- 1School of Resources and Environmental Engineering, Institute for Spatial Information Intelligence Analysis and Application, Hefei University of Technology, Hefei, China
- 2Anhui Province Engineering Laboratory for Mine Ecological Remediation, School of Resources and Environmental Engineering, Anhui University, Hefei, Anhui, China
With rapid economic development and the increasing demand for drinking water, a large amount of groundwater is exploited, resulting in a high F− content in groundwater, which is harmful to the environment and human body. In this study, 5,464 data points of fluoride in shallow groundwater were collected, and the F− content distribution, occurrence form and environmental impact of shallow groundwater were discussed. The results showed that 1) the F− content in shallow groundwater in China ranged from 0 to 60 mg/L, with a mean content of 0.90 mg/L; the lowest average F− content in shallow groundwater in Southwest China was 0.36 mg/L; South China (1.20 mg/L), Northeast China (1.25 mg/L) and Northwest China (1.25 mg/L) were considered high-fluoride areas, and North China (0.93 mg/L), East China (0.67 mg/L) and Central China (0.80 mg/L) were considered low-fluoride areas. The mean F− content in groundwater differed between provinces and cities. 2) The F− in shallow groundwater mainly occurred in ionic, complex ionic and organic fluoride molecular states. 3) The influence of a high F− content in shallow groundwater on the environment was mainly manifested in the increase in water F− concentration and soil F− and vegetable F− content. The influence of a high F− content on the human body was mainly manifested in an increase in urinary F− content in children, a high prevalence of dental fluorosis in children, an increase in skeletal fluorosis rate in adults with age, and an influence on cognitive function of older adults. These results provide a basis for F− pollution control and high-fluoride water treatment.
Introduction
F− is one of the most essential trace elements for human growth, and an appropriate amount of F− is conducive to preventing dental caries and promoting bone growth (Sarinana-Ruiz, et al., 2017; Gao et al., 2020; Li et al., 2020). Both insufficient and excessive F− cause great harm to human health. Specifically, a lack of F− tends to cause dental caries, and excessive intake leads to fluorosis (Katsanou et al., 2013; Tarki et al., 2020; Nafouanti et al., 2021; Senarathne et al., 2021). The common diseases caused by excessive F− intake are dental fluorosis and skeletal fluorosis, which may lead to death in severe cases (Xie et al., 1999; Mondal et al., 2016; Mohammadi et al., 2017; Liu et al., 2021). F− can cause biochemical effects, such as acute poisoning (vomiting, hemoptysis, hand and leg spasm, cardiac arrest, etc.), long-term chronic poisoning (gene mutation, allergic diseases, Alzheimer’s disease, etc.), and carcinogenic and mutagenic effects, mainly due to the effect of fluorination (Liu et al., 2015a; Patil et al., 2018; Kurdi 2016; Nikiforova 1982; Maitra et al., 2021; Morales-Arredondo et al., 2016). All F− is toxic. Acute fluorosis results when the daily intake of F− is higher than 4 mg, and its toxicity is higher than that of lead and lower than that of arsenic. Long-term F− accumulates in human teeth and bones under a high-fluoride environment, which can damage human soft tissue and intellectual development and even lead to an increased risk of tumors and leukemia (Mumtaz et al., 2015; Durrani and Farooqi, 2021; Senthilkumar et al., 2021). According to the latest reports, long-term, excessive intake of F− has also been linked to adverse cancer and distortion (Smith et al., 1979; Seraj et al., 2012; Nikiforova 1982; Su et al., 2021). Moreover, previous studies found that plants in environments with high F− concentrations may have impacted growth, morphological, photosynthetic and metabolic characteristics (Reddy and Kaur, 2008; Bhargava and Bhardwaj, 2010; Bustingorri et al., 2016; Meng and Wu 1996; Gao et al., 1998; Elloumi et al., 2015; Zhong et al., 2014; Adeyeye et al., 2021).
F− is soluble in water and readily absorbed by the body. The World Health Organization allows the F− concentration in water bodies to be 1.50 mg/L, and the allowable F− concentration in drinking water in China is 1.00 mg/L. If the F− concentration in a water body exceeds 1.00 mg/L, it is classified as high-fluoride water. In 2017, 13.333 million people suffered from dental fluorosis and 1.086 million from skeletal fluorosis in China. It has been reported that F− can be distributed and accumulate in tissues and organs with blood circulation. When internal F− reaches a certain concentration, it can seriously affect fetal brain development and osteogenic activity. Long-term F− intake seriously inhibits the activity of protease and plasma myeloperoxidase in human cells, the expression of the phosphatidylinositol-3-kinase threonine protein kinase (PI3K-Akt1) gene and protein synthesis and affects the metabolism of calcium and phosphorus (Xie et al., 1999; Yao et al., 2005; Wang et al., 2011; Fan et al., 2015; Xu et al., 2016; Hossain et al., 2021).
China is one of the largest high F− water areas worldwide. Except for Shanghai, high-fluoride groundwater exists in almost all provinces. High-fluoride water areas are mainly distributed in arid and semiarid areas in northern China. Most of the F− water concentrations are between 1.00 mg/L and 10.00 mg/L, exceeding the maximum permissible concentration of 1.00 mg/L in standards for drinking water quality (GB 5949-2006). Moreover, as detailed in this study, large amounts of data have measured the F− content in shallow groundwater in China (Lu et al., 2001; Zhou et al., 2005; Khair et al., 2014; Pi et al., 2015; Sun et al., 2015; Liu et al., 2015a; Li et al., 2019). In addition, by consulting the collected data and combining the results with literature findings, we established 5,464 datapoints of the F− concentration in shallow groundwater in China. With this database, the distribution of F− in shallow groundwater in China was determined based on the F− value of shallow groundwater in different provinces and regional blocks, and the occurrence form and environmental effect of F− were also discussed. In this way, the majority of the population can more intuitively understand F− in shallow groundwater in China, and this study provides a theoretical basis for the follow-up treatment of high-fluoride water. The purpose of this study is to quickly understand the distribution of F− in shallow groundwater in most regions of China through the establishment of a large number of F− concentration composition databases, contribute to the systematic assessment of the pollution behavior of F− in the environment, provide guidance for the geochemical behavior of F− in shallow groundwater in high-fluoride areas, and provide a basis for decision-makers to formulate targeted prevention and control measures of F− pollution.
Materials and methods
In this sample, the fluoride content of mine water in Panyi, Paner, Pansan, Guqiao, and Xieqiao of Huainan was measured. The data of fluorine content in other areas were obtained from (Lu et al., 2001; Zhou et al., 2005; Khair et al., 2014; Liu et al., 2015b; Pi et al., 2015; Li et al., 2019). A portable water quality tester (WTWoxi315i type) was used for on-site measurement of pH, total dissolved solids (TDS) and electrical conductivity (EC). The concentrations of F− were tested using an ion chromatograph (DIONEX, ICS-1500). The operating parameters of the suppressor were adjusted (ASRS=4 mm, I=30 mA) and the working pressure was set at 0.2 MPa. The mixed standard solution was prepared with standard substances and its peak height was measured. The standard curve was established with the peak height as the ordinate and the ion concentration as the abscissa, and the R2 was all greater than 0.999. Deionized water was used as the inhibitor and the solvent for the diluted samples. All samples were tested repeatedly for 3 times with a test accuracy of 0.01 mg/L. The anion test method referred to Environmental Protection Standards of China (HJ/T 84-2001).
