- 1School of Safety Science and Engineering, Henan Polytechnic University, Jiaozuo, Henan, China
- 2State Key Laboratory Cultivation Base for Gas Geology and Gas Control of Henan Province, Jiaozuo, Henan, China
- 3School of Resources and Geoscience, China University of Mining and Technology, Xuzhou, Jiangsu, China
Gas is one of the necessary contributing factors for coal and gas outburst accidents, and the gas desorbed in coal is the energy carrier in the outburst process. The study of gas desorption laws is the premise and basis for gas content determination and gas accident prevention. To solve the problem of inaccurate gas content measurement due to the unclear characteristics of rapid gas desorption in 0–10 s, the gas desorption experimental device was improved, the influence factors of gas desorption were studied experimentally, and a comprehensive analysis method was proposed based on the gas desorption rate, gas desorption efficiency, initial gas desorption amount and total desorbed gas. The experiment analysed five factors that affected gas desorption, including the degree of metamorphism, type of failure, particle size, pressure and temperature. The results show that there is a monotonically decreasing power function relationship between the initial gas desorption rate and time and a monotonically increasing logarithmic function relationship between the gas desorption amount and time; the curve has a limit value. The gas desorption amount is large in 0–10 s and increases slowly afterwards. Among the factors affecting gas desorption, their importance decreases in the order of pressure > metamorphism > particle size > failure type > temperature. This study is of great practical value for the calculation of gas losses in gas content determination, and the resulting gas desorption laws are of great importance in guiding gas control work.
1 Introduction
Excavation activities in coal mines cause the formation stress balance to be destroyed, thereby increasing the gas potential energy. Gas can be desorbed from the coal body and flooded into the mining tunnel in a very short period of time. The exposed coal is in a state of stress unloading. Under the dual action of in situ stress and gas, the coal is crumpled and damaged, resulting in the relaxation of pores and fissures. The diffusion resistance of free gas is reduced, and it continuously flows into the roadway, causing hidden dangers to production (Hao et al., 2000). The accurate determination of gas content is difficult but it is a crucial factor in coal mine production and is the premise of resource utilization and disaster prevention (Zhang, 2009; Wang et al., 2018; Wang et al., 2018b). To predict the risk of gas-related disasters, it is necessary to timely and accurately measure the initial gas release velocity and gas content.
The initial velocity of gas release (ΔP) is an important index for evaluating the risk of coal and gas outbursts in China (with ΔP ≥ 10 as the critical value). ΔP is an empirical value, and it is not accurate to use the same index under different metamorphism, pressure, temperature and other conditions (Zhang, 2006); this indicator represents the amount of gas emission in 10–60 s after atmospheric pressure adsorption but cannot reflect the instantaneous emission characteristics and actual attenuation changes of gas when different blocks are exposed. However, the instantaneous desorption and release of gas is the basic reason for the complex occurrence conditions and prominent dangers of coal gas (Fu and Yang, 2008). It is also the basis for coal seam gas content determination and outburst risk prediction (Jia and Chen, 2009; Jiang et al., 2009).
Therefore, the accurate determination of gas content and initial gas release velocity cannot be separated from the study of gas desorption laws. Previous researchers have performed much research on gas desorption, but there is a lack of research on gas release in the first 0–10 s and its influencing factors.
2 Experimental design
The desorption of gas in coal particles is a complex dynamic and hydrodynamic process. In the original state, the adsorbed gas, on the surface of the coal matrix or in the micropores, and the free gas in the fracture system are in a relative dynamic equilibrium. When the environmental factors around the tectonic coal change or under the action of vibration (Wang et al., 2021), the increased kinetic energy of the gas molecules is sufficient to overcome the gravitational effect of the adsorption potential well on the surface of the coal body; then, the adsorbed gas becomes free, and the adsorbed gas will be desorbed again.
The desorption of gas from coal is a complex process. First, the gas is desorbed from the adsorption state on the surface of the coal body into a free state (Wang et al., 2021b) and then desorbed from the coal body. To analyse the law of gas desorption under different conditions, based on the Langmuir adsorption model, the experimental equipment is improved, and the experimental conditions are established.
2.1 Experimental setup
The “Method for Determination of Methane Adsorption Capacity of Coal (High Pressure Volumetric Method)” (Ministry of coal industry of the people’s Republic of China, 1997) was adopted, and the experimental device was improved, which consisted of 7 units of aeration, air extraction, temperature control, adsorption, desorption, desorption rate measurement, and communication auxiliary (Table 1).
