- MNR Key Laboratory of Saline Lake Resources and Environments, Institute of Mineral Resources, Chinese Academy of Geological Sciences, Beijing, China
Fluorine (F) is one of the most important environmentally harmful elements released by volcanic activity, and the bentonite deposits that formed from volcanic ashes are potentially harmful to the environment. However, the mechanisms governing F-rich bentonite formation and its F speciation composition remain enigmatic. The F-rich bentonite deposits are widely distributed in the Early-Middle Strata of the Sichuan Basin, South China. Detailed mineralogical and geochemical studies were conducted on the bentonite deposits from five sections of the Sichuan Basin. X-ray diffraction (XRD) analyses indicate that the F-rich bentonites mainly contain quartz, carbonates (calcite and dolomite) or gypsum, and clay minerals, while the clay minerals are dominated by illite and illite/smectite (I/S). Clay mineralogical studies suggest that bentonites were transformed from volcanic ashes during diagenesis by smectite illitization. The major and trace element distribution in F-rich bentonite deposits altered from volcanic ashes is most likely derived from felsic magmas, and alteration of the parent rocks (e.g., rhyolites) to bentonite is associated with leaching and subsequent removal of F. The total fluorine content
1 Introduction
Fluorine (F) is the 13th element found in abundance on the Earth and one of the most important natural pollutants receiving increasing attention because of its high toxicity and reactivity, which are potentially harmful to the environment and humans (Halmer et al., 2002; Fuge, 2019; Schlesinger et al., 2020). Volcanoes are one of the most important natural sources of fluorine, and the global volcanogenic fluorine flux is estimated to range from 50 to 8,600 Gg/yr (Symonds et al., 1988; Halmer et al., 2002; Schlesinger et al., 2020). Volcanic eruptions are associated with the release of magmatic fluorine in the form of hydrogen fluoride through volcanic degassing and the huge volume of volcanic ash that likely delivers fluorine large distances from its source (Rubin et al., 1994). Although fluorine has been assumed to move rapidly from volcanic ash to water through dissolution of the glass fraction and mineral coatings precipitated on glass surfaces (Rubin et al., 1994; Bia et al., 2020), volcanic ash may be largely enriched in total fluorine relative to the original magmatic contents, e.g., a 6 − fold increase in volcanic ash from Ruapehu volcano (Cronin et al., 2003). Bentonite is an important type of clay deposit commonly formed from the alteration of volcanic ash (Altaner et al., 1984; Huff, 2016). Therefore, the bentonite deposits formed from volcanic ash in the marine and continental basins always had high fluorine concentrations (Starkey, 1982; Haamer and Karro, 2006), which may be potential long-term sinks or sources of fluorine in the environment.
Water-soluble fluorine in the sediments is most important for groundwater toxicity and contamination, making it a key factor in the retention and mobility of F in sediments (i.e., soil) (Wenzel and Blum, 1992; Deng et al., 2022). The environmental hazard of fluorine in volcanic ash is generally assessed by analyzing the content of water-soluble fluoride in the ash (Bellomo et al., 2007; Delmelle et al., 2021). However, the fluoride in volcanic ash also occurs in the form of poorly water-soluble compounds (Delmelle et al., 2021). Fluorine can likely be released under a variety of environmental conditions, which should be considered when evaluating the input and potential environmental hazards from F-rich volcanic eruptions (Bellomo et al., 2007). Few studies have been conducted on the bentonite deposits with high total fluorine concentrations regarding its F speciation, which complicates appropriate risk management for fluoride-rich altered volcanic ash (Starkey, 1982; Haamer and Karro, 2006). In addition, the material source of the F-rich bentonite deposits and their formation conditions remain enigmatic (Bellomo et al., 2007).
Distribution and development of bentonite in Early-Middle Triassic strata in the Sichuan Basin, South China, resulting in significant fluorine accumulation in the marine basin (Yan et al., 2015; Lin et al., 2020; Feng M. S. et al., 2021), making it an ideal field site to constrain the process of F-rich bentonite formation. In this study, we aim to characterize the fluorine speciation of bentonite deposits in the Sichuan Basin, South China using mineralogical methods and batch leaching experiments to decipher the formation process of F-rich bentonite deposits. This study is useful to evaluate appropriate risk management for F-rich bentonite deposits and improve our understanding of the behavior of volcanic fluorine accumulating in altered volcanic ash.
2 Geological setting
The Sichuan Basin was a stable craton basin located in the western region of the Yangtze block, northwest South China, bounded by the Qinling-Dabie orogen to the north, the Jiangnan orogen to the southeast, Songpan-Ganzi Terrance to the west, and the Sanjiang orogen to the southwest (Cawood et al., 2018; Liu et al., 2021) (Figure 1). The Yangtze Platform was stable and formed shallow-water carbonates from the Late Proterozoic to Middle Triassic (Lehrmann et al., 2005). The Early-Middle bentonite deposits are widely distributed in the Upper Yangtze Platform, South China. These bentonites are so-called “mung bean rock” (or “green bean rock”) which contains a high concentration of lithium and fluorine and has an area of about 7 × 106 km2 and a thickness of several tens of centimeters to tens of meters (Lin et al., 2020; Feng M. S. et al., 2021; Li et al., 2021). These altered volcanic ashes were believed to originate from the eruption of episodic volcanism caused by Large Igneous Province (Ma et al., 2019; Ju et al., 2020), and the extensive volcanic eruption caused by amalgamation and collision of terranes and continents during the closure of the Paleo-Tethys Ocean (Feng M. S. et al., 2021; Li et al., 2021), and the Indosinian movement (Xiao and Hu, 2005; Wang et al., 2019).
