- 1Northeast Electric Power Design Institute Co., Ltd. of China Power Engineering Consulting Group, Changchun, China
- 2College of Civil Engineering and Architecture, Zhejiang University, Hangzhou, China
Model tests were performed with different temperatures and soil stabilizers to investigate the effect of horizontal frost heave force on open tunnels in seasonal frozen regions. Experimental results show that the dissipation of the frost heave force accelerated with the increase in temperature, and the data indicate that compared with soil without stabilizers, the horizontal frost heave force decreased by 10% and 15% in soil with lignin fiber and Huaxia No. 1 soil stabilizer. It can be concluded that the inhibitory effect of Huaxia No. 1 soil stabilizer on frost heave force was more significant than that of lignin fiber. According to the test results, the relation expression between horizontal frost heave force and frost heave amount was fitted.
Introduction
China consists of about 54% seasonal frozen surface (Lai et al., 2018). The construction of pipes and cable tunnels in cold regions is often followed by frost damage. Especially when subjected to horizontal constraints, frozen soil will generate horizontal frost heave force, leading to the deformation and destruction of structures.
From the micro perspective, many attempts have been made to investigate the mechanism of frost heave. Frost heave is essentially a thermo-hydro-mechanical coupled problem based on the theoretical frame of a multi-phase porous medium for saturated frozen soils (He et al., 2012). Liquid transfer under a thermal gradient is assumed to be the cause of frost heave (Harlan, 1973). Mathematical models for simulating heat and liquid flow in freezing soils have been proposed by Taylor and Luthin (1978) and Horiguchi K (1987). Notably, it is essential to consider the influence of the stress and deformation field of the soil body in cold region engineering (Wang et al., 2005). Chen et al. (2002) established a thermo-moisture-deformation coupled theoretical frame for the freezing soil with the consideration of the force interaction between the soil skeleton and ice particles and the energy jump behaviors during the phase-changing between ice and water. He et al. (2012), He et al. (2018) simplified the heat-moisture-deformation model through the introduction of the Clapeyron equation to describe the temperature gradient on the moisture migration in the freezing zone. Bai (2019) developed a thermodynamic constitutive model based on particle arrangement, in which the irreversible consolidation of the normally consolidated saturated soils was attributed to the irreversible rearrangement of solid particles, and the temperature-induced elastic deformation of solid particles was dominant in soil with higher OCR.
From the macro perspective, temperature plays a key role in the development of horizontal frost heave force. Great progress has been made in the study of the influence of temperature on horizontal frost heave force. Ding (1983) examined the relationship between horizontal frost heave force and soil temperature through the study on the horizontal frost heave force of retaining walls. Liu (2000) proposed that the continuous low temperature would prompt the migration of water from the unfrozen zone to the frozen zone, and when the temperature dropped below 0°C, the water froze and the soil swelled. Liu (2005) found that in the process of freezing, the unfrozen water content decreased with the decrease in temperature, objectively resulting in the intensification of frost heave and elevation of the horizontal frost heave force. Work by Tong (1985) testified that horizontal frost heave force increased with a decrease in soil temperature. The force was lifted drastically with the temperature dropping from 0°C to −5°C and reached the peak at −5°C.
Physical, chemical, and integrated improvements of soil are widely used in engineering to reduce the settlement by freeze–thaw cycles (Zhang et al., 2015). The physical improvement is to enhance the physical strength of the soil by modifying the particle gradation (OLGUN M., 2013). The chemical improvement is to add components such as cement, lime, or chemical slurry to the soil, producing new substances with high strength (ESKIŞAR et al., 2015; HOTINEANU et al., 2015; Li et al., 2015). The integrated improvement combines physical and chemical improvement (Yang et al., 2019).
However, there is little research on the physical mechanism of the inhibitory effect of soil improvement on horizontal frost heave force. In addition, before soil improvement can be applied to prevent frost damage in engineering, more research is required to comprehensively characterize their frost heave property with different temperatures.
The present study used an indoor model test to investigate the characteristics of frost heave with different freezing temperatures and different soil stabilizers and explain the mechanism of soil improvement in restraining frost heave. A relation expression between frost heave force and frost heave amount was also introduced for tunnel and pipe designs.
Materials and methods
Model tests were performed on soil without soil stabilizers, with Huaxia No. 1 soil stabilizer and with lignin fiber. Each test involved frost heave force and amount measurement. All the tests were conducted in a closed system. This section presents the properties of the test materials and test procedures.
