and fracture combination) on the shear strength of loess is investigated by carrying out consolidated undrained triaxial shear tests. The results show that the existence of fractures in loess can significantly weaken the strength of the soil under consolidated undrained conditions. Compared with unfractured loess, fractures weaken the shear strength of loess mainly by weakening the cohesion. The internal friction angles of fractured loess, however, are hardly affected by the existence of fractures and the fracture distribution mode. Furthermore, a comparison of three fracture distribution modes, namely, the fracture angle, position and combination, reveals that the fracture angle is the most significant factor weakening the loess strength, followed by fracture combination, and the least is fracture position. In addition, confining pressure increments can greatly improve the shear strength of both unfractured and fractured loess, and confining pressure increments can inhibit the weakening effect of fractures on loess strength.
1 Introduction
Fractures are discontinuities. Due to the influence of the sedimentary environment, loess contains fractures at different scales, with different geneses and properties and that formed in different periods, making loess a special geological material with defects such as microcracks, pores and joints (Peng et al., 2019; Wang et al., 2020). Loess with fractures is known as fractured loess (Huang, 1983; Wang, 2000; Sun et al., 2016). Fracturing affects the physical and mechanical properties and engineering geological properties of loess; fracturing is the root cause of the poor macroporosity, water sensitivity and mechanical properties of loess (Zhao, 1994). The internal structure and deformation process of fractured loess are more complicated than those of unfractured loess, making fractured loess more likely to lead to geological disasters in loess areas (Liu et al., 2016; Ma et al., 2019; Wang et al., 2019; Leng et al., 2021). Gully Land Consolidation, Mountain Excavation and City Construction and Gully Consolidation and Table land protection were carried out in the Loess Plateau region. These major projects changed the stress state of each part of the slope of the Loess Plateau to different degrees, destroyed the original stability of the slope, and thus formed fractures with uneven distributions and different occurrences on the slope (Jiang, 2019; Huo et al., 2020; Peng et al., 2020). These fractures not only aggravate the existing geological hazards due to loess but also may lead to new geological problems (Li et al., 2014; Duan et al., 2019; Juang et al., 2019). Therefore, it is very important to study the influence of fractures on the mechanical properties of loess, which can provide a theoretical basis for the prevention of loess disasters.
In the 1930s, when scholars analyzed the causes of major accidents, such as engineering accidents and geological disasters, they found that fractures had a significant influence on the mechanical properties of soil. However, it was not until the 1960s that fractures truly attracted attention (Kong, 1994). (Fookes, 1965; Lo, 1970; Mcgown and Radwan, 1975) studied the morphology, distribution and genesis of fractures and the deformation, strength and stability of fractured soil. Since then, scholars have used different methods (field tests, experiments and numerical simulations) to study the weakening effect of soil strength under different conditions at different scales (macro, meso, and micro) (Potts et al., 1990). They found that fractures have a controlling effect on the strength and deformation of soil, and different fracture shapes and modes have different effects on the strength and deformation of soil.
Loess has special mechanical properties, such as water sensitivity, collapsibility, and structure, which lead to differences in the influence of fractures on the mechanical properties of loess and clay. Therefore, scholars have begun to study the influence of fractures on the deformation and strength of loess. For example (Zhao and Chen, 1981), used the torque ratio method to calculate the safety factor of slopes with fractures and without fractures and found that the safety factor of slopes with fractures decreased by 15% (Sun et al., 2016; Cheng et al., 2017; Fan et al., 2018; Gao, 2020). studied the strength of loess at different angles through unconfined compression strength tests, direct shear tests and triaxial shear tests and found that the angle had a significant influence on soil strength; that is, the closer the fractured angle was to the shear fracture angle of loess at 45°+φ/2, the greater the weakening effect of the fracture was. In addition to studying the weakening effect of the fracture angle (Wang et al., 2013; Gao, 2020), also found that the size and toughness of fracture have significant influence on loess strength. The smaller the fracture size and the rougher the surface morphology of fracture, the smaller the weakening effect of the fracture on the strength of loess (Lu et al., 2006b). conducted direct shear tests on large fractured loess with different formation periods and loading modes, and the experimental results showed that the earlier the fracture formed, the smaller the fracture weakening effect was. Moreover, the fracture effect of the multistage loading mode was smaller than that of continual loading (Wang et al., 2013; Luo et al., 2014; Cheng et al., 2019; Cheng et al., 2021). investigated the influence of the water content on fractured loess, and the results showed that the relationship between the internal friction angle and moisture content was parabolic and that the internal friction angle was extreme when the moisture content reached a certain value (Cheng et al., 2019). referred to the deterioration factor to describe the relationship between the fracture density and shear strength parameters. The deterioration factor of the internal friction angle had a linear relationship with the fracture density, while the deterioration factor of cohesion had a quadratic parabolic relationship. On the basis of experimental data (Yan et al., 2013; Fan et al., 2018), used the Duncan-Chang hyperbolic model and the modified Cam-clay model to describe the stress-strain relations of basic and fractured units of loess (Fan et al., 2018; Wang et al., 2002; Yu et al., 2020). proposed a double-parameter binary-medium model for fissured loess based on the breakage mechanics.
In conclusion, current research focuses on the weakening of soil strength by a single fracture (single angle and single position). However, in practice, the fractures are randomly distribution in loess, the position of the fracture in soil is random and cannot be completely in the center of the soil, and the fracture angle is not a single angle (Zhao et al., 2014). found that the fluctuation and scale of fractures had different influences on the strength of the soil. The mechanical properties of fractured loess in different distribution modes can more truly reflect the weakening effect of fractures on the strength of slopes. Therefore, it is necessary to study the influence of different distribution patterns of fractures on the mechanical properties of loess. Based on this, unfractured loess and fractured loess were investigated by performing consolidated undrained triaxial shear tests to study the shear properties in the shear process in this paper, analyze the influence of fracture modes (fracture angle, fracture position and composite fracture) on the mechanical characteristics of loess, and explore the weakening mechanism of loess strength on the fracture distribution pattern.
2 Study site
The Ansai District in Yan’an city, Shaanxi Province, China, is a typical loess gully landform with interlacing gullies, a long and narrow watercourse, and uneven terrain. Ninety-five percent of the area is mountainous, and only 24% of the area is arable. To solve the problems of serious soil wastage and the low economic value of development in this area, the Gully Stabilization and Land Reclamation project was carried out in Nangou in the Ansai District, with geographic coordinates of 36°34′39″N, 109°18′38″E (Figure 1A). The slope on both sides of the gully was excavated, and the excavated soil was filled in the gully to form a warping dam for later agricultural cultivation. However, a large number of issues, such as sheet erosion, rill erosion, local slip and slope foot collapse, occurred in the course of the Gully Stabilization and Land Reclamation project (Jiang, 2019). These issues are closely related to the rapid development of fractures (Skempton et al., 1969; Potts et al., 1990; George, 2014).