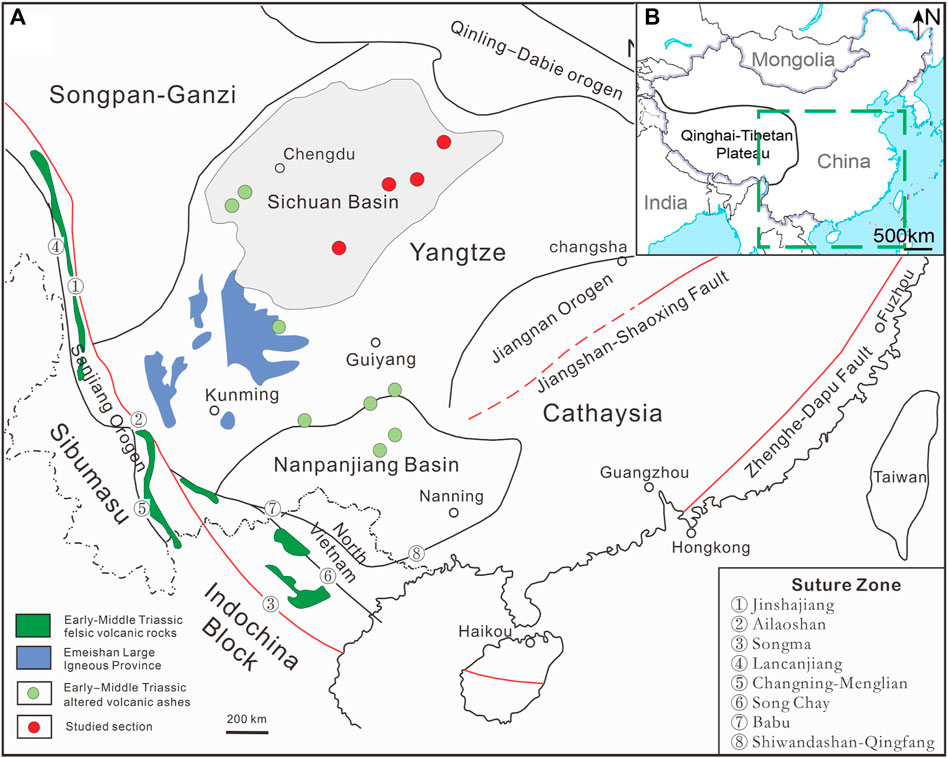
FIGURE 1. (A) Schematic geological map for South China and the distribution of Early-Middle Triassic bentonite deposits (based on the 1:5,000,000 geology map of China); (B) Insert map showing the location of the study area in China.
3 Materials and methods
3.1 Sampling
Five bentonite samples were collected from outcrops in five sections in South China, including the Yongchuan section, the Pinghong section, the Xiejiacao section, the Pianyazi section and the Kai section (Figure 1). The bentonite samples exhibit light yellowish, consolidated, and locally contain elliptical siliceous particles. It occurs as a stratified bed ranging from 30 cm to 2 m thick with no discernible stratification features in the layers. In the Pianyazi section, the altered volcanic ash occurs near the bottom of the Middle Triassic Leikoupo Formation, which is underlain and overlain by anhydrite (Figure 2). In other sections, the altered volcanic ashes usually occur at the bottom of the Middle Triassic Leikoupo Formation, which is underlain by the Lower Triassic Jialingjiang Formation in a shallow-water carbonate basin setting. The lower part of Leikoupo Formation consists of dolomite and the upper part of Jialingjiang Formation consists of limestone in the study area (Li et al., 2021).
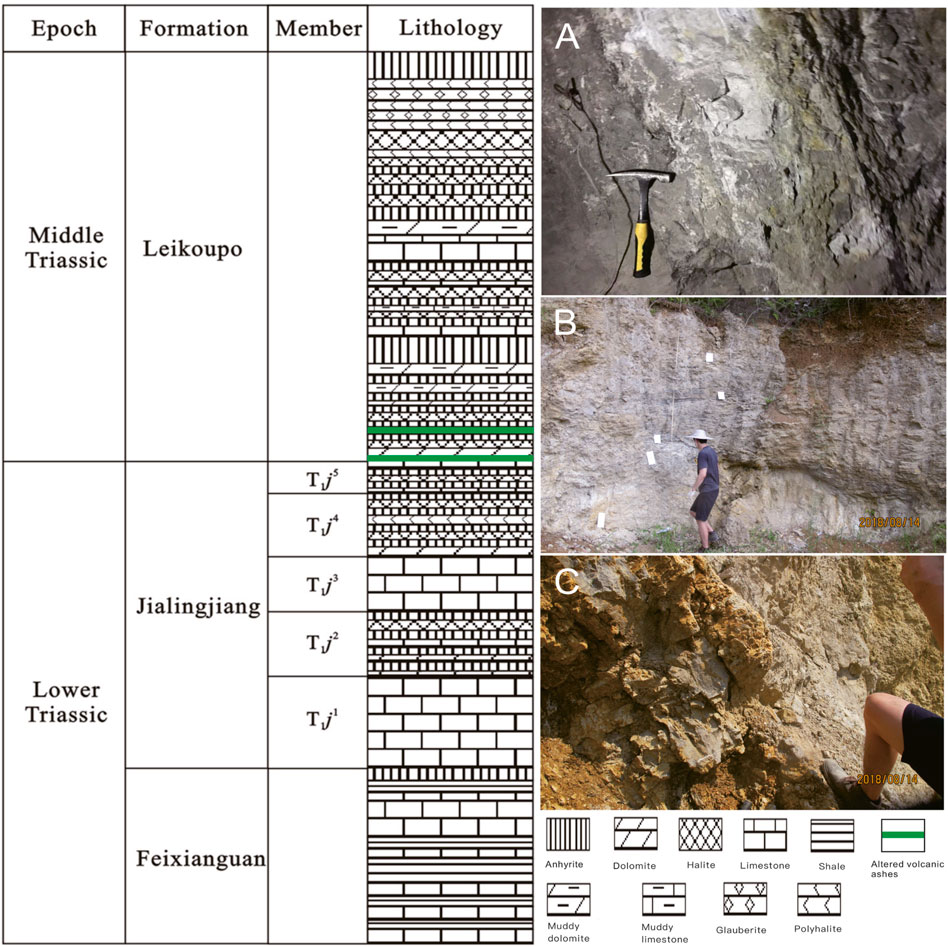
FIGURE 2. Generalized stratigraphy of the Early-Middle Triassic in the study area, South China (modified from Zhao et al. (2020)) and the representative photography showing outcrop of altered volcanic ashes; (A) Pianyazi section; (B) Pinghongcun section; (C) Kai section.