IBM SPSS 20.0 software was used for statistical analysis of the experimental data (range, mean and standard deviation). Univariate analysis of variance (ANOVA) was used to compare the F− content in different areas and temporal and spatial differences, and to analyze the differences between different groups at the 0.05 and 0.01 significance levels. When the variances of the samples were homogeneous, least significant difference (LSD) was used to analyze the difference between the two groups. When the samples did not meet the variance homogeneity and normal distribution assumptions, the nonparametric Kruskal–Wallis one-way rank test was used. Pearson correlation analysis was used to test the linear correlation between F− concentrations in groundwater in different provinces, and the significance test method was a sampling two-sided t test. Origin 2019 software was used to draw all graphics, and all experimental data were expressed as the mean ± standard deviation.
Abundance and distribution of F- in shallow groundwater in China
Abundance of F− in shallow groundwater in China
Some researchers have studied the content of F− in shallow groundwater in China and achieved meaningful results (Guo et al., 2007; Wang et al., 2009; Wen et al., 2013). For example, Chen et al. (2021) found that the F− concentration in shallow groundwater was generally more than 1.00 mg/L, with local concentrations of 4.00 mg/L and individual concentrations of more than 20.00 mg/L. When discussing the safety and sustainable development of groundwater supply in China, Wang et al. (2019) found that the F− content in groundwater in cold areas of North China ranged from 0.10 mg/L to 22.00 mg/L (with most sampling locations exhibiting concentrations below 8.00 mg/L), and in 90% of geothermal water, the concentration was greater than 2.00 mg/L. Wang et al. (2009) and Wen et al. (2013) found that the F− concentration in shallow groundwater in China was high and tended to increase with increasing transpiration. F− showed relatively high concentrations in geothermal fluid; for example, the F− concentration in shallow groundwater was 17.90–19.60 mg/L in the Yangbajing Geothermal field, Tibet (Guo et al., 2007).
Among the 5,464 groundwater samples collected in this study, the F− content in shallow groundwater in China was between 0 and 60 mg/L, and the arithmetic mean value was 0.90 mg/L (Table 1). The mean F− concentration met the standards for drinking water quality of 1.00 mg/L. Among all samples, the F− content in 2,341 samples was in the range of 0.50–1.00 mg/L, which is a suitable F− water concentration, accounting for 42.84% of all samples. Moreover, 26.1% (1,425 samples) of the samples were in the range of 0.00–0.50 mg/L, indicating a low F− concentration, and the F− content in shallow groundwater of 128 samples exceeded 4.00 mg/L, accounting for only 2.34% of all samples (Figure 1A). The F− content in shallow groundwater in China showed a lognormal distribution (Figure 1B). The mean F− content in shallow groundwater in China in this study was just between 0.50 and 1.00 mg/L, which met the standards of the groundwater discharge in China, was higher than the F− content in groundwater in Iran (0.47 ± 0.28 mg/L) (Mesdaghinia et al., 2010), and was similar to that in Saudi Arabia (1.01 mg/L) (Alabdulaaly et al., 2013).
F− content in shallow groundwater in different provinces and cities in China
The mean F− content in shallow groundwater in 23 provinces and cities in China is shown in Figure 2, and other provinces and cities not investigated were F−-suitable areas. The mean F− content in shallow groundwater in Jiangxi, Chongqing and Yunnan provinces was below 0.50 mg/L, indicating low- F− areas. The mean F− content in shallow groundwater in Beijing, Liaoning, Shanxi, Shandong, Henan, Zhejiang, Gansu and Guangdong provinces and cities was in the range of 0.50–1.00 mg/L, indicating medium-fluoride areas. The mean F− content in groundwater in Anhui, Xinjiang, Tibet, Inner Mongolia, Guangxi, Fujian, Ningxia, Shaanxi, Jiangsu, Tianjin, Jilin, and Hebei was above 1.00 mg/L, indicating high-fluoride areas, and the highest value of 60.00 mg/L was located in the Ruijin Fluorite Mining Area in Jiangxi (Ding et al., 2005).
Because shallow groundwater in high-fluoride water areas has been used as drinking water, long-term drinking of the water by residents would lead to fluorosis (Yong and Hua 1991; Zhu et al., 2006; Bo et al., 2007; Gao et al., 2013; Jianmin et al., 2015; Zhang et al., 2018). Xu and Liu (2011) studied the distribution and formation mechanism of F− in shallow groundwater in the Huaibei Plain, Anhui Province. It was concluded that the F− concentration in water was higher than 1.00 mg/L, the highest value was 14.40 mg/L, and the probability of F− poisoning was 90% when drinking high-fluoride water for a long duration. Li et al. (2017) monitored 42 phreatic water samples in Tongyu County, Jilin Province, and found that the F− concentration in this area was in the range of 0.73–3.48 mg/L, and the mean content was 1.82 mg/L. In our database, 690 of the 5,464 shallow groundwater samples were derived from high-fluoride water areas, with a mean content of 1.30 mg/L.
F− content in shallow groundwater in different regions of China
Figure 3 shows the mean F− content in shallow groundwater in different regions of China, including North China, South China, Northeast China, East China, Central China, Southwest China and Northwest China. The lowest F− content in shallow groundwater in Southwest China was 0.36 mg/L, which was lower than the standard for drinking water of 1.00 mg/L. The mean F− water contents in South China, Northeast China and Northwest China were 1.20 mg/L, 1.25 mg/L and 1.25 mg/L, respectively, all exceeding 1.00 mg/L, indicating high-fluoride water areas. The mean F− water content in North China, East China and Central China was 0.93 mg/L, 0.67 mg/L and 0.80 mg/L, which was in the range of 0.50–1.0 mg/L, which was suitable for residents to drink and indicated a suitable F− water area. The high F− content in shallow groundwater in South China, Northeast China and Northwest China was mainly because these areas are close to coastal areas and affected by marine transgression, weather, drought, scarce rain and strong evaporation. In addition, flat terrain, slow groundwater movement and hydrochemical conditions (pH, salinity, total dissolved solids (TDS), other ions) also have some influence on F− content, resulting in the enrichment of F− in shallow groundwater (Zhao et al., 2007; Xu et al., 2013; Feng et al., 2015; Lu et al., 2016).
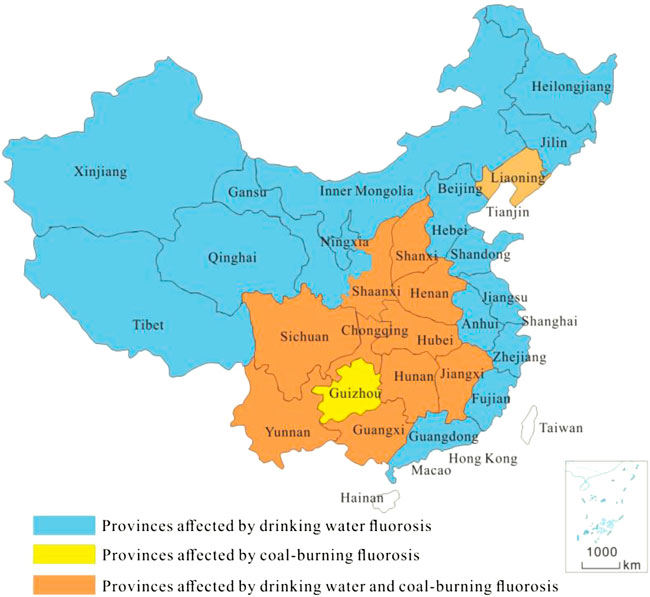
FIGURE 3. Provinces affected by drinking water fluorosis in various provinces in China (2017 years).
