- 1State Key Lab of Oil and Gas Reservoir Geology and Exploitation, Southwest Petroleum University, Chengdu, Sichuan, China
- 2School of Geoscience and Technology, Southwest Petroleum University, Chengdu, Sichuan, China
- 3Research Institute of Exploration and Development of PetroChina Southwest Oil and Gasfield Company, Chengdu, Sichuan, China
The research on the developmental characteristics and evolution process of pores in dolomite reservoirs is a hot spot in petroleum geology. In this paper, taking the dolomite reservoirs of the Longwangmiao Formation in the Gaoshiti area as an example, the differential distribution and evolution of pore parameters have been systematically studied combined with downhole cores, logging, cast thin sections, and scanning electron microscope data. We used statistical methods and image analysis techniques to quantitatively characterize the pore distribution of the Longwangmiao Formation in the study area. Moreover, the factors controlling the distribution of pores in the dolomite of the Longwangmiao Formation were identified, namely, lithology, sedimentary facies and diagenesis. Finally, the evolution mode of pores of the Longwangmiao Formation was constructed. The results show that the main types of pores developed in the Longwangmiao Formation in the study area include intergranular and intragranular dissolved pores, intercrystalline dissolved pores and mold pores. Among them, the intergranular dissolved pores are the main pore type. The pores with surface porosity higher than 0.5% in the Long 2 Member in the study area account for 46% of the total pores, while those in the Long 1 Member account for 25.5%. Therefore, the degree of development of pores in the Long 2 Member is better than the Long 1 Member. In addition, the granular dolomite in the grain shoal facies is favorable for the formation of large-scale pores, and the oolitic dolomite is the most favorable reservoir type. The most favorable factors for the development of pores are the atmospheric freshwater dissolution and dolomitization in the quasi-contemporaneous period; the former caused the formation of a large number of intergranular dissolved pores, while the dolomitized particles and their ring-edge cements in the latter promoted the retention of residual intergranular dissolved pores. The dissolution, dolomite cementation and hydrothermal action in the burial stage promoted the reformation of pores.
1 Introduction
In recent years, breakthroughs have been made in marine carbonate oil and gas exploration in the Sichuan Basin of China. For example, the largest marine carbonate monolithic gas reservoir in China, the Anyue Gas Field, has been discovered in the Gaomo area (Katz et al., 2021; Niu et al., 2022). Fine and quantitative characterization of pore structures is the key to study reservoir performance in gas reservoirs. This research mainly focuses on the type, shape and distribution of pores, pore structures and pore connectivities, as well as the qualitative and quantitative studies on the genesis and evolution mode of pores (Kun et al., 2014). Techniques for qualitative characterization of pores generally include cast thin sections, scanning electron microscopy, cathodoluminescence, and X-ray diffraction analysis (Franziska et al., 2021; Xiangchun et al., 2021; Jiang et al., 2022). They can be used to directly observe and describe the types and morphological characteristics of pores. However, for the quantitative characterization of pores, it generally needs to combine high-pressure mercury intrusion, gas adsorption, nuclear magnetic resonance, CT, image analysis software, etc. (Qiang et al., 2021; Cheng et al., 2022) to finely describe the distribution characteristics of pores with full pore size (Fenshu et al., 1995; Nan, 2020; Qiang et al., 2021; Vafaie et al., 2021).
At present, the research on the Lower Cambrian carbonate oil and gas reservoirs in the Sichuan Basin mainly focuses on the Longwangmiao Formation (Jinhu et al., 2014; Xi et al., 2017). According to previous studies, the shoal facies reservoirs developed in the Longwangmiao Formation are mainly hosted in sandy dolomite and oolitic dolomite, and the reservoir space is dominated by pores, caves and fractures of secondary origin, and its physical properties are characterized by medium-low porosity and low permeability (Da et al., 2022). However, there are still some problems that need to be improved: 1) Existing studies have ignored lithology and sedimentary facies as the main controlling factors of reservoirs (Zitong, 2007); 2) The effects of diagenesis, such as dissolution, dolomitization and dolomite cementation, on the development and evolution of reservoir pores at different diagenetic stages have not been elucidated (Da et al., 2021; Xing et al., 2021). In this paper, taking the dolomite reservoirs of the Longwangmiao Formation in the Gaoshiti area as an example, the differential distribution and evolution of pore parameters have been systematically studied combined with downhole cores, logging, cast thin sections, scanning electron microscope data, statistical methods and image analysis techniques. This study can provide new ideas for hydrocarbon exploration in the dolomite reservoirs of the Longwangmiao Formation.
2 Geological background
The study area is located in the Gaoshiti area in the central part of the Sichuan Basin. Its geographical location is located in the Ziyang City and the Tongnan County (Guoqi et al., 2015). This area belongs to the Leshan-Longnüsi paleo-uplift area in the central part of the Sichuan Basin (Caineng et al., 2014; Roberts, 2018; Yang et al., 2022) (Figure 1).
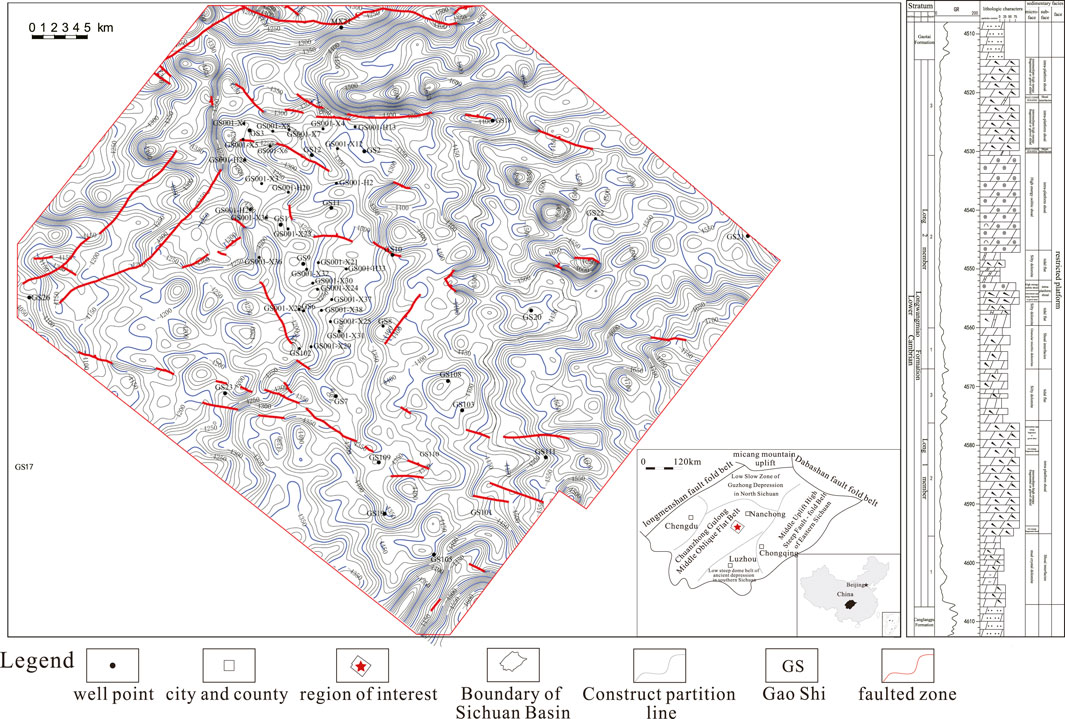
FIGURE 1. Location, structure, well distribution and division of stratigraphic units in the study area.
The Lower Cambrian Longwangmiao Formation in the study area are in integrated contact with the overlying Gaotai Formation and the underlying Canglangpu Formation. From bottom to top, the Longwangmiao Formation is divided into the Long 1 and Long 2 Members. Sandy dolomite, mud (powder) crystalline dolomite, clot dolomite and oolitic dolomite are mainly developed in the Long 1 Member; while the sand dolomite and oolitic dolomite are mainly developed in the Long 2 Member (Xuefei et al., 2015; Benjian et al., 2018). The strata of the Longwangmiao Formation in the study area show a gradually thickening trend from northwest to southeast. The Longwangmiao Formation in this area belongs to the limited platform sediments. The lower part of the Long 1 Member is the thick intra-platform shoal and the inter-shoal sea sediment, and the upper part is shallower and has a tidal flat environment. The lower part of the Long 2 Member is dominated by sandy shoal-oolitic shoal, inter-shoal sea-tidal flat-intra-platform shoal, and tidal flat deposits are mainly developed in the upper part (Genshun et al., 2013; Weiqiang et al., 2022). Pores are mostly developed in the granular dolomite in the upper part of Long 1 Member and the middle and upper part of Long 2 Member, and the pore types are mainly secondary pores (Choquette and Pray, 1974; Lucia, 1995). At present, it is believed that the distribution of Longwangmiao Formation reservoirs is relatively scattered, and most of them are concentrated in the Gaoshi 6∼Gaoshi 102 well area in the middle of the work area (Anjiang et al., 2017; Hongliu et al., 2018).
