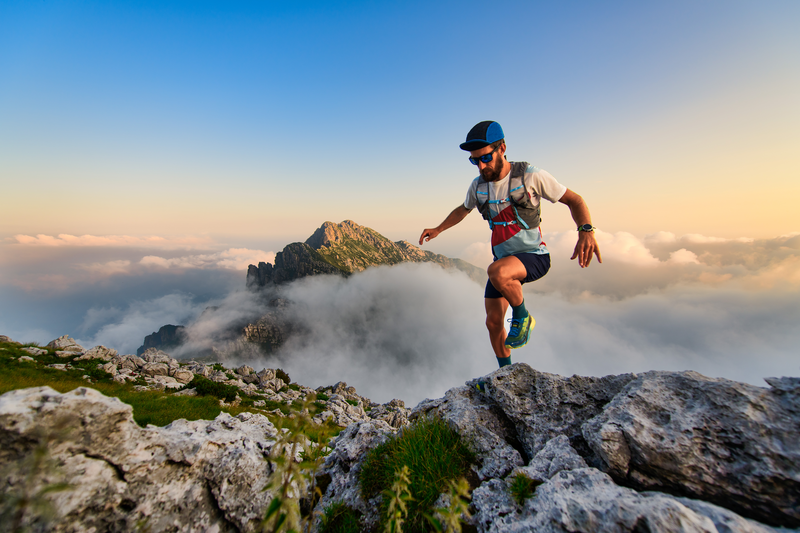
95% of researchers rate our articles as excellent or good
Learn more about the work of our research integrity team to safeguard the quality of each article we publish.
Find out more
ORIGINAL RESEARCH article
Front. Earth Sci. , 06 January 2023
Sec. Structural Geology and Tectonics
Volume 10 - 2022 | https://doi.org/10.3389/feart.2022.1042927
This article is part of the Research Topic Quantitative Characterization and Engineering Application of Pores and Fractures of Different Scales in Unconventional Reservoirs – Volume II View all 39 articles
The primary focus of oil and gas exploration for tight sandstone reservoirs is on a quantitative characterization of reservoir properties. This paper uses the tight sandstone reservoir developed in the Gaotaizi oil layer in the Qijia area of the Songliao Basin as an example. The petrology, physical properties, pore–throat characteristics, and the genesis of the densification of the oil-bearing sandstones are elucidated using casting thin-sections, scanning electron microscopy, 3D computerized tomography (CT), and petrophysical experimental techniques. The results show that the Gaotaizi oil layer is mainly composed of clastic rocks and contains small amounts of shell limestone. The clastic rocks are mainly lithic feldspar sandstone and feldspar lithic sandstone, while residual intergranular pores, intergranular dissolved pores, intragranular dissolved pores, intragranular pores and intercrystalline pores constitute the different pore types. Mercury intrusion and 3D computerized tomography analysis showed that micro-nano pores account for 53% of the total pores present. The pore–throat coordination number is distributed between 1 and 4, with an average of 1.8. The pores and throats in the Gaotaizi reservoir have poor connectivity. The porosity distribution of the Gaotaizi oil layer is 1.4%–22.5%, with an average of 9.5%, while the permeability distribution ranges from 0.01 to 27.10 mD, with an average of 0.41 mD. It is an ultra-low porosity and ultra-low permeability tight reservoir. The Gaotaizi oil layer is divided into three types of reservoirs through a systematic study of its pore developmental characteristics. Diagenetic processes like compaction and cementation result in a reduction in porosity and permeability. Compaction, calcite and siliceous cementation, and illite packing are primarily responsible for reservoir densification.
The increasing proportion of unconventional oil and gas resources in the global total makes the study of tight oil and gas reservoirs more pertinent (Fu, 2014; Shi et al., 2019; Zhang, 2016; Katz et al., 2021; Wang et al., 2022). The key to these reservoirs lies in the fine characterization of their properties. Tight reservoirs have a complex pore network space composed of pores and pore throats (Feng et al., 2019; Meng et al., 2020; Wu et al., 2021; Yang et al., 2022a). Pores, in a broad sense, are generally defined as free spaces between multiple framework particles, in which various fluids exist. Pore throats are essentially the narrow, elongated paths between rock grains that facilitate communication between and play a decisive role in the flow of fluids between pores (Shi et al., 2014; 2015; Li et al., 2016; Jiang et al., 2022). For the evaluation of conventional reservoirs, porosity and permeability are key parameters that reflect the basic physical properties of the reservoir. However, in the evaluation of unconventional oil and gas reservoirs, factors such as reservoir sedimentary cycle, structure and reservoir heterogeneity are considered, in addition to porosity and permeability (Wang et al., 1994; Asante-Okyere et al., 2021; Yang et al., 2022b; Niu et al., 2022; Owusu et al., 2022). Developing a combination of various experimental and simulation techniques that quantitatively characterize the internal pore structures of tight reservoirs is the future of reservoir geology.