Forms of F− in shallow groundwater in China
F− exists in many forms in the environment. Due to the limitations of monitoring methods, the impact of complex forms of F− in water on the human body and environment is unclear. Studies have shown that the occurrence of fluoride in groundwater is related to the incidence rate of endemic fluorosis and has a negative effect on the human body (Ren et al., 1996; Marimon et al., 2013; Wang et al., 2015). There is no elemental form of F− in nature, which usually exists widely in the form of ions (Rafique et al., 2008; Ramachandran et al., 2012; Li et al., 2014; Zhang et al., 2016; Hu et al., 2017). Moreover, F− also shows strong complexation and the ability to replace hydrogen atoms in organic compound molecules (Zhang et al., 2016). Wang et al. (2015) summarized that the forms of F− in water mainly include ionic states, complex anionic states and molecular states of organic compounds.
A large number of reports have shown that the forms of F− in water is impacted by water F− chemical reactions, and the pH, hydrochemical composition, hardness and temperature of water are the decisive factors affecting water F− reactions (Chen and Yu 1990).
Ionic F−
The erosion and leaching of fluorinated rocks and minerals (fluorite, cryolite and apatite) are the main sources of ionic F− in groundwater. F− often forms soluble sodium and potassium salts. The ionic state of F− is the most active form in water (Reddy et al., 2010; Li et al., 2014). Some researchers found that ionic F− is closely related to groundwater chemical conditions (pH, mineralization) and hydrochemical types when discussing the occurrence of F− in groundwater (Li et al., 2013; Sajil et al., 2014; Na et al., 2016; Magesh et al., 2016; Hu et al., 2017; Emenike et al., 2018).
Hu et al. (2017) studied the F− ion concentration and pH of groundwater in the Huaibei Plain and found that F− was detected only under alkaline conditions at pH>6. The F− content increased with increasing pH in the pH range from 7 to 8 (Zhao et al., 2007; Selvam 2015). Li et al. (2013) found that the chemical characteristics of high-fluoride groundwater in the Aksu area, Xinjiang, were as follows: there was a significant positive correlation between the F− concentration and Ca2+, Mg2+, and ρ(K++Na+)/ρ(Ca2+) because F− easily reacted with Ca2+ and Mg2+ to produce CaF2 and MgF2 precipitation, causing a decrease in the F− concentration. Sajil Kumar et al. (2014) and Hu et al. (2017) found that the F− concentration ion was high even when the concentrations of Na+ and HCO3− were high.
There was a significant positive correlation between F− content and TDS by studying the change in TDS and other ion contents (F− content) in the Kriya River Basin (Namaiti et al., 2016) and the change in F− and TDS contents in Ogun State (Abeokuta South) and Nigeria aquifers by Emenike et al. (2018). High salinity and HCO3−-Na+-type water in shallow water would also increase the concentration of F− in groundwater (Magesh et al., 2016).
Complex ionic F−
Bai et al. (2013) found that F− easily forms soluble complexes with organic and inorganic ions in water, such as [HF2]-, [H2F3]-, [AlF]2+, FeFn3−n, [MnF]+, and [ZnF]+, which can enhance its mobility in water and is mainly related to the strong chemical activity of F−. Due to redox reactions, dissolution precipitation and complex dissociation between water and rock, water-soluble F− in soil is the main source of F− for the production of complex F− (Wang et al., 2015). Sawant et al. (1985) and Lang et al. (2016) detected F− complexes using F−-selective electrodes. In addition, the formation of complex F− was also related to the pH of hydrochemical conditions.
Some researchers have found that Fe, Al, Mg, and Ca react with F− to form stable complexes and precipitates in acidic environments, but this complex reaction cannot occur in alkaline environments (Chen et al., 2012; Bai et al., 2013; Ying et al., 2014; Ye 2015). The complexation ability of F− increases with increasing acidity. F− primarily exists in three forms, namely, HF2−, HF, and F−, when the pH is lower than 3, while F− aluminum and F− complexes increase in acidic environments at pH values above 5. Therefore, an acidic environment is an important condition for the formation of F− complexes in groundwater (Chen 2012; Ying et al., 2014).
Due to the existence of F− in a complex anionic state, some scholars have proposed the preparation of adsorbents through complexation to remove F− from water. For example, Zhu et al. (2011) synthesized a F− removal adsorbent, namely, an iron hydroxide and aluminum hydroxide complex, by using the strong adsorption of F− onto Fe (III) and Al (III). Ma et al. (2011) synthesized Mg-Al-Fe composite hydrotalcite by the coprecipitation method after calcination at 500°C to adsorb F− in water.
Molecular F− in organic F− compounds
Molecular F− in organic fluorination refers to F− replacing part or all of the hydrogen atoms connected to carbon atoms in organic compounds. When the hydrogen atoms are completely replaced, they are called perfluorinated organic compounds (PFCs) (Hudlicky 1961). PFCs are a new anthropogenic pollutant that migrate into groundwater through surface water and widely exist in China’s groundwater system (Ma 2013).
PFCs are persistent organic pollutants produced by humans and are mainly derived from factories producing clothing, carpet, leather, waterproof and fire extinguishing agents, glass, steel and other factories, which discharge domestic sewage, industrial wastewater and waste gas into water bodies directly or indirectly (Liu et al., 2015a). Mak et al. (2009) detected PFCs in the water of major cities in China and found that the lowest PFC in drinking water was observed in Beijing, at only 0.71 ng/L, and that in Shanghai was the highest, reaching 130.00 ng/L. Qi et al. (2016) sampled groundwater from 16 public drinking water sources in the suburbs of Tianjin and found that perfluorobutane sulfonate (PFBs) and perfluoro-octanoic acid (PFOA) were the main PFC pollutants. The content of PFCs in groundwater ranged from 0.32 to 8.30 ng/L, with a mean content of 2.40 ng/L. Chen et al. (2016) detected the content of PFCs in rural areas in eastern China and collected 50 shallow groundwater contents, with a total concentration of 5.30–615.00 ng/L, the highest of which was in a F- industrial park.
In summary, F− is mainly stored in groundwater in ionic, complex ionic and organic fluoride molecular states, and the forms are also different under different conditions. In acidic environments, F− is mainly present as ionic fluoride complexes, and in alkaline environments, F− is mainly present as ionic fluoride (Zuo et al., 2019; He et al., 2020). Organic molecular F− is mainly derived from anthropogenic pollutants, but the mechanism of toxicity is still unclear. Therefore, it is necessary to understand the occurrence forms of F− in water, especially organic molecular F−. Based on these findings, we can further understand the mechanism of action to provide a theoretical basis for the negative effect of endemic fluorosis on the human body and F− removal.
Environmental effects of F- in shallow groundwater in China
Since the 1970’s, with rapid economic development and the increasing demand for drinking water, a large amount of groundwater has been exploited, resulting in high F− content in groundwater in China, which poses a threat to water safety. F− in shallow groundwater in China mainly derives from the dissolution of fluoride-containing rocks and minerals, as well as fluoride-containing wastewater, waste gas and waste residue discharged from anthropogenic pollution (clothing, glass production, phosphate rock processing, steel smelting, etc.) into the water, resulting in an increase in F− content (Kim et al., 2012; Lu et al., 2016; Fuge 2019). Some scholars (Sun et al., 1993; Lan et al., 2008; Bao et al., 2019) have conducted relevant investigations on the eco-environmental effects caused by F− pollution in shallow groundwater in China, mainly focusing on the content of F− in crops, the correlation between F− and water, and geofluorosis.
