- 1School of Resources and Environment, Henan Polytechnic University, Jiaozuo, China
- 2State Key Laboratory of Coal Resources and Safe Mining, College of Geoscience and Surveying Engineering, China University of Mining and Technology (Beijing), Beijing, China
- 3Collaborative Innovation Center of Coal Work Safety and Clean High Efficiency Utilization, Jiaozuo, China
The C1 coal of Latest Permian during mass extinction in eastern Yunnan was studied to reveal the terrestrial paleoenvironment and influence of geological events on coal-formation during mass extinction. An analysis of Rare Earth Elements (REEs) was conducted on the C1 coal from the Yantang Mine of Xuanwei, eastern Yunnan Province, which was deposited during the latest Permian. A total of 24 samples from coals, partings, roofs and floors from the C1 coal were taken from the fresh face in the underground mine. The results of the REEs analysis indicated that the total REE content (∑REE) in the C1 coal varies from 23.99 μg/g to 267.94 μg/g, averaged 122.69 μg/g. The C1 coal is enriched in light REE (LREE) relative to heavy REE (HREE), signifying the fractionation between LREE and HREE. Most samples of the coal seam C1 are depleted in Eu in various degrees and slightly depleted in Ce, especially two partings in sub-seams B1 and B3 which show the significant negative Eu anomalies. The geochemical characteristics of REE reveal that the C1 coal was deposited in a weak oxidation environment; the sedimentary environment was turbulent during the middle-later stage of coal-forming process; the C1 coal was affected by the basalt clastic materials from the Khangdian Oldland and acidic synsedimentary volcanic ash in the coal-forming period. The geochemical characteristics of Tonsteins in C1 coal are similar to those of marine Permian-Triassic boundary (PTB) volcanic ash layers in South China, which are both derived from the felsic volcanism caused by the closure of the Paleo-Tethys at the southwestern margin of the South China. Furthermore, the C1 coal was also affected by the eruption of Siberian large igneous province (SLIP) in the early stage.
1 Introduction
Rare Earth elements (REEs) present relatively stable chemical properties as well as a high degree of uniformity, and are easily preserved in a geologic body after sedimentation. The information about the sediment provenance that REEs carry is not easy to lose and is little affected by metamorphism. Therefore, REEs have been used as powerful geochemical indicators to reflect provenance compositions and changes, and provide geochemical evidences for the formation and evolution of coal seams (Wang et al., 2008; Xie et al., 2014; Arbuzov et al., 2018). At present, many studies on REEs in coals have been focusing on the characteristics of contents and the spatial distribution, occurrence, distribution pattern, provenance analysis and primary sedimentary environment of coals (Hao et al., 2004; Ren et al., 2006; Wu et al., 2013; Liu et al., 2015; Munir et al., 2018; Hedin et al., 2019; Ulrich et al., 2019). However, fewer studies have focused on the relationships between the geochemical characteristics of REEs and special geological events.
End-Permian time is one of the most important critical transition in geological history, and the largest mass extinction occurred at the Permian-Triassic transition (Wang et al., 2018; Guo et al., 2022). As the last coal deposited at the end Permian, the C1 coal recorded the terrestrial paleoenvironment changes during mass extinction (Wang et al., 2018), the geochemical characteristics of C1 coal may be closely related to the event. The geochemical characteristics of trace elements other than REEs have been studied by Shao et al. (2015), and they proposed that enriched elements mainly derived from continental weathering of the Emeishan basalt, synsedimentary volcanic ashes and medium-low temperature hydrothermal fluid. REEs, however, are more reliable tracers to reveal provenance and paleoenvironment, and can better constrain the environment and biological evolution events (Tian et al., 2014). In this paper, systematic REEs geochemical analyses of the latest Permian C1 coal in Xuanwei county have been conducted, combined with previous research results about major and trace elements in C1 coal to accurately trace the provenance change during the coal forming (Shao et al., 2015), reveal the sedimentary environment and tectonic background of coal forming, and discuss a series of geological events occurred on land during the mass extinction at the latest Permian, especially the effects of volcanic activities on coal-formation.
2 Geological setting
Xuanwei County is located in the eastern Yunnan Province and adjacent to the west Guizhou Province (Figure 1), which was tectonically located in the western part of the South China cratonic basin during the Late Permian (Shao et al., 2013). The siliciclastic sediments in this area were supplied predominantly by the Khangdian Oldland to the west. Meanwhile a long-term gradual easterly transgression throughout Late Permian provided sufficient accommodation space for the development of this important latest Permian coal province (Wang et al., 2011; Shao et al., 2013). The C1 coal is widely distributed in eastern Yunnan, mainly located in Xuanwei, Fuyuan and Weining areas, and lied in the uppermost portion of the Xuanwei Formation of Late Permian. The Xuanwei Formation unconformably underlaid by the Emeishan Basalt, and is conformably overlaid by the Kayitou Formation of Early Triassic (Figure 2). In the Xuanwei area, this formation consists mainly of grey fine-grained sandstone, siltstone and mudstone, some volcanic ash beds, and numerous coal seams including the C1 coal seam at its top (Wang et al., 2012). The Xuanwei Formation of Late Permian is mostly made of continental fluvial deposits (Wang et al., 2011).
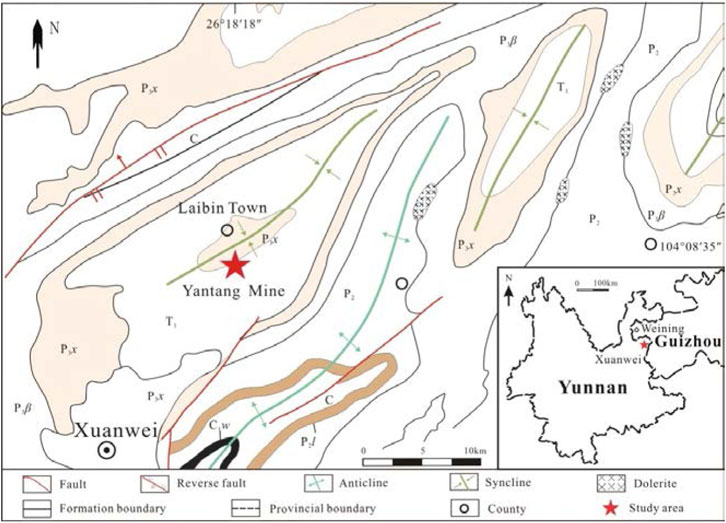
FIGURE 1. Location of the Yantang mine, Laibin Town, Xuanwei county, Yunnan Province (cited from Wang et al., 2018).