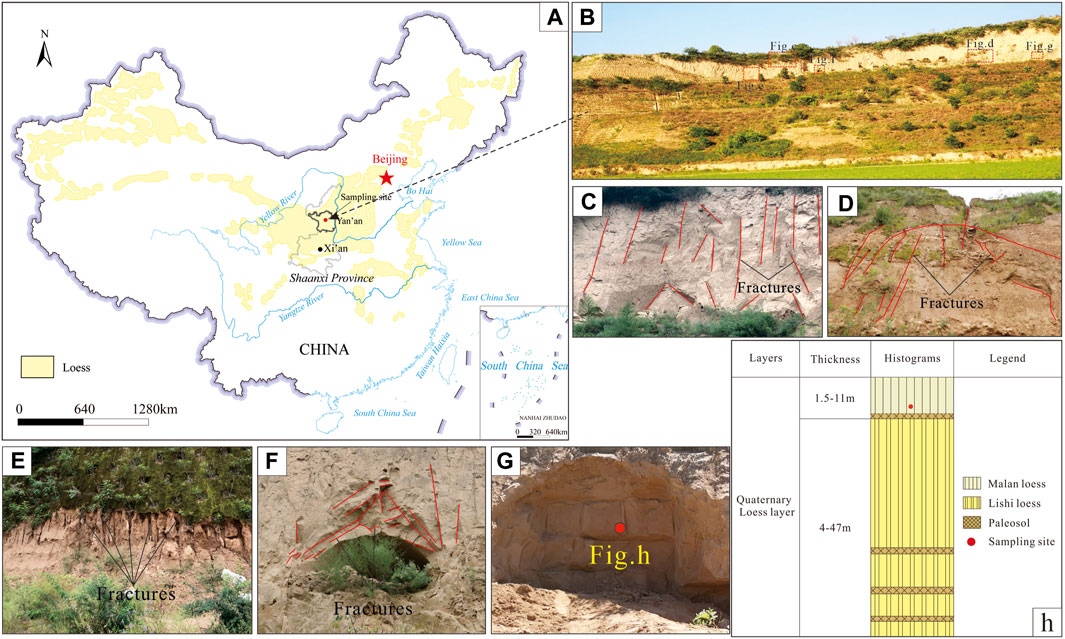
FIGURE 1. Location map:(A) distribution of loess in China and location of the study area in Yan’an city, China; (B) wide view of study side slope;(C–F) are close-up view of side slop fracture generated by different factors: (C) biological effect; (D) rainfall;(E) tectonic joint; (F) human activity; (G) a photograph of loess samples at the sampling site; (H) soil profile at the sampling site.
The investigation of the excavated slope in Nangou in the Ansai District revealed that the factors affecting the development of mesoscopic fractures are mainly as follows: (1) tectonic joints, which are ubiquitous in the loess layer. Tectonic joints have obvious and systematic occurrence features, strong penetration, and synchronization dislocation. In addition, the tectonic joints in groups extend far in a fixed direction, and the strike of each group is not affected by the topography (Figure 1C). (2) For the rainfall effect, the slope generates many erosion rills under rain erosion. As erosion intensifies, erosion rills continue to expand and even connect, forming fractures of different sizes (Figure 1D). (3) For biological effects, the penetration of plant roots and animal activities destroys the original integrity of the soil structure, resulting in fractures on slopes (Figure 1E). (4) For human activities, loess is generally in a state of lateral and vertical compression. When the stress state of loess changes (slope excavation, cave dwellings and other actions reduce the lateral or vertical forces), the stress is released immediately, and then the loess deforms, loosens or moves in the direction of the decrease in the force, thus forming a fracture (Figure 1F).
However, the Gully Stabilization and Land Reclamation project disrupted the original balance of the slope, and the rationality of the slope design was limited (slope angle design, slope protection and drainage conditions), which strengthened the influence of various factors and the coupling effect among them; that is, the fractures accelerated the expansion and connection, resulting in slip, collapse and other slope disasters.
3 Materials and methods
3.1 Test preparation
The loess samples used in the present experimental test were obtained from the foot of the investigated slope (Figure 1G). The loess in the investigation area is subdivided from top to bottom into two stratigraphical units: Malan loess (approximately 10 m thick) and Lishi loess (more than 45 m thick) (Figure 1H). In this study, loess samples were collected at a depth of 9 m below the surface and correspond to Malan loess. The soil samples were yellowish brown with a homogeneous soil fabric and a small number of plant roots and snail shells. The physical properties of undisturbed loess were determined according to the test methods of soil (GB/T50123-2019), and their values are listed in Table 1.
The fractured distribution pattern was identified as an important factor influencing the shear strength of soil (Kong, 1994; Sun et al., 2016; Zhao et al., 2022). According to the distribution patterns of fracture in study area in Figure 1, it can be found that fractured angle is not the only one, mainly between 50° and 70°. In addition, fractures are located in different position of the slope, and there is combination between the fracture. Therefore, this paper selects three representative distribution patterns for studying the shear strength of fractured loess as follows (Figure 2): (a) fracture angle, for which three fractures with different angles (50°, 60°, and 70°) through the center of the soil sample centers were prepared (Figure 2A); (b) fracture location, for which the fracture was located in the upper, middle and lower parts of the loess samples (Figure 2B); and (c) fracture combination, for which cross-composite fractures with different angles were prepared in the soil samples (Figure 2C). The samples with fractured combination were labeled as m+n, where m and n stand for the angles contained in the sample. For example, 50°+60° stands for the fractured loess with two angles of 50° and 60°.

FIGURE 2. Fracture distribution patterns: (A) Different fractured angle; (B) Different fractured position; (C) Different composite fracture.
The preparation process of the fractured loess sample was as follows. First, the soil samples collected from the site were crushed and placed into a 110°C constant temperature oven to dry the soil sample completely (approximately 24 h), and the dried soil sample was processed through a sieve with a diameter of 2 mm. Then, the sieved soil sample was tiled on the non-absorbable tray, and the appropriate amount of added water was sprayed with a sprayer. The soil sample was placed in a closed constant temperature and humidity environment for more than 48 h to ensure the uniform distribution of water in the soil. Subsequently, according to fracture pattern of the sample, the corresponding mass of wet soil is weighted and poured into the mold, and indenter with the corresponding shape is pressed to the corresponding position at a constant speed by jack. When the sample preparation is complete, the sample is gently pushed out of the mold by jack. Finally, the prepared sample was placed in a moisturizing dish for at least 48 h ensure the uniform distribution of water in the sample. Lubricant was smeared on the mold in the process of preparing the sample to prevent damage to the soil sample in the process of demolding.
3.2 Test apparatus
The triaxial shear test was carried out on fractured loess using a Geotechnical Digital Systems (GDS) high precision triaxial instrument. The instrument can simulate complex dynamic and static stress paths and can be used for conventional tensile and compression triaxial tests. The instrument is composed of a pressure chamber, axial loading system (0–2 kN±.0001 kN), pressure loading system (confining pressure (0–3 MPa±.001 kPa) and back pressure (0–1 MPa±.001 kPa)), test console system, data acquisition system and other systems. The confining pressure of the instrument can be pressurized by oil pressure or air pressure. The pore water flow can be obtained by a backpressure controller (0-200 CC ±.001 CC). The test was controlled, and data were collected by Global Digital System Laboratory (GDSLAB) software.