The Gaotaizi oil layer occurs within Cretaceous strata in the Qijia area of the Songliao Basin, China. It is a typical tight sandstone reservoir that has, in the past, been studied to assess its depositional environment, hydrocarbon accumulation conditions and diagenesis. The sand bodies developed in Gaotaizi oil layer in the Qijia area are sheet sand microfacies deposits from an underwater distributary channel. These large-area sand bodies create good reservoir conditions for the formation of fault–lithologic reservoirs such as sandstone lens bodies, sand body pinch-outs, and fault blocking. Diagenesis obviously controls the formation and distribution of oil and gas in the Gaotaizi oil layer in the Qijia area, and industrial oil and gas layers are usually formed in the middle diagenetic stage. From the edge to the center of the Qijia area, the diagenetic intensity gradually increases. The Gaotaizi oil layer in the Qijia area of the Songliao Basin has good source, reservoir and hydrocarbon accumulation conditions. The systematic study of the genesis of reservoir densification is crucial for the evolution of reservoir pore structures and the prediction of sweet spots. In this paper, the tight sandstone developed in the Gaotaizi oil layer in the Qijia area of the Songliao Basin is assessed; its petrology, physical properties, pore–throat characteristics, and the genesis of the densification of the oil-bearing sandstones are elucidated using casting thin-sections, scanning electron microscopy, 3D computerized tomography (CT), and petrophysical experimental techniques. The results of this study could potentially improve our understanding of the origins of reservoir densification in the Gaotaizi oil layer.
The Songliao Basin is a large Mesozoic and Cenozoic sedimentary basin superimposed on the Paleozoic basement in eastern China. The central part of the Songliao Basin is called the Central Depression Area, and includes the Qijia area—which is where this study is based. The Central Depression Area is the primary structural unit of the basin, and it is a large negative structural unit that experienced predominantly (relative) subsidence during basin development. The Central Depression Area has been the subsidence and deposition center of the Songliao Basin for a long time, and its present structural form is that of a slightly undulating large duplex syncline. The Qijia area is adjacent to the Daqing Placanticline in the east, western slope in the west, and Gulong Sag in the south. The study area encompasses about 3000 km2 (Figure 1). The Mesozoic and Cenozoic strata in this area are composed of Cretaceous, Paleogene, Neogene, and Quaternary sediments from bottom to top. The strata are divided into five oil-bearing zones, namely the Saltu, Putaohua, Gaotaizi, Fuyu, and Yangdachengzi layers. The Qijia area has well-developed strata, and the total thickness of the Jurassic to Tertiary sedimentary rocks varies between 7 and 10 km. There are several sets of source-reservoir-caprock assemblages developed here, including the target layer of this study—the Cretaceous Gaotaizi oil layer.
Cast thin-sections, mercury intrusion, physical property testing, field emission scanning electron microscopy, and micro-nano CT high-resolution imaging techniques were used to study the intrinsic properties of the Gaotaizi tight sandstone reservoir; a total of 100 samples and six wells were selected for this study (Figure 1). Cores from wells Jin 341, Jin 191, and Long 25 were chosen for the scanning electron microscope experiment. Cores of wells Qiping one and Long 25 were selected for the casting thin-section observation experiment. Cores of wells Jin 341, Jin 191, and Jin 45 were selected for the micro nano CT experiment.
Scanning electron microscopy is used for high-resolution topography observation of conductive and non-conductive samples. A field emission scanning electron microscope was used to study the internal structures of the samples. During sample preparation, samples were taken from fresh sections of bulk samples. A JCF-1600 ion sputterer was used to spray the conductive layer directly on the samples, and a JSM-7500F field emission scanning electron microscope was used to acquire secondary electron images. The resolution of the secondary electron images is 1 nm, and its magnification is 25–300,000 times.