For example, Sun et al. (1993) investigated the content and correlation between irrigation water and surrounding soil, grain and vegetables in fluorosis areas. The results showed that there was a significant positive correlation between the water F− content (5.35 mg/L) and soil water F− content (41.00 mg/L), wheat F− content (1.75 mg/kg) and spinach F− content (10.55 mg/L) (R1=0.92, P1<0.01; R2=0.58, P2<0.01; R3=0.40, P3<0.01). Lan et al. (2008) compared the F− content of vegetables in hot spring fluorosis areas and non-fluorosis areas of high F− groundwater. The F− content in vegetables in the fluorosis area was higher than that in the non-fluorosis area, and the F− fixation capacity of different parts was also different, such as roots (3.56 mg/kg) > flowers (3.23 mg/kg) > leaves (3.07 mg/kg) > stems (1.17 mg/kg). Bao et al. (2019) analyzed perfluoroalkyl substances (PFASs) in the groundwater of a fluorochemical park in Fuxin city and found that the contents of PFB and PFOA were as high as 21.20 μg/L and 2.51 μg/L, respectively, far exceeding the latest health warning issued by the U.S. Environmental Protection Agency. Moreover, PFB and PFOA were also detected in vegetables irrigated with groundwater.
If individuals in a certain area drink high-fluoride water for a long time, they can develop systemic chronic fluorosis disease (endemic fluorosis) (Srivastava et al., 2020). As shown in Figure 4, fluorosis in most provinces and cities in China is caused by drinking water fluorosis. Generally, when the F− concentration in drinking water is in the range of 1.00–3.00 mg/L, it increases the risk of dental fluorosis, and when the F− content in water is 4.00–6.00 mg/L, it easily causes skeletal fluorosis (Smith et al., 1977; Smith et al., 1979). According to the 2018 China Health Statistics Yearbook, 13,333,194 people suffered from dental fluorosis and 1,085,512 people suffered from skeletal fluorosis in 2017 in China. As shown in Figure 4, Jiangsu, Henan, Shandong, Hebei, Xinjiang and other provinces had the largest number of people suffering from dental fluorosis, with more than 1 million cases, while the number of people suffering from skeletal fluorosis in Inner Mongolia, Jiangsu, Shandong, Shaanxi and other provinces also exceeded 10 thousand. Li et al. (2005) collected blood samples from 60 newborns delivered in Zhaozhou County, a high-fluoride area in Heilongjiang Province. It was found that the serum osteocalcin (BGP) content in umbilical cord blood of newborns in the high-fluoride area was higher than that of newborns in a normal area, and the calcitonin (CT) content was lower than that of newborns in the normal area, revealing the changes in bone metabolism of newborns in the high-fluoride area. Zhai et al. (2019) investigated and analyzed the impact of changing F− precipitation on endemic fluorosis in Shandong Province. Using the cross-sectional survey method, 732 children aged 7-12 in fluorosis areas were investigated for dental fluorosis and urinary fluoride. The detection rate of dental fluorosis was 73.2%, the detection rate of dental fluorosis in girls (77.4%) was higher than that in boys (69.6%), and that in older adults group (76.9%) was higher than that in the younger group (65.5%). The urinary fluoride content of children with dental fluorosis (4.50 ± 2.70) was higher than that of children without dental fluorosis (1.90 ± 1.50). Ding et al. (2011) randomly detected the urinary F− content of 338 children in Hohhot, Inner Mongolia, and explored the relationship between urinary fluoride, children’s intelligence and dental fluorosis. The results demonstrated that when the F− content in drinking water was 1.31 ± 1.05 mg/L, the urinary fluoride content was negatively correlated with IQ, and there was a certain dose-response relationship with dental fluorosis. Chen et al. (2008) examined and collected the clinical symptoms, signs and X-ray examination of 1,517 adults over 30 years old in Qingdao, Shandong Province. It was found that the detection rate of X-ray skeletal fluorosis was as high as 15.00%, and with increasing age, the probability of suffering from skeletal fluorosis increased and the more serious the condition was. Some scholars have also found that an appropriate amount of low-dose fluoride in older adults can potentially protect cognitive function, but excessive intake may increase the risk of cognitive impairment (Li et al., 2017). Therefore, in terms of drinking water safety and public health, F− reduction and water improvement are still top priorities in China’s development in the next few years.
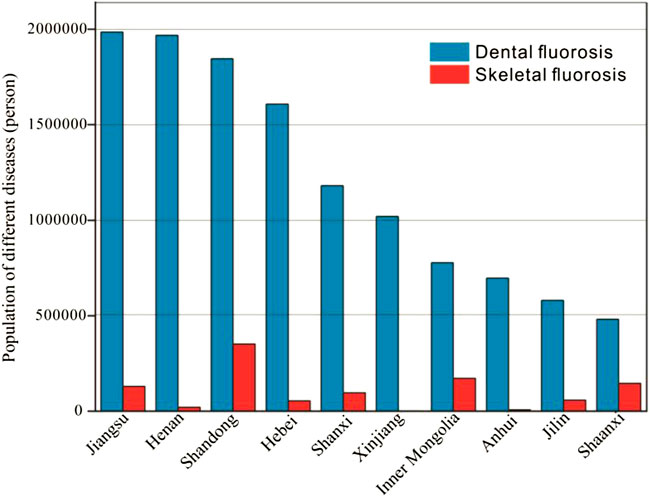
FIGURE 4. Number of patients with dental fluorosis and skeletal fluorosis in some provinces and cities in 2017 in China.
Conclusion
Based on the analysis of the content distribution characteristics, occurrence forms and environmental effects of F− in shallow groundwater in China, the environmental geochemical characteristics of F− in groundwater in China were obtained.
(1) The mean F− concentration in shallow groundwater in China was between 0.00 and 60.00 mg/L, and the mean value was 0.90 mg/L, which met the standard for drinking water and was similar to the mean F- content of 1.01 mg/L in Saudi Arabia but higher than that of 0.47 ± 0.28 mg/L in Iran.
(2) High-fluoride areas in different regions of China were divided as follows: low-fluoride area, Southwest region (0.36 mg/L); suitable F− areas, North China (0.93 mg/L), East China (0.67 mg/L) and Central China (0.80 mg/L); and high-fluoride areas, South China (1.20 mg/L), Northeast China (1.25 mg/L) and Northwest China (1.25 mg/L).
(3) F− in China’s groundwater mainly exists in three forms: ionic, ionic complex and organic fluoride molecular states. F− is mainly present as ionic F− complexes in acidic environments and as ionic F− in alkaline environments. Organic molecular F− is mainly derived from new anthropogenic pollutants, the toxicity mechanism of which is still unclear.
(4) The environmental effects caused by F− pollution in shallow groundwater in China were mainly concentrated in the content of F− in crops, the correlation between F− and water pollution, and endemic fluorosis. The higher the F− content in water is, the higher the F− content in soil, wheat, spinach and other vegetables. Moreover, the different parts of plants displayed different F− fixation capacities, higher F− concentrations were observed in plant roots than in flowers, stems, and pages. In addition, the symptoms of endemic fluorosis were found to cause people of different ages varied. The symptoms of endemic fluorosis caused people of different ages varied. (Fuhong and Shuqin, 1988; Li et al., 2015; Liu et al., 2018).
Data availability statement
The original contributions presented in the study are included in the article/Supplementary material, further inquiries can be directed to the corresponding author.
Author contributions
PZ: methodology, formal analysis, software, formal analysis, writing—original draft, and visualization. SZ and KX: data curation, validation, writing—review and editing. PS and LZ: writing—review and editing. LZ: supervision, conceptualization, resources, project administration, and funding acquisition.