3.3 Test procedure
Cylindrical samples with heights of 76 mm and diameters of 38 mm were prepared for testing. The aim of this study was to investigate the effects of the fracture angle, fracture location and fracture combination on the shear properties of loess. A total of nine groups of tests were carried out. Consolidated undrained triaxial shear tests were carried out for each group of samples under confining pressures of 200 kPa, 300 kPa and 400 kPa. The samples were consolidated under constant confining pressure for approximately 24 h until more than 95% of the excess pore pressure dissipated and entered the shear stage (Lian et al., 2019). The shear rate of the sample was .06 mm/min, and the test was completed when the axial strain reached 20%. During the test, the indoor temperature was controlled to within (20 ± 1)°C, and the data were recorded at a frequency of 10 s for each iteration.
4 Results
4.1 Effect of fractures on the strength of loess
To investigate the effect of fractures on the shear stress of loess, consolidated undrained triaxial shear tests were carried out on unfractured loess and fractured loess with fracture angles of 50° at different confining pressures of 200 kPa, 300 kPa, and 400 kPa. The relationship between deviator stress and strain is shown in Figure 3.
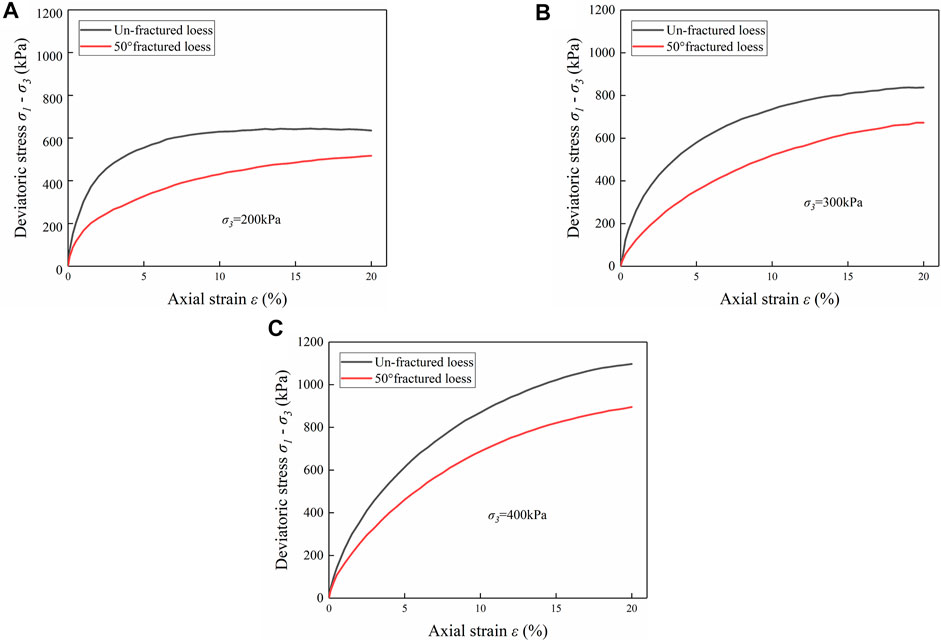
FIGURE 3. Deviator stress versus axial strain of without fractured loess and 50° fractured loess: (A) σ3=200 kPa; (B) σ3=300 kPa; (C) σ3=400 kPa σ3 confining pressure.
Figure 3 shows that the stress-strain relationship of the loess sample is that of strain hardening regardless of whether it has fractures. The stress-strain curve can be divided into two phases: (1) the elastic deformation stage. In this stage, the stress of the loess increases linearly with the strain. In the early stage of elastic deformation, the stress of fractured loess is basically the same as that of un-fractured loess. With increasing strain, the stress of un-fractured loess is gradually greater than that of fractured loess, and the difference increases gradually, which also indicates that the existence of fractures weakens the strength of loess. (2) The elastic-plastic deformation stage, in which the soil stress increases slowly and even tends to be stable with increasing strain. The stress difference between un-fractured loess and fractured loess gradually tends to be stable. In addition, there is an obvious inflection point between the two phases of stress-strain curve, the inflection point of the un-fractured loess is later than that of the fractured loess according to Figure 3, and the hardening degree of the curve is stronger than that of the fractured loess.
A contrastive analysis of stress-strain curves under different confining pressures (Figures 3A–C) reveals that the stress-strain curves of un-fractured loess are similar to those of fractured loess, both of which are strain-hardening types. As the confining pressure increases, the hardening degree of the curves gradually increases, and the hardening degree of un-fractured loess is more obvious. In the initial shearing stage, the difference in the stress between un-fractured and fractured loess is small, and the effect of the confining pressure on the stress of both loess types is basically the same. With increasing deformation, the stress difference decreases with increasing confining pressure under the same strain, which indicates that increasing confining pressure weakens the deterioration effect of fractures on loess strength. In addition, the larger the confining pressure is, the larger the strain is when loess enters the elastic-plastic deformation stage.
4.2 Effect of the fracture distribution mode on the strength of loess
According to the above research, the existence of fractures weakens the strength of loess, and the degree of weakening varies with the distribution mode. To investigate the effect of the fracture on the strength of loess, consolidated undrained triaxial shear tests were carried out on loess with different fractured distribution modes at confining pressures of 200 kPa, 300 kPa, and 400 kPa. Based on the test results, the influence of the fractured distribution modes on the shear characteristics of loess were analyzed and are described in the following section.
4.2.1 Effect of the fracture angle on the strength of loess
To examine the influence of the fracture angle on the strength of loess, experimental results of fractured loess with fracture angles of 50°, 60°, and 70° under confining pressures of 200 kPa, 300 kPa, and 400 kPa were selected as examples, as shown in Figure 4.
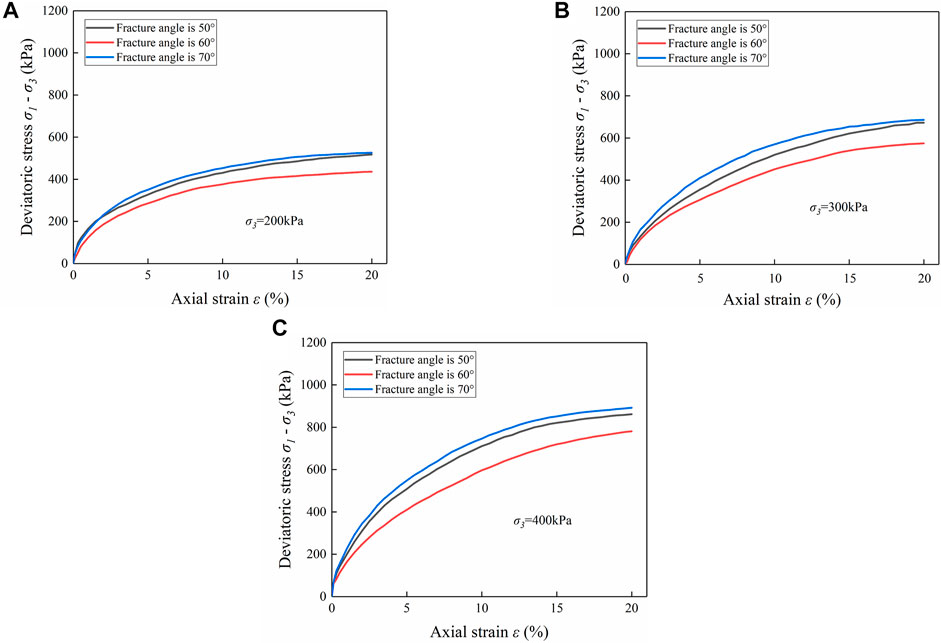
FIGURE 4. Deviator stress versus axial strain of loess with different fractured angles: (A) σ3=200kPa; (B) σ3=300kPa; (C) σ3=400 kPa.