The basic principle of high-pressure mercury intrusion is the process whereby the non-wetting phase of mercury overcomes capillary resistance and enters pores. At this time, the injection pressure is the capillary resistance under the corresponding pore throat size. The volume of mercury entering the pores represents the size of the connected pore volume at this pore throat size. An AutoPore IV9520 automatic mercury porosimeter from Micromerics was used for this experiment, and a computer controls the process of mercury intrusion and extrusion. The pressure can reach 414 MPa, and the pore diameter ranges from 10–3–1000 μm. The sample volume used in the experiment was in the range of 9.85–14.08 cm3.
In 3D CT, X-rays penetrate an object and ray intensity is attenuated due to density differences in the sample; the degree of attenuation follows the Beer-Lambert law. When X-rays penetrate the core, the density differences inside the sample result in different degrees of attenuation, forming a series of gray value images on the detector probe. In this study, a MicroX-CT400 CT machine was used for the 3D CT scanning experiments. The X-ray light source voltage of the CT machine is 60–140 KV, the exposure time is 30s, and the spatial resolution is 0.8 μm.
The Cretaceous Gaotaizi oil layer in the Qijia area is mainly composed of clastic rocks that contain a small amount of shell limestone. The clastic rocks are predominantly lithic feldspar sandstone, followed by feldspar lithic sandstone (Figure 2). The content of detrital components is 51%–97%, with an average value of 84.1%. Among detrital components, the content of quartz is 15%–37%, with an average of 28.6% and the content of feldspar is 19%–45%, with an average of 32.8%. The debris content is 14%–37%, with an average of 18.6%, of which igneous rock debris is the main component. Mesoclastic components range from 0% to 29%, with an average value of 3.8%. The average content of interstitials was 15.9%. Among the interstitials, the content of calcite is 0%–29%, with an average of 8.9%; the content of illite is 0%–11%, with an average of 3.5%; and the total amount of authigenic quartz and feldspar is about 3%.
The physical property test results of 2854 samples from 70 wells in the Gaotaizi reservoir in the Qijia area were used as basic data for physical property analysis. The porosity has a normal distribution; the distribution interval is 1.4%–22.5%, the main frequency is 2%–16%, and the average value is 9.5%. The permeability of the Gaotaizi reservoir has a wide distribution range; statistical results show that the distribution interval is 0.01–27.1 mD, the main frequency is 0.01–3 mD, the average value is 0.41 mD, and the median value is 0.09 mD. The samples with permeability less than 1 mD accounted for 91.6% of the total samples, and the samples with permeability less than 2 mD accounted for 96.0% of the total samples (Figure 3). Following the physical property classification standard of clastic rock reservoirs issued by the National Reserves Commission (Table 1), the Gaotaizi oil layer in the Qijia area is characterized as an ultra-low porosity and ultra-low permeability reservoir.
FIGURE 3. Frequency distribution characteristics of the (A) porosity and (B) permeability of the Gaotaizi reservoir in the Qijia area.
The porosity and permeability of the Gaotaizi oil layer in the Qijia area have an obvious positive correlation. As the porosity increases, the permeability of the rock samples increases exponentially, but the data points are discretely distributed. Samples with the same porosity can differ by a factor of up to 1000 in the maximum permeability; however, samples with the same permeability can differ in porosity by a factor of up to 10 (Figure 4).
FIGURE 4. Relationship between the porosity and permeability of the Gaotaizi oil layer in the Qijia area.
Quantitative analysis of cast thin-sections, ordinary scanning electron microscopy and field emission scanning electron microscopy suggest that multiple pore types are developed in the Gaotaizi oil layer in the Qijia area. These include residual intergranular, intergranular dissolved, intragranular dissolved, intragranular and intercrystalline pore types; a significant number are of the residual intergranular and dissolution pore types (Figure 5; Figure 6).
FIGURE 5. Bar chart of the pore types developed in the sandstones of the Gaotaizi oil layer in the Qijia area.
FIGURE 6. Photomicrographs of the main pore types developed in the Gaotaizi oil layer in the Qijia area; (A) Well Jin 341, 2076.49m, residual intergranular pores; (B) Well Jin 191, 1863.8m, intergranular and intergranular dissolved pores; (C) Well Qiping 1, 1956.12m, intercrystalline pores and quartz intragranular pores; (D) Well Long 25, 2043.43m, intracrystalline pores.