The principle of the test device is shown in Figure 1.
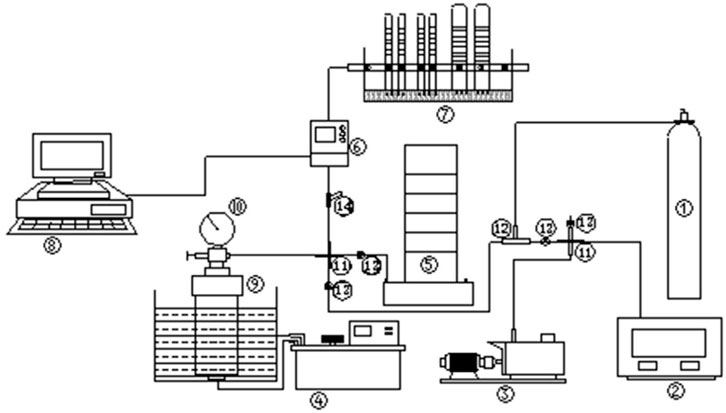
FIGURE 1. Principle picture of experimental device studying gas (CBM) desorption feature of tectonically coal.
2.2 Sample preparation
Coal samples were collected at the excavation face of the Changcun Mine in the Lu’an Mining Area and the Zouzhuang Mine in the Huaibei Mining Area. The coal was sampled from the newly disclosed coal, and its firmness coefficient and initial gas release velocity were determined. Later, it was transferred to the laboratory for sample preparation. A total of 30 coal samples with different degrees of metamorphism, failure types and particle sizes were prepared, the gas parameters were measured, and industrial analysis was performed. The characteristics of the experimental samples are shown in Table 2.
2.3 Experimental steps
2.3.1 Parameter design
A large number of studies have shown that gas desorption is controlled by the degree of coal metamorphism (Grażyna and Kinga, 1998; Zhang, 2018), the type of failure (Xie, 2014; Wang et al., 2020), particle size (Wang, 2018; Tu et al., 2022), equilibrium pressure (Li et al., 2018; Li et al., 2021), temperature (Liu, 2011; Li et al., 2022), moisture (Xiao, 2010; Zhang, 2011; Chen et al., 2013; Wang et al., 2018c), electromagnetic field (Xu et al., 2003; Li and Lei, 2012; Zhao and Deng, 2022) and so on.
Group 0 was selected as the control group, and 10 groups of experiments were designed with metamorphic degree, failure type, particle size, pressure and temperature as variables. To explore the influence of the metamorphic degree on gas desorption, gas coal with a low metamorphic degree was selected as Group 1. Geological structure is an important factor for the occurrence of coal and gas outbursts. Tectonic action will change the physical structure and chemical properties of coal, and the failure type of a coal body is an index used to measure the impact of tectonic action. Tectonic coal has the characteristics of low strength, poor cohesiveness, looseness and even pulverization after being damaged by extrusion deformation and shear crumpling. Therefore, structural coal from the same mining area is selected as Group 2 to study the influence of failure type on gas desorption. For primary structure coal, the smaller the particle size is, the larger the specific surface area. Group 3 is the experimental group of coal gas desorption with different particle sizes. According to the Langmuir adsorption model, pressure and temperature are important factors affecting the adsorption and desorption of gas on the coal surface. In China, the identification of coal and gas outbursts is based on the gas pressure (≤0.74 MPa), and the minimum experimental pressure is 0.5 MPa. Five groups of desorption experiments with different pressures are designed with an increasing interval of 0.5 MPa. According to the practice of production and the geothermal studies, the formation temperature of coal seams in China is mostly 21°C–37°C, so desorption experiments of 20°C, 30°C, and 40°C at different temperatures are designed. Due to the limitations of the experimental setup, influencing factors such as water content and the presence of an electromagnetic field were not tested. The experimental variables and parameters are shown in Table 3.
2.3.2 Operation steps
The experimental operation process is divided into 7 steps:
2.3.3 Experiment records and readings
1) 0–10 s, read the desorption amount every 1 s, counting 10 times;
2) 10–90 s, read the desorption amount every 2 s, counting 40 times in total;
3) 90–180 s, read the desorption amount every 5 s, counting 18 times in total;
4) 180–600 s, read the desorption amount every 10 s, counting 42 times in total;
5) 10–30 min, read the gas desorption amount every 1 min, counting 20 times;
6) 30–120 min, read the gas desorption amount every 5 min, counting 18 times in total;
7) 120–360 min, read the desorption amount every 10 min, counting 24 times in total;
8) After 6 h, read the gas desorption amount every 1 h until the reading no longer changes.
