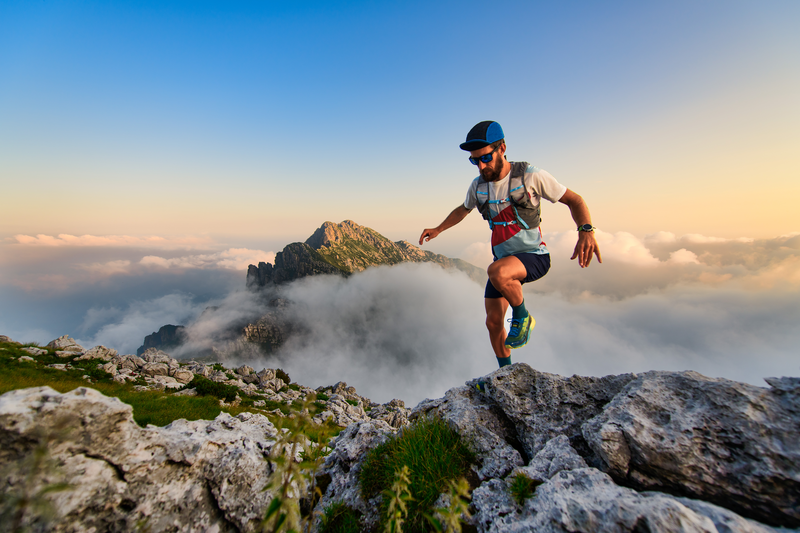
94% of researchers rate our articles as excellent or good
Learn more about the work of our research integrity team to safeguard the quality of each article we publish.
Find out more
EDITORIAL article
Front. Earth Sci. , 21 September 2022
Sec. Cryospheric Sciences
Volume 10 - 2022 | https://doi.org/10.3389/feart.2022.1021292
This article is part of the Research Topic Debris-Covered Glaciers: Formation, Governing Processes, Present Status and Future Directions View all 20 articles
Editorial on the Research Topic
Debris-Covered Glaciers: Formation, Governing Processes, Present Status, and Future Directions
Considerable parts of the ablation zone of many high mountain glaciers are covered with supraglacial debris. The presence of supraglacial debris complicates the interplay of processes linking climate change, topography, and glacier dynamics. Debris-covered glaciers (DCGs) thus differ significantly from their clean-ice counterparts, as they form a more complex system of forcing factors, couplings, and feedback mechanisms that are yet to be fully understood. Resolving the uncertain response and evolution of debris-covered glaciers is vital for devising sustainable management strategies for freshwater availability, glacier-related hazards, hydro-power generation, and also for more precise estimation of their contribution to eustatic sea level changes. The articles in this Research Topic cover conceptual, modelling, and observational approaches to study DCGs at point to glacier and regional scales.
Several contributions in this Research Topic include summaries of the processes and response characteristics of DCGs and the conceptual ways they differ from their clean-ice counterparts. Using Baltoro Glacier in the central Karakoram as an example, Huo et al. review several key DCG processes and recognise climate forcing, the properties and transport of debris, topography and cryokarst features as key factors controlling glacier behaviour and conclude that their integrated effects govern the ice loss from DCGs. Nicholson et al. highlight the fundamental ways in which debris partially decouples the glacier behaviour from climate forcing and the open challenges that need to be addressed in order to couple these time-dependent feedbacks within a modelling system to fully capture DCG development over time. Nicholson et al. and Mayer and Licciulli both argue that DCGs should not be expected to achieve a steady state in response to constant climatic forcing, making it difficult to interpret modern observations of the fluctuation of debris-covered glaciers on the basis of a changing climate alone.
Thermal properties (surface temperature, thermal inertia/resistance, diffusivity and conductivity) of the debris layer are valuable for understanding multiple DCG issues, particularly melting of the underlying ice. Patel et al. determine spatio-temporal variations in the in situ thermal properties of three glaciers in the Chandra basin of the Western Himalaya and find that debris-surface temperature correlated well with sub-debris temperature, and that both were directly related to near-surface air temperature. Field experiments such as these add to a growing database of empirical measurements of sub-debris melt rates and the effects of altitude and debris-thickness, and measurements of thermal resistance with debris layers of variable thickness. Indeed, Boxall et al. exploit data such as these alongside in situ debris thickness measurements and satellite-derived surface temperature to estimate the supraglacial debris thickness across High Mountain Asia. In contrast to some other regional studies they find at the mountain range scale that elevation and surface velocity are stronger predictors of debris thickness than slope or aspect. Herreid explores the usage and value of thermal data (acquired in field and remotely) for investigating DCG processes on Canwell Glacier, Alaska. He proposes methods for estimating the debris-thickness and bare-ice and sub-debris melt, and then compares results with other published models. This study recommends using thermal images acquired during cloudy conditions and sub-pixel correction of ASTER thermal imagery for enhancing their spatial resolution. Beneath the debris, there are very few measurements of ice thermal properties, but Miles et al. present measurements from several near-surface boreholes (1.5–7.0 m depth) and report the propagation of the winter season cold wave into Khumbu Glacier, Nepal, refining the knowledge on equilibrium line altitude and timings of onset/end of the ablation season in this high elevation Himalayan site, as well as providing more accurate information for thermo-mechanical models of ice-deformation.