3.2 Sample characterization
Clay minerals (<2 μm) were separated from bentonite samples according to the methods of Köster et al. (2021a). The mineralogy of bentonite samples was confirmed by XRD using an X-ray diffractometer (TTR-3, Rigaku Crop, Tokyo, Japan), and Cu Kα radiation
In this formula,
3.3 Sequential chemical extraction experiments
Fluorine species in bentonite samples were determined separately in six categories: water-soluble fraction
4 Results
4.1 Mineralogical and chemical composition of bentonites
The XRD data of the bulk bentonite samples show that the bentonites in the Pianyazi section consist of clay minerals (46%), quartz (36%), gypsum (10%), and K-feldspar (8%) (Figures 3A; Table 1). In other sections, the mineral compositions are variable and mainly consist clay minerals (1%–37%), quartz (2%–34%), K-feldspar (0%–19%), dolomite (7%–79%) and calcite (3%–63%). The clay minerals are composed of illite (0%–99%), I/S (0%–86%), smectite (0%–13%) and C/S (0%–27%) (Figures 3B; Table 2). The dominance of I/S in bentonites could be an indication of the digenetic transformation of smectite into illite. The relative proportion of smectite in the I/S of the bentonite is around 15% in Xiejiacao and Yongchuan, indicating R3 ordered I/S, and is around 65% in other sections, indicating R1 ordered I/S.
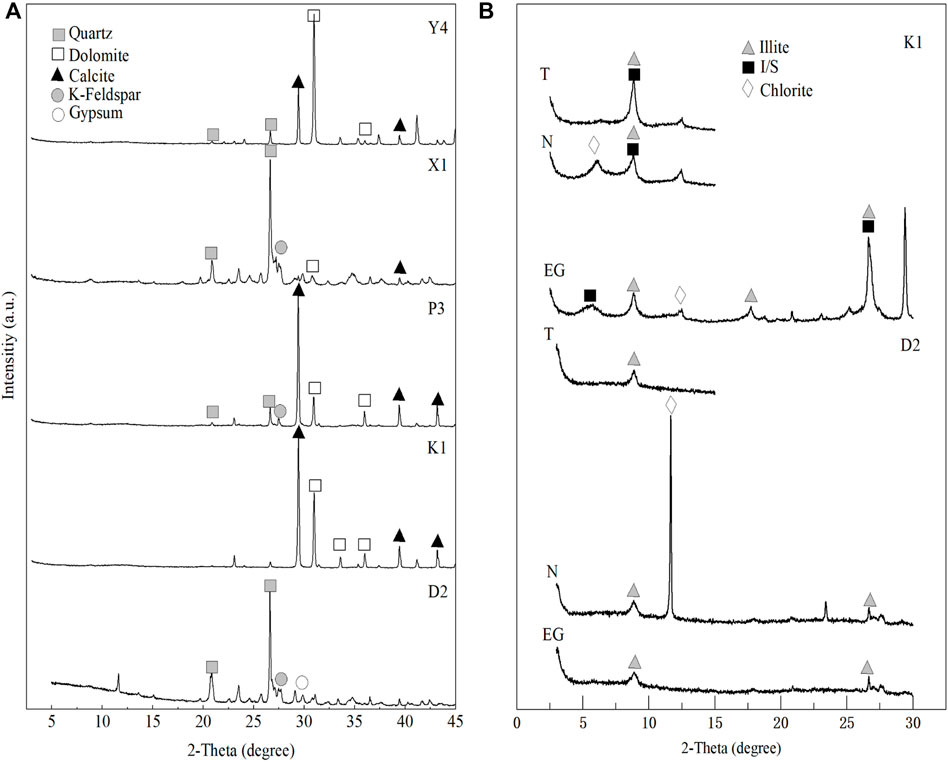
FIGURE 3. XRD pattern of bulk bentonites samples in Sichuan Basin, South China; (A) Bulk samples; (B) The diffraction pattern of clay minerals in representative samples (K1 and D2). N, Air-dried oriented clay samples; EG, Ethylene glycol-saturated clay samples; T, clay samples post heating to 550°C.