3 Experimental results and discussion
3.1 Experimental results
The results of 10 groups of gas desorption experiments under different conditions were counted, including the initial rate of gas desorption (V0), initial gas desorption capacity (T180), initial gas desorption efficiency (E180), total gas desorption (T480), and total gas desorption efficiency (E480). The ratio of gas desorption characteristic values of the experimental groups to that of control Group 0 was calculated under different experimental conditions (Table 4). Among them, desorption efficiency refers to the time required for gas desorption to reach 80% of the total amount of desorption.
3.1.1 Experimental results of group 0
The experimental conditions for Group 0: meager lean coal, primary structural coal (Type II), particle size 1.0–3.0 mm, pressure 1.00 MPa and temperature 30°C (Figure 2). Group 0 was selected as the control group, the gas desorption conditions under different experimental conditions were compared, and then the influence of various factors on the gas desorption rate and amount were analysed.
3.1.2 Experimental results of group 1
The experimental conditions for Group 1: gas coal, primary structural coal (Type II), particle size 1.0–3.0 mm, pressure 1.00 MPa and temperature 30°C (Figure 3).
3.1.3 Experimental results of group 2
The experimental conditions for Group 2: meagre lean coal, tectonic coal (Type IV), particle size 1.0–3.0 mm, pressure 1.00 MPa and temperature 30°C (Figure 4).
3.1.4 Experimental results of group 3
The experimental conditions for Group 3: meagre lean coal, primary structural coal (Type II), particle size 0.5–1.0 mm, pressure 1.00 MPa and temperature 30°C (Figure 5).
3.1.5 Experimental results of groups 4, 5, 6, and 7
To explore the influence of pressure on the gas desorption law, experimental Groups 4, 5, 6, and 7 were designed to conduct a comparative analysis of gas desorption under experimental pressures of 0.50, 1.50, 2.00 and 2.50 MPa, respectively, with meagre lean coal, primary structural coal, and particle sizes of 1.0–3.0 mm at 30°C. The results of the experiment are shown in Figure 6.
3.1.6 Experimental results of groups 8 and 9
To explore the influence of temperature on the gas desorption law, experimental Groups 8 and 9 were designed to conduct a comparative analysis of gas desorption at 20°C and 40°C, respectively, under the conditions of meagre lean coal, primary structural coal, and particle sizes of 1.0–3.0 mm with a pressure of 1.0 MPa. The results of the experiment are shown in Figure 7.
3.2 Discussion of the influencing factors of gas desorption
The rate of gas desorption in 90 s, the amount of gas desorption in 180 s and the total amount of gas desorption in 480 min were analysed through 10 groups of experimental studies on gas desorption under different conditions. The function curve and correlation coefficient of the experimental results are shown in Table 5.
3.2.1 Influence of the metamorphism degree on gas desorption
Studies have shown that the effect of the degree of metamorphism on the gas desorption rate is mainly controlled by the pore and fracture system in the coal body (Li, 2011). The adsorption and desorption capacity of coal depends on the pore structure and specific surface area of coal.
Table 6 (Li and Si, 2004; Zhang et al., 2006; Tang and Wang, 2010) shows that with the increase in the degree of metamorphism, the micropores and small pores in the coal increase, the specific surface area increases, and the adsorption capacity increases (Zhang, 2006; Zhang, 2009).
1) According to Figure 3A, we find that as the degree of metamorphism increases, the initial gas desorption rate increases significantly. The initial gas desorption rate of lean coal is 1.91 times that of gas coal.
2) Comparing Figure 2B and Figure 3B, we can see that the difference in the gas desorption amount within 10 s is obvious. The gas desorption amount of meagre lean coal reaches more than 60%, while that of the gas coal is less than 40%. With the increase in the degree of metamorphism, the initial (180 s) gas desorption capacity increases significantly: T180-0 is 1.99 ml/g, T180-1 is 0.95 ml/g, and the initial gas desorption capacity of lean coal is 2.09 times that of gas coal.
3) Comparing Figure 2C and Figure 3C, it can be seen that the initial gas desorption efficiencies of coals with different metamorphic degrees are higher. With the prolongation of desorption time, the desorption rate becomes slow, and the total amount of desorption increases slowly. The total amount of gas desorption in 480 min is similar, approximately 6.0 ml/g.