Figure 4 show that the stress-strain curves at different fracture angles are strain-hardening types. However, the inflection point and hardening degree of stress-strain curves at different angles are different. Under the same confining pressure, the hardening degree is at a minimum when the fracture angle is 60°, and the strain required to achieve the same deviator stress is larger. For example, when the confining pressure is 200 kPa and the deviator stress reaches 400 kPa, the strain required with a fracture angle of 60° is about 4.5%, while the strains required with fracture angles of 50° and 70° are only 3.1% and 2.8%, respectively. In addition, with the increase of confining pressure, the later the inflection point of stress-strain curve, the stronger the hardening degree of the curve.
According to the Standard for Geotechnical Testing Method (GB/T50123-2019), the shear strength for fractured loess or unfractured loess was at 15% axial strain in this study. Figures 3, 4 show that when the confining pressure is 200 kPa, the shear strength of the unfractured loess is 641.71 kPa. Compared with unfractured loess, the shear strength of loess decreases 24.37%, 35.24%, and 21.01% when the fracture angle is 50°, 60° and 70°, respectively. This indicates that the fracture has the largest weakening effect on the soil strength when the fracture angle is 60°.
In addition, the loess sample strength increases with increasing confining pressure. The shear strength of un-fractured loess increases by 37.17% when the confining pressure increases from 200 kPa to 400 kPa, when the fracture angles are 50°, 60°, and 70°, the shear strength of fractured loess increases by 40.87%, 42.26%, and 40.44%. In short, the shear strength of fractured loess increases more than that of unfractured loess, and the shear strength increases the most when the fracture angle is 60°.
4.2.2 Effect of the fracture position on the strength of loess
To explore the effect of the fracture position on the strength of loess, experimental results of fractured loess with upper, middle and lower fracture positions under confining pressures of 200 kPa, 300 kPa, and 400 kPa are taken as examples, as shown in Figure 5.
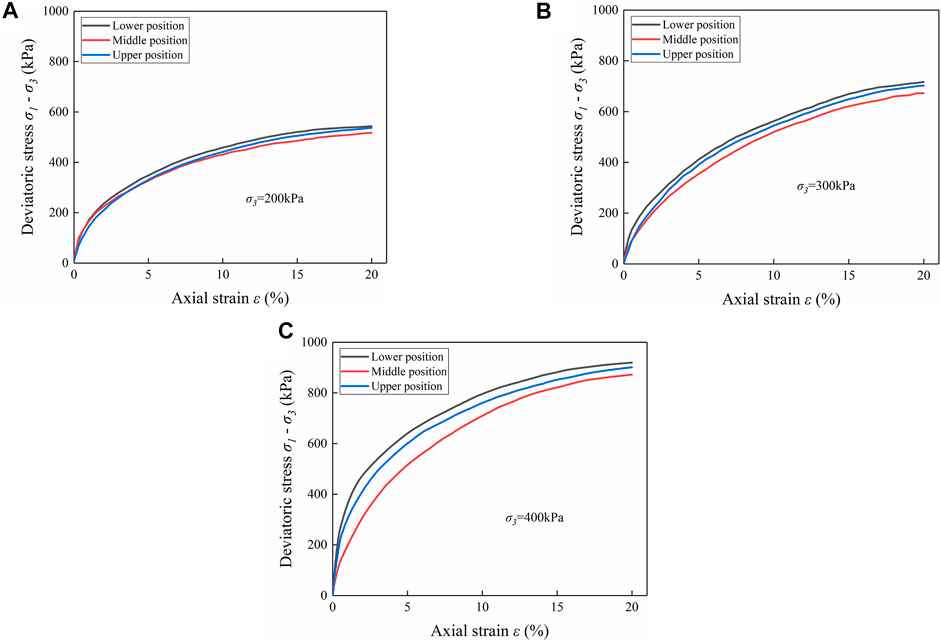
FIGURE 5. Deviator stress versus axial strain of loess with different fractured positions:(A) σ3=200 kPa; (B) σ3=300 kPa; (C) σ3=400 kPa.
As shown in Figure 5, the fracture position has a certain amount of effect on the stress of loess. The stress-strain curves of loess with different fracture positions are of the strain-hardening types, and the hardening degree of the curve is the least when the fracture is located in the middle position of the sample, while when it is located in the upper position of the sample, the hardening degree is slightly less than that of the lower part.
In addition, the inhibiting effect of confining pressure on the weakening effect of the fracture is different at different fracture locations. When the confining pressure is 200 kPa, the shear strength of loess with lower, middle and upper fracture positions are 520.69 kPa, 485.32 kPa, and 505.64 kPa, respectively. With increasing confining pressure to 400kPa, the shear strength of loess increases by 41.22%, 40.87%, and 40.86%, respectively, showing that the confining pressure has the most obvious weakening effect on loess with the middle fracture position, followed by the upper and lower positions.
4.2.3 Effect of the fracture combination on the strength of loess
To study the influence of the fracture combination on the strength of loess, experimental results of fractured loess with fracture combination angles of 50°+60°, 60°+70°, and 50°+70° under confining pressures of 200 kPa, 300 kPa, and 400 kPa are chosen as examples, as presented in Figure 6.
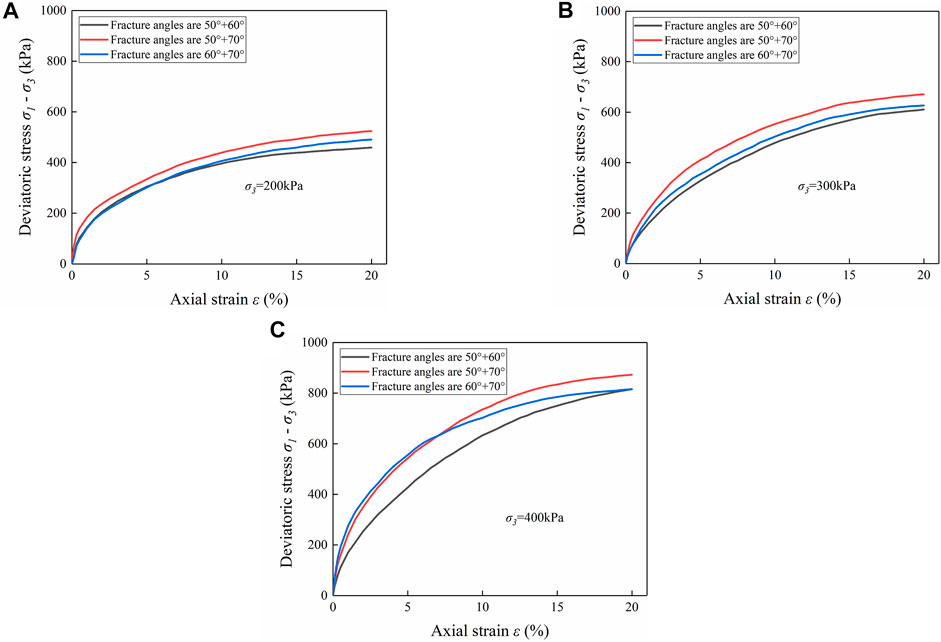
FIGURE 6. Deviator stress versus axial strain of loess with different composite fracture: (A) σ3=200 kPa; (B) σ3=300 kPa; (C) σ3=400 kPa.