Investigating the processes governing debris-transport within, on, and off the glacier surface is important for the extent and spatial distribution of the debris cover, and its evolution, which controls the magnitude of ablation, the genesis of glacier surface morphology, and the long-term glacier geometry (Huo et al.; Moore; Mayer and Licciulli). Huo et al. remind us that supraglacial debris moves due to advective-diffuse processes governed by ice-flow (operational on larger and longer spatio-temporal scales), gravitational movement (applicable at smaller and shorter spatio-temporal scales), and fluvial processes. Moore simulates the evolution of an idealized DCG on the timescale of complete de-glaciation with a model of coupled ablation and downslope debris transport, and finds that debris mobility can hasten ice mass loss compared to immobile debris. Highly mobile debris causes rapid topographic inversions and produces subdued supra- and post-glacial reliefs, whereas low debris mobility is characterised by more pronounced supra- but subdued post-glacial reliefs. Further, an intermediate debris mobility optimizes both postglacial relief and the rate of de-icing, and this novel realization may be an important component of the unexpectedly high mass loss rates of DCGs.
The uneven and hummocky topography of DCGs, which results from differential ablation, is adept at storing melt/rainwater and results in the formation of supraglacial ponds. Surface heterogeneities may also cause pronounced relief changes resulting in debris-slumping, which often expose ice-cliffs covered by a thin veneer of debris. Considered as glacier “melt hotspots,” the dynamics of these cryokarst features and eventually their contribution to glacier ablation are vital for processes governing the evolution of DCGs. Wendleder et al. (Baltoro Glacier, Karakoram; 2016-20), Sato et al. (Trakarding Glacier, Nepal; 2007-2018-2019) Loriaux and Ruiz (Verde Glacier, Chile; 2009-19) and Guo et al. (Halong Glacier, Tibet; August and September 2019) all use high resolution remotely sensed data (via aerial and spaceborne sensors) to monitor the characteristics and dynamics of supraglacial ponds and ice-cliffs. Important observations from these studies which further the existing knowledge about supraglacial ponds and ice-cliffs include:
1. newly formed ice-cliffs have gentle slopes and random aspects as compared to perennial ones, which are relatively steeper and with certain predominant aspect (north-west and south facing),
2. count and areas covered by these cryokarst features are maximum at the beginning of the ablation season and minimum at the end of ablation. Additionally, Wendleder et al. report that the supraglacial ponds fill between mid-April to mid-June and drain between mid-June to mid-September,
3. supraglacial ponds usually occupy gentle and stagnant regions, however, ice-cliffs appear less controlled by flow velocity and gradient,
4. areas surrounding these features are characterised by low surface ice velocities, high surface elevation changes (>2 m yr−1) and high mass loss (1.36 and 1.22 m.w.e.). Ice-cliff backwasting of 7.8 m/month to 24 m yr−1 is reported,
5. ice-cliff edge length strongly correlates with the ice-cliff inclined area, which will enable estimation of the ice cliff inclined area from coarser satellite-based images even without high-resolution DEMs.
Overall, these studies suggest that, besides regional meteorology and surface gradients, the occurrence and seasonality of cryokarst features is significantly related with the englacial and supraglacial hydrological processes, and the new insights will allow further assessment of their overall contribution to DCG mass loss and long term behaviour.
On the glacier-scale, Anderson et al. explore the role of dynamic thinning in DCG mass loss and evolution. They firstly assess the inter-relationship between melt-hotspots, debris-thickness, and ice-dynamics of Kennicott Glacier, Alaska, and subsequently simulate their changes over decadal timescales to understand the pathways of glacier evolution. Glacier bed topography is found to control ice-flow and thus debris expansion, which follows the changes in flow direction. Feedbacks involving supraglacial pond positions, relief, debris characteristics and ice-cliff locations are shown to be instrumental in governing thinning rates, with ice ablation at high-elevation feeding through into decreased ice-flux (and thus emergence) down-glacier. Similar studies on other glaciers will help determine if these relationships are site specific or generalised.
Suitable sub-debris melt and DCG runoff modelling underpins all assessments of downstream hydrological influences of DCGs. Steiner et al. integrate field and remotely acquired data within a distributed energy balance model for estimating the melt rates of Lirung Glacier, Nepal at an hourly scale. They test the sensitivity of their results towards thermal conductivity and debris thickness. They also validate their results with the surface lowering rates from field-derived DEMs. A key finding is that simple modelling approaches do not accurately simulate the diurnal melt pattern, but are capable of quantifying total melt, and therefore have merit for application at catchment scale. Similarly, Billington et al. implement a new 1-D model based on the simultaneous heat and water transfer model on North Changri Nup Glacier, Nepal Himalaya for estimating DCG ablation. The model results simulate the characteristic pattern of decreasing ablation with increasing debris thickness. However, the observed melt rates show little dependence on the debris thickness, possibly due to the influence of a patchy snow-cover at the study site. The study highlights the patchy snow-cover during the ablation season as a possible unquantified source of uncertainty in estimating sub-debris ice melt.