A large number of fine pores are developed in the Gaotaizi oil layer in the Qijia area. In this study, field emission scanning electron microscopy and 3D CT high-resolution imaging technology, combined with conventional analysis and testing methods are used to compile a multi-scale, quantitative characterization of the pore characteristics of the oil layer. Pores with diameters greater than 1 μm are defined as micro-scale pores, pore diameters between 1000 and 500 nm are defined as sub-micron pores, and pores with diameters less than 500 nm are defined as nano-scale pores.
The identification of micron-scale pores was carried out using the traditional casting thin-section method. The reservoir space of the Gaotaizi oil layer is mainly composed of residual intergranular pores and dissolution pores. The pore radius in the oil layer is mainly between 10 and 20 μm, and the maximum value is as high as 50 μm. The average surface porosity and average porosity of the Gaotaizi reservoir in the Qijia area are 2.2% and 9.5%, respectively, and the micron-sized pores account for about 23% of the total porosity.
Precise quantitative characterization of micron-scale pores is achieved using CT. According to the CT analysis results of the six groups of samples, the average pore radius of the samples was 2 μm, the average throat radius was 0.58 μm, the average throat length was 9.66 μm, and the coordination number ranged from 2.5 to 22.0, with an average of 10.5. A high coordination number indicates good pore–throat connectivity. The CT analysis results showed that the porosity of the samples was 3.0%–8.1%, with an average value of 6.2%. This value is lower than the average porosity of 12% found in conventional physical property tests. Conventional physical property analysis measures the sum of pore volumes at different scales. The comparison between CT porosity and conventional porosity shows that micro-nano pores account for 53% of reservoir pores.
Sub-micron pores can be qualitatively identified by ordinary scanning electron microscopy and field emission scanning electron microscopy (Figures 6C,D). Observational data suggests that the sub-micron pores are mainly composed of clay mineral intracrystalline pores, quartz intragranular pores, and intragranular dissolved pores; their content accounts for about 10% of the total number of pores.
According to the mercury intrusion data of 94 samples from ten wells, the Gaotaizi oil layer in the Qijia area is dominated by nano-scale pore throats. The space with pore throat radius of less than 1 μm accounts for 97%, and the space with pore throat diameter of less than 1 μm accounts for 90% (Figure 7). According to the relationship between the pore throat radius distribution and the cumulative mercury injection percentage (Figure 8), reservoirs are divided into three types. The pore throat radius of Type I reservoirs is 0.05–2 μm, the average porosity is 10.7%, and the average permeability is 0.62 mD. The pore throat radius of Type II reservoirs is 0.005–0.1 μm, the average porosity is 7.7%, and the average permeability is 0.1 mD. The pore throat radius of Type III reservoirs is less than 0.005 μm, the average porosity is 4.9%, and the average permeability is 0.1 mD.
The Gaotaizi oil layer in the Qijia area has poor pore structures. The pore–throat coordination number is mainly distributed between 1 and 4, with an average of 1.8. It can go as high as 13.4 for some pore–throat coordination number. The pores and pore throats are poorly connected (Figure 9). There is strong heterogeneity in the distribution of pore throats in the Gaotaizi oil layer; the distribution of pores and pore throats as reflected in a sample with a diameter of 2 mm is extremely uneven. This suggests that the sandstone was strongly affected by sedimentation and diagenesis, which enhances the complexity of fluid seepage.
FIGURE 9. Three-dimensional pore–throat structures of the Gaotaizi oil layer based on three-dimensional CT scanning; (A) Jin 341 well, dry layer, carbonate cementation, porosity of 2.6%; (B) Jin 341 well, oil immersion, porosity of 5.8%, good pore connectivity; (C) Jin 191 well, oil immersion, porosity of 7.9%, well developed pores and good connectivity; (D) Jin 45 well, dry layer, porosity of 8.1%, extremely well-developed pores and good connectivity, coordination number of 13.4.
A delta front facies depositional environment characterizes the Gaotaizi oil layer in the Qijia area. Large amounts of siltstone formed in this environment. After the sediments passed the syngeneic stage and entered the burial diagenetic stage, the effects of compaction that pervaded the burial had great influence on the physical properties of the reservoir (Liu et al., 2019; Vafaie et al., 2021). The Gaotaizi oil layer suffered intense compaction as a consequence of fine grain size and high shale content, resulting in low reservoir porosity and permeability (Figure 10). Calculations suggest that the original porosity of the reservoir reduced by 60% due to compaction. Compaction is an important mechanism that results in porosity reduction. Increasing overlying stress ensures that the fluids in the rock pores are continuously discharged, changing the physical properties of the reservoir drastically (Tian et al., 2019; Jiang et al., 2020). The magnitude of the overburden stress is mainly related to the burial depth of the strata. Therefore, depth is an important parameter reflecting the strength of compaction.