4) In the early stage of gas desorption, the time required for the gas desorption volume of Group 1 to reach 80% is 122 s, which is much longer than Group 0 at 68 s. In the whole process of gas desorption, it took approximately 200 min for both groups to reach 80%. The analysis shows that the amount of gas desorption differs greatly in the early stage of gas desorption in coal with different metamorphic degrees, the pores and fissures in coal with high metamorphic degrees are well developed, and the gas adsorption and desorption capacity is strong.
3.2.2 Influence of the failure types on gas desorption
The desorption rate of coal gas is closely related to its damage degree. In the same mining area, the same coal seam, and the same location, the measurement of the initial gas release velocity (ΔP) of samples with different damage degrees and types shows that with the increase in the damage degree, the initial gas release velocity increases, that is, the gas desorption rate increases.
The pore-fracture system of tectonic coal is fully developed, and the rich internal surface area gives a strong gas adsorption capacity, but the low strength of the coal makes it easy to close microcracks under high pressure conditions and form “coal bricks”, thus sealing a large amount of high-energy gas (Zhang and Zhang, 2005). Wang Chaojie et al. studied the pressure gradient on the coal surface and the gas expansion energy generated during tectonic coal desorption (Wang et al., 2021c), establishing the evolution law of key gas parameters during the initial desorption process.
1) According to Figure 4A, the initial gas desorption rate of Type IV coal is significantly higher than that of Type II, and V0-2 is 7.22 ml/(gs). The initial rate of gas desorption from tectonic coal is 1.49 times that of primary structural coal; the difference in desorption rate is mainly in the first 30 s. With the extension of desorption time, the gas desorption rate of coal with different failure types tends to be equal.
2) Comparing Figures 2B,C and Figures 4B,C, the gas resolution of Type IV coal in the same mine and coal seam is significantly higher than that of Type II coal, and the more serious the damage of the coal body is, the larger the amount of gas desorption will be; the T180-3 of Type IV coal is 3.01 ml/g, which is 1.51 times that of Type II coal, and the T480-3 is 8.01 ml/g, which is 1.28 times that of Type II coal.
3) In the early stage of gas desorption, the time needed for the gas desorption of Group 2 to reach 80% was 77 s, which was almost the same as that of Group 0. However, in the whole process of gas desorption, it only takes 121 min for the desorption amount of Group 2 to reach 80%, which is far less than that of Group 0. This indicates that the initial gas desorption rate of Type IV coal is high, but the decay is faster, and the variation in gas desorption velocity in Type Ⅳ coal is greater per unit time. The gas desorption of tectonic coal can be divided into two different processes: first, a part of the methane adsorbed on the outer surface of coal and the surface of open large pores can communicate with the surrounding environment, and methane directly exchanges with the environment to produce desorption. Second, the other part of the adsorbed methane on the internal pore surface of coal must undergo a diffusion process to exchange with the environment and produce desorption. Surface adsorption and desorption can be completed instantaneously, while the diffusion process is relatively slow, so the desorption speed of structural coal is faster.
3.2.3 Influence of the particle size on gas desorption
There is a correlation between the coal failure type and the coal particle size on the gas desorption law. The experimental results show that the gas desorption rate is highly sensitive to the particle size of the coal sample. The larger the particle size is, the greater the distance of gas diffusion and flow, the greater the resistance, and the smaller the desorption rate and the desorption amount; the smaller the particle size is, the shorter the distance of gas diffusion and migration, and the larger the desorption rate and the desorption amount; and the effect of particle size on gas desorption has a limit value. When the limit particle size is reached, the desorption rate no longer changes with the particle size. Li et al. (2019) established a mathematical expression for the desorption index K1, believing that the smaller the particle size of the drill cuttings is, the more significant the desorption performance.
1) According to Figure 5A, as the particle size decreases, the initial gas desorption rate increases: V0-3 is 7.83 ml/(gs). The initial gas desorption rate of coal with particle sizes of 0.5–1.0 mm is 1.62 times that of coal with particle sizes of 1.0–3.0 mm.
2) Comparing Figure 2B and Figure 5B, there is little difference in the total amount of gas desorption in 180 s for coal with different particle sizes. The desorption capacity of small particle size coal is only 0.42 ml/g more than that of large particle size coal.
3) Comparing Figure 2C and Figure 5C, it can be seen that the gas desorption capacity of coal with a particle size of 0.5–1.0 mm in 480 min is significantly higher, which is 10.53 ml/g, 1.68 times higher than that of coal with a particle size of 1.0–3.0 mm.