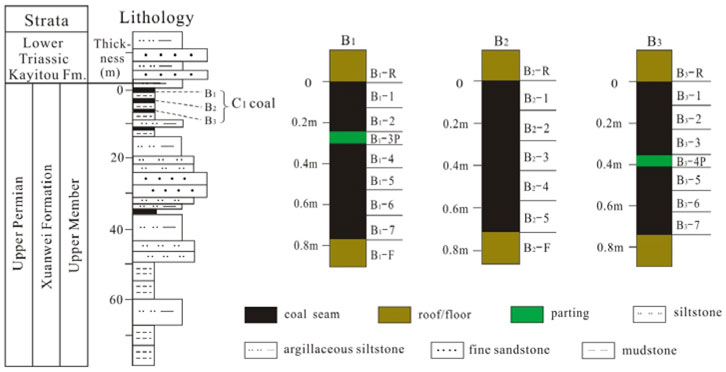
FIGURE 2. The stratigraphic section of mineable coal seam and sampling horizons of the Yantang Mine.
3 Sampling and experimental methods
3.1 Sample description and basic data
Samples were collected from the Yantang Mine of Laibin Town, Xuanwei County, and the coal seam C1 is the only minable seam in this mine which included three sub-seams B1, B2, and B3 in descending order. Within the sub-seam B1 and B3, a 5 cm-thick Tonstein (kaolinite claystone altered from volcanic ash) layer exists (Figure 2). The total of 24 collected coal-seam channel samples including coal, Tonstein, roof and floor, were taken from the fresh face of the underground mine. The sampling site was located at N 26°18′18″ and E 104°08′35″ and the samples were collected with a vertical spacing of about 10 cm. All samples collected were at least 1 kg in weight and were immediately stored in plastic bags to ensure as little contamination as possible. The method of sample collection followed the Chinese National Standard for Collecting Channel Samples GB/T 482-2008.
The partial proximate analysis, sulfur contents and vitrinite reflectance of C1 coal are presented in Table 1. The analytical data show that all samples are bituminous coal with a medium-high ash yield, ultralow total sulfur content and low-medium volatile content. According to coal ash classification (GB/T 15224.1–94), the coal samples B1-1 and B2-3 are classified as mixture of coal and mudstone since their ash yields exceeds 50%. Therefore, these are not included in the discussion of the C1 coal in the present paper.
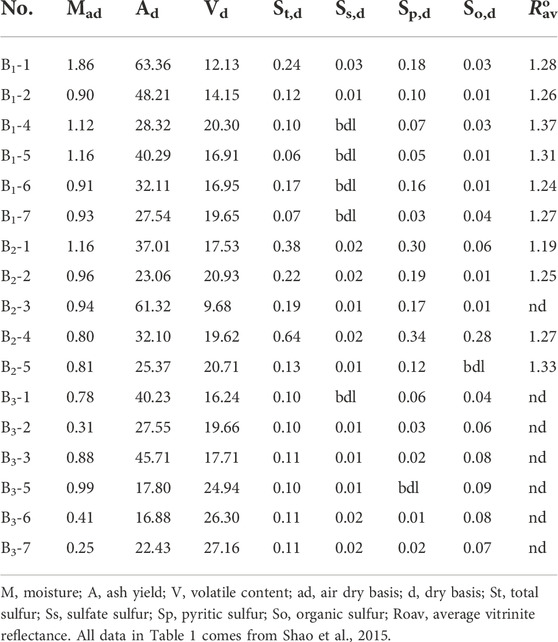
TABLE 1. Partial proximate analysis, sulfur contents and vitrinite reflectance in coals from the Yantang Mine in Xuanwei, Yunnan (%).
3.2 Analytical methods
The bulk samples were air-dried, crushed, ground to less than 200-mesh and dried for 2 h in a drying oven at 105°C∼110°C. The samples were then preprocessed by low-temperature airproof acid digestion, whose detailed procedure is described hereafter. An accurately weighed sample of 0.0500 g was mixed with 1 ml of HF, 3 ml of HNO3 and 1 ml of HClO4 in a dedicated 25 ml digestion vessel. After the cap assembles were sealed, the vessel was put into a low-temperature electric warming plate for heating at 125°C for 24 h. Finally, the residue was dissolved with 1% HNO3 and then transferred to a 50 ml volumetric flask. The prepared samples were then determined for their REE compositions using the inductively coupled-plasma mass spectrometry (ICP-MS) (Finnigan MAT), with the relative analysis error of ±5%, according to the “GB/T 14506.30–2010”. The REEs testing was performed at the Analytical Laboratory of Beijing Research Institute of Uranium Geology. The final results were reported as the µg/g in coal with a dry basis for each sample.
4 Results
The contents of REEs and other trace elements in the C1 coal obtained by ICP-MS analysis are presented in Tables 2, 3. The total REE content (∑REE) in C1 coals (excluding roof, floor and Tonstein) varies from 23.99 μg/g to 267.94 μg/g, averaged 122.69 μg/g, which is slightly lower than that of average Chinese coal with 135.89 μg/g (Dai et al., 2012). Compared with the Late Permian coal in the adjacent area, the average ∑REE in C1 coal is slightly lower than that of Yueliangtian coals from Panxian county, western Guizhou (136 μg/g, marine and continental transitional environment, cited from Wang et al., 2017), but higher than that of continental coals from Shizong county and Fuyuan county in eastern Yunnan (101 μg/g and 105.9 μg/g, respectively, cited from Wang et al., 2017 and Wang et al., 2012). The ∑REE of the roof and floor strata ranges from 212.39 μg/g to 458.89 μg/g, averaged 326.01 μg/g, which is 2.65 times of that of coal, indicating that the total amount of REEs in mudstone is significantly higher than that of coal. However, there is not significant vertical variation of REE contents among benches of individual coal seam, as seen from Figure 3.