The chemical compositions of the bentonites are different in the study sections, which is consistent with the XRD results (Table 3). In Xiejiacao and Pianyazi sections, the most abundant major constituent of the bentonite was
4.2 Speciation of fluorine
The total F content
4.3 Risk assessment of F-rich bentonites
Li et al. (2005) proposed a method for assessing the risk of fluorine in soil based on the statistical relationship between the geochemical characteristics of fluorine in soils with high fluorine content and the local occurrence of endemic fluorosis in China, which were described as follows:
where
If the
5 Discussion
5.1 The formation process of F-rich bentonites
Bentonites are volcanic ashes that have undergone significant devitrification to dioctahedral smectite, and volcanic ashes are a precursor material for bentonites (Altaner et al., 1984; Huff, 2016; Namayandeh et al., 2020). Generally, smectite formed in marine subaqueous environments during diagenetic alteration of volcanic glass shards, releasing alkalis and alkaline Earth elements after initial hydration and cation exchange between the fluids and volcanic glass shards (De La Fuente et al., 2000; Huff, 2016; Hong et al., 2019). Under these conditions, the pH and salinity increased, favoring smectite formation (Hong et al., 2019; Milesi et al., 2019). In this study, the clay minerals of bentonites are composed of illite and I/S. The I/S in bentonites were derived from the illitization of smectite in subaqueous environments (McCarty et al., 2009; Gong et al., 2018). The transformation of smectite to illite by a mixed-layer I/S is a common mineralogical reaction that occurs during the diagenesis of altered volcanic ashes, with temperature and potassium availability being the main controlling factors (Nesbitt and Young, 1984; Cuadros, 2006; McCarty et al., 2009). The simplest form of smectite illitization can be described as the following reaction pathway (Bethke et al., 1986):
However, smectite illitization starts at about 70 ∼ 80 °C and lead to a decrease in smectite content according to the following reaction pathway: smectite → random I/S → ordered I/S → illite (e.g., Altaner and Ylagan, 1997; Cuadros, 2006; Abedini and Calagari, 2012; Gong et al., 2018). In this study, the mineralogy and geochemical composition of the bentonite are variable, which were probably controlled by the depositional environments (Hong et al., 2019). In the Pianyazi section, the presence of gypsum suggests that the bentonites at this site were probably formed in a restricted, subaqueous environment. In other sections, however, the presence of calcite and the absence of gypsum indicate that the bentonite at this site was probably formed in a subaqueous environment. Alteration of volcanic ash releases bicarbonate and cations drive precipitation of authigenic carbonate and clay minerals (Köster et al., 2021b). Furthermore, the high field strength elements (e.g., Nb, Zr) and
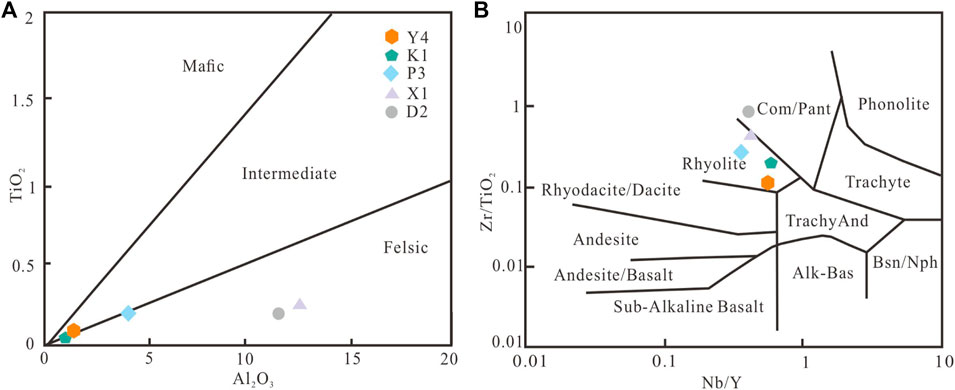
FIGURE 5. (A) Plot of
Volcanoes emit a variety of gases that include hydrogen fluoride and hydrogen chloride, which are the main components of high-temperature volcanic gas (Cronin et al., 2003; Bia et al., 2020; Delmelle et al., 2021). The volcanic gases interact rapidly with volcanic ash particles and especially with atmospheric water to form acidic aerosols (Gutmann et al., 2018; Zelenski et al., 2020). The smaller volcanic ash particles have a large surface area relative to their mass, which can transport significant amounts of soluble fluorine to pastures far downwind from an erupting volcano. Total fluorine in volcanic ash can be enriched by many factors compared to the original magmatic content, for example, by a factor of six relative to the original magmatic content in Ruapehu volcano (Cronin et al., 2003). Another important source of Early-Middle Triassic altered volcanic ash is the chemical weathering of F-rich volcanic rocks in the vicinity of the basin. High fluorine concentrations have always been found in felsic igneous rocks (Chowdhury et al., 2019; Liu et al., 2020; Amézaga-Campos et al., 2022). In this study, the altered volcanic ashes were considered to be derived from the eruption of episodic volcanism (e.g., Xiao and Hu, 2005; Lin et al., 2020; Feng M. S. et al., 2021). F is removed from minerals by chemical weathering at almost the same rate as other elements (Jacks et al., 2005). The CIA values in the collected samples vary between 54 and 76 with an average value of 65, which is lower than the PAAS value of 70 but higher than the UCC value of 48 (Taylor and McLennan, 1985). The presence of clay minerals (e.g., I/S and illite) in the samples is also evidence of chemical weathering and alteration of volcanic ash. Bentonites show Ca enrichment compared to the probable source rocks, most likely due to precipitation of the Ca-carbonate precipitation by alteration of volcanic ashes. The F contents in the samples show a strong negative correlation with the CIA values, which is most likely due to the fixation of fluorine in clay and secondary minerals in altered volcanic samples (Figure 6).
5.2 Enrichment mechanism of fluorine speciation
5.2.1 Adsorption of fluorine by clay minerals
Most of the F in bentonites was stored in residual silicates.
5.2.2 Adsorption of fluorine by carbonates
In this study, bentonites have high
5.2.3 Adsorption of fluorine by Fe (hydr)oxides
In this study,
6 Conclusion
The Early-Middle Triassic bentonite deposits in the Sichuan Basin, South China have anomalous high F contents that pose a major potential environmental hazard. In this work, the F speciation, mineralogical, and chemical composition of bentonites were studied in detail. The detailed mineralogical and geochemical composition of the bentonites suggests that the bentonites were transformed during diagenesis from volcanic ash by smectite illitization, and volcanic ashes are most likely derived from felsic magmas. The
Data availability statement
The original contributions presented in the study are included in the article/Supplementary Material, further inquiries can be directed to the corresponding author.