Figure 6 shows that the stress-strain curves of fractured loess with different fracture combinations are strain-hardening types. Loess with a fracture combination angle of 50°+70° has the highest degree of hardening, followed by fractured loess with a combined angle of 60°+70°, and loess with a combined angle of 50°+60° has the lowest degree of hardening.
As shown in Figure 6, the shear strength fracture loess with fracture combination angles of 50° + 60° decreases by 31.66% at the confining pressure of 200 kPa. Comparatively, the shear strength decreases by 26.54% with confining pressure of 400 kPa. However, for confining pressure is 200 kPa, the shear strength of loess with fracture combination angles of 60°+70°, 50°+70° decreases by 23.27%, 28.42%, respectively, and the shear strength decrease by 18.33%, 23.18% at the confining pressure of 400 kPa.
4.3 Shear strength of fractured loess
The distribution mode of fractures plays an important role in the development of the shear strength parameters of fractured loess. The shear strength parameters of fractured loess, including cohesion (c) and the internal friction angle (φ), were obtained from the triaxial tests based on the Mohr-Coulomb failure criterion (Li, 2014), as shown in Figure 7.
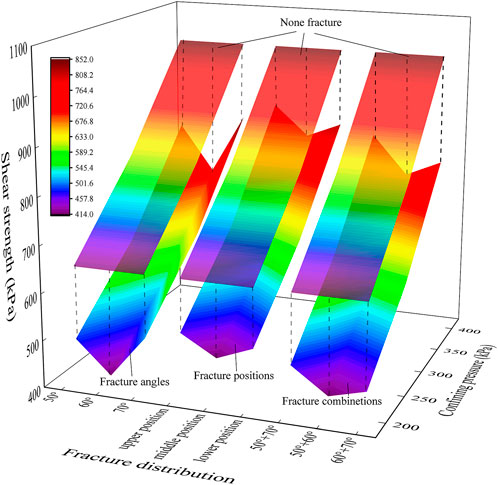
FIGURE 7. Shear strength envelope surface in the three-dimensional space of fracture distribution-confining pressure-shear strength.
Figure 7 shows that both the cohesion and internal friction angle of fractured loess decrease compared with those of the un-fractured loess. However, the amount of decrease of cohesion is greater as comparing with that of the internal friction angle. For different fractured angle (50°, 60°, 70°) loess, the cohesion is decreased by 37.55%–57.44%, whereas internal fraction angle is decreased by 5.44%–12.11%. However, the cohesion and internal friction of loess is non-linear change with fractured angle. As the fracture angle increases from 50° to 60°, the cohesion increases by 13.8%, whereas the internal friction angle increases by only 5.3%. Interestingly, As the fracture angle increases from 60° to 70°, the cohesion decreases by 19.89%, and the internal friction angle decreases by 6.67%.
In addition, when fractured angle is 50° at different positions of the sample, the cohesion is decreased by 39.24%–43.64%, while the internal friction angle is decreased by only 2.12%–6.81%. When the fracture is located in the middle of the sample, the cohesion and internal friction angle decrease the most. For different fracture combinations, the cohesion is decreased by 42.91%–51.10%, whereas the internal friction angle is decreased by only 5.79%–10.80%. When the combined fracture angle is 50°+60°, the cohesion and internal friction angle decrease the most.
5 Discussion
The effect of fractures on the shear strength of soils (such as clay, loess, expansive soil, etc.) has attracted the attention of a number of researchers in the past several decades (Na et al., 2022; Sun et al., 2015; Vitone and Cotecchia, 2011; Xu et al., 2022; Zhao et al., 2014), and they have found that fractures can significantly weaken the strength of soils. In this paper, the same conclusion is obtained by triaxial shear tests of loess, but the weakening degree of different distribution patterns of fractures is different. Previous studies used different test methods (such as plane strain, direct shear and unconfined compression strength tests) for this purpose (Lu et al., 2006b; Wang, 2008; Cheng et al., 2017; Lu et al., 2015). Despite the different test methods, the same conclusion, that fracture distribution patterns play an important role in contributing to the weakening of shear strength in loess, was obtained (Kong, 1994; Lu et al., 2006a; Cheng et al., 2019).
5.1 Fracture effect on the shear strength of loess
Figure 8 is a three-dimensional diagram of the fracture distribution pattern, confining pressure and shear strength of loess. It can be seen that different fracture distribution pattern have different effects on shear strength of fractured loess. It mainly includes the following two points: (1) When confining pressure is same, the concave-convex degree of strength plane (fracture distribution-shear strength plane) is the largest under different fracture angle modes, which indicate that the sample shear strength in this mode has the largest variation range, that is, the fracture angle has the most obvious effect on the sample shear strength. The next is fracture combination, and the least is the fracture position. This result is in agreement with other studies on loess (Kong, 1994; Gao, 2020). (2) Under different distribution pattern of fracture, the strength of loess increases and the deterioration degree of the strength decreases with confining pressure. This indicates that the increase of confining pressure weakens the deterioration effect of the fracture on the loess strength, and the greater the deterioration degree, the more obvious the inhibition effect of confining pressure. This may be because in the consolidation process, the sample volume is compressed under the confining pressure, the pore ratio and moisture content of soil are reduced, the soil particles are close to each other, and the interaction force between particles increases (Li, 2014). Second, the confining pressure can restrain the sliding of soil particles and control the deformation of soil, and the inhibitory effect is stronger with increasing confining pressure. In short, confining pressure can weaken the fracture weakening effect on the loess. In practical engineering, the confining pressure indicates the burial depth of the soil. According to the test results of this paper, the burial depth can weaken the fracture weakening effect.