3 Methods
In this paper, taking the Cambrian Longwangmiao Formation dolomite in the Gaoshiti area as an example, we carried out core observations, casting thin sections and scanning electron microscope experiments. Cores from the Longwangmiao Formation with a total of 345 m from eight wells in the study area were used for core observations. Cast thin sections were used for observations under polarized light microscopy. Its purpose is to obtain the lithology, pore types, pore size, and surface porosity parameters of pores or cavities. A total of 367 cast thin sections were used for the extraction of pore parameters. The differences in the development and distribution of pores in the Longwangmiao Formation of different wells are compared by combining the constraints of stratigraphy, lithology, natural gamma, resistivity and sedimentary facies type.
The statistical methods and image analysis techniques were used to quantitatively characterize the pore distribution of the Longwangmiao Formation in the study area. Moreover, the factors controlling the distribution of pores in the dolomite of the Longwangmiao Formation were identified, namely, lithology, sedimentary facies and diagenesis. Finally, the evolution mode of pores of the Longwangmiao Formation was constructed.
4 Results
4.1 Pore types
The main types of pores developed in the Longwangmiao Formation in the study area include intergranular dissolved pores, intragranular dissolved pores, intercrystalline dissolved pores, intercrystalline pores, mold pores, and dissolved pores/cavities.
4.1.1 Intergranular dissolved pores
Intergranular dissolved pores are mainly developed in granular dolomite such as sandy dolomite and oolitic dolomite. This type of pores are usually irregular and curved pores with scattered distribution and good pore connectivity, and the pore diameters are distributed between 0.01 mm and 1 mm (Figures 2A,B). Under the microscope, the intergranular dissolved pores formed in the later stage are mostly half or fully filled with asphalt and dolomite.
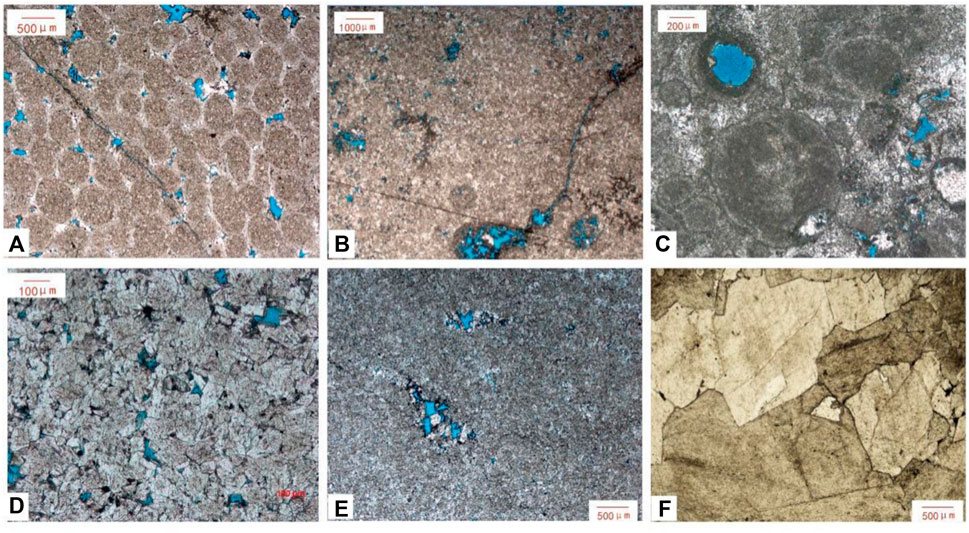
FIGURE 2. Development characteristics of different pore types in the Longwangmiao Formation in the study area. (A) Intergranular dissolved pores, oolitic dolomite, Well GS6, 4546.25, single polarized light; (B) Intergranular and intragranular dissolved pores, sandy dolomite, 4662.5 m, Well GS23, single polarized light; (C) Mold pore, sand dolomite, well GS7, 4635.55 m, single polarized light; (D) Intercrystalline pores and intercrystalline dissolved pores, sugar-like fine-crystalline dolomite, Well GS11, 4571.57 m, single polarized light; (E) Intercrystalline dissolved pores, powder crystal dolomite, Well GS11, 4584.22 m, single polarized light; (F) Intracrystalline dissolved pores, giant crystal dolomite, well MX12, 4637.41–4637.84 m, single polarized light.
4.1.2 Intragranular dissolved pores
Intragranular dissolved pores are irregular in shape (curved edges) and mostly exist in the isolated form. Moreover, the isolated intragranular dissolved pores and intergranular dissolved pores with poor connectivity are associated with each other. Together, they constitute the storage space of granular dolomite (Figure 2B). The pore diameter of intragranular dissolved pores is mainly between 0.01 and 0.5 mm, and some of the pores are semi-filled with bright crystal dolomite or asphalt.
4.1.3 Mold pores
Mold pores are usually developed in granular dolomite, and their shape and size depend on the shape of the particles and the degree of dissolution. The mold pores are generally regular oval or circular, and the maximum diameter of the pores does not exceed 0.2 mm. This type of pores are mostly distributed in isolated form, with extremely poor connectivity, and semi-filled asphalt is occasionally seen (Figure 2C).
4.1.4 Intercrystalline pores
Intercrystalline pores mainly appear in sugar-like dolomite whose original rock structures have been destroyed. They have straight boundaries and are mostly developed between dolomite crystals. The pore diameter is mostly less than 0.5 mm. It was observed under the microscope that the larger the dolomite crystal, the higher the degree of eumorphism, and the more developed the intercrystalline pores (Figure 2D).
4.1.5 Intergranular dissolved pores
Intercrystalline dissolved pores coexist with intercrystalline pores. The straight boundaries are partially eroded into irregular curved or microdentate morphologies. In addition, the pore diameter is mostly less than 0.5 mm, and the surface porosity is usually between 0.1% and 0.5% (Figure 2E). Microscopic observation showed that the intergranular dissolved pores were mostly half or fully filled with asphalt.
4.1.6 Intracrystalline dissolved pores
Intragranular dissolved pores are generally isolated on the surface of dolomite crystals. Its morphology is relatively regular and the pores are not connected (Figure 2F). Most of the intragranular dissolved pores have a pore size ranging from 1.5 μm to 4 μm. However, some of this type of pores can also be observed with naked eyes. Under the scanning electron microscope, it was observed that some dissolved pores in the crystals were half filled with nanocrystals, so that the porosity was reduced to a certain extent.
4.2 Longitudinal pore distribution characteristics
The pores of the Longwangmiao Formation in the study area are well developed in the Long 2 Member. They are mainly developed in the oolitic dolomite and sandy dolomite of granular shoal facies, and the pore types are mainly intergranular dissolved pores. From bottom to top, the content of intragranular dissolved pores decreases, the content of intergranular dissolved pores increases, the content of dolomite in the first stage decreases, while that in the second stage increases, and the total surface porosity decreases. For horizons, pores with surface porosity higher than 0.5% in the Long 2 Member account for 46% of total pores, while pores with surface porosity higher than 0.5% in the Long 1 Member account for 25.5% of total pores.
In terms of lithology, the average surface porosity of pores in oolitic dolomite and sandy dolomite is higher, which are 2.8% and 1.5%, respectively. In terms of lithofacies, the average surface porosity of pores in oolitic shoal and sandy shoal is higher, which are 2.8% and 2.7%, respectively. In terms of pore types, intergranular dissolved pores accounted for 74.8% of the pores developed in granular dolomite, and intergranular dissolved pores accounted for 72.6% of the pores developed in granular dolomite.
In the entire Longwangmiao Formation, intergranular dissolved pores account for a relatively high proportion, accounting for about 60% of the total pores in the Long 1 Member, and about 65% of the Long 2 Member. For the fillings inside the pores, the average content of the cement formed in the second stage is 55.6%, and that formed in the first stage is 43.4%. In the case of high quartz content, even if the intergranular dissolved pores and the first-stage cement content are high, the rock face porosity is low (Figure 3).