Author contributions
YL designed the research and wrote the manuscript. MZ, YZ, and EX discussed the results and commented on the manuscript.
Funding
This research is supported by the National Natural Science Foundation of China (42102115), State Public-interest Scientific Institution Basic Research Fund (Grant Number: SYSCR 2019-05), and the Geological Survey Program of the China Geological Survey (DD20190172).
Acknowledgments
YL acknowledges funding from China Scholarship Council. In a previous form, this manuscript benefitted from extensive comments from Dr. ELena Maters.
Conflict of interest
The authors declare that the research was conducted in the absence of any commercial or financial relationships that could be construed as a potential conflict of interest.
Publisher’s note
All claims expressed in this article are solely those of the authors and do not necessarily represent those of their affiliated organizations, or those of the publisher, the editors and the reviewers. Any product that may be evaluated in this article, or claim that may be made by its manufacturer, is not guaranteed or endorsed by the publisher.
References
Abedini, A., and Calagari, A. (2012). The mineralogy and geochemistry of Permian lateritic ores in east of Shahindezh, West-Azarbaidjan province. Iran. J. Crystallogr. Mineral. 20, 59–72.
Abedini, A., and Calagari, A. A. (2017). Geochemistry of claystones of the Ruteh Formation, NW Iran: Implications for provenance, source-area weathering, and paleo-redox conditions. Neues Jahrb. Min. Abh. 194, 107–123. doi:10.1127/njma/2017/0040
Abedini, A., and Khosravi, M. (2022). Geochemical constraints on the Zola-Chay river sediments, NW Iran: Implications for provenance and source-area weathering. Arab. J. Geosci. 15, 1515–1517. doi:10.1007/s12517-022-10822-y
Abedini, A., Rezaei Azizi, M., and Calagari, A. A. (2018). Lanthanide tetrad effect in limestone: a tool to environment analysis of the ruteh formation, NW Iran. Acta Geodyn. Geomater. 15, 229–246. doi:10.13168/agg.2018.0017
Abedini, A., Habibi Mehr, M., Khosravi, M., and Calagari, A. A. (2019a). Geochemical characteristics of the karst-type bauxites: an example from the kanirash deposit, NW Iran. Arab. J. Geosci. 12, 475–516. doi:10.1007/s12517-019-4601-z
Abedini, A., Rezaei Azizi, M., and Calagari, A. A. (2019b). REE mobility and tetrad effects in bauxites: an example from the kanisheeteh deposit, NW Iran. Acta Geodyn. Geomater. 193, 11–26. doi:10.13168/agg.2019.0002
Abedini, A., Khosravi, M., and Dill, H. G. (2020a). Rare Earth element geochemical characteristics of the late Permian Badamlu karst bauxite deposit, NW Iran. J. Afr. Earth Sci. 172, 103974. doi:10.1016/j.jafrearsci.2020.103974
Abedini, A., Rezaei Azizi, M., and Dill, H. G. (2020b). Formation mechanisms of lanthanide tetrad effect in limestones: an example from arbanos district, NW Iran. Carbonates Evaporites 35, 1–18. doi:10.1007/s13146-019-00533-z
Abedini, A. (2017). Mineralogy and geochemistry of the hizeh-jan kaolin deposit, northwest of varzaghan, east-azarbaidjan province, NW Iran. Iran. J. Crystallogr. Mineral. 24, 647–660.
Altaner, S. P., and Ylagan, R. F. (1997). Comparison of structural models of mixed-layer illite/smectite and reaction mechanisms of smectite illitization. Clays Clay Min. 45, 517–533. doi:10.1346/ccmn.1997.0450404
Altaner, S. P., Hower, J., Whitney, G., and Aronson, J. L. (1984). Model for K-bentonite formation: Evidence from zoned K-bentonites in the disturbed belt, Montana. Geol. 12, 412–415. doi:10.1130/0091-7613(1984)12⟨412:mfkfef⟩2.0.co;2
Amézaga-Campos, B. S., Villanueva-Estrada, R., Carrillo-Chavez, A., Morales-Arredondo, J. I., and Morán-Ramírez, J. (2022). Hydrogeochemistry characterization of an overexploited municipal, agricultural, and industrial aquifer, central Mexico. Appl. Geochem. 142, 105310. doi:10.1016/j.apgeochem.2022.105310
Bellomo, S., Aiuppa, A., D’Alessandro, W., and Parello, F. (2007). Environmental impact of magmatic fluorine emission in the Mt. Etna area. J. Volcanol. Geotherm. Res. 165, 87–101. doi:10.1016/j.jvolgeores.2007.04.013
Berry, R. W. (1999). Eocene and oligocene otay-type waxy bentonites of san diego county and baja California: Chemistry, mineralogy, petrology and plate tectonic implications. Clays Clay Min. 47, 70–83. doi:10.1346/ccmn.1999.0470108
Bethke, C. M., Vergo, N., and Altaner, S. P. (1986). Pathways of smectite illitization. Clays Clay Min. 34, 125–135. doi:10.1346/ccmn.1986.0340203