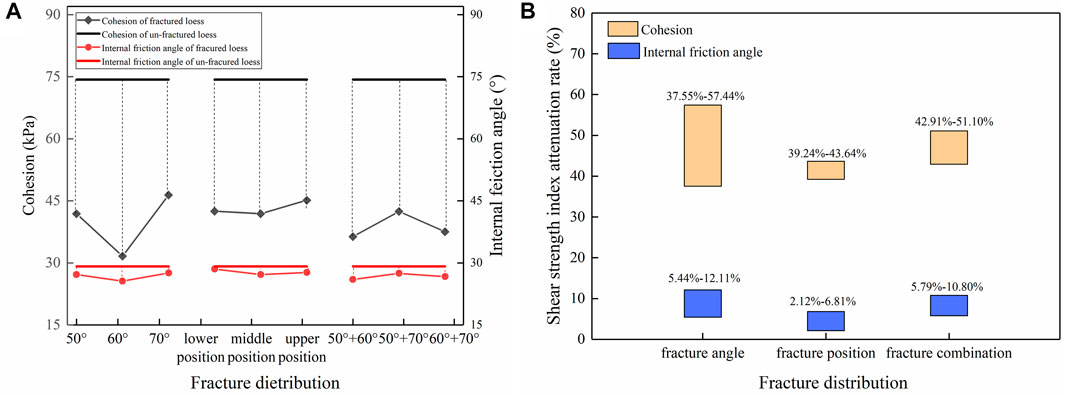
FIGURE 8. Curve of cohesion and internal friction angle of fracture loess with different fracture distribution pattern. (A) shear strength index; (B) shear strength index attenuation rate.
The difference of soil strength development in different fracture distribution patterns lead to the different failure modes (Lu et al., 2006a). For un-fractured loess, the sample occurs drum rupture (Figure 9A). For fracture loess, there are three the failure modes of sample: (1) fracture along original fracture. This failure mode mainly occurs at low confining pressure, and the failure occurs along the original fracture and even slippage (Figure 9B). (2) bending fracture. When the confining pressure is high, the soil is prone to bending failure. In particular, when the fracture is farther away from the sample center, the bending failure is more obvious (Figure 9C). (3) drum rupture. The original fracture of the sample is more closed and is not destroyed along the fracture, which mainly occurs when the fracture is located in the center of the sample and the confining pressure is high (Figure 9D).
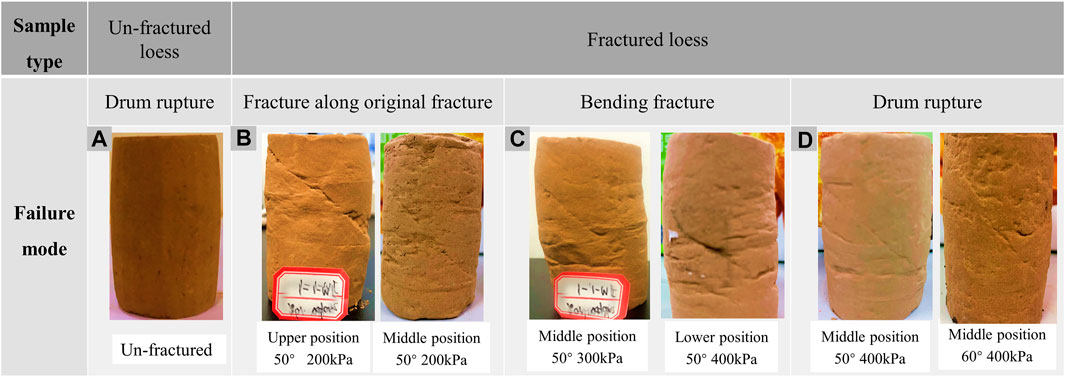
FIGURE 9. The failure modes for the un-fractured loess and fracture loess. Un-fractured loess: (A) drum rupture; fractured loess: (B) fracture along original fracture; (C) bending fracture; (D) drum rupture.
5.2 The effect of fracture on the shear strength parameter of loess
The shear strength weakening of soil is due to the change of cohesion and internal friction angle (Sun et al., 2016). Figure 7B shows the relationship between the attenuation range of sample cohesion and internal friction angle and fracture distribution patterns. It can be found the cohesion of fractured loess changes obviously with the fracture distribution pattern, but change in the internal friction angle is minor. This indicates that the weakening of the loess strength by fractures is mainly the weakening of the cohesion of the loess rather than the internal friction angle, which may be mainly because the cohesion of soil is provided by the cementation between the soil particles, and the existence of fractures destroys the continuity of the soil structure and reduces the overall cementation of the particles (Zhang, 2018; Gao, 2020). Furthermore, the internal friction force is mainly the friction force between particles, which is related to the soil density, particle size, particle shape and mineral composition (Zhao, 1994; Li, 2014; Sun et al., 2016), so the effect of fracture on internal friction angle is less than that on cohesion. In this paper, the influence of fracture angle on shear strength parameter is greater than that of fracture combination and fracture position. Interestingly, when the fracture is located in the upper position and lower position of the sample, there is a small change in the cohesion of the fractured loess and the internal friction angle is almost the same. This also explains that the strength difference of soil when the fracture is located in the upper position and lower position of the sample. The same conclusion is also obtained by (Jiang et al., 2022). Further studies are required (expanding test to other fracture position) to check the possibility of generalization of the present findings.
5.3 Analysis of the weakening mechanism of fractured loess strength
(Zhao, 1994; Fan et al., 2018; Tudisco et al., 2019; Song et al., 2022) found that the fractures weaken the loess strength, and the same conclusion is reached in this paper. In addition, it is found that the fracture angle is one of the important factors affecting soil strength weakening in this paper. This can be explained by Mohr Coulomb theory (Long, 2015) (For fractured loess (Wang, 2008), found that the theoretical failure surface (the theoretical failure surface is the surface with the angle of 45°+φ/2 from the surface of the maximum principal stress) determined by Mohr Coulomb theory was closer to the measured value through comparative of different strength theory methods).
The angle between the fracture plane and the maximum principal stress surface is assumed to be β, according to Mohr’s stress circle theory the normal stress σ and shear stress τ acting on the fracture surface are as follows:
It is assumed that the shear strength τf of the fracture surface obeys the Coulomb-Navier criterion, namely:
φj and cj are friction angle and cohesion of fracture surface respectively. It can be obtained from the above three equations:
The formula can be transformed into:
It can be concluded that when 2β-φj=π/2, that is, β=π/4+φj/2≈60°, the shear strength of fractured loess is the minimum. This is the same as the experimental results in this paper, that is, the more similar the fracture is to the failure surface, the strength of soil is the minimum, the more likely the loess is to be damaged. Angle is an important factor affecting the similarity between failure surface and fracture (Sun et al., 2016; Cheng et al., 2017; Gao, 2020), so the fracture angle has the greatest influence on the strength of loess among the three fracture distribution modes studied in this paper.
For loess with composite fracture, the more similar the composite fracture is to the theoretical failure surface, the more likely the soil will be damaged. In addition, due to the different strength of fracture at different angles, stress concentration is easy to occur at the junction of composite fracture. When the strength difference between the two fractures increases, the stress concentration becomes more obvious and the soil strength decreases more (Cao and Ge, 1990). According to the above theory, it can be concluded that the shear strength of soil should be the same when the same fracture is located at different positions of the sample, but the experimental results show that the shear strength is different when the fracture is located at different positions in the loess sample. Zhou et al. (2015) used numerical analysis software to find that the difference in plastic zone within the sample when the fracture is located at the different positions of the sample is the main reason for the sample strength difference.