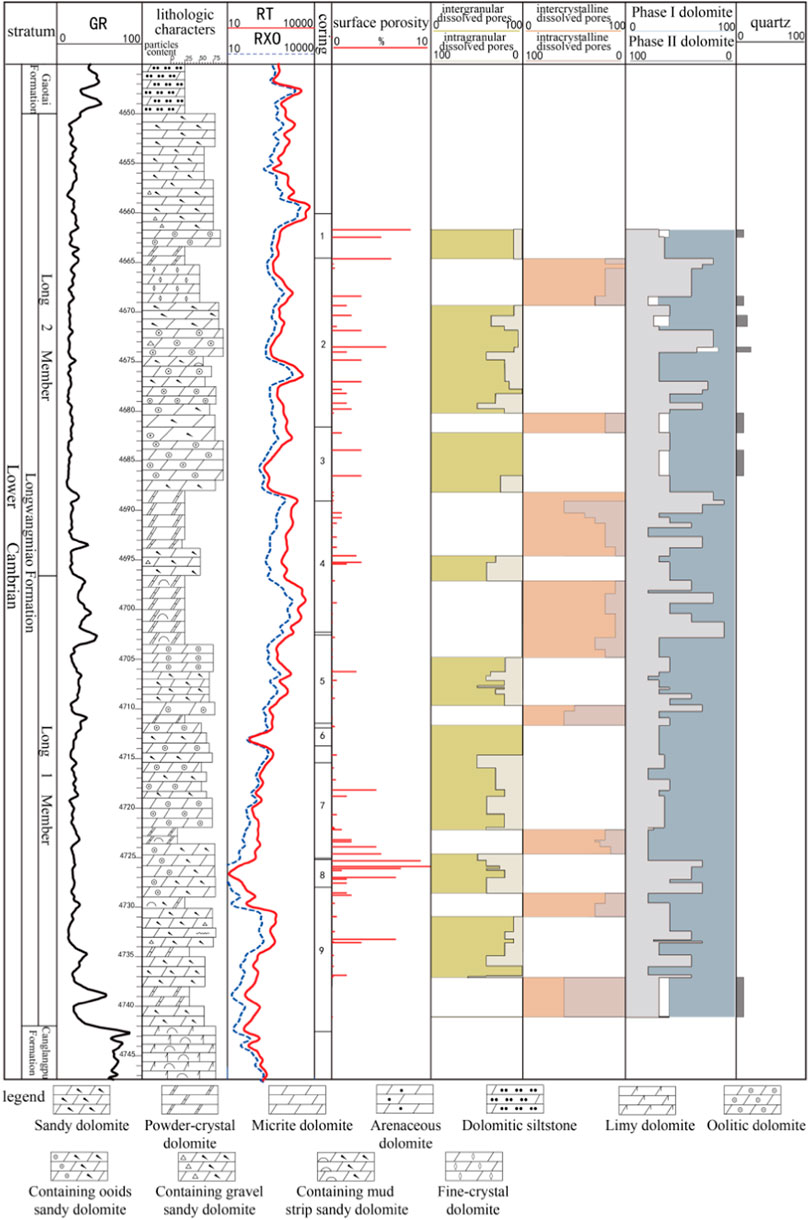
FIGURE 3. Development characteristics of pores of the Longwangmiao Formation in Well Gaoshi 23 in the study area.
4.3 Lateral distribution of pores
The surface porosity of the Longwangmiao Formation in the study area is proportional to the thickness of the granular shoal, intergranular dissolved pores and the content of the first-phase cements; however, it is inversely proportional to the second-phase cements and the quartz.
From west to east, the stratigraphic thickness of the granular shoal in the Longwangmiao Formation tends to decrease, while the proportion of the intergranular dissolved pores tends to decrease. At the same time, in the pore-developed section, the content of cements in the first stage decreased, while the cements in the second stage increased, and the surface porosity gradually decreased from 1.6% to 0.6%. From south to north, the granular shoal shows a thickening trend, and the proportion of dissolved pores between grains shows an upward trend. At the same time, in the pore-developed section, the content of cements in the first stage increased, while the cements in the second stage decreased, and the surface porosity gradually increased from 1.2% to 1.9% (Figure 4). It is revealed that the surface porosity of the pores of the Longwangmiao Formation in the study area is closely related to the content of grains, intergranular dissolved pores, two-phase cements, and quartz.
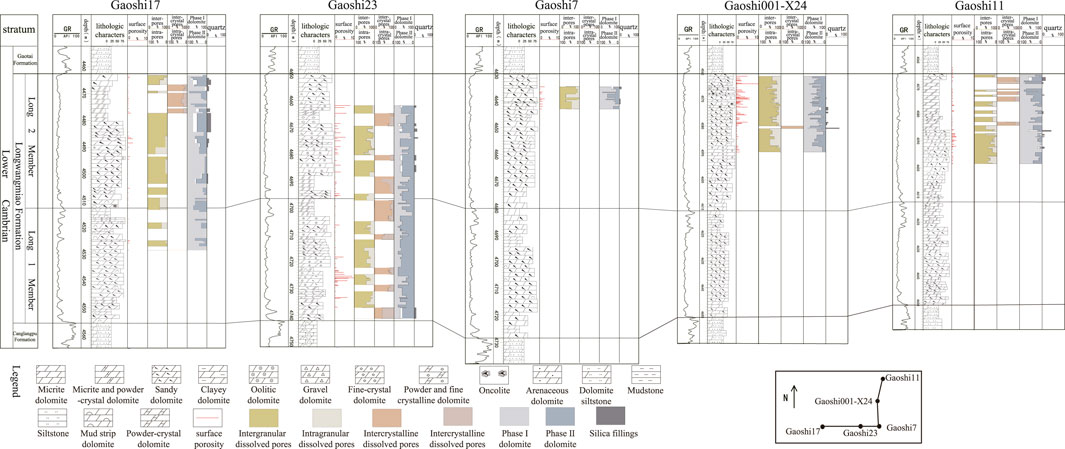
FIGURE 4. Comparison of pore development characteristics of the Longwangmiao Formation in the study area through Wells Gaoshi 17-Gaoshi 23-Gaoshi 7-Gaoshi 001-X24 -Gaoshi 11.
5 Discussion
5.1 Influence of lithology on differential distribution of pores
The pore distribution is related to the content, size and shape of the particles. Granular dolomite is the main reservoir rock type of the Longwangmiao Formation in the study area. Among them, the development degree of pores in the oolitic dolomite is higher than that in sandy dolomite (Figure 5), and the thickness of the granular dolomite in the Long 2 Member is thicker than that of the Long 1 Member. The pore distribution of the Longwangmiao Formation dolomite has significant vertical differences.
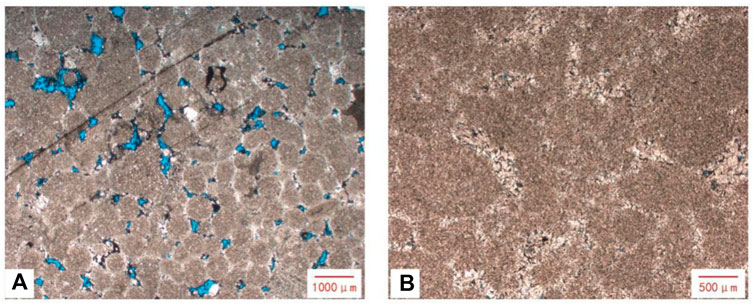
FIGURE 5. Microscopic characteristics of different types of granular pores in the Longwangmiao Formation in the study area. (A) Oolitic dolomite pores, GS6, 4546.78–4546.88 m, single polarized light; (B) Sandy dolomite pores, GS17, 4505.2 m, single polarized light.
At the same time, in the granular dolomite, the oolitic dolomite samples with a surface porosity greater than 0.5 accounted for 69%, the sandy dolomite samples with a surface porosity greater than 0.5 accounted for 52%, and the oolitic dolomite had a higher surface porosity than the sandy dolomite (Figures 6A,B). In the grain dolomite, the samples with a surface porosity greater than 0.5 accounted for 36% of the total samples; in the crystalline dolomite, the samples with a surface porosity greater than 0.5 accounted for 4% of the total samples; the grain dolomite had a higher face porosity than the crystalline dolomite (Figure 6). The proportion of oolitic dolomite in the Long 2 Member with a surface porosity greater than 0.5% is 62%; while the samples with a surface porosity greater than 0.5% in the Long 1 Member is 7%. The proportion of sandy dolomite samples with surface porosity greater than 0.5% in the Long 2 Member is 44%; while the samples with surface porosity greater than 0.5% in the Long 1 Member is 8%.