4) The experimental results also show that the desorption amount of coals with different failure types is very different, even if the particle size is the same, that is, the initial gas desorption increase rate of Type II coal (primary structured coal) is significantly higher than that of Type IV coal (tectonic coal).
3.2.4 Influence of the pressure on gas desorption
The gas adsorption equilibrium pressure is an important factor affecting the gas desorption law. It not only characterizes the gas content in coal but also provides the energy required for gas desorption and diffusion. The gas desorption rate and desorption amount of the same coal sample increased with increasing gas adsorption equilibrium pressure in the same time period.
1) According to Figure 6A, with the increase in adsorption equilibrium pressure, the initial rate of gas desorption increases continuously. Pressure has a particularly significant effect on the gas desorption rate at the initial stage (within 10 s), and the desorption rate does not change much in 10–90 s.
2) Comparing Figure 2B and Figures 6B,D,F,H, it can be seen that with the increase in adsorption equilibrium pressure, the initial gas desorption amount increases continuously. The effect of pressure on the initial gas desorption rate and desorption amount is significant.
3) Comparing Figure 2C and Figures 6C,E,G,I, it can be seen that with the increase of adsorption equilibrium pressure, the total amount of gas desorption also increases, T480-4 is 5.39 ml/g, T480-0 is 6.28 ml/g, T480-5 is 10.22 ml/g, T480-6 is 12.69 ml/g, T480-7 is 12.98 ml/g, and with the increase of pressure, the time for gas desorption to reach more than 80% is gradually shortened.
4) Furthermore, the gas desorption rate and desorption amount of Type IV coal (tectonic coal) increase faster with pressure. At the same time, with the increase in adsorption equilibrium pressure, the gas desorption rate of Type IV coal decays faster and tends to be stable after 10 s.
5) The effect of pressure on gas desorption is mainly to increase the ability of coal particles to adsorb gas. The increase in the gas concentration gradient in the coal changes the stress state of the coal particles, and the increase in pore stress mainly changes the shape of the coal particle pores, making the elastic deformation of coal significant and improving the gas desorption rate. The intuitive response is that as the pressure increases, the gas desorption rate of coal particles increases. The effect of pressure on gas desorption is obvious, regardless of the desorption amount, desorption rate, desorption efficiency and so on.
3.2.5 Influence of the temperature on gas desorption
Some studies suggest that with increasing temperature, the ability of coal to adsorb gas gradually decreases (Kim, 1977; Fails, 1996). However, Krooss et al. (2002) believed that when the gas adsorption equilibrium pressure was higher than a certain value, the gas adsorption isotherm curves of coal at different temperatures would appear to cross; when the gas adsorption equilibrium pressure was higher than the pressure at the cross point, with increasing temperature, the adsorption amount under the same pressure also increased. From the perspective of gas expansion energy, Li et al. (2022) found that with increasing desorption time, the degree of linear correlation between gas expansion energy, desorption rate and desorption amount decreased.
Gas adsorption by coal is an exothermic process, and gas desorption is an endothermic process. Temperature has a great influence on gas desorption. The higher the temperature is, the higher the ability of gas molecules to desorb from the pores and surface of coal, and the easier it is for gas to be desorbed from the coal body to become free gas. The amount of gas desorbed per unit time and the amount of gas desorbed cumulatively in the same time period are also larger. Therefore, temperature is an important factor affecting the law of coal gas desorption.
1) According to Figure 7A, the gas desorption rate does not change significantly, and the desorption rate of Type IV coal (tectonic coal) only slightly varies with temperature. In the initial stage (within 30 s), the gas desorption rate decays rapidly at 20°C, but the gas desorption rate decays slowly at 40°C.
2) Comparing Figures 2B,C and Figures 7B–E, the effect of temperature change on the total amount of gas desorption is almost the same, but its increment over time is different. Initial amount of gas desorption followed the order T180 (20°C) > T180 (30°C) > T180 (40°C), while the total amount of gas desorption followed the order T480 (20°C) > T480 (40°C) > T480 (30°C). The analysis indicated that with increasing temperature, the gas content adsorbed in the coal body decreased correspondingly.
3.3 Tradeoff analysis of the influencing factors for gas desorption
Some scholars have performed a considerable amount of research on the influencing factors of gas desorption, and they have differing opinions on which is the single dominating factor. However, there are few studies on the weight analysis of each factor. Structural deformation has a very important effect on the particle size and adsorption/desorption properties of coal, and deformation can promote gas desorption, which increases as the deformation increases (Li et al., 2022). Through regression analysis, some scholars believe that the influence sensitivity order of gas desorption is pressure > temperature > particle size > moisture > moulding pressure (Wang et al., 2019).