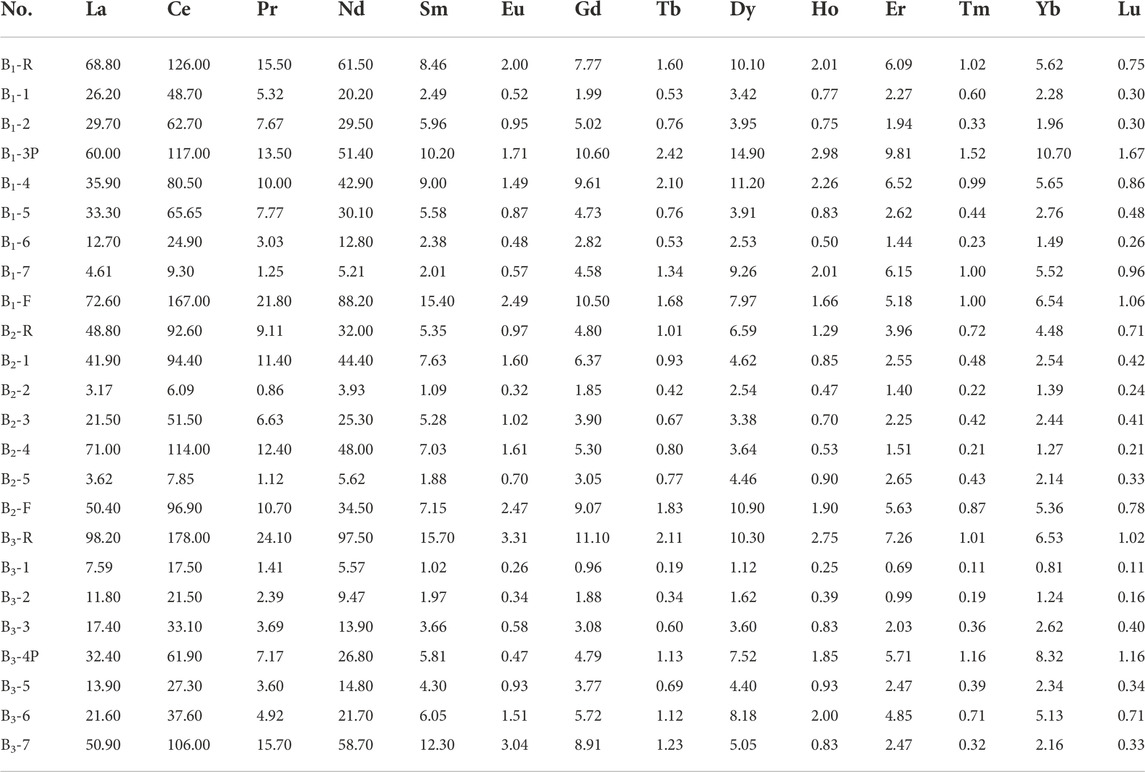
TABLE 2. REE contents of the late permian coal seam samples from the Yantang Mine, Xuanwei, Yunnan (µg/g).
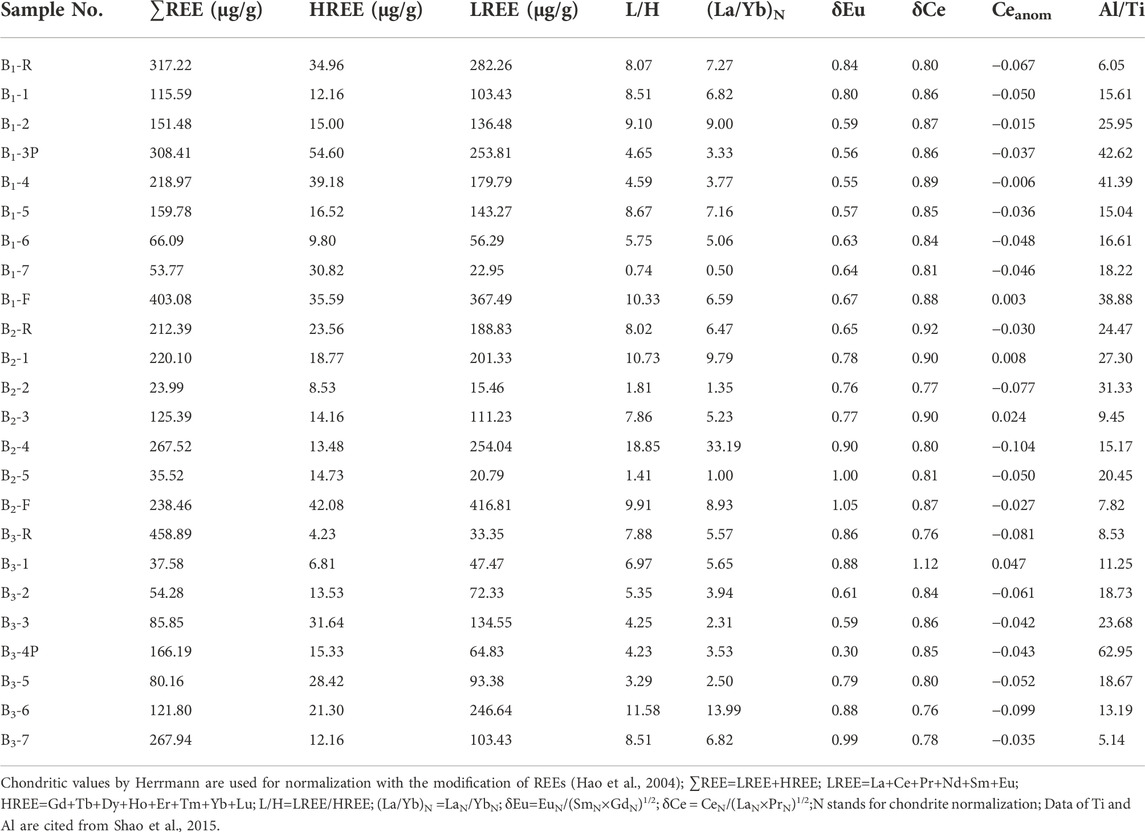
TABLE 3. The REE and elements data of the late permian coal seam samples from the Yantang Mine, Xuanwei, Yunnan.
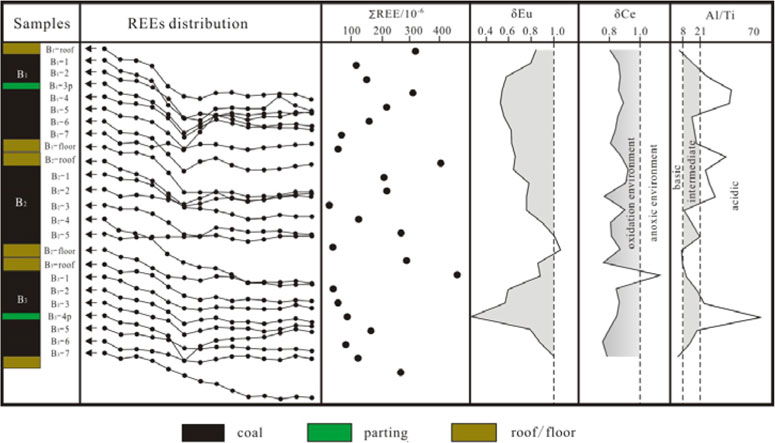
FIGURE 3. Stratigraphic distribution of patterns of ∑REE, δEu, δCe and Zr/Hf in all coal samples from Yantang Mine, Xuanwei, Yunnan.
The REEs contents of chondrites were taken from Herrmann (Hao et al., 2004) and the chondrite-normalized REE distribution patterns of all samples from the seam C1 are showed in Figure 3. All of the curves show a certain slope and are tilted to the right. The curves of Light REE (LREE) are slightly sloping compared with Heavy REE (HREE). The samples from seam B1 and B3 show a similar distribution trend respectively, while the curves of samples from seam B2 are haphazard. The (La/Yb)N ratios of all samples change from 0.50 to 33.19, with an average value of 6.73, indicating that LREE were significantly enriched relative to HREE. This is consistent with all other studies which reveal the predominance of the LREE relative to HREE.