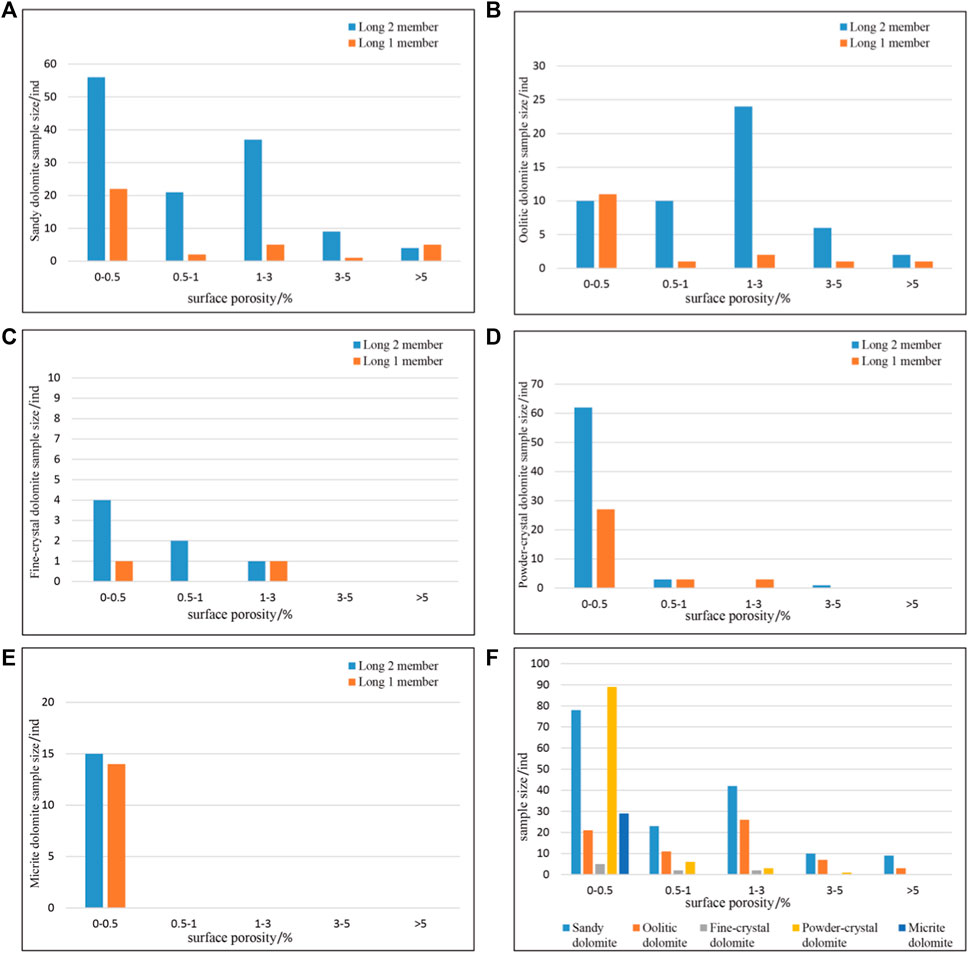
FIGURE 6. Histogram of surface porosity distribution of various lithologies of the Longwangmiao Formation in the study area. (A) Sandy dolomite; (B) Oolitic dolomite; (C) Fine-crystal dolomite; (D) Powder-crystal dolomite; (E) Micrite dolomite; (F) Histogram of surface porosity distribution in the Longwangmiao Formation.
The average total surface porosity of various lithologies is arranged in descending order: oolitic dolomite1.9%, sandy dolomite 1.51%, fine crystal dolomite 0.93%, powder crystal dolomite 0.38% and micrite dolomite 0.2% (Figures 6A–F). According to the above analysis, most of the high-porosity reservoirs of various lithologies are distributed in the Long 2 Member, so the development of pores in the Long 2 Member is good. In the same horizon, the proportion of surface porosity of different lithologies is significantly different. Granular dolomite is the main reservoir rock in the Longwangmiao Formation in the study area, and oolitic dolomite has the best development of pores.
5.2 Influence of sedimentary facies on differential distribution of pores
The distribution of pores is controlled by sedimentary facies. The granular shoal is the main reservoir lithofacies of the Longwangmiao Formation in the study area. Among them, the oolitic shoal is the most developed, and its development thickness in the Long 2 Member is higher than that of the Long 1 Member. Therefore, the development of pores has an obvious horizon differences.
Statistics show that the samples with a surface porosity greater than 0.5% in the granular shoal accounted for 36% of the total samples; the samples with a surface porosity greater than 0.5 in the dolomitic flat and the intershoal sea accounted for 4% of the total samples (Figure 7E). At the same time, the proportion of samples with oolitic shoal facies greater than 0.5 was 69%; while the proportion of samples with sandy shoal facies greater than 0.5% was 52% (Figures 7A,B).
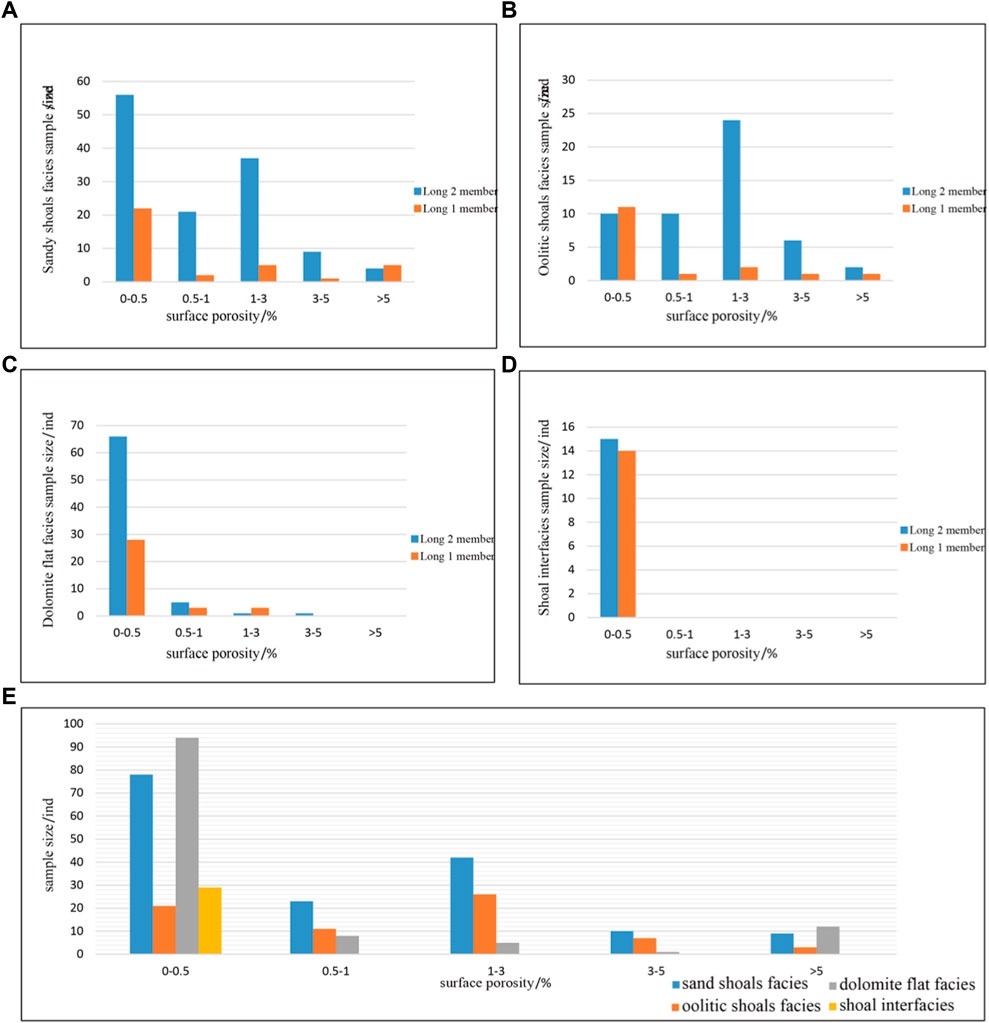
FIGURE 7. Histogram of surface porosity distribution of various lithofacies of the Longwangmiao Formation in the study area. (A) Histogram of surface porosity distribution of sandy shoals facies; (B) Histogram of surface porosity distribution of oolitic shoals facies; (C) Histogram of surface porosity distribution of dolomite flat facies; (D) Histogram of surface porosity distribution of shoal interfacies; (E) Histogram of surface porosity distribution in the Longwangmiao Formation.