Bia, G., Borgnino, L., Zampieri, G., and Garcia, M. G. (2020). Fluorine surface speciation in South Andean volcanic ashes. Chem. Geol. 532, 119402. doi:10.1016/j.chemgeo.2019.119402
Budyanto, S., Kuo, Y.-L., and Liu, J. (2015). Adsorption and precipitation of fluoride on calcite nanoparticles: A spectroscopic study. Sep. Purif. Technol. 150, 325–331. doi:10.1016/j.seppur.2015.07.016
Calagari, A. A., Abedini, A., and Fatahi, S. (2015). Mineralogical and geochemical characteristics of the Sangab bentonite deposit, Isfehan province, Iran. njgpa. 227, 125–139. doi:10.1127/njgpa/2015/0504
Carpenter, R. (1969). Factors controlling the marine geochemistry of fluorine. Geochim. Cosmochim. Acta 33, 1153–1167. doi:10.1016/0016-7037(69)90038-6
Cawood, P. A., Zhao, G., Yao, J., Wang, W., Xu, Y., and Wang, Y. (2018). Reconstructing south China in phanerozoic and precambrian supercontinents. Earth. Sci. Rev. 186, 173–194. doi:10.1016/j.earscirev.2017.06.001
Chipera, S. J., and Bish, D. L. (2002). Thermal evolution of fluorine from smectite and kaolinite. Clays Clay Min. 50, 38–46. doi:10.1346/000986002761002658
Chowdhury, A., Adak, M. K., Mukherjee, A., Dhak, P., Khatun, J., and Dhak, D. (2019). A critical review on geochemical and geological aspects of fluoride belts, fluorosis and natural materials and other sources for alternatives to fluoride exposure. J. Hydrol. X. 574, 333–359. doi:10.1016/j.jhydrol.2019.04.033
Cronin, S. J., Neall, V., Lecointre, J., Hedley, M., and Loganathan, P. (2003). Environmental hazards of fluoride in volcanic ash: a case study from Ruapehu volcano, New Zealand. J. Volcanol. Geotherm. Res. 121, 271–291. doi:10.1016/s0377-0273(02)00465-1
Cuadros, J. (2006). Modeling of smectite illitization in burial diagenesis environments. Geochim. Cosmochim. Acta 70, 4181–4195. doi:10.1016/j.gca.2006.06.1372
De La Fuente, S., Cuadros, J., Fiore, S., and Linares, J. (2000). Electron microscopy study of volcanic tuff alteration to illite-smectite under hydrothermal conditions. Clays Clay Min. 48, 339–350. doi:10.1346/ccmn.2000.0480305
Delmelle, P., Maters, E. C., Calkins, J. A., Gaspard, F., Opfergelt, S., and Jenkins, S. F. (2021). Eruptive style controls the formation of silicon hexafluoride salts on volcanic ash: The case of the 2010 eruption of eyjafjallajökull volcano, Iceland. Chem. Geol. 579, 120327. doi:10.1016/j.chemgeo.2021.120327
Deng, L., Wang, J., Xu, B., Yang, X., and Hu, A. (2022). Fluorine speciation in loess, related quality assessment, and exposure risks implication in the Shaanxi Loess Plateau. Environ. Earth Sci. 81, 326–413. doi:10.1007/s12665-022-10437-2
Du, J., Wu, D., Xiao, H., and Li, P. (2010). Adsorption of fluoride on clay minerals and their mechanisms using X-ray photoelectron spectroscopy. Front. Environ. Sci. Eng. China 5, 212–226. doi:10.1007/s11783-010-0255-5
Farrah, H., Slavek, J., and Pickering, W. (1987). Fluoride interactions with hydrous aluminum oxides and alumina. Soil Res. 25, 55–69. doi:10.1071/sr9870055
Feng, M.-S., Meng, W.-B., Zhang, C.-G., Qing, H.-R., Chi, G.-X., Wang, J., et al. (2021a). Geochronology and geochemistry of the ‘green-bean rock’(GBR, a potassium-rich felsic tuff) in the Western margin of the Yangtze platform, SW China: Significance for the Olenekian-Anisian boundary and the Paleo-Tethys tectonics. Lithos 382, 105922. doi:10.1016/j.lithos.2020.105922
Feng, X., Steiner, Z., and Redfern, S. A. (2021b). Fluorine incorporation into calcite, aragonite and vaterite CaCO3: Computational chemistry insights and geochemistry implications. Geochim. Cosmochim. Acta 308, 384–392. doi:10.1016/j.gca.2021.05.029
Fuge, R. (2019). Fluorine in the environment, a review of its sources and geochemistry. Appl. Geochem. 100, 393–406. doi:10.1016/j.apgeochem.2018.12.016
Gong, N., Hong, H., Huff, W. D., Fang, Q., Bae, C. J., Wang, C., et al. (2018). Influences of sedimentary environments and volcanic sources on diagenetic alteration of volcanic tuffs in South China. Sci. Rep. 8, 7616–7712. doi:10.1038/s41598-018-26044-w
Gutmann, A., Bobrowski, N., Roberts, T. J., Rüdiger, J., and Hoffmann, T. (2018). Advances in bromine speciation in volcanic plumes. Front. Earth Sci. (Lausanne). 6, 213. doi:10.3389/feart.2018.00213
Haamer, K., and Karro, E. (2006). High fluoride content of K-bentonite beds in Estonian Paleozoic carbonate rocks. Fluoride 39, 132–137.