Test materials
The adopted scale factor for the model and prototype is 1:5. The model tunnel is a cubic tunnel of length 1.8 m, width 0.6 m, and height 0.6 m, with a lining thickness of 0.1 m. The Young’s modulus of the tunnel is 6 GPa. Tunnel details are shown in Figure 1.
The physical properties of the soil are summarized in Table 1. The unit weight of the soil is adjusted to real conditions by adding some water with compaction by layers.
The soil stabilizers used for the tests are Huaxia No. 1 soil stabilizer and lignin fiber. The physical properties of lignin fiber and Huaxia No.1 are listed in Table 2 and Table 3. Huaxia No. 1 is a liquid soil stabilizer made in China and composed of environment-friendly inorganic materials and a small amount of activator. Huaxia No. 1 is representative of the chemical improvement. According to previous foundation treatment cases, its preferred dosage is 0.01% and is applied in the model tests. Lignin fiber, as a physical improvement material, is solid and has the advantages of environmental protection and low price. Referring to a previous study by Chen et al. (2020), the optimum addition ratio of lignin fiber was 1:100 because the best effect of soil improvement against the deterioration of the elastic modulus caused by freeze–thaw cycles is achieved under this addition ratio. The aforementioned ratios are the ratios of the mass of soil stabilizers to the mass of dry soil.
Equipment and test procedures
The model test system is self-designed for this specific experiment and consists of a model tank, strain sensors, earth pressure sensors, temperature sensors, two freezers, and a data acquisition system. The model box size is 2 m × 0.8 m × 1 m (length × width × height). The strain sensors are located inside the model tunnel to measure the deformation of the tunnel. The earth pressure sensors are attached outside the tunnel sidewalls. Data from the earth pressure sensors are the sum of the lateral earth pressure and the horizontal frost heave force. The sensors are distributed in three sections, as shown in Figure 2. Two 300 L freezers are used as the refrigeration system for the test, with a temperature-controlling range of −26°C–10°C and fluctuations of ± 0.5°C. A 5-mm-thick layer of thermal insulation foam is laid on the surface of the frozen soil.
The model tunnel was put into the box when the foundation was filled to 0.2 m high. To prevent freezing damage in actual engineering, the cable tunnels were not fully constructed in the frozen soil layer, with only the upper part covered by frozen soil. Therefore, the upper 20 cm of the model tunnel was located in frozen soil. After the filling process, all the sensors were installed, and a cover plate was placed on the top with pressure applied through the weights for pre-compaction. For better application of test results in engineering, the lowest temperature was set as 10°C, which was a representative temperature in the design of the cable tunnels in seasonal frozen areas. The transition from ice crystals to free water increases with the increase in temperature, resulting in the increase in the speed of variation of the frost heave force and amount. Therefore, more sampling points are set near 0°C. Readings of the sensors were recorded at −10°C, −5°C, −2.5°C, −1.5°C, −1.0°C, −0.5°C, and 0°C.
Results
The frost heave response to temperature
The horizontal frost heave force is equal to the reading of the lateral earth pressure sensor minus the static earth pressure. The frost heave amount reflects the deformation of the frozen soil. Given that the model box is rigid, the tunnel’s lateral deformation is the frost heave amount of the soil.
Group A investigated the effect of freezing temperature on the frost heave force and the frost heave force without soil stabilizers. The results of Figure 3 indicated that the frost heave force decreased with increasing temperature. The average horizontal frost heave force at −10°C, −5°C, and 0°C was 17.70 kPa, 14.64 kPa, and 8.93 kPa, respectively. From −10°C to −5°C, the frost heave force decreased by 3.06 kPa and 17.2% in percentage. From −5°C to −0°C, the frost heave force decreased by 5.71 kPa and 32.2% in percentage. The decrease of horizontal frost heave force from 10°C to −5°C was lower than that from −5°C to 0°C. It is noted that the higher the freezing temperature is, the faster the ice crystals melt and the faster the frost heave force dissipates.
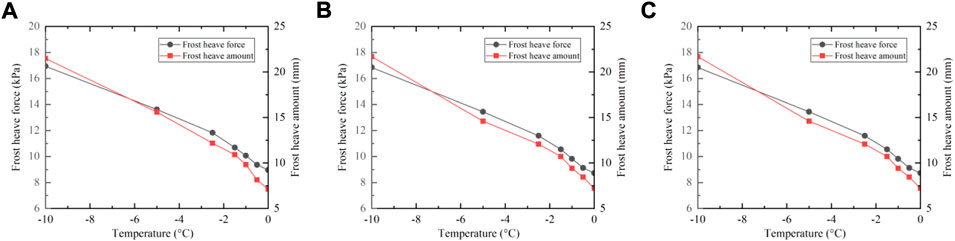
FIGURE 3. Change rules of frost heave force and amount in group A. (A) Section 1. (B) Section 2. (C) Section 3.