FIGURE 10. Photomicrographs of the compaction and cementation in the Gaotaizi oil layer in the Qijia area; (A) Well Jin 341, 2076.49 m, compaction; (B) Well Qiping 1, 1956.12 m, cementation.
The formation and evolution of strata in the Qijia area show are linked to the changing reservoir properties. From the younger Nenjiang Formation to the older Quantou Formation, reservoir porosity and permeability generally decrease linearly with increasing burial depth. The decreasing trend of porosity is the most obvious. From 1 to 2 km, the porosity decreases by about 10%. Increasing the depth of burial increases the vertical ground stresses. Under normal compaction conditions, rock porosity should gradually decrease; however, a sharp decrease in porosity in the initial stage is followed by a more gradual decrease with increasing burial depth. Therefore, vertical ground stresses are usually most sensitive to the porosity of tight reservoirs, while the sensitivity to permeability is relatively low (Figures 11A,B).
FIGURE 11. Variation of (A) porosity, (B) permeability, and (C) rock density of tight sandstone reservoirs with depth in the Qijia area.
The density of the rock increases linearly with increasing burial depth, reflecting the tighter arrangement of the grains (Figure 11C). Some zones that develop have abnormal porosity and permeability, but normal rock density. These abnormal physical properties are mainly dissolved pore development belts. In the Qijia area, the abnormal physical properties are mainly developed in the Qingshankou and Quentou formations.
Many kinds of cements are produced during the diagenesis of tight sandstones (Nabawy et al., 2009; Zhang et al., 2009; Jiang et al., 2020; Liu et al., 2023). The precipitation of cements further aggravates the process of densification of the reservoir. It is difficult for a single diagenetic mechanism or diagenetic product to make reservoirs tight; current tight reservoirs are usually the result of the coupling of various diagenetic changes.
There is an obvious negative correlation between the physical properties and the carbonate content of the Gaotaizi oil layer in the Qijia area (Figure 12). When the carbonate content is less than 4%, the distribution interval of porosity and permeability is large; they have no clear relationship with carbonate content. When the carbonate content is greater than 4%, the porosity and permeability decrease rapidly with increasing carbonate content. Here, the relationship between porosity and carbonate rock content is more obvious; thus, carbonate rock content has a greater impact on porosity, than on permeability.
FIGURE 12. Relationship of (A) porosity and (B) permeability with carbonate content of the Gaotaizi oil layer.
There are two stages of carbonate cementation in the Gaotaizi oil layer in the Qijia area. Generally, early calcite cementation in the cores aids the development of dry layers. The boundary between the lower oil layer and the dry layer is clear, reflecting the early formation of this type of calcite. Late calcite cementation occurs mainly in the form of plaques that persist primarily in residual intergranular pores or metasomatic feldspar dissolution pores after compaction (Figures 5C,D). In addition to carbonate cements, a small amount of authigenic quartz also developed in the Gaotaizi oil layer. Under the influence of strong cementation and compaction, the Gaotaizi oil layer was transformed into a tight reservoir.
Illite clay minerals are particularly developed in the Gaotaizi oil layer in the Qijia area (Figure 13). Under the microscope, these minerals are mostly infected by crude oil, and appear black or brown. Illite typically occurs in three forms; as flakes, needles, and bridges. The coniferous and bridging illites of secondary origin are dominant, while the flaky illites were likely formed during original deposition. Illite clay minerals are packed into various pore spaces. Their existence drastically reduces the porosity of the reservoir, while simultaneously increasing the difficulty of fluid percolation through the pores. Therefore, their presence severely affects the physical properties of the reservoir. A large number of intercrystalline pores are developed in illite clay minerals, although they are not effective spaces for the storage of oil.
FIGURE 13. Developmental characteristics of the illite clay minerals in the Gaotaizi reservoir under field emission scanning electron microscopy.