Halmer, M. M., Schmincke, H. U., and Graf, H. F. (2002). The annual volcanic gas input into the atmosphere, in particular into the stratosphere: a global data set for the past 100 years. J. Volcanol. Geotherm. Res. 115, 511–528. doi:10.1016/s0377-0273(01)00318-3
He, B., Zhong, Y. T., Xu, Y. G., and Li, X. H. (2014). Triggers of permo-triassic boundary mass extinction in South China: The siberian traps or paleo-tethys ignimbrite flare-up? Lithos 204, 258–267. doi:10.1016/j.lithos.2014.05.011
Hiemstra, T., and Riemsdijk, W. V. (2000). Fluoride adsorption on goethite in relation to different types of surface sites. J. Colloid Interface Sci. 225, 94–104. doi:10.1006/jcis.1999.6697
Hong, H., Algeo, T. J., Fang, Q., Zhao, L., Ji, K., Yin, K., et al. (2019). Facies dependence of the mineralogy and geochemistry of altered volcanic ash beds: An example from Permian-Triassic transition strata in southwestern China. Earth. Sci. Rev. 190, 58–88. doi:10.1016/j.earscirev.2018.12.007
Hong, H., Jin, X., Wan, M., Ji, K., Liu, C., Algeo, T. J., et al. (2020). Occurrence of anatase in reworking altered ash beds (K-bentonites and tonsteins) and discrimination of source magmas: a case study of terrestrial permian–triassic boundary successions in China. Clay Min. 55, 329–341. doi:10.1180/clm.2021.2
Jacks, G., Bhattacharya, P., Chaudhary, V., and Singh, K. (2005). Controls on the Genesis of some high-fluoride groundwaters in India. Appl. Geochem. 20, 221–228. doi:10.1016/j.apgeochem.2004.07.002
Ju, P., Wang, X., Wang, Z., Liu, X., Zhong, J., and Zhang, Z. (2020). Characteristics and geological significance of the triassic mungbean rocks in the wenquan town area, northern chongqing. Geoscience 34, 431. doi:10.19657/j.geoscience.1000-8527.2020.018
Kiaeshkevarian, M., Calagari, A. A., Abedini, A., and Shamanian, G. (2020). Geochemical and mineralogical features of karst bauxite deposits from the alborz zone (northern Iran): Implications for conditions of formation, behavior of trace and rare Earth elements and parental affinity. Ore Geol. Rev. 125, 103691. doi:10.1016/j.oregeorev.2020.103691
Kitano, Y., and Okumura, M. (1973). Coprecipitation of fluoride with calcium carbonate. Geochem. J. 7, 37–49. doi:10.2343/geochemj.7.37
Köster, M., Kars, M., Schubotz, F., Tsang, M.-Y., Maisch, M., Kappler, A., et al. (2021a). Evolution of (bio-) geochemical processes and diagenetic alteration of sediments along the tectonic migration of ocean floor in the Shikoku Basin off Japan. Geochem. Geophys. Geosyst. 22, e2020GC009585. doi:10.1029/2020gc009585
Köster, M., Manners, H. R., Meixner, A., Kasemann, S. A., Staubwasser, M., Morono, Y., et al. (2021b). “Volcanic ash alteration as driver of (bio-)geochemical iron cycling in deep marine sediments of the Nankai Trough,” in Goldschmidt2021, July 4, 2021. doi:10.7185/gold2021.6097
Lehrmann, D. J., Enos, P., Payne, J. L., Montgomery, P., Wei, J., Yu, Y., et al. (2005). Permian and triassic depositional history of the Yangtze platform and great bank of guizhou in the nanpanjiang basin of guizhou and guangxi, south China. Albertiana 33, 149–168.
Leontopoulou, G., Christidis, G. E., Rousakis, G., Müller, N. S., Papatheodorou, G., and Geraga, M. (2021). Provenance analysis of sediments in the south-east aegean during the upper quaternary: a composite approach based on bulk and clay mineralogy and geochemistry. Clay Min. 56, 229–249. doi:10.1180/clm.2022.2
Li, J., Xie, Z., Xu, J., and Wu, W. (2005). Preliminary study on guideline on soil health quality index of fluorine and method of its evaluation in China. J. Zhejiang Univ. 31, 593–597.
Li, W., Shi, Z., Yin, G., Tian, Y., Wang, Y., and Zhang, J. (2021). Origin and tectonic implications of the early Middle Triassic tuffs in the Western Yangtze Craton: Insight into whole-rock geochemical and zircon U-Pb and Hf isotopic signatures. Gondwana Res. 93, 142–161. doi:10.1016/j.gr.2020.12.030
Lin, Y., Zheng, M., Zhang, Y., Xing, E., Redfern, S. A., Xu, J., et al. (2020). Mineralogical and geochemical characteristics of triassic lithium-rich K-bentonite deposits in Xiejiacao section, south China. Minerals 10, 69. doi:10.3390/min10010069
Liu, Q., Zhang, B., Zhai, D., Han, Z., Chi, Q., Nie, L., et al. (2020). Continental-scale distribution and source identification of fluorine geochemical provinces in drainage catchment sediment and alluvial soil of China. J. Geochem. Explor. 214, 106537. doi:10.1016/j.gexplo.2020.106537
Liu, S., Yang, Y., Deng, B., Zhong, Y., Wen, L., Sun, W., et al. (2021). Tectonic evolution of the Sichuan Basin, southwest China. Earth. Sci. Rev. 213, 103470. doi:10.1016/j.earscirev.2020.103470
Ma, S., Wang, D., Sun, Y., Li, C., and Zhong, H. (2019). Geochronology and geochemical characteristics of lower-middle Triassic clay rock and their significances for prospecting clay-type lithium deposit. Earth Sci. 44, 2–14. doi:10.3799/dqkx.2018.343
McCarty, D. K., Sakharov, B. A., and Drits, V. A. (2009). New insights into smectite illitization: A zoned K-bentonite revisited. Am. Mineral. 94, 1653–1671. doi:10.2138/am.2009.3260
Milesi, V. P., Jézéquel, D., Debure, M., Cadeau, P., Guyot, F., Sarazin, G., et al. (2019). Formation of magnesium-smectite during lacustrine carbonates early diagenesis: Study case of the volcanic crater lake Dziani Dzaha (Mayotte–Indian Ocean). Sedimentology 66, 983–1001. doi:10.1111/sed.12531