Funding
This work was supported by the National Natural Science Foundation of China (No. 42072201), Natural Science Foundation of Anhui Province (No. 1608085QD79).
Acknowledgments
We would like to thank the editors and reviewers for providing suggestions regarding the language and extensive discussion.
Conflict of interest
The authors declare that the research was conducted in the absence of any commercial or financial relationships that could be construed as a potential conflict of interest.
Publisher’s note
All claims expressed in this article are solely those of the authors and do not necessarily represent those of their affiliated organizations, or those of the publisher, the editors and the reviewers. Any product that may be evaluated in this article, or claim that may be made by its manufacturer, is not guaranteed or endorsed by the publisher.
References
Adeyeye, O. A., Xiao, C. L., Zhang, Z. H., Yawe, A. S., and Liang, X. J. (2021). Groundwater fluoride chemistry and health risk assessment of multi-aquifers in Jilin Qianan, Northeastern China. Ecotoxicol. Environ. Saf. 211, 111926. doi:10.1016/j.ecoenv.2021.111926
Alabdulaaly, A. I., Al-Zarah, A. I., and Khan, M. A. (2013). Occurrence of fluoride in ground waters of Saudi Arabia. Appl. Water Sci. 3 (3), 589–595. doi:10.1007/s13201-013-0105-2
Bai, S. Y., Li, W., Liu, F., Hua, J., and Li, Z. Y. (2013). Research progress of groundwater fluoride removal and prospect of Schwittmann stone application. Water supply drainage 49 (S1), 4–8.
Bao, J., Yu, W., Liu, Y., Wang, X., Jin, Y. H., and Dong, G. H. (2019). Perfluoroalkyl substances in groundwater and home-produced vegetables and eggs around a fluorochemical industrial park in China. Ecotoxicol. Environ. Saf. 171, 199–205. doi:10.1016/j.ecoenv.2018.12.086
Bhargava, D., and Bhardwaj, N. (2010). Effect of sodium fluoride on seed germination and seedling growth of Triticum aestivum var. J. Phytology 2 (4), 41–43.
Bo, Z., Mei, H., Zhang, X., and Zhao, Y. (2007). Fluoride distribution in aquatic environment and its health effect in the Western Region of the Songnen Plain, Northeast China. Environmental Monitoring and Assessment, 379–386.
Bustingorri, C., Balestrasse, K., and Lavado, R. S., (2016). Effects of high arsenic and fluoride soil concentrations on soybean plants. Phyton 84 (2), 407–416. doi:10.32604/phyton.2015.84.407
Chen, J., Gao, Y. Y., Qian, H., Ren, W. H., and Qu, W. G. (2021). Hydrogeochemical evidence for fluoride behavior in groundwater and the associated risk to human health for a large irrigation plain in the Yellow River Basin. Science of the Total Environment, 800
Chen, P. Z., Li, P., Yun, Z. J., Li, H. X., Su, H., and Li, Z. Y. (2008). Investigation and analysis on prevalence of skeletal fluorosis in adults in endemic fluorosis areas of Qingdao city. Chin. J. Endemic Dis. Control 1, 12–14.
Chen, S. F. (2012). Experimental study on treatment of high fluoride groundwater by modified natural zeolite. Beijing: China University of Geosciences.
Chen, S., Jiao, X. C., Gai, N., Li, X. J., Wang, X. C., Lu, G. H., et al. (2016). Perfluorinated compounds in soil, surface water, and groundwater from rural areas in eastern China. Environ. Pollut. 211, 124–131. doi:10.1016/j.envpol.2015.12.024
Ding, L. X., and Luo, X. H. (2005). “Distribution of high fluoride groundwater in Jiangxi Province and water improvement in drinking water endemic fluorosis areas in poor mountainous areas,”China , 229–232.National Symposium on groundwater resources and environment.
Ding, Y., Yan, H. G., Sun, H., Han, H., Wang, W., Ji, X., et al. (2011). The relationships between low levels of urine fluoride on children"s intelligence, dental fluorosis in endemic fluorosis areas in Hulunbuir, Inner Mongolia, China. J. Hazard. Mater. 186 (2-3), 1942–1946. doi:10.1016/j.jhazmat.2010.12.097
Durrani, T. S., and Farooqi, A. (2021). Groundwater fluoride concentrations in the watershed sedimentary basin of Quetta Valley, Pakistan. Environ. Monit. Assess. 193 (10), 644. doi:10.1007/s10661-021-09365-8
Elloumi, N., Zouari, M., Chaari, L., Rouina, B. B., Abdallah, F. B., and Kallel, M. (2015). Morphological and physiological changes induced in Olea europaea, and Prunus dulcis, exposed to air fluoride pollution. Braz. J. Bot. 38 (1), 99–106. doi:10.1007/s40415-014-0112-z
Emenike, P. G., Tenebe, I. T., and Jarvis, P. (2018). Fluoride contamination in groundwater sources in Southwestern Nigeria: Assessment using multivariate statistical approach and human health risk. Ecotoxicol. Environ. Saf. 156, 391–402. doi:10.1016/j.ecoenv.2018.03.022
Fan, B., Yu, Y., and Zhang, Y., (2015). PI3K-Akt1 expression and its significance in liver tissues with chronic fluorosis. Int. J. Clin. Exp. Pathol. 8 (2), 1226–1236.
Feng, J. G., Duan, J. F., and Gao, Z. J., (2015). Fluoride characteristics in shallow groundwater in the gaomi area, Shandong province, China. China: WIT Transactions on the Built Environment, 465–472.
Fuge, R. (2019). Fluorine in the environment, a review of its sources and geochemistry. Appl. Geochem. 100, 393–406. doi:10.1016/j.apgeochem.2018.12.016
Fuhong, R., and Shuqin, J. (1988). Distribution and formation of high-fluorine groundwater in China. Environ. Geol. Water Sci. 12 (1), 3–10. doi:10.1007/bf02574820
Gao, H. J., Jin, Y. Q., and Wei, J. L. (2013). Health risk assessment of fluoride in drinking water from Anhui Province in China. Environ. Monit. Assess. 185 (5), 3687–3695. doi:10.1007/s10661-012-2820-9
Gao, J. X., Liu, R. A., and Shu, J. M., (1998). Research Progress on the effects of fluoride on plant metabolism. Chin. J. Environ. Eng. 3, 24–29.