Most samples of the C1 coal are depleted in Eu at various degrees, with δEu values ranging from 0.55 to 1.00 for an average of 0.74, and with a peak negative value (0.30) appearing in Tonstein B3-4. The δCe value of C1 coals show slightly negative or free anomalies, ranging from 0.76 to 1.12, averaged 0.85.
The REE patterns may be subsequently altered to a variable extent by post-depositional, diagenetic exchange or surface weathering (Zeng et al., 2001; Blake et al., 2017). As a special organic sedimentary rock, coal may also be affected by the above-mentioned factors during the coal-forming process, which could affect REEs fractionation and enrichment. For instance, δCe value easily changes due to diagenesis, which then leads to a significantly positive correlation between δCe and δEu, a significantly negative correlation between δCe and (Dy/Sm)N, and a significantly positive correlation between δCe and ∑REE (Shields et al., 2001; Zeng et al., 2011; Qu et al., 2018). To confirm the influence of diagenesis on samples from seam C1, the above three correlation coefficients are calculated as -0.10, 0.15 and 0.18, respectively (Figure 4). The correlation values are not significant, showing that diagenesis has very little influence on the Ce anomaly in the C1 coal. In consideration of this result, the Ce anomaly is regarded to reflect the characteristics of the terrestrial environment during sedimentary period as it represents the information of the original samples.
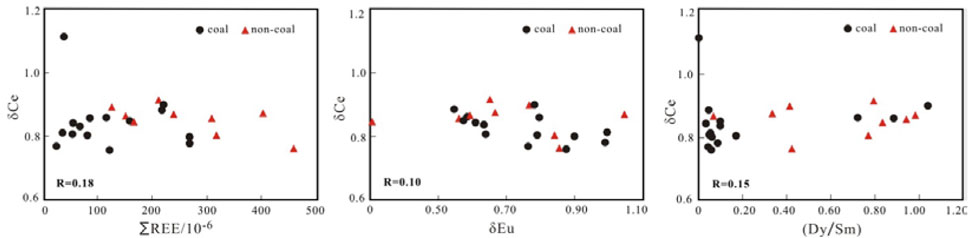
FIGURE 4. δCe correlate with δEu, (Dy/Sm)N and ∑REE in the C1 coal of Yantang Mine, Xuanwei, Yunnan.
5 Discussion
5.1 Sedimentary environment
Ce anomaly is an effective redox indicator, which can be used to analyze sedimentary environment and paleo-water medium conditions. The δCe greater than 1 indicates a reducing condition, while that less than 1 reflects a more oxidizing condition (Qu et al., 2018). In general, the negative Ce anomaly indicates the marine sedimentary environment, while the mafic basalt has slightly positive or free Ce anomaly (Zhao et al., 2019; Wang et al., 2020). The δCe of samples from the seam C1 are mostly between 0.76 and 0.92, averaged 0.84, indicating a slightly negative Ce anomaly (Table 3 and Figure 3), which is significantly different from the REE characteristics of seawater (Qu et al., 2018; Huang et al., 2019). Therefore, the influence of sea water can be excluded. The above discussion of δCe in samples from seam C1 suggested that the coal-forming peatland was affected by the input of basic basalt clastic materials from the Khangdian Oldland, and the coalforming peatland was in weak oxidation environment.
In addition, Ceanom is also widely used in the identification of paleoredox conditions. The Ceanom is greater than 0 indicates water hypoxia, while that less than 0 reflects oxidized water (Tian et al., 2018). Most Ceanom of samples from seam C1 are less than 0 (Table 2), indicating the weak oxidation environment of coal-forming peatland. Only a few samples in seam B2 have Ceanom greater than 0, indicating that peatland oxidation and reduction environments alternate during coalforming, suggesting a turbulent coal-forming environment.
5.2 The character of volcanic ash
Eu anomaly is inherited from the source rock, and as such Eu is generally recognized as an indicator of the nature of provenances. Basalt shows mostly no negative Eu anomaly, while granite and other acidic rocks present obvious negative Eu anomaly (Chen et al., 1990; Wang et al., 2019). Since the main provenance area of the coal-bearing basin in the study area is basalt (and its weathering and alteration products) originating from Khangdian Oldland, there should be no Eu anomaly in the C1 coal samples. However, most coal bench samples of the C1 seam are depleted in Eu at various degrees (Figure 3 and Table 2), except those at the roofs and floors and their vicinity. According to the sedimentary sequence of the C1 coal, the bottom coal of seam B3 that was first deposited has no Eu anomaly, and then the degree of negative Eu anomaly increases gradually upward, and reaches the maximum value of the whole coal seam rapidly at Tonstein B3-4 (δEu is 0.30), and then decreases rapidly upward. There was no Eu anomaly in the floor and bottom coal of the sub-seam B2 which deposited followed by the sub-seam B1, and the degree of negative Eu anomaly increased slowly from the bottom of sub-seam B2 upward to sub-seam B1 (B1-4) and Tonstein (B1-3), reaching the maximum Eu negative anomaly, and then the Eu negative anomaly decreased rapidly. The Eu negative anomaly degree of Tonstein B1-3 is significantly lower than that of B3-4, which may be caused by the input of acidic volcanic materials, and acidic volcanic activities are more intense during the B3 coal-forming period.
The Al in normal igneous rocks resides mostly in feldspars and the Ti in mafic minerals. Therefore, the Al/Ti ratio is an important parameter to determine the parent rock composition. Generally, basic rock with a Al/Ti ratio of 3–8 and intermediate rock with a Al/Ti ratio of 8–21, 21–70 indicate acidic rock (Hayashi et al., 1997). The Al/Ti ratio of Tonstein B1-3 and B3-4 is 42.62 and 62.95, respectively, whereas that of most coals is between 9.45 and 41.39 (Table 3). Integration of δEu and Al/Ti ratios suggest that there were twice significantly acidic volcanic activities during coal-forming (Figure 7A).
Tonstein is the preserved “relic” of volcanic activity, the δEu with 0.56 and 0.30 of Tonstein B1-3 and B3-4 are very close to that of five typical PTB ash beds in South China (He et al., 2014; Tan et al., 2019), so it is inferred that the two volcanic activities are the same provenance with those indicated by the PTB volcanic ash layer in South China. Furthermore, the similar shaped curves and negative Eu anomaly indicate that the Tonsteins and ash beds have the same REE origin from volcanic rocks related to plate subduction (Siberian Traps) (Widiatama et al., 2021) (Figure 5).
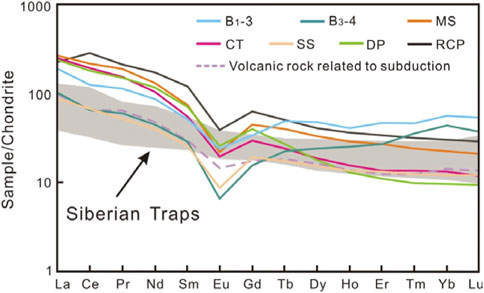
FIGURE 5. Chondrite-normalized REE patterns of the tonsteins in the C1 coal, PTB volcanic ashes and subduction-related rock.