The average total surface porosity of each lithofacies is arranged in descending order: oolitic shoal 1.9%, sandy shoal 1.22%, dolomitic flat 0.38% and shoal interfacies 0.2% (Figures 7A–E). According to the analysis, most of the high-porosity reservoirs of various lithofacies are distributed in the Long 2 Member, so the Long 1 Member reservoir has a good degree of pore development. In the same horizon, the proportion of face porosity of different lithofacies is obviously different. The granular shoal is the main reservoir lithofacies in the Longwangmiao Formation in the study area, and the oolitic shoal, as the main reservoir lithofacies in the Longwangmiao Formation, has the best degree of pore development.
5.3 Influence of diagenesis on differential distribution of pores
The dolomite of the Longwangmiao Formation in the study area has experienced a complex diagenetic system during the long burial process (Mindong et al., 2014; Haas et al., 2017; Mengyan et al., 2017; Pajdak et al., 2017; Qiang et al., 2021).
Among them, the main diagenesis controlling the development of pores is the dissolution of atmospheric fresh water between dolomite particles, dolomitization, dolomite cementation and hydrothermal action (Feifei, 2018). Tectonic activities promote the formation of tectonic fractures. These fractures aremostly filled with siliceous minerals such as dolomite and quartz and asphalt in the late diagenetic stage (Jingjie et al., 2020; Lingfang et al., 2020; Ying et al., 2019; Ke et al., 2022).
5.3.1 Dissolution
The dissolution of the dolomite in the Longwangmiao Formation in the study area includes the atmospheric freshwater dissolution in the paracontemporaneous period and the burial dissolution in the mesodiagenetic stage. Atmospheric freshwater dissolution is the main factor controlling the development of intergranular and intragranular dissolved pores in granular dolomite. They are positively correlated with the surface porosity (Figures 8C,D), and the positive correlation between the intergranular dissolved pores and the surface porosity is better. Intercrystalline and intracrystalline dissolved pores are developed in crystalline dolomite, and the correlation between these two types of dissolved pores and surface porosity is not obvious (Figures 8A,B). Therefore, dissolution in crystalline dolomite is not the main factor controlling pore distribution. Burial dissolution usually results in enlarged dissolution of structural fractures, but they are usually partially or fully filled with bitumen (Figure 9C). Therefore, the correlation between tectonic fractures and surface porosity is poor (Figure 8E).
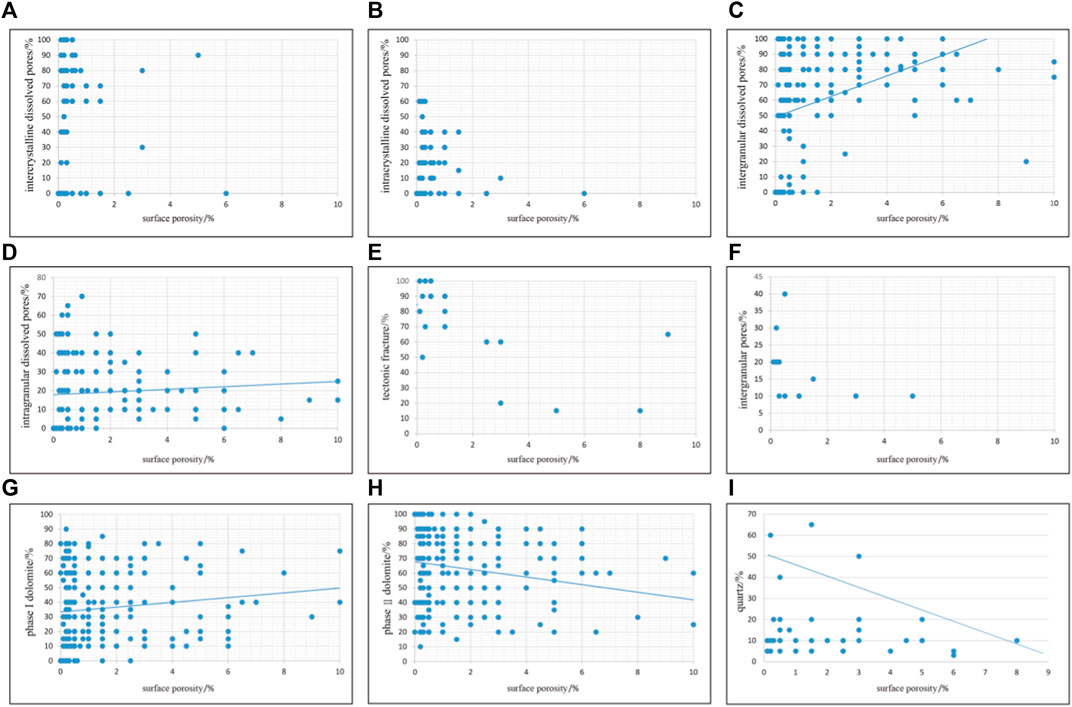
FIGURE 8. Surface porosity distribution under various diagenesis of the Longwangmiao Formation in the study area. (A) Relationship between intercrystalline dissolved pores and surface porosity; (B) Relationship between intracrystalline dissolved pores and surface porosity; (C) Relationship between intergranular dissolved pores and surface porosity; (D) Relationship between intragranular dissolved pores and surface porosity; (E) Relationship between tectonic fracture and surface porosity; (F) Relationship between intergranular pores and surface porosity; (G) Relationship between phase I dolomite and surface porosity; (H) Relationship between phase Ⅱ dolomite and surface porosity; (I) Relationship between hydrothermal quartz and surface porosity.
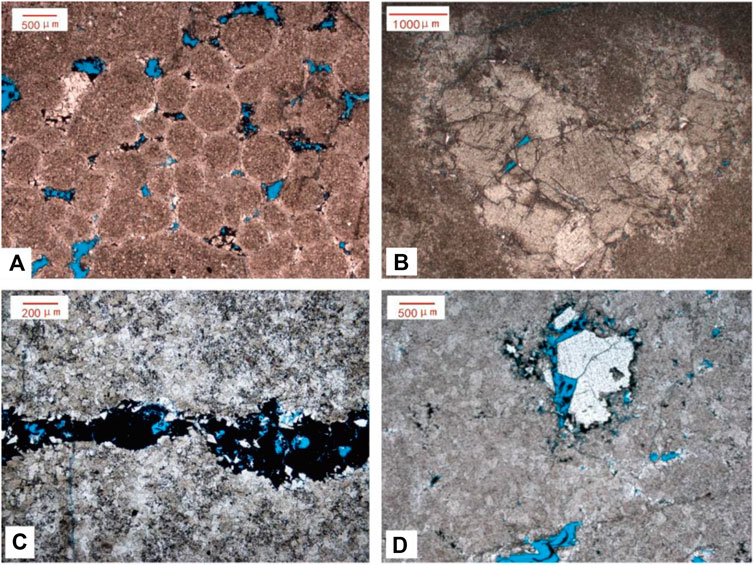
FIGURE 9. Diagenesis types and characteristics of the Longwangmiao Formation in the study area. (A) The first-stage annular dolomite cement developed around the grains, Well GS6, 4545.49 m, single polarized light; (B) Pores filled with medium and coarse-grained cements from the second stage, Well GS23, 4691.65 m, single polarized light; (C) Structural dissolving fracture nearly completely filled with dolomite and asphalt, Well MX16, 4755.47 m, single polarized light; (D) Pore filled with hydrothermal quartz, well GS001-X24, 4580.27 m, single polarized light.
5.3.2 Dolomitization and dolomite cementation
The dolomite developed in the target layer in the study area is generally the product of dolomitization and dolomite cementation. This study shows that dolomitization and dolomite cementation have different effects on pore development at different diagenetic stages (Dawei et al., 2021): ① Quasi-contemporaneous evaporative seawater stage: This stage represents the main stage of dolomitization. Affected by strong evaporation and reflux infiltration, dolomitization occurs in high-porosity, high-permeability limestone. A large number of intercrystalline pores are developed in crystalline dolomite (Lianqiang et al., 2019), but according to statistics, there is no correlation between intercrystalline pores and surface porosity (Figure 8F). At the same time, in granular dolomite, dolomitization led to the replacement of calcite cements around intergranular dissolved pores with dolomite. Therefore, the first-phase powder-fine-grained dolomite metasomatism has a good positive correlation with surface porosity (Figure 8G). They support and protect the residual pores (Figure 9A); ② In the early diagenetic stage, the cementation of dolomite resulted in the formation of the second-stage fine-medium crystalline dolomite cements in the intergranular dissolved pores, and some of the pores were filled (Figure 9B). The content of fine-medium crystalline dolomite cements in the second stage is negatively correlated with the surface porosity (Figure 8H); ③ In the middle diagenetic stage, dolomite cementation continued, and coarse-megacrystalline dolomite was occasionally seen.