Gao, Z. J., Shi, M. J., Zhang, H. Y., Feng, J. G., Fang, S. Y., and Cui, Y. C. (2020). Formation and in situ treatment of high fluoride concentrations in shallow groundwater of a semi-arid region: Jiaolai basin, China. Int. J. Env. Res. Pub. He. 17 (21), 8075. doi:10.3390/ijerph17218075
Guo, Q., Wang, Y., Ma, T., and Ma, R. (2007). Geochemical processes controlling the elevated fluoride concentrations in groundwaters of the Taiyuan Basin, Northern China. J. Geochem. Explor. 93 (1), 1–12. doi:10.1016/j.gexplo.2006.07.001
He, X. D., Li, P. Y., Ji, Y. J., Wang, Y. H., Su, Z. M., and Vetrimurugan, E. (2020). Groundwater arsenic and fluoride and associated arsenicosis and fluorosis in China: Occurrence, distribution and management. Expo. Health 12 (3), 355–368. doi:10.1007/s12403-020-00347-8
Hossain, M., Patra, P. K., Ghosh, B., Khatun, A., and Nayek, S. (2021). Sensitive assessment of groundwater-associated, multi-exposure health hazards in a fluoride-enriched region of West Bengal, India. Environ. Geochem. Health 43 (11), 4515–4532. doi:10.1007/s10653-021-00942-x
Hu, Y., Xia, C., Dong, Z., and Liu, G. (2017). Geochemical characterization of fluoride in the groundwater of the Huaibei Plain, China. Anal. Lett. 50 (5), 889–903. doi:10.1080/00032719.2016.1199027
Jianmin, B., Yu, W., and Juan, Z., (2015). Arsenic and fluorine in groundwater in Western Jilin province, China: Occurrence and health risk assessment. Nat. Hazards 77 (3), 1903–1914. doi:10.1007/s11069-015-1682-1
Katsanou, K., Siavalas, G., and Lambrakis, N. (2013). Geochemical controls on fluoriferous groundwaters of the Pliocene and the more recent aquifers: The case of Aigion region, Greece. J. Contam. Hydrology 155, 55–68. doi:10.1016/j.jconhyd.2013.08.009
Khair, A. M., Li, C., Hu, Q., Gao, X., and Wanga, Y. (2014). Fluoride and arsenic hydrogeochemistry of groundwater at Yuncheng basin, Northern China. Geochem. Int. 52 (10), 868–881. doi:10.1134/s0016702914100024
Kim, S. K., Im, J. K., Kang, Y. M., Jung, S. Y., Kho, Y. L., and Zoh, K. D. (2012). Wastewater treatment plants (WWTPs)-derived national discharge loads of perfluorinated compounds (PFCs). J. Hazard. Mater. 201, 82–91. doi:10.1016/j.jhazmat.2011.11.036
Kurdi, M. S. (2016). Chronic fluorosis: The disease and its anaesthetic implications. Indian J. Anaesth. 60 (3), 157–162. doi:10.4103/0019-5049.177867
Lan, D., Wu, D., Li, P., Wang, T., Chen, C., and Wang, W. (2008). Influence of high-fluorine environmental background on crops and human health in hot spring-type fluorosis-diseased areas. Chin. J. Geochem. 27 (4), 335–341. doi:10.1007/s11631-008-0335-4
Lang, W., Zhang, W., Tang, Y., Xu, J., and Zhang, L. (2016). Determination of boron trifluoride in boron trifluoride complex by fluoride ion selective electrode. Trans. Tianjin Univ. 22 (1), 83–88. doi:10.1007/s12209-016-2646-6
Li, L., Zhou, J. L., Qi, W. Q., Chen, F., and Zeng, Y., (2019). Distribution characteristics and formation process of fluoride in groundwater in oasis area of Hotan River Basin. J. Arid Land Resour. Environ. 33 (1), 114–120.
Li, J. X., Wang, Y. T., Zhu, C. J., Xue, X. B., Qian, K., Xie, X. J., et al. (2020). Hydrogeochemical processes controlling the mobilization and enrichment of fluoride in groundwater of the North China Plain. Sci. Total Environ. 730, 138877. doi:10.1016/j.scitotenv.2020.138877
Lu, M. S., Wu, E. J., and Li, J. M. (2001). Hydrogeochemical study on the Genesis of shallow high fluoride groundwater in Southwest Shandong. Coal Geol. Explor. 29 (5), 39–42.
Li, Y., Qing, L. S., and Jing, L. I. (2005). Determination and analysis of serum osteocalcin and calcitonin in cord blood of new born infants in excess-fluoride-area. Chin. J. Endemiology 24 (1), 83–84.
Li, Q., Jia, R. L., and Zhou, J. L., (2013). Chemical characteristics of high fluoride groundwater in Aksu, Xinjiang. Arid land Resour. Environ. 27 (12), 87–92.
Li, P., Qian, H., Wu, J., Chen, J., Zhang, Y., and Zhang, H. (2014). Occurrence and hydrogeochemistry of fluoride in alluvial aquifer of Weihe River, China. Environ. Earth Sci. 71 (7), 3133–3145. doi:10.1007/s12665-013-2691-6
Li, M., Gao, Y., Cui, J., Li, Y., Li, B., Liu, Y., et al. (2015). Cognitive impairment and risk factors in elderly people living in fluorosis areas in China. Biol. Trace Elem. Res. 172 (1), 53–60. doi:10.1007/s12011-015-0568-0
Liu, H., Guo, H., Yang, L., Wu, L., Li, F., Li, S., et al. (2015a). Occurrence and formation of high fluoride groundwater in the Hengshui area of the North China Plain. Environ. Earth Sci. 74 (3), 2329–2340. doi:10.1007/s12665-015-4225-x
Liu, Y., Dong, W. N., and Li, H., (2015b). Present situation and research progress of perfluorinated organic compounds pollution in surface water. Environ. Eng. 33 (2), 43–47.
Lu, J., Qiu, H., Lin, H., Yuan, Y., Chen, Z., and Zhao, R. (2016). Source apportionment of fluorine pollution in regional shallow groundwater at You’xi County southeast China. Chemosphere 158, 50–55. doi:10.1016/j.chemosphere.2016.05.057
Li, X. L., Sun, X. Q., and Bian, J. M., (2017). Fluoride distribution and health risk assessment in phreatic water in Tongyu County, Jilin Province. Water Resour. Power 6, 30–34.
Liu, C. H., Wang, W., and Wei, Z. R., (2018). Distribution characteristics and genesis of high fluoride groundwater in weishan lake watershed. Acta Geosci. Sin. 39 (3), 107–113. (In Chinese)
Liu, J. T., Peng, Y. M., Li, C. S., Gao, Z. J., and Chen, S. J. (2021). A characterization of groundwater fluoride, influencing factors and risk to human health in the southwest plain of Shandong Province, North China. Ecotoxicol. Environ. Saf. 207, 111512. doi:10.1016/j.ecoenv.2020.111512
Ma, W., Zhao, N., Yang, G., Tian, L., and Wang, R. (2011). Removal of fluoride ions from aqueous solution by the calcination product of Mg-Al-Fe hydrotalcite-like compound. Desalination 268 (1-3), 20–26. doi:10.1016/j.desal.2010.09.045
Ma, L. (2013). “Occurrence and distribution characteristics of new pollutants in groundwater. Chinese Society of Environmental Science,”China (Yunan: Chinese Society of Environmental Sciences). 5.Proceedings of the annual conference of Chinese society for environmental sciences.
Magesh, N. S., Chandrasekar, N., and Elango, L. (2016). Occurrence and distribution of fluoride in the groundwater of the tamiraparani River Basin, South India: A geostatistical modeling approach. Environ. Earth Sci. 75 (23), 1483. doi:10.1007/s12665-016-6293-y
Maitra, A., Keesari, T., Roy, A., and Gupta, S. (2020). Fluoride contamination in and around selected geothermal sites in odisha, eastern India: Assessment of ionic relations, fluoride exposure and remediation. Environ. Sci. Pollut. Res. 28 (15), 18553–18566. doi:10.1007/s11356-020-10948-0
Mak, Y. L., Taniyasu, S., Yeung, L., Lu, G., Jin, L., Yang, Y., et al. (2009). Perfluorinated compoundsin tap water from China and several other countries. Environ. Sci. Technol. 43 (13), 4824–4829. doi:10.1021/es900637a
Marimon, M. P. C., Roisenberg, A., Viero, A. P., Camargo, F. A. D., and Suhogusoff, A. V. (2013). Evaluation of the potential impact of fluorine-rich fertilizers on the guarani aquifer system, rio grande do sul, southern Brazil. Environ. Earth Sci. 69 (1), 77–84. doi:10.1007/s12665-012-1935-1
Meng, F. P., and Wu, F. Z. (1996). Effects of fluoride on plant physiology and Biochemistry. J. Ecol. Rural Environ. 12 (1), 42–46.