The variation of frost heave amount indicated that the frost heaving of the soil was reduced with temperature increment. The decrease of heating-up was accelerated by temperature increment. Similar to the behavior observed for frost heave force, it could be seen that the curve of frost heave amount also reached the maximum slope around −1.5°C, and the phenomenon was consistent with the accelerated melting rate of the ice crystals, as reflected by the horizontal frost heave force.
It was also observed that the frost heave amount–temperature curves of sections 1, 2, and 3 showed a similar variation trend, and their values were close, which indicated that the frost heave deformation of the soil along the sidewall was uniform. To eliminate the errors, the average of the frost heave amount of sections 1, 2, and 3 is taken as the frost heave amount of the model test. The frost heave amount at −10°C, −5°C, and 0°C was 22.19 mm, 15.60 mm, and 7.53 mm, respectively. The frost heave amount decreased by 6.59 mm and 29.7% in percentage from −10°C to −5°C and by 8.07 mm and 36.4% from −5°C to 0°C.
Group B investigated the effect of the frost heave of the soil with lignin fiber (1:100). The test results of group B in Figure 4 showed that the frost heave force was uniformly distributed along the tunnel when lignin fiber was added to the soil.
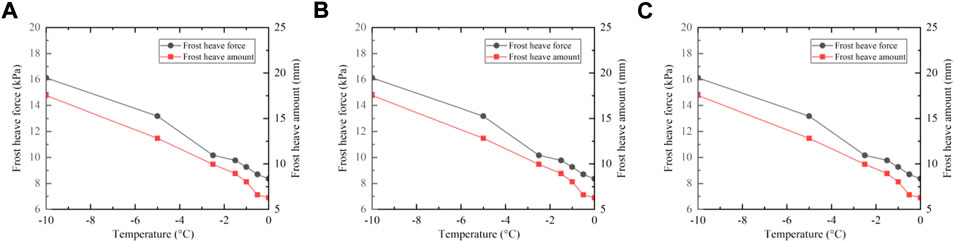
FIGURE 4. Change rules of frost heave force and amount in group B. (A) Section 1. (B) Section 2. (C) Section 3.
The average horizontal frost heave force at −0°C, −5°C, and 0°C was 16.19 kPa, 13.20 kPa, and 8.33 kPa, respectively. From −10°C to −5°C, the frost heave force decreased by 2.99 kPa (18.5%). From −5°C to −0°C, the frost heave force decreased by 4.87 kPa (30.1%). The frost heave amount at −10°C, −5°C, and 0°C was 18.23 mm, 12.82 mm, and 6.68 mm, respectively. The frost heave amount decreased by 5.41 mm (24.4%) from −10°C to −5°C and by 6.14 mm (27.7%) from −5°C to 0°C.
Compared with group A (no soil stabilizer added), the horizontal frost heave force was reduced by 5%–10%, and the frost heave amount was reduced by 15%–20% after adding lignin fiber.
Group C studied the effect of the frost heave of soil with Huaxia No. 1 soil stabilizer (1:10000). The test results are in Figure 5. The average horizontal frost heave force at −0°C, −5°C, and 0°C was 15.44 kPa, 12.00 kPa, and 7.69 kPa, respectively. From −10°C to −5°C, the frost heave force decreased by 3.44 kPa (22.3%). From −5°C to −0°C, the frost heave force decreased by 4.31 kPa (27.9%). The frost heave amount at −10°C, −5°C, and 0°C was 18.52 mm, 12.61 mm, and 6.57 mm, respectively. The frost heave amount decreased by 5.91 mm (26.7%) from −10°C to −5°C and by 6.04 mm (27.2%) from −5°C to 0°C.