5.3.3 Hydrothermal action
In the late diagenetic stage, the dissolution of the deep burial environment promotes the expansion dissolution of the fractures and dissolved pores. Afterwards, siliceous minerals such as quartz are formed through hydrothermal action and fill the pores. According to statistics, quartz composition is negatively correlated with surface porosity (Figure 8I). Therefore, siliceous minerals such as quartz formed by hydrothermal action severely block the pores, thereby reducing the porosity of the rock (Figure 9D).
5.4 Diagenetic sequence and pore evolution
According to the analysis of the pore development characteristics and controlling factors of the Longwangmiao Formation, the diagenetic evolution of the reservoir and the development law of pores are obtained (Chilingar and Bissell, 1966; Davies and Smith, 2006; Mindong et al., 2014).
(1) Normal seawater stage of quasi-contemporaneous period: The Longwangmiao Formation carbonate rocks in the study area were formed in a seawater diagenetic environment. The earliest bright-crystalline fibrous calcite cements were formed under the action of cementation. They grow ring-like around the grain edges and destroy the primary intergranular pores, leading to a dramatic drop in porosity (Figure 10A).
(2) Quasi-contemporaneous atmospheric freshwater stage: It represents a sedimentary environment in which the sea level is gradually decreasing. At this time, the sediments of the granular shoal such as sandy shoal and oolitic shoal on the sedimentary high landform were briefly exposed to the atmospheric sedimentary rock environment. The reservoir is subjected to selective dissolution by freshwater leaching, and a large number of intergranular dissolved pores and a small amount of intragranular dissolved pores are formed. The development of dissolved pores improves the overall porosity of the study area (Figure 10B).
(3) Quasi-contemporaneous evaporating seawater stage: Evaporation causes the magnesium-rich fluids to permeate and flow downward, and all the interstitials and particles are dolomized. At this stage, the early calcite cement was replaced by dolomite, and the first-stage dolomite began to form in the intergranular dissolved pores. The edges of the intergranular pores began to precipitate clean straight euhedral powder-crystalline dolomite, and fine-grained rhombic dolomite cements were partially visible (Figure 10C). The dolomite developed at the edge of the first-stage ring grains can support and protect the residual pores to a certain extent.
(4) Early diagenetic stage: At this stage, the reservoir begins to enter a shallow burial environment. However, due to the support and protection of the first-stage dolomite, the pore structures were not destroyed. But at this time, dolomite cementation occurs again, so that fine-medium-crystalline euhedral rhombic dolomite cements are generally formed in the pores. They continue to fill intra-particle, inter-particle pores and cause a dramatic reduction in porosity. In addition, weak structural rupture also occurred, and a small number of structural fractures were formed (Figure 10D).
(5) Middle diagenetic stage: As the depth increases, the reservoir enters a medium-deep burial environment. Further dolomite cementation occurs at this stage, and coarse-crystalline and giant-crystalline dolomite cements are formed. At this stage, pressure-dissolution begins to occur, and a large number of sutures begin to develop. A small number of fractures were formed by tectonic action, but the fractures were mostly filled with dolomite or asphaltene at a later stage (Figure 10E). Therefore, fractures have little effect on the reservoir performance of the reservoir. Overall, the porosity at this stage continued to decrease.
(6) Late diagenetic stage: In deep burial environments, intergranular dissolved pores, structural fractures and their internal fillings will further develop under the action of hydrothermal fluids and acidic fluids (Qilong et al.,2020). However, cements such as euhedral saddle-shaped dolomite and euhedral quartz formed by hydrothermal action and asphalt formed by oil and gas cracking are partially or fully filled (Figure 10F). At this stage, the porosity reaches a minimum value.
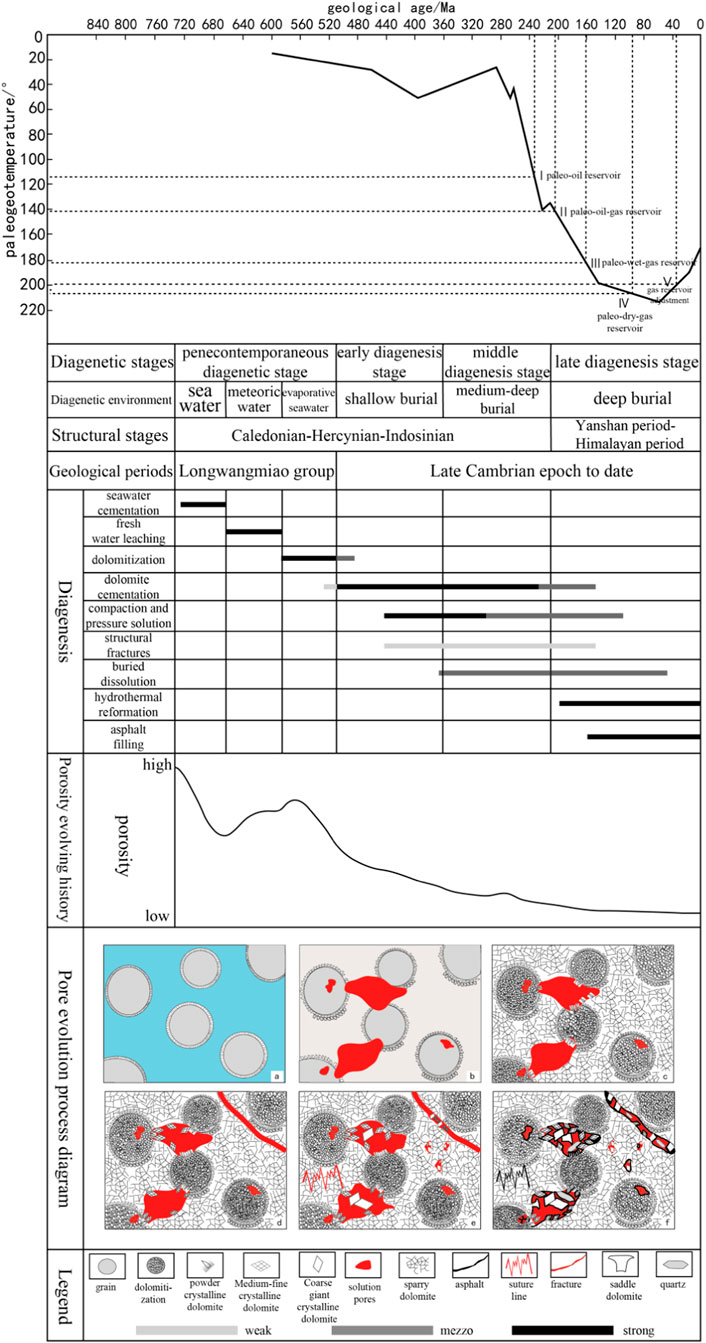
FIGURE 10. Schematic diagram of the burial history, diagenesis sequence and pore evolution of the Longwangmiao Formation in the study area. (A) is the Normal seawater stage of quasi-contemporaneous period, (B) is the Quasi-contemporaneous atmospheric freshwater stage, (C) is the Quasi-contemporaneous seawater stage, and (D) is the Early seawater stage. (E) is Middle stage, (F) is Late stage.
6 Conclusion
(1) The factors controlling the distribution of pores in the dolomite of the Longwangmiao Formation in the Gaoshiti area were identified, namely, lithology, sedimentary facies and diagenesis, and in turn, the pore evolution model of the Longwangmiao Formation was constructed.
(2) The main types of pores developed in the Longwangmiao Formation in the study area include intergranular dissolved pores, intragranular dissolved pores, intracrystalline dissolved pores and mold pores\. Among them, intergranular dissolved pores are the main pore type.
(3) The proportion of pores with surface porosity greater than 0.5% in the Long 2 Member in the study area is 46%, while that in the Long 1 Member is 25.5%. Therefore, the degree of development of pores in the Long 2 Member is better than that of the Long 1 Member. In addition, the grain dolomite in the grain shoal facies is favorable for the formation of large-scale pores, and the oolitic dolomite is the most favorable reservoir type.