5.3 Source and tectonic background of the C1 coal
REEs have special geochemical characteristics, and are stable during weathering, erosion, transportation, deposition and early diagenesis. Therefore, the REEs have been used to infer the material source of sediments (Bai et al., 2015; Tian et al., 2018; Qu et al., 2018). The different chondrite-normalized patterns of sub-seam B1, B2 and B3 (Figure 3) show different coal-forming process. With few exceptions, the distribution curves of REEs in the first deposited sub-seam B3 are most similar, indicating the stable and single provenance. The distribution curves and abundance of REEs in samples from the sub-seam B2 have more variability than sub-seam B3. In addition, Ceanom of samples reflected that the oxidation and reduction environment appeared alternately during coal-forming of sub-seam B2. All of the above results indicate that the sedimentary environment of peat swamp was relatively turbulent and the provenance was unstable during this period. The distribution curves of REEs in the latest sub-seam B1 are slightly different. Besides, the abundance of ∑REE in top coal suddenly decreases, inferred that the input of terrigenous substances replaces the paleoplant, and finally leads to the termination of coal-forming process.
Diagrams of w(La)/w(Th)—w(Hf) is commonly used to discriminate the source rocks and tectonic setting (Bai et al., 2015; Qu et al., 2018). The result shows that coals from the seam C1 are from multiple sources, such as tholeiite, andesite island arc, felsic and basic rocks (Figure 6). Tonsteins, seam roofs and floors are plotted mainly into the felsic and basic rocks area (Figure 6). All samples from the seam C1 are deposited in the passive continental margin.
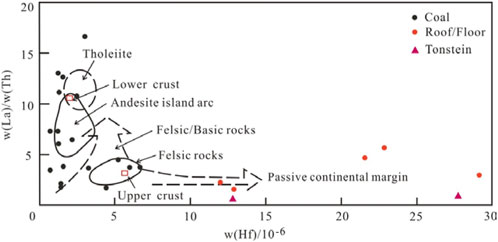
FIGURE 6. w(La)/w(Th)—w(Hf) discrimination diagram for provenance attribute Data of Zr and Hf are cited from Shao et al. (2015).
Tonsteins in the seam C1 classify as acidic rock on the Al2O3 versus TiO2 and diagram of Nb/Y—Zr/TiO2 (Figures 7A,B), and Tonsteins originate from felsic magmatic arc.
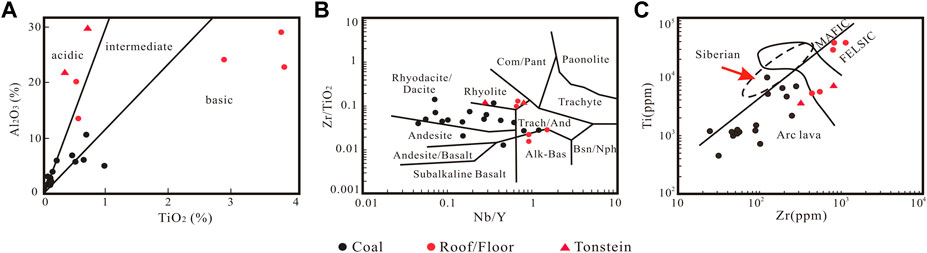
FIGURE 7. (A) Zr versus Ti diagram (modified from He et al., 2014), (B) plots of mass fractions of Al2O3 versus TiO2, and (C) Zr/TiO2 versus Nb/Y diagram (modified from He et al., 2014).
Integration of dispersion of coal samples plotted in above diagrams and variation of distribution curves of REEs, it can be inferred that the provenance of C1 coal is complex and influenced by the input of basalt and acidic volcanic ash during coal-forming process (Figures 3, 6, 7). Concretely, the roofs and floors originated from the basalt clastic materials of the KhangDian Oldland, while the Tonstein layer is derived from the acidic synsedimentary volcanic ash. Based on the dual influence of volcanism and basic basalt clastic material, the source rocks of the coal seam C1 show basic, intermediate and acidic (Figure 7A).
5.4 The relationship between coal formation and volcanic activity
During the Permian-Triassic, the Paleo-Tethys along a subduction zone between the South China and Indochina cratons closed gradually, and Indochina Plate moved rapidly northward and collided with other plates, consequently felsic volcanic activities were extraordinarily active(Cawood, 2005; Wang et al., 2019; Zhao et al., 2019) (Figure 8A). During one eruption, dust nanoparticles can travel thousands of kilometers through the stratosphere and affect all areas of the Earth for years (Ermolin et al., 2018). The volcanic ash falling to South China was altered in different sedimentary environments to form kaolinite, illite or illite/smectite, such as Tonsteins interbedded in C1 coal from Xuanwei area and five typical marine PTB sections in South China (Figures 8B,C) (He et al., 2014; Wang et al., 2018). Multiple volcanic claystone beds are widely developed in South China, which become more frequent and thicker towards southwest margin of Yangtze, indicating that the source of PTB tuff and volcanic claystone is in the southwest margin of the Yangtze Plate (Sun et al., 2013; Wang et al., 2022). Recently, previous studies on the source of PTB volcanic ash in South China have shown that, the PTB volcanism occurred near southwest South China and their adjacent convergent continental margin due to the closure of Paleo-Tethys. Furthermore, volcanic ash derived from subduction-zone arc between South China and Indochina cratons, which is not the trigger of PTB mass extinction due to limited eruption (Isozaki et al., 2007; Zhao et al., 2013; He et al., 2014; Wang et al., 2018; Wang et al., 2019; Zhao et al., 2019; Hou et al., 2020).