The Gaotaizi oil layer in the Qijia area has undergone a complex process of diagenesis (Figure 14). Compaction persisted throughout the entire diagenetic process, and was strongest in the early stage of diagenesis. At this stage, a small amount of kaolinite formed on the surface of detrital particles such as quartz and feldspar. Feldspars experienced hydration in the shallow burial stage, and provided a material source for the early secondary increase of quartz. In the middle diagenetic stage, as the degree of the thermal evolution of organic matter increased, organic matter entered a low maturity stage. A large number of organic acids were generated, soluble minerals such as feldspar were dissolved, and secondary pores were formed. It provided space and a material source for late calcite cementation. When the organic matter entered the oil generation threshold, a large volume of hydrocarbons were expelled from the source layer. These hydrocarbons entered the pores of the reservoir, changed the original fluid environment, and inhibited cementation. In areas where hydrocarbon charging was not affected, the process of cementation continued. Thus, compaction, calcite cementation, siliceous cementation, and illite filling are the main reasons for reservoir densification. Analyzing the diagenetic evolution of the Gaotaizi reservoir in the Qijia area suggests that the reservoir has entered the middle diagenetic stage, which is conducive to the formation of tight reservoirs.
1) The petrology, physical properties, pore–throat characteristics, and the genesis of the densification of the oil-bearing tight sandstones in the Gaotaizi oil layer in the Qijia area of the Songliao Basin are explained using casting thin-sections, scanning electron microscopy, 3D CT, and petrophysical experimental techniques.
2) The Gaotaizi oil layer is mainly composed of clastic rocks and contains a small amount of shell limestone. The clastic rock types are mainly lithic feldspar sandstone, followed by feldspar lithic sandstone. The pores are primarily of the residual intergranular, intergranular dissolved, intragranular dissolved, intragranular and intercrystalline pore types.
3) Mercury intrusion and 3D CT analysis show that micro-nano pores account for 53% of the total pores present. The pore–throat coordination number is distributed between 1 and 4, with an average of 1.8. The pores and pore throats in the Gaotaizi reservoir have good connectivity. The porosity distribution of the Gaotaizi oil layer ranges from 1.4% to 22.5%, with an average of 9.5%. The permeability distribution ranges from 0.01 to 27.10 mD, with an average of 0.41 mD. Thus, the Gaotaizi oil layer is an ultra-low porosity and ultra-low permeability tight reservoir.
4) The systematic study of the developmental characteristics of pores allows the Gaotaizi oil layer to be divided into three types of reservoirs. Diagenetic processes including compaction and cementation result in a reduction in porosity and permeability. Thus, compaction, calcite cementation, siliceous cementation, and illite packing are the primary reasons for the densification of the Gaotaizi reservoir.
The original contributions presented in the study are included in the article/supplementary material, further inquiries can be directed to the corresponding author.
SS developed the idea for this paper and wrote the manuscript; YZ, CE, YB, WW, and JH were involved in data interpretation.
This paper is financially supported by the Science and Technology Department of Shaanxi Province project “Multi-periods mixed source shale oil accumulation contribution and its controlling factors of Qingshankou Formation in Gulong Sag, Songliao Basin”, Grant No.:2023-YBGY-077. The authors wish to acknowledge to the project.
Author YZ was employed by Exploration and Development Research Institute of Jilin Oilfield Company Ltd.
The remaining authors declare that the research was conducted in the absence of any commercial or financial relationships that could be construed as a potential conflict of interest.
All claims expressed in this article are solely those of the authors and do not necessarily represent those of their affiliated organizations, or those of the publisher, the editors and the reviewers. Any product that may be evaluated in this article, or claim that may be made by its manufacturer, is not guaranteed or endorsed by the publisher.