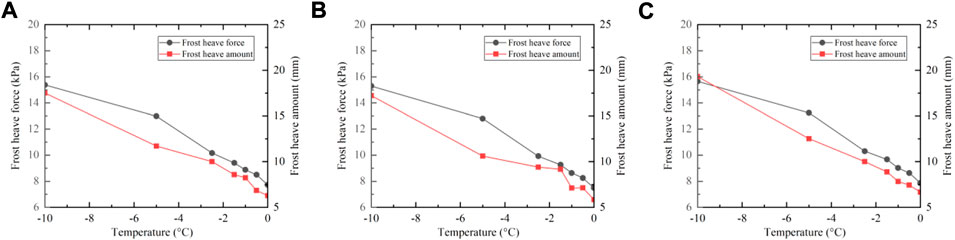
FIGURE 5. Change rules of frost heave force and amount in group C. (A) Section 1. (B) Section 2. (C) Section 3.
Compared to groups A and B, in the group with the No. 1 soil stabilizer, the frost heave force was reduced by 15%–20%, and the frost heave amount was reduced by 14%–21%. The difference between groups B and C showed that the horizontal frost heave force of the soil with Huaxia No. 1 added to it was 5% less than that of soil with lignin fiber, and the variance of frost heave amount was within 5%.
Validation of the model test results
The model test results are translated to the prototype results by applying scale factors. To verify the reliability and safety of the application of the test results in design, the force of the prototype results and the force valued by the design method of the code for anti-freezing design of hydraulic buildings GB/T 50662-2011 (2011) are compared. Since the frozen soil with stabilizers has not been considered in the standard, only the results of group A are discussed in this section. The standard divides the degree of frost heave into five levels, and the corresponding frost heave amount and force are given for each level. Due to the large interval between the values given in the table, linear interpolation is required to obtain accurate data. A comparison of the horizontal frost heave force is shown in Table 4.
The table shows that the horizontal frost heave force of the prototype results is slightly larger than the horizontal frost expansion force calculated by the design method of the standard, and the overall relative error is within 5%. Therefore, the results from the tests can ensure safety and applicability in design.
Fitting relation of frost heave
A reliable and simple relationship between frost heave force and frost heave amount is significant to promote further application of the test results in engineering. Referring to previous experimental studies, power function can be used to fit the relationship between the horizontal frost heave force and amount (Zhou, 1993). Therefore, the power function is selected for the fitting curve. MATLAB was used to fit the frost heave force and frost heave force under different conditions. The fitting results are shown in Figure 6.
The fitting formula is F=axb, where F is horizontal frost heave force, x is frost heave amount, and a and b are parameters to determine. When no soil stabilizers are added, a is 0.022, b is 0.68, and the fit error is 0.977, which meets the requirements. It can be seen that the force increases nonlinearly with the increase of frost heave, with the change rate gradually decreasing. When lignin fiber and Huaxia No. 1 are added, a in the formula becomes 0.019 and 0.0018, respectively. The coefficient b remains unchanged. Therefore, the relationship between frost heave force and frost heave amount under the experimental condition can be expressed by the equation F=ax0.68.
Discussion
The temperature effect of frost heave has been investigated in model tests.
When ice crystals were formed via low temperatures, they disrupted the linkage between soil particles and squeezed them into a new skeleton. As the structure constrains the frost heave, the frozen soil will exert frost heave force on the constrained body. At the same time, the original small pores will also penetrate each other due to the extrusion, forming larger pores. The transition from ice crystals to free water increases with the increase in temperature, objectively resulting in the lift of pore water pressure and reduction of effective stress. Thus, the horizontal frost heave force and amount decrease with the increase in temperature. However, the expansion of pores cannot be recovered immediately after the ice crystals thaw. With the continuous increment of temperature, the soil skeleton recovers faster. Therefore, horizontal frost heave force dissipated faster with the increase in soil temperature.
After the lignin fiber is mixed with the soil, it is interlaced into nets or clusters. The frost heave can induce dislocation between the fiber and the soil. As a single fiber is pulled, the joint force of the adjacent fibers will be affected, forming a three-dimensional force structure so that the load can be evenly dispersed to other areas. In this case, the interface forces are mainly the friction and bonding force between the fiber and the soil. In addition, the fiber can effectively limit the sliding of the soil. This restriction effect is more significant when the fiber is interlaced in a network structure. Therefore, lignin fiber can redistribute the uneven frost heave force in the soil, thereby inhibiting the local deformation caused by frost heave.
Huaxia No. 1 soil stabilizer consists of inorganic materials as the main agent and a small amount of activator. The activator can catalyze the hydration reaction between the inorganic stabilizer and the soil to generate a reticulated C–S–H gel. The gel can cement the dispersed soil particles, increase the viscosity of the pore water, prevent the unfrozen water from migrating to the freezing edge, and weaken the soil permeability and water migration, inhibiting the frost heave.