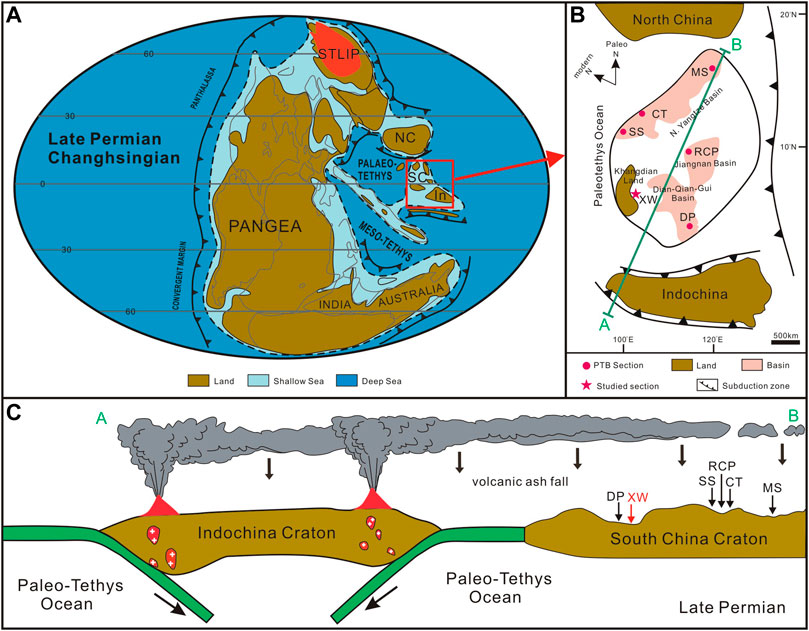
FIGURE 8. (A) Global paleogeography at late permian (Changhsingian) (modified from Metcalfe, 2013; Wang et al., 2019); (B) Potential volcanic ash source areas and inferred direction of ash transport (A→B) in South China and Indochina area during late permian (modified from Wang et al., et al., 2018; Zhao et al., 2019); (C) Composite cross-section of Late Permian South China and Indochina Craton, showing subduction of the Paleo-Tethys Plate beneath the Indochina Craton margin (modified from Wang et al., 2018; Zhao et al., 2019). Abbreviations in A: STLIP-Siberian Traps Large Province; NC-North China; SC-South China; In-Indochina Craton; B: DP-Dongpan, XW-Xuanwei, SS-Shangsi, RCP-Rencunping, CT-Chaotian, MS-Meishan.
The age of Tonstein (252.0 ±2.3 Ma, Wang et al., 2018) of the sub-seam B1 overlaps with age of granite (235–252 Ma, Ma et al., 2019) as evidence of subduction-zone, and Xuanwei area is immediately adjacent to the continental magmatic arc of the southwest margin of the South China at the End-Permian (Wang et al., 2018). By comparing REE patterns of two Tonsteins, five typical PTB volcanic ash layers, volcanic rocks related to subduction and Siberian traps (Figure 5) (He et al., 2014; Liu et al., 2015), it is found that the distribution curves of the first three are very similar, except the last one, mainly due to the absence of negative Eu anomaly. In addition, the REE patterns of Tonsteins are slightly different from those of PTB volcanic ash layers, such as little fractionation of LREE and HREE and flatter distribution curve, which may be caused by the volcanic ash falling in different sedimentary environments. Tonsteins were deposited in continental fluvial sedimentary environment, and the input of terrigenous materials tends to flatten the distribution curves of REE, which is different from the marine PTB volcanic ashes (Liu et al., 2018). The diagrams of Al2O3-TiO2, Nb/Y-Zr/TiO2 and Zr-Ti (Figure 7) also confirmed that the two layers of Tonstein derived from volcanic magmatic arc and were acidic, which was different from the basic SLIP (Kamo et al., 2003). Therefore, it was inferred that Tonsteins in the C1 coal were from felsic volcanism of the End-Permian, rather than SLIP.
Significantly, the bottommost coal sample B3-7, which has been plotted in the SLIP area (Figure 7C), and its δEu of 0.99, Al/Ti of 5.14, REE pattern curve and diagram of w(La)/w(Th)-w(Hf) and Zr-Ti, indicating that it has been affected by basic basalts. It is further confirmed that C1 coal was influenced by SLIP in its early stage. Compared with Indochina craton, SLIP and eastern Yunnan Province are more far apart. However, the SLIP is known as the largest continental volcanic event since Phanerozoic, and volcanic ash nanoparticles can transport to worldwide. With the overlap between constraint on SLIP eruption and C1 coal forming, therefore it can be inferred that the C1 coal was affected by SLIP in the early stage of coal forming.
6 Conclusion
The C1 coal from Xuanwei County, Yunnan Province contains two layers of Tonstein. REEs, several major and trace elements data indicate the following:
(1) The total REE content of the C1 coal ranges from 23.99 to 267.94 μg/g, averaged 122.69 μg/g. C1 coal samples are enriched in LREE relative to HREE and show significant fractionation between LREE and HREE. Most samples including coals, Tonsteins, roofs and floors present varying degrees of negative Eu anomaly, especially two Tonsteins in sub-seam B1 and B3 with the significant negative Eu anomalies. Most samples of the seam C1 are slightly depleted in Ce.
(2) The C1 coal-forming peatland was affected by the input of basic basalt clastic materials from Khangdian Oldland and acidic volcanic ash. The C1 coal was deposited in a weak oxidation environment, and experienced turbulent environment during the middle and later stage of coal-forming period.
(3) The geochemical characteristics of Tonsteins in the C1 coal are similar to those of the marine PTB volcanic ash layers in South China, which are both derived from the felsic volcanism caused by the closure of the Paleo-Tethys at the southwestern margin of the South China. Furthermore, the C1 coal was also affected by the eruption of SLIP in the early stage.
Data availability statement
The original contributions presented in the study are included in the article/supplementary material, further inquiries can be directed to the corresponding author.
Author contributions
JW searched the literature; JW and LS analyzed the data and wrote the paper; ZY and XW collected samples and performed the experiments.
Funding
This work is supported by the National Natural Science Foundation of China (No. 41602123 and 41572090), and the Fundamental Research Funds for the Universities of Henan Province (No. NSFRF220401).
Conflict of interest
The authors declare that the research was conducted in the absence of any commercial or financial relationships that could be construed as a potential conflict of interest.
Publisher’s note
All claims expressed in this article are solely those of the authors and do not necessarily represent those of their affiliated organizations, or those of the publisher, the editors and the reviewers. Any product that may be evaluated in this article, or claim that may be made by its manufacturer, is not guaranteed or endorsed by the publisher.
References
Arbuzov, S. I., Maslov, S. G., Finkelman, R. B., Mezhibor, A. M., Ilenok, S. S., Blokhin, M. G., et al. (2018), Modes of occurrence of rare Earth elements in peat from Western Siberia Rare Earth and major element geochemistry of Eocene fine-grained sediments in oil shale- and coal-bearing layers of the Meihe Basin, Northeast China. J. Asian Earth Sci. of Geochemical Exploration 184, 4089–48101 97
Blake, J. M., Peters, S. C., and Johannesson, K. H. (2017). Application of REE geochemical signatures for mesozoic sedimentprovenance to the gettysburg basin, Pennsylvania. Sediment. Geol. 349, 103–111. doi:10.1016/j.sedgeo.2016.12.009
Cawood, P. A. (2005). Terra australis orogen: Rodinia breakup and development of the pacific and iapetus margins of gondwana during the neoproterozoic and paleozoic. Earth. Sci. Rev. 69, 249–279. doi:10.1016/j.earscirev.2004.09.001
Chen, D. Q., and Chen, G. (1990). Practical geochemistry of rare earth elements. Beijing: Metallurgical Industry Press, 228–230. (in Chinese).