Namayandeh, A., Modabberi, S., and López-Galindo, A. (2020). Trace and rare Earth element distribution and mobility during diagenetic alteration of volcanic ash to bentonite in eastern Iranian bentonite deposits. Clays Clay Min. 68, 50–66. doi:10.1007/s42860-019-00054-9
Nesbitt, H. W., and Young, G. M. (1982). Early Proterozoic climates and plate motions inferred from major element chemistry of lutites. Nature 299, 715–717. doi:10.1038/299715a0
Nesbitt, H. W., and Young, G. M. (1984). Prediction of some weathering trends of plutonic and volcanic rocks based on thermodynamic and kinetic considerations. Geochim. Cosmochim. Acta 48, 1523–1534. doi:10.1016/0016-7037(84)90408-3
Rathore, V. K., and Mondal, P. (2017). Competitive adsorption of arsenic and fluoride onto economically prepared aluminum oxide/hydroxide nanoparticles: Multicomponent isotherms and spent adsorbent management. Ind. Eng. Chem. Res. 56, 8081–8094. doi:10.1021/acs.iecr.7b01139
Ren, C., Zhou, M., Liu, Z., Liang, L., Li, X., Lu, X., et al. (2021). Enhanced fluoride uptake by layered double hydroxides under alkaline conditions: Solid-state NMR evidence of the role of surface >MgOH sites. Environ. Sci. Technol. 55, 15082–15089. doi:10.1021/acs.est.1c01247
Rubin, C. H., Noji, E. K., Seligman, P. J., Holtz, J. L., Grande, J., and Vittani, F. (1994). Evaluating a fluorosis hazard after a volcanic eruption. Archives Environ. Health Int. J. 49, 395–401. doi:10.1080/00039896.1994.9954992
Schlesinger, W. H., Klein, E. M., and Vengosh, A. (2020). Global biogeochemical cycle of fluorine. Glob. Biogeochem. Cycles 34, e2020GB006722. doi:10.1029/2020gb006722
Starkey, H. C. (1982). The role of clays in fixing lithium, 1278. Washington, DC: US Government Printing Office.
Sugitani, K. (1996). Anomalously low Al2O3/TiO2 values for archean cherts from the pilbara block, Western Australia—Possible evidence for extensive chemical weathering on the early Earth. Precambrian Res. 80, 49–76. doi:10.1016/s0301-9268(96)00005-8
Symonds, R. B., Rose, W. I., and Reed, M. H. (1988). Contribution of c1-and f-bearing gases to the atmosphere by volcanoes. Nature 334, 415–418. doi:10.1038/334415a0
Taylor, S. R., and McLennan, S. M. (1985). The continental crust: its composition and evolution. Palo Alto, CA: Blackwell Scientific Pub.
Tessier, A., Campbell, P. G., and Bisson, M. (1979). Sequential extraction procedure for the speciation of particulate trace metals. Anal. Chem. 51, 844–851. doi:10.1021/ac50043a017
Wang, N., Zhang, X., He, B., Zhao, F., Jing, C., and Ren, G. (2019). Zircon U-Pb geochronology and geochemistry of the mung bean rocks of the middle Triassic Guanling formation in Dazhai area of Qianxi county, Guizhou Province. Mineral. Pet. 39, 91–100.
Wenzel, W. W., and Blum, W. E. (1992). Fluorine speciation and mobility in F-contaminated soils. Soil Sci. 153, 357–364. doi:10.1097/00010694-199205000-00003
Winchester, J., and Floyd, P. (1977). Geochemical discrimination of different magma series and their differentiation products using immobile elements. Chem. Geol. 20, 325–343. doi:10.1016/0009-2541(77)90057-2
Xiao, J., and Hu, R. (2005). Sedimentary-volcanic tuffs formed during the early Middle Triassic volcanic event in Guizhou Province and their stratigraphic significance. Chin. J. Geochem. 24, 338–344. doi:10.1007/bf02873796
Yan, C., Jiang, H., Lai, X., Sun, Y., Yang, B., and Wang, L. (2015). The relationship between the “green-bean rock” layers and conodont chiosella timorensis and implications on defining the early-middle triassic boundary in the nanpanjiang basin, south China. J. Earth Sci. 26, 236–245. doi:10.1007/s12583-015-0535-x
Yi, C.-Y., Wang, B.-G., and Jin, M.-G. (2013). Fluorine speciation and its distribution characteristics in selected agricultural soils of north China plain. Environ. Sci. 34, 3195–3204.
Zelenski, M., Kamenetsky, V., Taran, Y., and Kovalskii, A. (2020). Mineralogy and origin of aerosol from an arc basaltic eruption: Case study of Tolbachik volcano, Kamchatka. Geochem., Geophys., Geosyst. 21, e2019GC008802. doi:10.1029/2019gc008802
Keywords: fluorine speciation, bentonite, altered volcanic ashes, Sichuan Basin, Early-Middle Triassic
Citation: Lin Y, Zheng M, Zhang Y and Xing E (2023) Fluorine speciation and origin of Early-Middle Triassic bentonite deposits in Sichuan Basin, South China. Front. Earth Sci. 10:1045267. doi: 10.3389/feart.2022.1045267
Received: 19 September 2022; Accepted: 31 October 2022;
Published: 16 January 2023.
Edited by:
Ali Abedini, Urmia University, IranReviewed by:
Maryam Khosravi, Isfahan University of Technology, IranAkram Alizadeh, Urmia University, Iran
Copyright © 2023 Lin, Zheng, Zhang and Xing. This is an open-access article distributed under the terms of the Creative Commons Attribution License (CC BY). The use, distribution or reproduction in other forums is permitted, provided the original author(s) and the copyright owner(s) are credited and that the original publication in this journal is cited, in accordance with accepted academic practice. No use, distribution or reproduction is permitted which does not comply with these terms.
*Correspondence: Yongjie Lin, bGlueW9uZ2ppZTIwMTRAMTYzLmNvbQ==