The results presented here can be used as a design basis for the weakening effect of fractures in engineering. However, it is important to point out that the relationship observed herein is obtained from studying fractures only with angles of 50°, 60° and 70°, while the shear strength of loess with a wider range of fracture angles has not been explored. Hence, further testing, including large-scale fracture angle shear tests, is necessary to check whether the conclusions drawn from this research are still applicable. Nevertheless, this study provides baseline information for future studies of large-scale fracture angles and engineering practice in the field.
6 Conclusion
The influence of fractures on the shear strength of loess was studied by a consolidated undrained triaxial shear test. Moreover, the effect of the fracture distribution pattern on the loess strength was considered, and the obtained results were discussed. The following conclusions were drawn.
The stress-strain relationship of fractured and unfractured loess is a strain-hardening type. For a given confining pressure, the degree of the stress-strain curve hardening of unfractured loess is greater than that of fractured loess, and the difference between them decreases with increasing confining pressure.
The shear strength of samples is significantly affected by fracture distribution patterns. A comparison of the fracture angle, fracture position and fracture combination reveals that the loess strength is the most sensitive to the change in the fracture angle, and the closer the fracture angle is to the Mohr-Coulomb failure angle, the smaller the strength of loess. The influence of the fracture combination on the loess strength is greater than that of the fracture position.
For loess samples with or without fractures, the shear strength increases profoundly as the confining pressure increases from 200 kPa to 400 kPa. For fractured loess, the greater the weakening effect of fractures on the loess strength is, the stronger the inhibition effect of the confining pressure is.
Compared with the unfractured loess, the decrease in cohesion contributes the most to the decrease in the shear strength of the fractured loess. The value of the internal friction angle has little change with different fracture distribution patterns.
Data availability statement
The original contributions presented in the study are included in the article/supplementary material, further inquiries can be directed to the corresponding author.
Author contributions
Conceptualization, PM; Investigation, LC, PM, and FX; conceived and designed the tests, LC and PM; tests LC and FX; YL, JZ, JN, and RY contributed materials and theoretical foundations; writing—original draft, LC; writing—review and editing, PM; All authors have read and agreed to the published version of the manuscript.
Funding
This work was financially supported by the Program of National Natural Science Foundation of China (41790441, 42107198, 42007257, 41907235); Young Talent Fund of Association for Science and Technology in Shaanxi, China (20220719).
Conflict of interest
Author FX was employed by the Shanxi Railway Engineering Investigation Co., Ltd., China.
The remaining authors declare that the research was conducted in the absence of any commercial or financial relationships that could be construed as a potential conflict of interest.
Publisher’s note
All claims expressed in this article are solely those of the authors and do not necessarily represent those of their affiliated organizations, or those of the publisher, the editors and the reviewers. Any product that may be evaluated in this article, or claim that may be made by its manufacturer, is not guaranteed or endorsed by the publisher.
References
Cao, J. C., and Ge, X. R. (1990). Model test study on shear Shear mechanical characteristic of combined joints. Rock soil Mech. 11 (2), 23–37. (in Chinese).
Cheng, L. H., Nie, R. S., and Liu, F. (2017). An experimental study of the uniaxial compressive strength of fractured loess. Hydrogeol. Eng. Geol. 44 (5), 80–85+91. (Chinese with English abstract).
Cheng, Y. X., Cao, B. B., and Zhang, D. W. (2019). Experimental study on the effect of fractures density on shear strength of loess. Sci. Tech-nology Eng. 19 (28), 284–289. (Chinese with English abstract).
Cheng, Y. X., Huo, A. D., Zhao, Z. X., and Peng, J. (2021). Analysis of loess fracture on slope stability based on centrifugal model tests. Bull. Eng. Geol. Environ. 80 (5), 3647–3657. doi:10.1007/s10064-021-02135-3
Duan, X., Dong, Q., Ye, W. J., Zhou, J. l., and Oh, E. (2019). Study on adverse effects of groundwater level rising induced by land creation engineering in hilly and gully area of the Loess Plateau. J. Mt. Sci. 16 (12), 2739–2753. doi:10.1007/s11629-019-5549-x
Fan, W., Deng, L. S., and Yuan, W. N. (2018). Double parameter binary-medium model of fissured loess. Eng. Geol. 236, 22–28. doi:10.1016/j.enggeo.2017.09.014
Fookes, P. G. (1965). Orientation of fissures in stiff overconsolidated clay of the siwalik system. Geotechnique 15 (2), 195–206. doi:10.1680/geot.1965.15.2.195
Gao, M. M. (2020). Study on the influence of precast joints on the mechanical properties of loess. Xi’an, China: Chang’an University. (Chinese with English abstract).
George, R. O. (2014). Analysis of the stability of embankments on clay Foundations. Int. J. Eng. Technol. Res. 2 (1), 1–11.
Huang, W. (1983). Soil engineering properties. Beijing: China Water Conservancy and Hydropower Press.
Huo, A. D., Peng, J. B., Cheng, Y. X., Luo, P., Zhao, Z., and Zheng, C. (2020). Hydrological analysis of Loess Plateau highland control schemes in dongzhi plateau. Front. Earth Sci. 8, 14. doi:10.3389/feart.2020.528632
Jiang, J. k. (2019). Analysis of stability and slope ratio optimization of excavated slope of gully Land consolidation project in the loess hilly area:— a case study of Nangou in yanan city. Xi’an, China: Chang’an University. (Chinese with English abstract).
Jiang, T., Yin, C. Y., Wang, J. F., and Li, H. J. (2022). Experimental study on the effect of crack location and opening on shear strength of rock mass. J. North China Univ. Water Resour. Electr. Power (Natural Sci. Ed. 43, 85–93.
Juang, C. H., Dijkstra, T., Wasowski, J., and Meng, X. (2019). Loess geohazards research in China: Advances and challenges for mega engineering projects. Eng. Geol. 251, 1–10. doi:10.1016/j.enggeo.2019.01.019
Leng, X. L., Wang, C., Zhang, J., Sheng, Q., Cao, S., and Chen, J. (2021). Deformation development mechanism in a loess slope with seepage fissures subjected to rainfall and traffic load. Front. Earth Sci. 9, 15. doi:10.3389/feart.2021.769257
Li, P. Y., Qian, H., and Wu, J. H. (2014). Environment: Accelerate research on land creation. Nature 510 (7503), 29–31. doi:10.1038/510029a
Lian, B. Q., Peng, J. B., Zhan, H. B., and Wang, X. (2019). Mechanical response of root-reinforced loess with various water contents. Soil Till Res. 193, 85–94. doi:10.1016/j.still.2019.05.025
Liu, Z., Wang, L. X., Yi, X., Mao, Q., and Liu, Y. (2016). The water source situation and crises of the irrigation areas on the Loess Plateau at the origin of the Silk Road, China. Environ. Earth Sci. 75 (15), 1144. doi:10.1007/s12665-016-5955-0
Lo, K. Y. (1970). The operational strength of fissured clays. Geotechnique 20 (1), 57–74. doi:10.1680/geot.1970.20.1.57
Long, F. (2015). Research on the computational method of earth pressure for the excavations in fissured clay in Chengdu area. Chengdu China: Southwest Jiaotong University. (Chinese with English abstract).