Dai, S. F., Ren, D. Y., Chou, C. L., Finkelman, R. B., Seredin, V. V., and Zhou, Y. P. (2012). Geochemistry of trace elements in Chinese coals: A review of abundances, genetic types, impacts on human health, and industrial utilization. Int. J. Coal Geol. 94 (3), 3–21. doi:10.1016/j.coal.2011.02.003
Ermolin, M. S., Fedotov, P. S., Malik, N. A., and Karandashev, V. K. (2018). Nanoparticles of volcanic ash as a carrier for toxic elements on the global scale. Chemosphere 200, 16–22. doi:10.1016/j.chemosphere.2018.02.089
Guo, B. Q., Gao, D., Guo, H. L., Liu, J. G., Wang, J. H., and Shi, Y. (2022). Geochemistry of the Permian–Triassic boundary strata in the southwest margin of the North China Craton: Provenance and tectonic implications. Geol. J.,23 1–14. doi:10.1002/gj.4558
Hao, L. B., and Qi, C. M. (2004). Principles of geochemistry, 2. Beijing: Geological Press, 54–55. (in Chinese)., pp.
Hayashi, K. I., Fujisawa, H., Holland, H. D., and Ohmoto, H. (1997). Geochemistry of ∼1.9 Ga sedimentary rocks from northeastern Labrador, Canada. Geochimica Cosmochimica Acta 61 (19), 4115–4137. doi:10.1016/s0016-7037(97)00214-7
He, B., Zhong, Y. T., Xu, Y. G., and Li, H. X. (2014). Triggers of permo-triassic boundary mass extinction in South China: The siberian traps or paleo-tethys ignimbrite flare-up? Lithos 204, 258–267. doi:10.1016/j.lithos.2014.05.011
Hedin, B. C., Capo, R., Stewart, B. W., Hedin, R. S., Lopano, C. L., and Stuckman, M. Y. (2019). The evaluation of critical rare Earth element (REE) enriched treatment solids from coal mine drainage passive treatment systems. Int. J. Coal Geol. 208, 54–64. doi:10.1016/j.coal.2019.04.007
Hou, H. H., Shao, L. Y., Tang, Y., Li, Y. N., Liang, G. D., Xin, Y. L., et al. (2020). Coal seam correlation in terrestrial basins by sequence stratigraphy and its implications for palaeoclimate and palaeoenvironment evolution. J. Earth Sci., 1–24.
Huang, Q. H., Bai, X. F., Wang, H., Cheng, H. G., and Zhu, Z. Y. (2019). Geochemical characteristics and geological significance of trace elements and rare Earth elements in carbonate rocks at Middle-Upper Permian boundary in Linxi area, Inner Mongolia. Glob. Geol. 38 (3), 611–622.
Isozaki, Y., Shimizu, N., Yao, J. X., Ji, Z. S., and Matsuda, T. (2007). End-Permian extinction and volcanism-induced environmental stress: The Permian-Triassic boundary interval of lower-slope facies at Chaotian, South China. Palaeogeogr. Palaeoclimatol. Palaeoecol. 252, 218–238. doi:10.1016/j.palaeo.2006.11.051
Kamo, S. L., Czamanske, G. K., Amelin, Y., Fedorenko, V. A., Davis, D. W., and Trofimov, V. R. (2003). Rapid eruption of Siberian flood-volcanic rocks and evidence for coincidence with the Permian-Triassic boundary and mass extinction at 251 Ma. Earth Planet. Sci. Lett. 214, 75–91. doi:10.1016/s0012-821x(03)00347-9
Liu, D. N., Zhou, A. C., and Chang, Z. G. (2015). Geochemistry characteristics of major and rare Earth elements in No.8 raw and weathered coal from Taiyuan Formation of Datong coalfield. J. China Coal Soc. 40 (2), 422–430. (in Chines, with English abstract).
Liu, Q. Q., Chi, Q. H., Wang, X. Q., Zhou, J., Liu, H. L., Liu, D. S., et al. (2018). Distribution and influencing factors of rare Earth elements in carbonate rocks along three continental-scale transects in eastern China. Earth Sci. Front. 25 (4), 99–115. (in Chines, with English abstract).
Ma, X. H., Chen, C. J., Zhao, J. X., Qiao, S. L., and Zhou, Z. H. (2019). Late permian intermediate and felsic intrusions in the eastern central asian orogenic belt: Final-stage magmatic record of paleo-asian oceanic subduction? Lithos 326-327, 265–278. doi:10.1016/j.lithos.2018.12.022
Metcalfe, I. (2013). Gondwana dispersion and Asian accretion: Tectonic and palaeogeographic evolution of eastern Tethys. J. Asian Earth Sci. 66, 1–33. doi:10.1016/j.jseaes.2012.12.020
Munir, M. A. M., Liu, G. J., Yousaf, B., Ali, M. U., Abbas, Q., and Ullah, H. (2018). Enrichment of Bi-Be-Mo-Cd-Pb-Nb-Ga, REEs and Y in the permian coals of the huainan coalfield, anhui, China. Ore Geol. Rev. 95, 431–455. doi:10.1016/j.oregeorev.2018.02.037
Qu, X. R., Li, J., Sun, C. R., Zhang, Q. H., Tang, S. H., and Wei, J. G. (2018). Geochemistry characteristics of rare Earth elements in the late Paleozoic black shale from eastern Ordos Basin. J. China Coal Soc. 43 (4), 1083–1093. (in Chinese, with English abstract).
Ren, D. Y., Zhao, F. H., Dai, S. F., Zhang, J. Y., and Luo, K. L. (2006). Trace element geochemistry of coal. Beijing: Science Press, 321–334. (in Chinese).
Shao, L. Y., Gao, C. X., Zhang, C., Wang, H., Guo, L. J., and Gao, C. H. (2013). Sequence-palaeogeography and coal accumulation of late permian in southwestern China [J]. Acta sedimentol. sin. 31 (5), 856–866. (in Chinese, with English abstract).
Shao, L. Y., Wang, J., Hou, H. H., Zhang, M. Q., Wang, H., Spiro, B., et al. (2015). Geochemistry of the C1 coal of latest permian during mass extinction in Xuanwei, yunnan. Acta Geol. sin. 89 (1), 163–179. (in Chinese, with English abstract).
Shields, G., and Stille, P. (2001). Diagenetic constraints on the use of cerium anomalies as palaeoseawater redox proxies: An isotopic and REE study of Cambrian phosphorites. Chem. Geol. 175 (1-2), 29–48. doi:10.1016/s0009-2541(00)00362-4
Sun, Y. D. (2013). Coupling climatic and biodiversity changes with volcanisms during the Palaeozoic-Mesozoic upheavals in South China. Wuhan: China University of Geosciences PhD thesis. (in Chinese with English abstract).
Tan, C., Yuan, X. J., Yu, B. S., Liu, C., Li, W., and Cui, J. W. (2019). Geochemical characteristics and paleoclimatic implications of the upper permian and middle-lower triassic strata in southern ordos basin. GEOSCIENCE 33 (3), 615–628. (in Chinese with English abstract).