Asante-Okyere, S., Ziggah, Y. Y., and Marfo, S. A. (2021). Improved total organic carbon convolutional neural network model based on mineralogy and geophysical well log data. Unconv. Resour 1, 1–8. doi:10.1016/j.uncres.2021.04.001
Feng, J., Zhang, B. W., Feng, Z. H., Wang, Y. C., Zhang, J. H., and Fu, X. F., (2019). Crude oil movement in tight sandstone reservoirs in the northern Songliao Basin Sexual Influencing Factors. Petroleum Explor. Dev 46 (2), 312–321. doi:10.11698/PED.2019.02.11
Fu, X. L. (2014). Distribution of Gaotaizi oil layers and accumulation analysis in Qijia area of northern Songliao Basin, China. J. Chengdu Univ. Technol. Nat. Sci. Ed 41 (4), 422–427. doi:10.3969/j.issn.1671-9727.2014.04.03
Jiang, W., Zhang, P., Li, D., Li, Z., Wang, J., and Duan, Y., (2022). Reservoir characteristics and gas production potential of deep coalbed methane: Insights from the no. 15 coal seam in shouyang block, Qinshui Basin, China. Unconv. Resour 2, 12–20. doi:10.1016/j.uncres.2022.06.001
Jiang, Y. L., Liu, X. J., Zhao, X. Z., Jin, F. M., Liu, J. D., and Lv, X. Y. (2020). Comprehensive identification of oil and gas accumulation period by fluid inclusion technique and reservoir bitumen characteristics: A case study of the paleozoic buried hill in beidagang, huanghua depression. Earth Sci 45 (3), 980–988. doi:10.3799/dqkx.2019.016
Katz, B., Gao, L., Little, J., and Zhao, Y. R. (2021). Geology still matters – unconventional petroleum system disappointments and failures. Unconv. Resour 1, 18–38. doi:10.1016/j.uncres.2021.12.001
Li, Y. L., Zhang, Y. F., Yin, S. L., Cong, L., Yan, M., and Tian, X. X. (2016). Multi-scale characterization of tight sandstone reservoir space: A case study of Gaotaizi oil layer in Qijia area, Songliao Basin. Petroleum Nat. Gas Geol 37 (6), 915–922. doi:10.11743/ogg20160613
Liu, C., Jiang, T., Yang, Y., and Ma, J. (2023). Temporal and spatial variations of high-resolution strontium, carbon, and oxygen isotopic chemostratigraphy at the end-Permian crisis boundary in South China. Gondwana Res 113, 89–101. doi:10.1016/j.gr.2022.10.015
Liu, R. C., Ren, Z. L., Ma, K., Zhang, Y. Y., Qi, K., and Yu, C. Y., (2019). Classification of hydrocarbon accumulation phases of yanchang formation in southern ordos basin. Geoscience 33 (6), 1263–1274. doi:10.19657/j.geoscience.1000-8527.2019.06.12
Meng, Y. L., Zhang, L., Tao, S. Z., Li, G. H., Li, X. N., and Dai, T. J., (2020). Subdivision and application of diagenetic stages of clastic rocks: Taking Qijia-Gulong area in Songliao Basin as an example. China Univ. Min. Technol. Chin. J 49 (5), 920–930. doi:10.13247/j.cnki.jcumt.001158
Nabawy, B. S., Géraud, Y., Rochette, P., and Bur, N. (2009). Pore-throat characterization in highly porous and permeable sandstones. AAPG Bull 93 (6), 719–739. doi:10.1306/03160908131
Niu, X., Feng, S., You, Y., Xin, H., Liang, X., and Hao, B., (2022). Analyzing major controlling factors of shale oil 'sweet spots' in the Chang-7 member of the Triassic Yanchang Formation, Ordos Basin. Unconv. Resour 2, 51–59. doi:10.1016/j.uncres.2022.07.001
Owusu, E. B., Tetteh, G. M., Asante-Okyere, S., and Tsegab, H. (2022). Error correction of vitrinite reflectance in matured black shales: A machine learning approach. Unconv. Resour 2, 41–50. doi:10.1016/j.uncres.2022.07.002
Shi, L. Z., Qi, Y., Zhang, Y. S., Wang, Z. Z., and Wang, B. (2019). Simulation of "four history" and its geological significance in Qijia area, Songliao Basin. Geol. Explor 55 (2), 661–672. doi:10.12134/j.dzykt.2019.02.021
Shi, L. Z., Wang, Z. Z., Zhang, G., Zhang, Y. S., and Xing, H. Y. (2015). Formation conditions and distribution law of tight oil in Qijia area, Songliao Basin. Petroleum Explor. Dev 42 (1), 44–50. doi:10.11698/PED.2015.01.05
Shi, L. Z., Wang, Z. Z., and Zhang, Y. S. (2014). Distribution and geological characteristics of tight oil in Gaotaizi oil layer in Qijia area, Songliao Basin. Nat. Gas. Geosci 25 (12), 1943–1950. doi:10.11764/j.issn.1672-1926.2014.12.1943