In this paper, the coal property factors (metamorphic degree, failure type, particle size) and environmental factors (pressure, temperature) are experimentally studied. To weigh the sensitivity of each factor, the R-value method is proposed, which combines four indicators: gas desorption rate, gas desorption efficiency, initial gas desorption volume and total gas desorption.
The results of the correlation analysis are shown in Table 7.
4 Conclusion
1) Under different experimental conditions, there is a monotonically decreasing power function relationship between the initial gas desorption rate and time, that is, Vt=a t - b (a, b are regression constants). There is a monotonically increasing logarithmic function relationship between the gas desorption amount and time, namely, Tt=aln(t) + b (a, b are regression constants), and the curve has a limit value.
2) In the initial stage of gas desorption (0–180 s), the gas desorption amount is very large in 0–10 s, and then the gas desorption amount increases slowly, while the desorption rate is almost uniform. From the perspective of the entire gas desorption process, the gas desorption amount increases rapidly in 0–30 min, and the gradient of the increase in gas desorption amount decreases in 30–180 min; at 180 min, the gas desorption amount is approximately 80% of the total desorption. When the desorption time is longer than 180 min, the gas desorption amount basically stablizes.
3) The weight of the following factors affecting gas resolution is listed as follows: pressure > degree of metamorphism > particle size > type of failure > temperature. If the coal is subjected to strong structural extrusion and shearing during the formation process, the coal is broken and pulverized, and abundant micropores and inner surfaces are formed. These microcracks easily accumulate free gas. However, coal with high pressure, serious damage, and the poor mechanical strength of powdery or mylonitic coal is beneficial to the rapid desorption and release of gas within 0–10 s in the initial stage of gas desorption, and the risk of gas outburst is high.
Data availability statement
The datasets presented in this study can be found in online repositories. The names of the repository/repositories and accession numbers can be found in the article/Supplementary Material.
Author contributions
All authors listed have made a substantial, direct, and intellectual contribution to the work and approved it for publication. XH: Experiment, Data curation, Formal analysis, Writing—original draft. H-qC: Conceptulization, Funding acquisition, Supervision. HZ: Conceptualization, Experiment. Z-zW: Supervision. Z-hW: Data curation. G-sS: Modify, Correction.
Funding
This work was supported by the National Scientific Foundation of China (41372160) and plan support for key scientific research projects of colleges and universities in Henan Province (20B620001).
Conflict of interest
The authors declare that the research was conducted in the absence of any commercial or financial relationships that could be construed as a potential conflict of interest.
Publisher’s note
All claims expressed in this article are solely those of the authors and do not necessarily represent those of their affiliated organizations, or those of the publisher, the editors and the reviewers. Any product that may be evaluated in this article, or claim that may be made by its manufacturer, is not guaranteed or endorsed by the publisher.
References
Chen, X. J., Cheng, Y. P., He, T., and Li, X. (2013). Water injection impact on gas diffusion characteristic of coal. J. Min. Saf. Eng. 03, 443–448. doi:10.13210/j.cst.2013.03.024
Fails, T. G. (1996). Coalbed methane potential of some Variscan foredeep basins. Geol. Soc. Lond. Spec. Publ. 109 (1), 13–26. doi:10.1144/gsl.sp.1996.109.01.02
Fu, X. W. J., and Yang, T. H. (2008). Gas irradiation feature of tectonic coal. J. China coal Soc. 07, 775–779. doi:10.3321/j.issn:0253-9993.2008.07.011
Grażyna, C. S., and Kinga, B. (1998). The effect of coal metamorphism on methane desorption. Fuel (Lond). 6, 645–648. doi:10.1016/S0016-2361(97)00177-4
Hao, J. S., Yuan, C. F., and Zhang, Z. X. (2000). The tectonic coal and its effects on coal and gas outburst. J. Jiaozuo Inst. Technol. 06, 403–406. doi:10.3969/j.issn.1673-9787.2000.06.001
Jia, D. X., and Chen, X. J. (2009). Test and research on gas desorption law of intensive failed coal. Coal Sci. Technol. 06, 64–66. doi:10.13199/j.cst.2009.06.69.jiadx.025
Jiang, B., Qin, Y., Ju, Y. W., Wang, J. L., and Li, M. (2009). The coupling mechanism of the evolution of chemical structure with the characteristics of gas of tectonic coals. Earth Sci. Front. 02, 262–271. doi:10.3321/j.issn:1005-2321.2009.02.020
Kim, A. G. (1977). Estimating methane content of bituminous coalbeds from adsorption data. Pittsburgh, PA: United States Department of the Interior, Bureau of Mines, 8245.