Tian, X. L., Luo, K. L., Wang, S. B., and Ni, R. X. (2014). Geochemical characteristics of trace elements and rare Earth elements during the Cryogenian-Ediacaran transition in Yangtze Gorges area. J. Palaeogeogr. 16 (4), 483–502. (in Chinese, with English abstract).
Ulrich, M., Cathelineau, M., Munoz, M., Boiron, M. C., Teitler, Y., and Karpoff, A. M. (2019). The relative distribution of critical (Sc, REE) and transition metals (Ni, Co, Cr, Mn, V) in some Ni-laterite deposits of New Caledonia. J. Geochem. Explor. 197, 93–113. doi:10.1016/j.gexplo.2018.11.017
Wang, H., Shao, L. Y., Hao, L. M., Zhang, P. F., Glasspool, I. J., Wheeley, J. R., et al. (2011). Sedimentology and sequence stratigraphy of the Lopingian (Late Permian) coal measures in southwestern China. Int. J. Coal Geol. 85, 168–183. doi:10.1016/j.coal.2010.11.003
Wang, H., Shao, L. Y., Newton, R. J., Bottrell, S. H., Wignall, P. B., and Large, D. J. (2012). Records of terrestrial sulfur deposition from the latest Permian coals in SW China. Chem. Geol. 292-293, 18–24. doi:10.1016/j.chemgeo.2011.11.005
Wang, J., Shao, L. Y., Wang, H., Spiro, B., and Large, D. (2018). SHRIMP zircon U-Pb ages from coal beds across the Permian-Triassic boundary, eastern Yunnan, southwestern China. J. Palaeogeogr. 7 (2), 117–129. doi:10.1016/j.jop.2018.01.002
Wang, P. P. (2017). Enrichment and differentiation mechanism of minerals and trace elements in the Late Permian coals from eastern Yunnan and western Guizhou Province. Beijing: China University of Mining and Technology PhD thesis. (in Chinese, with English abstract).
Wang, Q., and Yang, R. D. (2008). Tracing study on REEs of coal measure in late permian in bijie city, Guizhou province, China. J. Chin. rare earth Soc. 26 (1), 102–107. (in Chinese, with English abstract).
Wang, T., Zhu, X. M., Dong, Y. L., Chen, H. H., Su, B., Liu, Y., et al. (2020). Trace elements as paleo sedimentary environment indicators: A case study of the paleogene anjihaihe formation in the northwestern junggar basin. Acta Geol. Sin. 94 (12), 3830–3851. (in Chinese with English abstract).
Wang, X. B., Dai, S. F., Chou, C. L., Zhang, M. Q., Wang, J. M., Song, X. L., et al. (2012). Mineralogy and geochemistry of late permian coals from the taoshuping mine, yunnan province, China: Evidences for the sources of minerals. Int. J. Coal Geol. 96-97, 49–59. doi:10.1016/j.coal.2012.03.004
Wang, X. D., Cawood, P. A., Zhao, L. S., Chen, Z. Q., Lyu, Z. Y., and Ma, B. (2019). Convergent continental margin volcanic source for ash beds at the Permian-Triassic boundary, South China: Constraints from trace elements and Hfisotopes. Palaeogeogr. Palaeoclimatol. Palaeoecol. 519, 154–165. doi:10.1016/j.palaeo.2018.02.011
Wang, Y., Cao, J., Zhang, B. L., Liao, Z. W., Zhang, B., Liu, J. C., et al. (2022). Genesis of the wangpo bed in the sichuan basin: Formation by eruptions of the emeishan large igneous province. Palaeogeogr. Palaeoclimatol. Palaeoecol. 2022 (594), 110935. doi:10.1016/j.palaeo.2022.110935
Widiatama, A. J., Natalia, H. C., Ikhram, R., Santy, L. D., Wahyudiono, J., Wiguna, L. R. S., et al. (2021). Pedaro formation, equivalent of plover sandstone at savu island, outer banda arc. IOP Conf. Ser. Earth Environ. Sci. 882 (1), 012052–012044. doi:10.1088/1755-1315/851/1/012052
Wu, D., Sun, R. Y., and Liu, G. J. (2013). Characterization of REE geochemistry of the permian coals from the zhuji coal mine. Huainan Coalf. its Geol. Implic. Acta Geol. sinica 87 (8), 1158–1166. in Chinese, with English abstract.
Xie, Y. Y., Meng, J., and Guo, L. F. (2014). REE geochemistry of modern eolian dust deposits in Harbin city, Heilongjiang province, China: Implications for provenance. Catena 123, 70–78. doi:10.1016/j.catena.2014.07.008
Zeng, J. W., Xu, R., and Gong, Y. M. (2011). Hydrothermal activities and seawater acidification in the Late Devonian F-F transition: Evidence from geochemistry of rare Earth elements. Sci. China Earth Sci. 54, 540–549. (in Chinese, with English abstract). doi:10.1007/s11430-011-4171-8
Zhao, L. S., Chen, Z. Q., Algeo, T. J., Chen, J. B., Chen, Y. L., Tong, J. N., et al. (2013). Rare Earth element patterns in conodont albid crowns: Evidence for massive inputs of volcanic ash during the latest Permian biocrisis? Glob. Planet. Change 105, 135–151. doi:10.1016/j.gloplacha.2012.09.001
Zhao, T. Y., Algeo, T. J., Feng, Q. L., Zi, J. W., and Xu, G. Z. (2019). Tracing the provenance of volcanic ash in Permian-Triassic boundary strata, South China: Constraints from inherited and syn-depositional magmatic zircons. Palaeogeogr. Palaeoclimatol. Palaeoecol. 516, 190–202. doi:10.1016/j.palaeo.2018.12.002
Keywords: the latest Permian, rare Earth elements, coal-forming environment, volcanism, eastern Yunnan
Citation: Wang J, Shao L, Yan Z and Wang X (2023) The coal-forming environment during mass extinction in the latest permian: Evidence from geochemistry of rare Earth elements. Front. Earth Sci. 10:1057831. doi: 10.3389/feart.2022.1057831
Received: 30 September 2022; Accepted: 31 October 2022;
Published: 18 January 2023.
Edited by:
Mingjun Zou, North China University of Water Resources and Electric Power, ChinaReviewed by:
Haihai Hou, Liaoning Technical University, ChinaJun Shen, China University of Geosciences Wuhan, China
Dongdong Wang, Shandong University of Science and Technology, China
Copyright © 2023 Wang, Shao, Yan and Wang. This is an open-access article distributed under the terms of the Creative Commons Attribution License (CC BY). The use, distribution or reproduction in other forums is permitted, provided the original author(s) and the copyright owner(s) are credited and that the original publication in this journal is cited, in accordance with accepted academic practice. No use, distribution or reproduction is permitted which does not comply with these terms.
*Correspondence: Juan Wang, NjY2NzY4NzJAcXEuY29t