Lu, Q. Z., Peng, J. B., and Fan, W., (2006a). Deformation characteristics of fissured loess in triaxial compression tests. Highway 8, 1–4. (Chinese with English abstract).
Lu, Q. Z., Peng, J. B., and Fan, W., (2006b). Direct shear test of large size fractured loess. Highway 5, 184–187. (Chinese with English abstract).
Luo, Y., Wang, T. H., Liu, X. J., and Zhang, H. (2014). Laboratory study on shear strength of loess joint. Arab. J. Sci. Eng. 39 (11), 7549–7554. doi:10.1007/s13369-014-1318-x
Ma, P. H., Peng, J. B., Wang, Q. Y., Zhuang, J., and Zhang, F. (2019). The mechanisms of a loess landslide triggered by diversion-based irrigation: A case study of the south jingyang platform, China. Bull. Eng. Geol. Environ. 78 (7), 4945–4963. doi:10.1007/s10064-019-01467-5
Mcgown, A., and Radwan, A. M. (1975). The presence and influence of fissures in the boulder clays of west central Scotland. Can. Geotech. J. 12 (7), 84–97. doi:10.1139/t75-007
Peng, J. B., Wang, Q. Y., and Men, Y. M. (2019). Landslides in Loess Plateau. Beijing,China: Science Press. (In Chinese).
Peng, J. B., Wang, Q. Y., and Zhuang, J. Q. (2020). Dynamic formation mechanism of landslide disaster on the Loess Plateau. J. Geomechan 26 (5), 714–730.
Potts, D. M., Dounias, G. T., and Vaughan, P. R. (1990). Finite element analysis of progressive failure of Carsington embankment. Geotechnique 40 (1), 79–101. doi:10.1680/geot.1990.40.1.79
Skempton, A. W., Schuster, R. L., and Petley, D. J. (1969). Joints and fissures in the london clay at wraysbury and edgware. Geotechnique 19 (2), 205–217. doi:10.1680/geot.1969.19.2.205
Song, X. Q., Yan, X. S., Duan, Z., Tuo, J., Sun, Q., and Yuan, X. (2022). The effect of high temperature on the fracture damage of loess. Eng. Fract. Mech. 262, 108270. doi:10.1016/j.engfracmech.2022.108270
Sun, P., Peng, J. B., Chen, L. W., Lu, Q., and Igwe, O. (2016). An experimental study of the mechanical characteristics of fractured loess in Western China. B Eng. Geol. Environ. 75 (4), 1639–1647. doi:10.1007/s10064-015-0793-y
Tudisco, E., Vitone, C., Mondello, C., Viggiani, G., Hall, S. A., Cotecchia, F., et al. (2019). Influence of fissure inclination and confining pressure on the local behaviour of natural clays. 7th Int. Symposium Deformation Charact. Geomaterials 92, 03004. doi:10.1051/e3sconf/20199203004
Vitone, C., and Cotecchia, F. (2011). The influence of intense fissuring on the mechanical behaviour of clays. Geotechnique 61 (12), 1003–1018. doi:10.1680/geot.9.p.005
Wang, J. G., Leung, C. F., and Ichikawa, Y. (2020). A simplified homogenisation method for composite soils. Comput. Geotechnics 29 (6), 477–500. doi:10.1016/s0266-352x(02)00004-6
Wang, J. (2000). Theory and application of ground fissures. Xian,Beijing: Shaanxi Science and Technology Press. (in Chinese).
Wang, S. K., Peng, J. B., Zhuang, J. Q., Kang, C., and Jia, Z. (2019). Underlying mechanisms of the geohazards of macro Loess discontinuities on the Chinese Loess Plateau. Eng. Geol. 263, 105357. doi:10.1016/j.enggeo.2019.105357
Wang, T. X., Luo, Y., and Wang, J. J. (2013). Experimental research on shear strength of the loess joints. Chin. J. Undergr. Space Eng. 9 (3), 497–501+551. (Chinese with English abstract).
Wang, Z. X. (2008). Study on the shear of sanyuan fissured loess under plain strain condition. Xi’an, China: Chang’an University. (Chinese with English abstract).
Xu, J., Zhou, L. Y., Li, Y. F., Ding, J., Wang, S., and Cheng, W. C. (2022). Experimental study on uniaxial compression behavior of fissured loess before and after vibration. Int. J. Geomechanics 22 (2), 11. doi:10.1061/(asce)gm.1943-5622.0002259
Yan, F. R., Fan, W., and He, T. Y. (2013). Study on binary-medium model of fissured loess. 2nd Int. Conf. Civ. Eng. Transp. 256-259 (256–259), 240–244. doi:10.4028/www.scientific.net/amm.256-259.240
Yu, D., Liu, E. L., Sun, P., Xiang, B., and Zheng, Q. (2020). Mechanical properties and binary-medium constitutive model for semi-through jointed mudstone samples. Int. J. Rock Mech. Min. Sci. 132, 104376. doi:10.1016/j.ijrmms.2020.104376
Zhang, D. W. (2018). Experimental study on shear strength of fissured loess. Xi’an, China: Chang’an University. (Chinese with English abstract).
Zhao, N., Meng, L. X., Wang, L. G., and Yibin, Z. (2022). Numerical simulation of creep fracture evolution in fractured rock masses. Front. Earth Sci. 10, 13. doi:10.3389/feart.2022.901742
Zhao, X. M., and Chen, Y. L. (1981). Stability analysis of cut slopes in uniform loess. China Civ. Eng. J. 1, 43–49. (Chinese with English abstract).
Zhao, X., Yang, Y. H., and Zhu, Y. J. (2014). Analysis of impact of crack surface on shear strength of strong expansive soil. Rock Soil Mechan 35 (1), 130–133. (Chinese with English abstract).
Zhao, Z. X. (1994). The influence of fissure on shear strength of soil. Subgr. Eng. 5, 11–16. (Chinese with English abstract).
Keywords: loess, fracture distribution, shear strength, fracture effect, fracture deterioration mechanism
Citation: Chen L, Ma P, Zhao J, Xie F, Yan R, Leng Y and Nan J (2023) Effect of fracture distribution on the triaxial shear behavior of loess. Front. Earth Sci. 10:1087286. doi: 10.3389/feart.2022.1087286
Received: 02 November 2022; Accepted: 29 December 2022;
Published: 11 January 2023.
Edited by:
Bin Li, Chinese Academy of Geological Science, ChinaReviewed by:
Meiben Gao, Xihua University, ChinaXiaojun Guo, Institute of Mountain Hazards and Environment (CAS), China
Copyright © 2023 Chen, Ma, Zhao, Xie, Yan, Leng and Nan. This is an open-access article distributed under the terms of the Creative Commons Attribution License (CC BY). The use, distribution or reproduction in other forums is permitted, provided the original author(s) and the copyright owner(s) are credited and that the original publication in this journal is cited, in accordance with accepted academic practice. No use, distribution or reproduction is permitted which does not comply with these terms.
*Correspondence: Penghui Ma, spawnkobe@163.com