Krooss, B. M., Bergen, F. V., Gensterblum, Y., Siemons, N., Pagnier, H. J. M., and David, P. (2002). High-pressure methane and carbon dioxide adsorption on dry and moisture-equilibrated Pennsylvanian coals. Int. J. Coal Geol. 51 (2), 69–92. doi:10.1016/S0166-5162(02)00078-2
Li, C. W., and Lei, D. J. (2012). Experimental research of the influence of static electric field on the characteristics of coal gas emission. J. China Coal Soc. 06, 28–30. doi:10.13225/j.cnki.jccs.2012.06.019
Li, C. W., Xue, H. L., Hu, P., Guan, C., and Liu, W. B. (2018). Effect of stress on the diffusion kinetics of methane during gas desorption in coal matrix under different equilibrium pressures. J. Geophys. Eng. 15 (3), 841–851. doi:10.1088/1742-2140/aaa8ad
Li, L., Sun, Z. G., Wang, F. K., and Zhang, K. Z. (2019). Study on the gas desorption law and indicator influencing factors of fixed-size coal samples. Sci. Rep. 9 (1), 17134. doi:10.1038/s41598-019-53211-4
Li, S. G., Bai, Y., Lin, H. F., Yan, M., Long, H., and Guo, D. D. (2021). Effect of N2/CO2 injection pressure on CH4 desorption in gas-bearing coal rock. Nat. Gas. Ind. 03, 80–89. doi:10.3787/j.issn.1000-0976.2021.03.009
Li, X. S., Ju, Y. W., Song, Y., Yan, Z. F., and Li, Q. G. (2022a). Particle size and internal structure of deformed coal: Microstructure and adsorption/desorption characteristics of CO2 and CH4. Front. Earth Sci. (Lausanne). 10, 876196. doi:10.3389/feart.2022.876196
Li, X. W., Wang, C. J., Chen, Y. J., and Li, H. N. (2022b). Influence of temperature on gas desorption characterization in the whole process from coals and its application analysis on outburst risk prediction. Fuel 321, 124021. doi:10.1016/J.FUEL.2022.124021
Li, X. Y., and Si, S. L. (2004). Coalbed gas desorption characteristics of coal reservoir in China. Coal Geol. Explor. 03, 27–29. doi:10.3969/j.issn.1001-1986.2004.03.009
Li, Y. B. (2011). Experimental study on initial stage features of gas desorption of tectonic coal. Master’s thesis. China: Henan Polytechnic University.
Liu, Y. W. (2011). Study on gas emission rules, mechanism and dynamic model from coal particle. Doctoral dissertation. China: Henan Polytechnic University.
Ministry of coal industry of the people's Republic of China (1997). Method for the determination of methane sorption on coal (high pressure volumetric method). China: China Standard Publishing House.
Tang, D. Z., and Wang, W. (2010). Control mechanism of coal reservoir physical properties and prediction method of favorable reservoi. Beijing: Science Press.
Tu, Q. Y., Cheng, Y. P., Xue, S., and Ren, T. (2022). Effect of particle size on gas energy release for tectonic coal during outburst process. Fuel 307, 121888. doi:10.1016/J.FUEL.2021.121888
Wang, C. J., Li, X. W., Xu, C. H., Chen, Y. J., Tang, Z. X., Zhang, C., et al. (2021a). Dynamic characterization during gas initial desorption of coal particles and its influence on the initiation of coal and gas outbursts. Processes 9 (7), 1101. doi:10.3390/PR9071101
Wang, C. J., Yang, S. Q., Li, J. H., Li, X. W., and Jiang, C. L. (2018a). Influence of coal moisture on initial gas desorption and gas-release energy characteristics. Fuel 232, 351–361. doi:10.1016/j.fuel.2018.06.006
Wang, F. K., Liang, Y. P., Li, X. D., Li, L., Li, J. G., and Chen, Y. L. (2019). Orthogonal experimental study on multifactor conditions for gas desorption in coal. Adv. Civ. Eng. 2019, 1–12. doi:10.1155/2019/3028721
Wang, Y. L. (2018). Experimental study on gas desorption and initial velocity of gas dispersion in low permeability and outburst coal seam in Guizhou. Master’s thesis. China: Guizhou University.