The unique character of DCGs means they respond differently to clean ice glaciers under the same climatic forcing, and observations of these changes are useful in disentangling the primary controls. Racoviteanu et al. (glaciers in the Manaslu region, Nepal; 1970-2019), Majeed et al. (87 glaciers in the Pangong region, Ladakh, Himalaya; 1990-2019) and Falaschi et al. (three glaciers in the Patagonian Andes; 1958–2020) use aerial and satellite remote sensing and limited field data (Racoviteanu et al.), and report recent snapshots (shorter timescale) of climate driven response of debris-covered glaciers in different regions. Key findings from these studies include:
1. DCGs mostly show an increase in debris extent and thickness downglacier, ice deceleration, decrease in slope, persistent negative mass balance, ascending equilibrium line altitude, and open crevasses,
2. spatio-temporal predominance of the cryokarst features and high mass loss around these features,
3. retreat and surface thinning rates on DCGs exceed those on clean-ice counterparts,
4. formation of new proglacial lakes in the vicinity of DCGs are prevalent and that the lake-terminating glaciers experience twice as much thinning compared to land-terminating ones.
Further, based on the analysis of 975 DCGs across HMA, Shea et al. propose a new metric termed as the Debris Emergence Elevation (DEE) for ascertaining the regional debris-covered glacier changes over decadal time scales, which could be used to reconstruct their relative mass changes. This metric appears to have potential for acting as a reliable proxy of DCG status as their geometric response is known to be sensitive to the location at which debris is added to the system or emerges at the surface (Nicholson et al.).
While findings of these shorter-timeframe studies create a base-line dataset for understanding of DCG behaviour and response, they do not elucidate the details of the theory of formation and long-term evolution of DCGs and the relevant controlling processes (Huo et al.; Nicholson et al.). This requires a well-validated and adequately parametrized numerical model that integrates the primary forcing factors, feedbacks and couplings (Huo et al.; Anderson et al.; Nicholson et al.; Mayer and Licciulli). To this end, Nicholson et al. provide a detailed review of the fundamental processes and concepts that control the evolution of DCGs and numerical modelling attempts to simulate the same together with their limitations. They explain the key ways in which debris-cover decouples a glacier system from climate forcing and that development of debris-cover over time should prevent the glacier from achieving a steady state. They also discuss existing bottle-necks of realistic representation of DCGs through modelling approaches. Mayer and Licciulli investigate these aspects in some depth, demonstrating that they have an inherent tendency to switch to an oscillating state (retreat and advance cycles) that would occur even without any variations in climate. The advance phase of such oscillations would end with the separation of the heavily debris-loaded lowermost glacier tongue, at time scales of decades to centuries.
This compendium primarily consolidates the state-of-art knowledge about the processes governing DCG behaviour, highlights the recent insights regarding their evolution, and identifies critical future research directions. This is still a rapidly evolving field, and further research is needed on aspects such as debris-production (variability and emergence), multi-scale hydrological processes, and the long-term coupling that supraglacial debris provides between landscapes, their climate, and DCGs themselves over geological timescales.
AS wrote the first draft of the manuscript. All authors contributed to manuscript revision, read, and approved the submitted version.
The authors declare that the research was conducted in the absence of any commercial or financial relationships that could be construed as a potential conflict of interest.
All claims expressed in this article are solely those of the authors and do not necessarily represent those of their affiliated organizations, or those of the publisher, the editors and the reviewers. Any product that may be evaluated in this article, or claim that may be made by its manufacturer, is not guaranteed or endorsed by the publisher.
Keywords: debris-covered glacier (DCG), debris transport, ablation hotspots, sub-debris ablation, glacier evolution
Citation: Shukla A, Nicholson L, Banerjee A, Fujita K and Quincey DJ (2022) Editorial: Debris-covered glaciers: Formation, governing processes, present status and future directions. Front. Earth Sci. 10:1021292. doi: 10.3389/feart.2022.1021292
Received: 17 August 2022; Accepted: 29 August 2022;
Published: 21 September 2022.
Edited and reviewed by:
Michael Lehning, Swiss Federal Institute of Technology Lausanne, SwitzerlandCopyright © 2022 Shukla, Nicholson, Banerjee, Fujita and Quincey. This is an open-access article distributed under the terms of the Creative Commons Attribution License (CC BY). The use, distribution or reproduction in other forums is permitted, provided the original author(s) and the copyright owner(s) are credited and that the original publication in this journal is cited, in accordance with accepted academic practice. No use, distribution or reproduction is permitted which does not comply with these terms.
*Correspondence: Aparna Shukla, YXBhcm5hLnNodWtsYTIyQGdtYWlsLmNvbQ==
Disclaimer: All claims expressed in this article are solely those of the authors and do not necessarily represent those of their affiliated organizations, or those of the publisher, the editors and the reviewers. Any product that may be evaluated in this article or claim that may be made by its manufacturer is not guaranteed or endorsed by the publisher.
Research integrity at Frontiers
Learn more about the work of our research integrity team to safeguard the quality of each article we publish.