For inner model tests, the boundary effect caused by the model size will affect the accuracy of the test results. It should also be noted that cable tunnels in engineering experience complicated force conditions. In seasonal cold regions, cable tunnels are affected by freeze–thaw cycles. Therefore, work on horizontal frost heave force under different freeze–thaw cycles should be carried out, and it is suggested that more in situ testing should be conducted.
Conclusion
The experimental study on the effect of frost heave force on the open-cut tunnel was carried out, and the frost heave characteristics of frost heave with different freezing temperatures and different soil stabilizers were investigated. The main conclusions are as follows:
1) The frost heave force is closely related to the freezing temperature. The transition from ice crystals to free water increases with the increase in temperature, objectively resulting in the lift/elevation of pore water pressure and reduction of effective stress.
2) Soil improvement is an effective way to reduce horizontal frost heave force and ensure the safety of the structures in engineering. Lignin fiber, a representative of physical improvement, can redistribute the uneven frost heave force in the soil, thereby inhibiting the local deformation caused by frost heave. Huaxia No. 1 soil stabilizer can cement the dispersed soil particles, increase the viscosity of the pore water, prevent the unfrozen water from migrating to the freezing edge, and weaken the soil permeability and water migration, inhibiting the frost heave.
3) The fitting relation expression between horizontal frost heave force and frost heave amount was obtained. A more accurate value of frost heave force in the design of open-cut tunnels in cold regions can be obtained by this expression than existing value methods in standards.
Data availability statement
The raw data supporting the conclusion of this article will be made available by the authors without undue reservation.
Author contributions
ZM-Y: putting forward the conceptualization and methodology; LH-Y, YL-D, and LX-Z: conducting the model test; WZ-H and XT-D: writing and reviewing the manuscript.
Conflict of interest
ZM-Y, LH-Y, YL-D, and LX-Z were employed by Northeast Electric Power Design Institute Co., Ltd., of China Power Engineering Consulting Group.
The remaining authors declare that the research was conducted in the absence of any commercial or financial relationships that could be construed as a potential conflict of interest.
Publisher’s note
All claims expressed in this article are solely those of the authors and do not necessarily represent those of their affiliated organizations, or those of the publisher, the editors, and the reviewers. Any product that may be evaluated in this article, or claim that may be made by its manufacturer, is not guaranteed or endorsed by the publisher.
References
Bai, B., Yang, G. C., Li, T., and Gao-sheng, Y. (2019). A thermodynamic constitutive model with temperature effect based on particle rearrangement for geomaterials. Mech. Mater. 139, 103180. doi:10.1016/j.mechmat.2019.103180
Chen, C., Guo, W., and Ren, Y. X. (2020). Properties and microscopic analysis of lignin fiber-reinforced soils under freeze-thaw cycles. Chin. J. Geotechnical Eng. 42 (S2), 135–140. doi:10.1016/j.coldregions.2017.11.010
Chen, F. X., Li, N., and Chen, G. D. (2002). The theoretical frame of multi-phase porous medium for the freezing soil. Chin. J. Rock Mech. Eng. 24 (2), 213–217. doi:10.6052/0459-1879-2005-2-2003-039
Ding, J. K. (1983). Workshop on the 2nd national conference of permafrost. Lanzhou: The geographical society of China and China civil engineering society.
Eskişar, T., Altun, S., and Kalipcilar, İ. (2015). Assessment of strength development and freeze-thaw performance of cement treated clays at different water contents. Cold Regions Sci. Technol. 111, 50–59. doi:10.1016/j.coldregions.2014.12.008
Harlan, R. L. (1973). Analysis of coupled heat-fluid transport in partially frozen soil. Water Resour. Res. 9 (5), 1314–1323. doi:10.1029/wr009i005p01314
He, M., Feng, X. P., and Li, N. (2018). Improvement of coupled thermo-hydro-mechanical model for saturated freezing soil. Chin. J. Geotechnical Eng. 40 (7), 1212–1220.
He, M., Li, N., and Liu, N. F. (2012). Analysis and validation of coupled heat-moisture-deformation model for saturated frozen soils. Chin. J. Geotechnical Eng. 34 (10), 1858–1865.