Wang, Z. Y., Cheng, Y. P., Wang, L., Zhou, H. X., He, X. X., Yi, M. H., et al. (2020). Characterization of pore structure and the gas diffusion properties of tectonic and intact coal: Implications for lost gas calculation. Process Saf. Environ. Prot. 135, 12–21. doi:10.1016/j.psep.2019.12.020
Wang, Z. Z., Fu, X. H., Deng, Z., and Pan, J. N. (2021b). Investigation of adsorption-desorption, induced strains and permeability evolution during N2-ECBM recovery. Nat. Resour. Res. 30 (5), 3717–3734. doi:10.1007/s11053-021-09884-8
Wang, Z. Z., Fu, X. H., Hao, M., Li, G. F., Pan, J. N., Niu, Q. H., et al. (2021c). Experimental insights into the adsorption-desorption of CH4/N2 and induced strain for medium-rank coals. J. Petroleum Sci. Eng. 204, 108705. doi:10.1016/j.petrol.2021.108705
Wang, Z. Z., Pan, J. N., Hou, Q. L., Niu, Q. H., Tian, J. J., Wang, H. C., et al. (2018b). Changes in the anisotropic permeability of low-rank coal under varying effective stress in Fukang mining area, China. Fuel 234, 1481–1497. doi:10.1016/j.fuel.2018.08.013
Wang, Z. Z., Pan, J. N., Hou, Q. L., Yu, B. S., Li, M., and Niu, Q. H. (2018c). Anisotropic characteristics of low-rank coal fractures in the Fukang mining area, China. Fuel 211, 182–193. doi:10.1016/j.fuel.2017.09.067
Xiao, Z. G. (2010). Experimental study and application of inhibitory effect on gas desorption of coal seam injected with water. Doctoral dissertation. China: Henan Polytechnic University.
Xie, X. X. (2014). Experimental study on gas loss quantity of tecnonic coal. Master’s thesis. China: Henan Polytechnic University.
Xu, K., Liu, M. J., and Liu, Y. W. (2003). Study of the adsorption and desorption (surface modification) in alternative electronic field. Coal Geol. Explor. 03, 23–26. doi:10.1007/s11769-003-0003-x
Zhang, G. H. (2011). Experimental study of external solution invasion impact on gas desorption about coal with gas. Doctoral dissertation. China: Liaoning Technical University.
Zhang, Q. (2018). Influence of metamorphic grade on gas adsorption/desorption characteristics of outburst coal. Master’s thesis. China: China University of Mining and Technology.
Zhang, X. M., Han, B. S., and Li, J. W. (2006). CBM storage character of lignite and gas content estimate method. Coal Geol. Explor. 34, 28–30. doi:10.3969/j.issn.1001-1986.2006.03.008
Zhang, Y. G. (2006). Evolution of deformed coal and process of coal mechanochemistry. Doctoral dissertation. China: Taiyuan university of technology.
Zhang, Z. M., and Zhang, Y. G. (2005). Gas geological law and gas prediction[M]. Beijing: China Coal Industry Publishing House, 23–31.
Keywords: gas content, gas desorption, weighting analysis, gas pressure, metamorphism, particle size
Citation: He X, Cui H-q, Zhang H, Wang Z-z, Wang Z-h and Shi G-s (2023) Experimental study and weighting analysis of factors influencing gas desorption. Front. Earth Sci. 10:1053142. doi: 10.3389/feart.2022.1053142
Received: 25 September 2022; Accepted: 04 November 2022;
Published: 16 January 2023.
Edited by:
Junjian Zhang, Shandong University of Science and Technology, ChinaReviewed by:
Zhen Lou, China University of Mining and Technology, ChinaYanyan Chen, Research Institute of Petroleum Exploration and Development (RIPED), China
Copyright © 2023 He, Cui, Zhang, Wang, Wang and Shi. This is an open-access article distributed under the terms of the Creative Commons Attribution License (CC BY). The use, distribution or reproduction in other forums is permitted, provided the original author(s) and the copyright owner(s) are credited and that the original publication in this journal is cited, in accordance with accepted academic practice. No use, distribution or reproduction is permitted which does not comply with these terms.
*Correspondence: Hong-qing Cui, aHFjdWlAaHB1LmVkdS5jbg==; Hang Zhang, MzgxNTM3MzQxQHFxLmNvbQ==