Mesdaghinia, A., Vaghefi, K. A., Montazeri, A., Mohebbi, M. R., and Saeedi, R. (2010). Monitoring of fluoride in groundwater Resources of Iran. Bull. Environ. Contam. Toxicol. 84 (4), 432–437. doi:10.1007/s00128-010-9950-y
Mohammadi, A. A., Yousefi, M., and Mahvi, A. H. (2017). Fluoride concentration level in rural area in Poldasht city and daily fluoride intake based on drinking water consumption with temperature. Data Brief 13 (C), 312–315. doi:10.1016/j.dib.2017.05.045
Mondal, D., Dutta, G., and Gupta, S. (2016). Inferring the fluoride hydrogeochemistry and effect of consuming fluoride-contaminated drinking water on human health in some endemic areas of Birbhum district. West Bengal. Environ. Geochem. Health 38 (2), 557–576. doi:10.1007/s10653-015-9743-7
Morales-Arredondo, I., Rodriguez, R., Armienta, M. A., and Villanueva-Estrada, R. (2016). The origin of groundwater arsenic and fluorine in a volcanic sedimentary basin in central Mexico: A hydrochemistry hypothesis. Hydrogeol. J. 24 (4), 1029–1044. doi:10.1007/s10040-015-1357-8
Mumtaz, N., Pandey, G. C., and Labhasetwar, P., (2015). Global fluoride occurrence, available technologies for fluoride removal, and electrolytic defluoridation: A review. Crit. Rev. Environ. Sci. Technol. 45 (21), 00–2389. doi:10.1080/10643389.2015.1046768
Nafouanti, M. B., Li, J. X., Mustapha, N. A., Uwamungu, P., and Al-Alimi, D. (2021). Prediction on the fluoride contamination in groundwater at the Datong Basin, Northern China: Comparison of random forest, logistic regression and artificial neural network. Appl. Geochem. 132, 105054. doi:10.1016/j.apgeochem.2021.105054
Namaiti, T., Zhang, F., and Shi, Q. D. (2016). Spatial distribution of fluoride ions in keriya River basin. Arid. zone study 33 (5), 1125–1131.
Nikiforova, V. I. (1982). Mechanism of the mutagenic action of fluorine. Tsitol. Genet. 16 (6), 40–42.
Patil, M. M., Lakhkar, B. B., and Patil, S. S. (2018). Curse of fluorosis. Indian J. Pediatr. 85 (5), 375–383. doi:10.1007/s12098-017-2574-z
Pi, K., Wang, Y., Xie, X., Su, C., Li, J., et al. (2015). Hydrogeochemistry of co-occurring geogenic arsenic, fluoride and iodine in groundwater at Datong Basin, northern China. J. Hazard. Mater. 300, 652–661. doi:10.1016/j.jhazmat.2015.07.080
Qi, Y., Huo, S., Hu, S., Xi, B., Su, J., and Tang, Z. (2016). Identification, characterization, and human health risk assessment of perfluorinated compounds in groundwater from a suburb of Tianjin, China. Environ. Earth Sci. 75 (5), 432. doi:10.1007/s12665-016-5415-x
Rafique, T., Naseem, S., Bhanger, M. I., and Usmani, T. H. (2008). Fluoride ion contamination in the groundwater of Mithi sub-district, the Thar Desert, Pakistan. Environ. Geol. 56 (2), 317–326. doi:10.1007/s00254-007-1167-y
Ramachandran, M., Sabarathinam, C., Ulaganthan, K., Paluchamy, A., Sivaji, M., and Hameed, S. (2012). Mapping of fluoride ions in groundwater of dindigul district, tamilnadu, India—Using GIS technique. Arab. J. Geosci. 5 (3), 433–439. doi:10.1007/s12517-010-0216-0
Reddy, D. V., Nagabhushanam, P., Sukhija, B. S., Reddy, A., and Smedley, P. (2010). Fluoride dynamics in the granitic aquifer of the Wailapally watershed, Nalgonda District, India. Chem. Geol. 269 (3-4), 278–289. doi:10.1016/j.chemgeo.2009.10.003
Reddy, M. P., and Kaur, M. (2008). Sodium fluoride induced growth and metabolic changes inSalicornia brachiataRoxb. Water Air Soil Pollut. 188 (1-4), 171–179. doi:10.1007/s11270-007-9533-7
Ren, F. H., Zeng, J. H., and Liu, W. S., (1996). Hydrogeochemical environment of high fluoride groundwater and the relationship between the occurrence form of fluoride and the prevalence of geofluorosis - a case study of North China Plain. Acta Geosci. Sin. 17 (1), 85–97.
Sajil Kumar, P. J., Jegathambal, P., and James, E. J. (2014). Factors influencing the high fluoride concentration in groundwater of Vellore District, South India. Environ. Earth Sci. 72 (7), 2437–2446. doi:10.1007/s12665-014-3152-6
Sarinana-Ruiz, Y. A., Vazquez-Arenas, J., Sosa-Rodriguez, F. S., Labastida, I., Armienta, M. A., Aragon-Pina, A., et al. (2017). Assessment of arsenic and fluorine in surface soil to determine environmental and health risk factors in the Comarca Lagunera, Mexico. Chemosphere 178, 391–401. doi:10.1016/j.chemosphere.2017.03.032
Sawant, R. M., Rizvi, G. H., Chaudhuri, N. K., and Patil, S. K. (1985). Determination of the stability constant of Np (V) fluoride complex using a fluoride ion selective electrode. J. Radioanalytical Nucl. Chem. Articles 89 (2), 373–378. doi:10.1007/bf02040601
Selvam, S. (2015). A preliminary investigation of lithogenic and anthropogenic influence over fluoride ion chemistry in the groundwater of the southern coastal city, Tamilnadu, India. Environ. Monit. Assess. 187 (3), 106–1131. doi:10.1007/s10661-015-4326-8
Senarathne, S., Jayawardana, J. M. C. K., and Chandrajith, R. (2021). Influence of climate on groundwater fluoride in different climatic domains in a hard rock terrain of Sri Lanka: Implications to community health. Environ. Geochem. Health 44, 3677–3686. doi:10.1007/s10653-021-01090-y
Senthilkumar, M., Mohapatra, B., Gnanasundar, D., and Gupta, S. (2021). Identifying fluoride endemic areas and exposure pathways for assessment of non-carcinogenic human health risk associated with groundwater fluoride for Gujarat state, India. Environ. Sci. Pollut. Res. 28 (36), 50188–50203. doi:10.1007/s11356-021-14156-2
Seraj, B., Shahrabi, M., Shadfar, M., Ahmadi, R., FallahzadehM., , Eslamlu, H. F., et al. (2012). Effect of high water fluoride concentration on the intellectual development of children in makoo/Iran. J. Dent. 9 (3), 221–229.
Smith, F. A., Hodge, H. C., and Dinman, B. D. (1977). Airborne fluorides and man: Part I. C R C Crit. Rev. Environ. Control 8 (1-4), 293–371. doi:10.1080/10643387709381665
Smith, F. A., Hodge, H. C., and Dinman, B. D. (1979). Airborne fluorides and man: Part II. C R C Crit. Rev. Environ. Control 9 (1), 1–25. doi:10.1080/10643387909381666
Srivastava, S., and Flora, S. J. S. (2020). Fluoride in drinking water and skeletal fluorosis: A review of the global impact. Curr. Envir. Health Rpt. 7 (2), 140–146. doi:10.1007/s40572-020-00270-9
Su, H., Kang, W. D., Li, Y. R., and Li, Z. (2021). Fluoride and nitrate contamination of groundwater in the Loess Plateau, China: Sources and related human health risks. Environ. Pollut. 286, 117287. doi:10.1016/j.envpol.2021.117287
Sun, W. B., Li, Y. J., and Zhao, S. X. (1993). Study on the relationship between irrigation water and fluoride content in soil, grain and vegetable. Chin. J. Local Epidemiol. 2, 89–91.