(4) The development of pores in the Longwangmiao Formation in the study area is controlled by lithology and sedimentary facies. The average porosity of various lithologies is: oolitic dolomite 1.9%, sandy dolomite 1.51%, fine crystal dolomite 0.93%, powder crystal dolomite 0.38%, and micrite dolomite 0.2%. Moreover, the average porosity of different sedimentary facies is: 1.9% for oolitic shoals, 1.22% for sandy shoals, 0.38% for dolomitic flats, and 0.2% for shoal interfacies.
(5) The development of pores in the Longwangmiao Formation in the study area is controlled by different diagenesis in different stages: ① In the normal seawater stage of the quasi-contemporaneous period, seawater cementation caused the formation of calcite cements at the edge of the earliest ring grains, and the destruction of primary pores led to a sharp decrease in porosity; ② In the atmospheric freshwater stage of the quasi-contemporaneous period, the granular shoal sediments were leached by freshwater in the atmospheric sedimentary environment, and a large number of intergranular dissolved pores were formed; ③ During the evaporative seawater stage of the quasi-contemporaneous period, the dolomitization caused by strong evaporation and reflux infiltration resulted in the formation of dolomite cements at the edge of the ring grains. Under the support of cements, a large number of residual pores are retained; ④ In the early diagenetic stage, the dolomite cementation promoted the formation of the second-stage fine-medium crystalline dolomite cements in the shallow burial environment, and then, a large number of pores were filled and the porosity decreased sharply; ⑤ In the middle diagenetic stage, further dolomite cementation in the middle-deep burial stage resulted in the formation of coarse-megacrystalline dolomite. At this time, almost all the pores are filled, and the porosity continues to decrease; ⑥ In the late diagenetic stage, the saddle-shaped dolomite, quartz and other minerals are formed under the action of deep burial environment and hydrothermal fluids, and the pores and fractures are partially or fully filled with bitumen formed along with oil and gas migration, and the final reservoir porosity reaches the lowest level.
Data availability statement
The original contributions presented in the study are included in the article/supplementary material, further inquiries can be directed to the corresponding author.
Author contributions
HQ and ZY are responsible for the idea and writing of this paper, XZ and ML are responsible for the data interpretation and CZ, WX, FH, and QS are responsible for sample collection and data testing.
Funding
This research was financially supported by the National Natural Science Foundation of China (41702163 and 41702122) and the Science and Technology Cooperation Project of the CNPC-SWPU Innovation Alliance (No. 2020CX010301).
Conflict of interest
The authors declare that the research was conducted in the absence of any commercial or financial relationships that could be construed as a potential conflict of interest.
Publisher’s note
All claims expressed in this article are solely those of the authors and do not necessarily represent those of their affiliated organizations, or those of the publisher, the editors and the reviewers. Any product that may be evaluated in this article, or claim that may be made by its manufacturer, is not guaranteed or endorsed by the publisher.
References
Anjiang, S., Anping, H., Liyin, P., and Min, P. (2017). Origin and distribution of grain dolostone reservoirs in the cambrian Longwangmiao Formation, Sichuan Basin, China. Acta Geol. Sin. 91 (1), 13072. doi:10.1111/1755-6724.13072
Benjian, Z., Di, X., Xingzhi, W., Like, Z., Sicong, L., and Xun, Y. (2018). Sedimentary characteristics and distribution patterns of grain shoals in the lower cambrian Longwangmiao Formation, southern Sichuan Basin, SW China. Arab. Geosci. 11, 135. doi:10.1007/s12517-018-3486-6
Caineng, Z., Jinhu, D., Chunchun, X., Zecheng, W., Baomin, Z., Guoqi, W., et al. (2014). Formation, distribution, resource potential, and discovery of Sinian–Cambrian giant gas field, Sichuan Basin, SW China. Petroleum Explor. Dev. 41 (03), 306–325. doi:10.1016/S1876-3804(14)60036-7
Cheng, Z., Yong, S., Yiren, F., Peiqiang, Y., Xinmin, G., Fei, W., et al. (2022). Application and prospect of low-field nuclear magnetic resonance technology in accurate characterization of coal pore structure. J. China Coal Soc. 47 (02), 828–848. doi:10.13225/j.cnki.jccs.xr21.1766
Chilingar, G. V., and Bissell, H. J. (1966). Dolomitization and limestone diagenesis. A symposium. Chem. Geol. 1, 272–275. doi:10.1016/0009-2541(66)90022-2
Choquette, P. W., and Pray, L. C. (1974). Geological nomenclature and classification of porosity in sedimentary carbonates. AAPG Bull. 54, 207–250.
Da, G., Mingmin, W., Ye, T., Lili, H., Chunyan, S., Xinmiao, H., et al. (2022). Control of sea level changes on high-frequency sequence and sedimentary evolution of Lianglitage Formation in the Tazhong Area. Geol. China, 1, 19
Da, G., Mingyi, H., Anpeng, L., Wei, Y., Wuren, X., and Chunyan, S. (2021). High-Frequency sequence and microfacies and their impacts on favorable reservoir of Longwangmiao Formation in central Sichuan Basin. Earth Sci. 46 (10), 3520–3534. doi:10.3799/dqkx.2020.382
Davies, G. R., and Smith, L. B. (2006). Structurally controlled hydrothermal dolomite reservoir facies: An overview. Am. Assoc. Pet. Geol. Bull. 90 (11), 1641–1690. doi:10.1306/05220605164
Dawei, L., Chunfang, C., Yongjie, H., Yanyan, P., and Lei, J. (2021). Multistage dolomitization and Formation of ultra-deep lower cambrian Longwangmiao Formation reservoir in central Sichuan Basin, China. Mar. Petroleum Geol. 123, 104752. doi:10.1016/j.marpetgeo.2020.104752
Feifei, Z. (2018). Reservoir properties and controlling factors of dolomite in Longwangmiao Formation, northeastern Sichuan Basin. Beijing: D. China University of Petroleum.
Fenshu, J., Pingping, S., and Kewen, L. (1995). Fractal characteristics of sandstone pore structure and its application. Fault-Block Oil Gas Field 1, 16–21.
Franziska, S., Liwen, H., Pierre, D., Xianghui, X., Donald, L., and Smith, O. M. (2021). Synchrotron X-ray microtomography and multifractal analysis for the characterization of pore structure and distribution in softwood pellet biochar. Biochar 3 (4), 671–686. doi:10.1007/S42773-021-00104-3
Genshun, Y., Jingao, Z., Weihong, Z., Jianyong, Z., Liyin, P., Yi, H., et al. (2013). Characteristics and distribution rule of lower cambrian Longwangmiao grain beach in Sichuan Basin. Mar. Orig. Pet. Geol. 18 (04), 1–8. doi:10.3969/j.issn.1672-9854.2013.04.001
Guoqi, W., Jinhu, D., Chunchun, X., Can, Z., Wei, Y., Ping, S., et al. (2015). Characteristics and accumulation modes of large gas reservoirs in Sinian-Cambrian of Gaoshiti-Moxi region, Sichuan Basin. Acta Pet. Sin. 36 (01), 1–12. doi:10.7623/syxb201501001
Haas, J., Hips, K., Budai, T., Gyori, O., Lukoczki, G., Kele, S., et al. (2017). Processes and controlling factors of polygenetic dolomite Formation in the transdanubian range, Hungary: A synopsis. Int. Earth Sci. 106 (3), 991–1021. doi:10.1007/s00531-016-1347-7
Hongliu, Z., Wenzhi, Z., Zhaohui, X., Qilong, F., Suyun, H., Zecheng, W., et al. (2018). Carbonate seimic sedimentology: Acase study of cambrian Longwangmiao Formation gaoshiti-moxi area, Sichuan Basin, China. Petroleum Explor. Dev. 45 (05), 775–784. doi:10.11698/PED.2018.05.03
Jiang, W., Zhang, P., Li, D., Li, Z., Wang, J., Duan, Y., et al. (2022). Reservoir characteristics and gas production potential of deep coalbed methane: Insights from the no. 15 coal seam in shouyang block, Qinshui Basin, China. Unconv. Resour. 2, 12–20. doi:10.1016/j.uncres.2022.06.001
Jingjie, Y., Jinmin, S., and Shugen, L. (2020). etcGenetic analysis of dolomite in the lower cambrian Longwangmiao Formation in northeastern sichuan. Acta Sedimentol. Sin. 38 (06), 1284–1295. doi:10.14027/j.issn.1000-0550.2019.108
Jinhu, D., Cai, Z., Chunchun, X., Haiqing, H., Ping, S., Yueming, Y., et al. (2014). Theoretical and technical innovations in strategic discovery of a giant gas field in Cambrian Longwangmiao Formation of central Sichuan paleo-uplift, Sichuan Basin. Petroleum Explor. Dev. 41 (03), 294–305. doi:10.1016/S1876-3804(14)60035-5
Katz, B., Gao, L., Little, J., and Zhao, Y. R. (2021). Geology still matters – unconventional petroleum system disappointments and failures. Unconv. Resour. 1, 18–38. doi:10.1016/j.uncres.2021.12.001
Ke, Z., Feng, B., Liu, Y., Cui, Z., and Liu, X. (2022). Dissolution and sedimentation patterns of typical minerals in artificial reservoirs under different environments. Unconv. Resour. 2, 60–71. doi:10.1016/j.uncres.2022.08.004
Kun, J., Suping, Y., Hao, W., Miaochun, L., and Zhongyi, T. (2014). Advances in characterization of pore system of gas shales. J. Geol. China Univ. 20 (01), 151–161. doi:10.16108/j.issn1006-7493.2014.01.009
Lianqiang, Z., Haifeng, Y., and Xuemei, L. (2019). Mineral filling period and hydrocarbon accumulation of cambrian Longwangmiao Formation in Anyue structure, Sichuan Basin. Petroleum Geol. Experimen 41 (06), 812–820.