Horiguchi, K. (1987). An osmotic model for soil freezing. Cold Reg. Sci. Technol. 14, 13–22. doi:10.1016/0165-232x(87)90040-1
Hotineanu, A., Bouasker, M., Aldaood, A., and Al-Mukhtar, M. (2015). Effect of freeze-thaw cycling on the mechanical properties of lime -stabilized expansive clays. Cold Regions Sci. Technol. 119, 151–157. doi:10.1016/j.coldregions.2015.08.008
Lai, J., Wang, X., Qiu, J., Zhang, G., Chen, J., Xie, Y., et al. (2018). A state-of-the-art review of sustainable energy based freeze proof technology for cold region tunnels in China. Renew. Sustain. Energy Rev. 82, 3554–3569. doi:10.1016/j.rser.2017.10.104
Li, L., Shao, W., Li, Y., and Cetin, B. (2015). Effects of climatic factors on mechanical properties of cement and fiber reinforced clays. Geotech. Geol. Eng. (Dordr). 33 (3), 537–548. doi:10.1007/s10706-014-9838-4
Liu, H. X., Zhu, W. Z., Zhu, G. X., Gao, A. D., Zeng, S. X., et al. (2000). Relationship between theory og frost heave and frost heaving force. J. Glaciol. Geocryol. 3 (2), 73–74.
Liu, J. K., Tong, C. J., and Fang, J. H. (2005). Introduction of geotechnical engineering in cold area. Beijing,China: China Railway Publishing House.
Olgun, M. (2013). The effects and optimization of additives for expansive clays under freeze-thaw conditions. Cold Regions Sci. Technol. 93, 36–46. doi:10.1016/j.coldregions.2013.06.001
Taylor, G. S., and Luthin, J. N. (1978). A model for coupled heat and moisture transfer during soil freezing. Can. Geotech. J. 15 (4), 548–555. doi:10.1139/t78-058
Tong, C. J., and Guan, F. N. (1985). Prevention and control soil frost heave in building. Beijing, China: Water Conservancy and Hydropower Press.
Wang, T. X., Li, N., and Xie, D. Y. (2005). Necessity and means in research on soil coupled heat-moisture-stress issues. Rock Soil Mech. 26 (3), 488–493. doi:10.16285/j.rsm.2005.03.031
Xu, M. B., Su, J. L., Te, H., Hu, Z. G., Li, A. G., et al. (2011). Code for anti-freezing design of hydraulic buildings GB/T 50662-2011. Beijing, China: China Planning Press.
Yang, Q. W., Pei, X. J., and Huang, R. Q. (2019). Research on the effect of freeze and thaw cycles on the property and damage mechanism of M-CMC stabilized soil. Chin. J. Rock Mech. Eng. 38 (1), 3102–3113.
Zhang, Y. J., Yu, C. X., Ling, F., Wenping, Y., Xu, L., and Bo, C. (2015). Experimental study on asbestos fiber reinforced fly ash soil-cement for soft soil enhancement. J. Eng. Geol. 23 (5), 982–988. doi:10.13544/j.cnki.jeg.2015.05.024
Keywords: horizontal frost heave force, open-cut tunnel, Huaxia No. 1 soil stabilizer, lignin fiber, model test
Citation: Meng-Ya Z, Hong-Yi L, Li-Dong Y, Xing-Zhou L, Zi-Hao W and Tang-Dai X (2023) Experimental study on horizontal frost heave force on open-cut tunnels in seasonal frozen regions. Front. Earth Sci. 10:1022058. doi: 10.3389/feart.2022.1022058
Received: 18 August 2022; Accepted: 31 October 2022;
Published: 10 January 2023.
Edited by:
Chong Xu, National Institute of Natural Hazards, Ministry of Emergency Management, ChinaReviewed by:
Alfredo Reder, Euro Mediterranean Center on Climate Change, Ca’ Foscari University of Venice, ItalyBing Bai, Beijing Jiaotong University, China
Danqing Song, Tsinghua University, China
Lan Cui, Institute of Rock and Soil Mechanics, Chinese Academy of Sciences (CAS), China
Sujatha Evangelin Ramani, SASTRA University, India
Copyright © 2023 Meng-Ya, Hong-Yi, Li-Dong, Xing-Zhou, Zi-Hao and Tang-Dai. This is an open-access article distributed under the terms of the Creative Commons Attribution License (CC BY). The use, distribution or reproduction in other forums is permitted, provided the original author(s) and the copyright owner(s) are credited and that the original publication in this journal is cited, in accordance with accepted academic practice. No use, distribution or reproduction is permitted which does not comply with these terms.
*Correspondence: Wang Zi-Hao, MjIxMTIwMDJAemp1LmVkdS5jbg==