Sun, X., Wang, S., Zhao, H., and Yuan, R. (2015). Distribution Characteristics and Source of Fluoride in Groundwater in Lower Plain Area of North China Plain: A Case Study in Nanpi County. Environmental Science 36 (11), 4051–4059.
Tarki, M., Enneili, A., and Dassi, L. (2020). An appraisal of natural fluorine contamination of paleogroundwater in Tozeur oases, southern Tunisia, with emphasis on the anthropogenic impact. Appl. Geochem. 120, 104661. doi:10.1016/j.apgeochem.2020.104661
Wang, L., Huang, J. C., and Li, M. (2015). Analysis of occurrence forms of fluoride in groundwater and negative effects on human body. J. Hunan Ecol. Sci. 2 (03), 18–25.
Wang, M., Li, X., He, W. Y., and Li, J. X. (2019). Distribution, health risk assessment, and anthropogenic sources of fluoride in farmland soils in phosphate industrial area, southwest China. Environmental Pollution 249, 423–433.
Wang, L. X., Wang, K. Z., Wang, X. N., and Wang, Q. (2011). Changes in myeloperoxidase glutathione S-transferase, and endocrine function in sterile males from areas with highly fluorided water. J. Pathogen Biol. 6 (09), 661–662.
Wang, Y., Shvartsev, S. L., and Su, C. (2009). Genesis of arsenic/fluoride-enriched soda water: A case study at datong, northern China. Appl. Geochem. 24 (4), 641–649. doi:10.1016/j.apgeochem.2008.12.015
Wen, D., Zhang, F., Zhang, E., Wang, C., Han, S., and Zheng, Y. (2013). Arsenic, fluoride and iodine in groundwater of China. J. Geochem. Explor. 135, 1–21. doi:10.1016/j.gexplo.2013.10.012
Xie, Z. M., Wu, W. H., and Xu, J. M. (1999). Migration and transformation of fluoride in environment and its ecological effects. Chin. J. Environ. Eng. 2, 40–53.
Xu, F., Ma, T., Shi, L., Zhang, J., Wang, Y., and Dong, Y. (2013). The hydrogeochemical characteristics of high iodine and fluoride groundwater in the hetao plain, inner Mongolia. Procedia Earth Planet. Sci. 7, 908–911. doi:10.1016/j.proeps.2013.03.183
Xu, G., and Liu, L. (2011). “The distribution and formation mechanism of the fluoride in shallow groundwater of Huaibei plain in Anhui province,”, 294–297.International symposium on water resource and environmental protection.
Xu, W., Zhou, J. M., Qin, W., Shen, L., and Li, X. D. (2016). Fluoride removal from drinking water by the process combined internal circulating fluidized bed and membrane. Chin. J. Environ. Eng. 10 (9), 4698–4704.
Yao, L., Shao, Q. L., Li, J., and Fu, Z. Q. (2005). Determination and analysis of serum osteocalcin and calcitonin in cord blood of new born infants in excess-fluoride-area. Chin. J. Endemiology 24 (1), 83–84.
Ye, Y. Y. (2015). Competitive adsorption of co-existing ions in fluoride removal with modified Pullulan composite adsorbent. China: Huazhong University of Science and Technology.
Ying, H., Zhang, X. C., and Hao, H. J. (2014). The influence of citric acid with different pH on fluoride components in tea garden soil leaching solution ring. Environ. Chem. 11, 1885–1892.
Yong, L., and Hua, Z. W. (1991). Environmental characteristics of regional groundwater in relation to fluoride poisoning in North China. Environ. Geol. Water Sci. 18 (1), 3–10. doi:10.1007/bf01704572
Zhai, L. P., Xi, J., Li, L., Gao, J., Wang, K., and Wei, G. X. (2019). Effects of water-improving and defluoridation projects on dental fluorosis in 7 - 12 years old children and related factors in Shandong Province. Chin. Jour 38 (1), 45–49.
Zhang, H. T., Tong, J. D., Jiang, S., Zhang, X. L., and Zhang, S. W., (2018). Analysis of screening results of fluoride content in drinking water of residents in fluorosis area of decentralized water supply in Jilin Province. Chin. J. Control Endemic Dis. 33 (5), 509–569.
Zhang, S., Lyu, Y., Su, X., Bian, Y., Yu, B., and Zhang, Y. (2016). Removal of fluoride ion from groundwater by adsorption on lanthanum and aluminum loaded clay adsorbent. Environ. Earth Sci. 75 (5), 401. doi:10.1007/s12665-015-5205-x
Zhang, Y. Z., Wang, B., Wang, W., Li, W. C., Huang, J., Deng, S. B., et al. (2016). Occurrence and source apportionment of per- and poly-fluorinated compounds (PFCs) in North canal basin, beijing. Sci. Rep. 6 (1), 36683. doi:10.1038/srep36683
Zhao, S. Z., Wan, X. K., and Huang, Z. F., (2007). Genetic analysis of high fluoride water in Hetao region of Inner Mongolia. Testing 26 (4), 320–324.
Zhong, Q. S., Lin, Z. L., and Chen, C. S., (2014). Effect of fluoride-stress on photosynthesis of tea leaves. Fujian J. Agric. Sci. 29 (3), 233–238.
Zhou, L., Feng, Q. Y., and Li, H. Y. (2005). Hydrogeochemical characteristics of fluoride in shallow groundwater of tongshan area. J. China Univ. Min. Technol. 15 (2), 110–113.
Zhu, C., Bai, G., Liu, X., and Li, Y. (2006). Screening high-fluoride and high-arsenic drinking waters and surveying endemic fluorosis and arsenism in Shaanxi province in Western China. Water Res. 40 (16), 3015–3022. doi:10.1016/j.watres.2006.06.026
Zhu, H., Wang, G., and Wang, H., (2011). “Removal of fluoride from water by using the synthetical iron-aluminum hydroxide complexes,”, 6771–6773.International conference on electric technology and civil engineering.
Keywords: shallow groundwater, F-distribution, environmental effects, occurrence, form
Citation: Zhao P, Zhang S, Xu K, Zhao Y, Shen P, Zhu L and Zheng L (2023) Fluorine in shallow groundwater in China: A review of distribution, occurrence and environmental effects. Front. Earth Sci. 10:1084890. doi: 10.3389/feart.2022.1084890
Received: 31 October 2022; Accepted: 14 November 2022;
Published: 10 January 2023.
Edited by:
Dun Wu, Anhui Jianzhu University, ChinaReviewed by:
Herong Gui, Suzhou University, ChinaJian Chen, Anhui University of Science and Technology, China
Copyright © 2023 Zhao, Zhang, Xu, Zhao, Shen, Zhu and Zheng. This is an open-access article distributed under the terms of the Creative Commons Attribution License (CC BY). The use, distribution or reproduction in other forums is permitted, provided the original author(s) and the copyright owner(s) are credited and that the original publication in this journal is cited, in accordance with accepted academic practice. No use, distribution or reproduction is permitted which does not comply with these terms.
*Correspondence: Ping Zhao, bmp1emhwQHNpbmEuY29t