Lingfang, Z., Yixiong, Q., Xiaobo, S., Bo, C., Donghua, Y., and Yong, L. (2020). Characterization, distribution and origin of dolomite reservior in the upper Lei 4 member of the Middle Triassic, Pengzhou gas field, Western Sichuan Basin. Oil Gas Geol. 41 (01), 177–188. doi:10.11743/ogg20200116
Lucia, J. F. (1995). Rock-fabric/petrophysical classification of carbonate pore space for reservoir characterization. AAPG Bull. 79, 1275–1300.
Mengyan, X., Mingyi, H., Da, G., Di, W., Jingyi, W., and Lingshan, L. (2017). Characteristivs and main controlling factors of lower cambrian Longwangmiao Formation beach-facies reservoirs in gaoshiti-moxi area, central Sichuan Basin. J. Guilin Univ. Technol. 37 (01), 37–46.
Mindong, J., Wei, Z., Xiucheng, T., Ling, L., Zongyin, L., Bing, L., et al. (2014). Characteristics and controlling factors of beach-controlled karst reservoirs in cambrian Longwangmiao Formation, moxi-gaoshiti area, Sichuan Basin, NW China. Petroleum Explor. Dev. 41 (06), 712–723. doi:10.1016/S1876-3804(14)60085-9
Mindong, J., Xiucheng, T., Wei, Z., Ling, L., and Zongyin, L. (2014). Beach-controlled karst reservoir characteristics and controlling factors of Longwangmiao Formation in Moxi-Gaoshiti area, Sichuan Basin, China. Petroleum Explor. Dev. 41 (6), 650–660.
Nan, Q. (2020). Research progress of microscopic pore structure characterization of reservoir. Petrochem. Ind. Appl. 39 (11), 17–22. doi:10.3969/j.issn.1673-5285.2020.11.004
Niu, X., Feng, S., You, Y., Xin, H., Liang, X., Hao, B., et al. (2022). Analyzing major controlling factors of shale oil 'sweet spots' in the Chang-7 member of the Triassic Yanchang Formation, Ordos Basin. Unconv. Resour. 2, 51–59. doi:10.1016/j.uncres.2022.07.001
Pajdak, A., Godyn, K., Kudasik, M., and Murzyn, T. (2017). The use of selected research methods to describe the pore space of dolomite from copper ore mine, Poland. Environ. Earth Sci. 76 (11), 1–16. doi:10.1007/s12665-017-6724-4
Qiang, D., Jian, C., Bo, Y., Zixin, J., Fei, L., Ziwen, Z., et al. (2021). Quantitative characterization and classification of pore structure in chang 4+5 member in block hu-154, ordos basin. Xinjiang Pet. Geol. 42 (04), 410–417. doi:10.7657/XJPG20210403
Qilong, F., Suyun, H., Zhaohui, X., Wenzhi, Z., Shuyuan, S., and Hongliu, Z. (2020). Depositional and diagenetic controls on deeply buried Cambrian carbonate reservoirs: Longwangmiao Formation in the Moxi–Gaoshiti area, Sichuan Basin, southwestern China. Mar. Petroleum Geol. 117, 104318. doi:10.1016/j.marpetgeo.2020.104318
Roberts, J. A. (2018). Dolomite and dolomitization,” in M. Encyclopedia of geochemistry (Berlin: Springer-Verlag).
Vafaie, A., Kivi, I. R., Moallemi, S. A., and Habibnia, B. (2021). Permeability prediction in tight gas reservoirs based on pore structure characteristics: A case study From south western Iran. Unconv. Resour. 1, 1–8. doi:10.1016/j.uncres.2021.04.001
Weiqiang, Y., Pingping, L., Haoru, C., Zheng, L., Caijun, L., Zhehang, X., et al. (2022). Origin and significance of carbonate shoal depositional cycles: A case study of the cambrian Longwangmiao Formation, Sichuan Basin, SW China. Asian Earth Sci. 226, 105083. doi:10.1016/J.JSEAES.2022.105083
Xi, L., Zhenhua, G., Yujin, W., Xiaohua, L., Manlang, Z., Wuren, X., et al. (2017). Geological characteristics and development strategies for Cambrian Longwangmiao Formation gas reservoir in Anyue gas field, Sichuan Basin, SW China. Petroleum Explor. Dev. 44 (3), 428–436. doi:10.1016/S1876-3804(17)30049-6
Xiangchun, L., Jiaxing, G., Shuang, Z., Yi, L., Mengya, W., and Weidong, L. (2021). Combined characterization of scanning electron microscopy, pore and crack analysis system, and gas adsorption on pore structure of coal with different volatilization. Earth Sci. 47 (5), 1876–1889. doi:10.3799/dqkx.2021.195
Xing, T., Dailin, Y., Jiayi, Z., Yiting, S., Yunlong, W., Yu, Y., et al. (2021). Microscopic characterization of dolomite reservoir based on CT imaging technique-a case study of the fourth member of Sinian Dengying Formation in Moxi-Longnvsitai area, central Sichuan. Acta Sedimentol. Sin. 39 (05), 1264–1274. doi:10.14027/j.issn.1000⁃0550.2020.055
Xuefei, Y., Xingzhi, W., and Hao, T. (2015). Study on sedimentary microfacies of Longwangmiao Formation in moxi area, central Sichuan Basin, China. Acta Sedimentol. Sin. 33 (05), 972–982. doi:10.14027/j.cnki.cjxb.2015.05.013
Yang, X., Fan, J., Zhang, Y., Li, W., Du, Y., and Yang, R. (2022). Microscopic pore structure characteristics of tight limestone reservoirs: New insights from section 1 of the permian maokou formation, southeastern Sichuan Basin, China. Unconv. Resour. 2, 31–40. doi:10.1016/j.uncres.2022.08.001
Ying, R., Dakang, Z., honglong, G., Haitao, S., Hao, P., Xiaowei, Z., et al. (2019). Origin of dolomite of the lower cambrian Longwangmiao Formation, eastern Sichuan Basin, China. Carbonates Evaporites 34 (3), 471–490. doi:10.1007/s13146-017-0409-7
Keywords: gaoshiti area, Longwangmiao formation, quantitative characterization of pore parameters, pore distribution, diagenesis, pore evolution model
Citation: Qu H, Yan Z, Zheng C, Xu W, He F, Sun Q, Zhang X and Li M (2023) Quantitative characterization and origin of differences in pore parameter distribution: A case study of the lower cambrian longwangmiao formation in the gaoshiti area of central Sichuan Basin. Front. Earth Sci. 10:1043148. doi: 10.3389/feart.2022.1043148
Received: 13 September 2022; Accepted: 30 September 2022;
Published: 10 January 2023.
Edited by:
Shuai Yin, Xi’an Shiyou University, ChinaCopyright © 2023 Qu, Yan, Zheng, Xu, He, Sun, Zhang and Li. This is an open-access article distributed under the terms of the Creative Commons Attribution License (CC BY). The use, distribution or reproduction in other forums is permitted, provided the original author(s) and the copyright owner(s) are credited and that the original publication in this journal is cited, in accordance with accepted academic practice. No use, distribution or reproduction is permitted which does not comply with these terms.
*Correspondence: Haizhou Qu, cXVoYWl6aG91QHN3cHUuZWR1LmNu