Tian, X. R., Zhang, Y. Y., Zhou, Q. G., Yu, Z. C., and Guo, Z. J. (2019). Tight oil charging characteristics of the Lower Permian Fengcheng formation in Mahu sag, Junggar Basin: Evidence from fluid inclusions in alkaline minerals. Aata Petrloei Sin 40 (6), 646–659. doi:10.7623/syxb201906002
Vafaie, A., Kivi, I. R., Moallemi, S. A., and Habibnia, B. (2021). Permeability prediction in tight gas reservoirs based on pore structure characteristics: A case study from south western Iran. Unconv. Resour 1, 9–17. doi:10.1016/j.uncres.2021.08.001
Wang, C., Song, G. X., and Liu, Z. W. (1994). Enclosure of authigenic mineral from northern part of songliao basin and its application. Petroleum Geol. Oilfield Dev. Daqing 13 (4), 19–22. doi:10.19597/j.issn.1000-3754.1994.04.006
Wang, H., Zhou, S., Li, S., Zhao, M., and Zhu, T. (2022). Comprehensive characterization and evaluation of deep shales from Wufeng-Longmaxi Formation by LF-NMR technology. Unconv. Resour 2, 1–11. doi:10.1016/j.uncres.2022.05.001
Wu, W. T., Zhao, J. Z., Meng, Q. A., Lin, T. F., Zhang, G., and Zhang, J. Y., (2021). Accumulation mechanism of tight sandstone oil in Gaotaizi oil layer in Qijia area, Songliao Basin. Petroleum Nat. Gas Geol 42 (6), 1376–1388. doi:10.11743/ogg20210612
Yang, X., Fan, J., Zhang, Y., Li, W., Du, Y., and Yang, R. (2022). Microscopic pore structure characteristics of tight limestone reservoirs: New insights from section 1 of the permian maokou formation, southeastern sichuan basin, China. Unconv. Resour 2, 31–40. doi:10.1016/j.uncres.2022.08.001
Yang, Y., Chen, S., Dai, C., Wang, X., Li, X., and Zeng, Q., (2022). Prediction of glutenite reservoirs using an adaptive Bayesian seismic inversion in the slope zone of Mahu Depression in Junggar Basin, NW China. Unconv. Resour 2, 21–30. doi:10.1016/j.uncres.2022.06.002
Zhang, J. C., James, L., and William, S. (2009). Stress, porosity, and failure-dependent compressional and shear velocity ratio and its application to wellbore stability. J. Petroleum Sci. Eng 69, 193–202. doi:10.1016/j.petrol.2009.08.012
Zhang, J. Y. (2016). The main controlling factors for oil-bearing properties of tight sandstone reservoirs in source rocks in continental depression basins: A case study of Gaotaizi oil layer in Qijia sag, central depression, northern Songliao Basin. J. Sedimentology 34 (5), 991–1002. doi:10.14027/j.cnki.cjxb.2016.05.018
Keywords: tight reservoir, diagenesis, pore structure, Gaotaizi oil layer, Qijia area
Citation: Si S, Zhao Y, Er C, Bai Y, Wu W and He J (2023) Reservoir properties and genesis of tight sandstones—A case study from the Gaotaizi oil layer in the Qijia area, Songliao basin. Front. Earth Sci. 10:1042927. doi: 10.3389/feart.2022.1042927
Received: 13 September 2022; Accepted: 22 December 2022;
Published: 06 January 2023.
Edited by:
Wenlong Ding, China University of Geosciences, ChinaReviewed by:
Nan Wu, Yangtze University, ChinaCopyright © 2023 Si, Zhao, Er, Bai, Wu and He. This is an open-access article distributed under the terms of the Creative Commons Attribution License (CC BY). The use, distribution or reproduction in other forums is permitted, provided the original author(s) and the copyright owner(s) are credited and that the original publication in this journal is cited, in accordance with accepted academic practice. No use, distribution or reproduction is permitted which does not comply with these terms.
*Correspondence: Junhao He, OTEzODQ0MzIyQHFxLmNvbQ==
Disclaimer: All claims expressed in this article are solely those of the authors and do not necessarily represent those of their affiliated organizations, or those of the publisher, the editors and the reviewers. Any product that may be evaluated in this article or claim that may be made by its manufacturer is not guaranteed or endorsed by the publisher.
Research integrity at Frontiers
Learn more about the work of our research integrity team to safeguard the quality of each article we publish.