- 1State Key Laboratory of Simulation and Regulation of Water Cycle in River Basin, China Institute of Water Resources and Hydropower Research, Beijing, China
- 2Yinshanbeilu Grassland Eco-hydrology National Observation and Research Station, China Institute of Water Resources and Hydropower Research, Beijing, China
- 3College of Hydrology and Water Resources, Hohai University, Nanjing, China
The adaptability of crops reflects the ability to continue to grow in the changing environment. Its adaptability in adversity plays an important role in its own growth and development. The root system is the main organ for crops to absorb water and nutrients, it can adjust its own morphology, physiological, biochemical to improve its water absorption and thus adapt to drought stress. Previous studies mostly focused on the above ground part of crops, but less on the underground part of crops due to the complexity of root observation. In order to study the adaptability of summer maize under different drought conditions, taking “Denghai 618” as the experimental material, with prototype observation and micro root window as technical support, under the ventilation shed, two treatment groups of light drought (LD) and moderate drought (MD) and the same rehydration after drought are set, as well as the normal water supply for control inside the shed (CS) and Control outside the shed (COS). The changes of root morphology and the law of root water absorption under drought and Rehydration after different drought were analyzed. The results showed that: 1) Under drought stress, the root system of maize adapts to drought by thinning and increasing fine roots to improve root water absorption. Under the MD, the root adaptation to drought is more obvious: promoting root growth. Root biomass was no significant difference between the drought treatment group and the CS. The root biomass of the drought treatment group was significantly lower than that of the COS due to the thinner root system. 2) The total amount of root water absorption in the CS and COS increased steadily with the increase of days. In the drought treatment group, the root water absorption decreased with the increase of drought degree, that is, COS > CS > LD > MD. This study provides a reference for revealing the self-adaptive regulation mechanism of summer maize roots under drought conditions.
1 Introduction
Maize (Zea mays L.) is one of the main food crops in the world, According to the data and statistics of the Food and Agriculture Organization of the United Nations (FAO, 2022), in the past decade, the global average annual maize harvest area was about 190.67 million hectares, yield of maize was about 1.05 billion tons, accounting for about 40%. China is a big maize producer, according to statistics (Lu, 2021), maize planting area and total output reached 44.97 million hectares and 265 million tons, of which the maize planting area accounted for 40.2% of China’s grain planting area. Maize is mainly planted in arid or semi-arid areas, of which more than 35% is planted in the Huang-huai -hai Plain, which is the main maize producing area in China (Liu, 2012). However, in terms of maize production, maize production is mainly limited by drought (Zhou et al., 2011; Li et al., 2019). Drought causes changes in the available water in the soil (Campos et al., 2004; Ren et al., 2014), which will affect the growth and development of maize and eventually lead to yield reduction, the yield of maize plays a major role in ensuring food and feed security (Egamberdiyeva, 2007; Sposito, 2013).
Crop adaptability refers to its own survival ability to regrow in the changing environment. From the perspective of crop adaptation, many scholars analyze the adaptive changes of crops under drought stress from the morphology (Qi et al., 2012; Prince et al., 2022), physiology and biochemistry of crops (Annicchiarico et al., 2012; Hasanuzzaman et al., 2019). In drought stress, crops preferentially provide limited water to the root system, which is an important organ for crops to supplement nutrients and absorb water (Liu et al., 2021). It can first sense changes in the soil environment and then take the initiative to find places with sufficient soil moisture content, mainly by elongating and increasing fine roots to improve the water absorption capacity of the roots and ensure the growth and development of crops themselves (Jiang et al., 2016; Gan and Zha, 2020). Such as Qiao (2018) found that under drought stress, the root length, root diameter and root volume of maize decreased, but the total root biomass, root shoot ratio and root density increased. At this time, the aboveground part adapts to drought through evaporation reduction, which is mainly manifested as wilting and curling (Kadioglu and Terzi, 2007). In terms of crop physiology (Monika et al., 2012; Shemi et al., 2021), drought stress can reduce water loss by changing enzyme activity, gas exchange, leaf water potential, abscisic acid, and chlorophyll content in crops (Mahdid et al., 2011; Nezhadahmadi et al., 2013). Because the observation of the underground part is more difficult than that of the above ground part, the research of crop root system is not as profound as that of aboveground part (Stephanie and Smet, 2012).
In order to deeply understand the adaptive ability of summer maize under drought stress, this study take the root system of summer maize as the breakthrough point, set up different degrees of drought in the field with prototype observation and micro root window as technical support, and analyzed the adaptive changes of root morphology and root water absorption of summer maize. The purpose of this study was to test the following hypotheses: 1) Compared with the CS, MD promoted the root growth of summer maize, and LD was not conducive to root growth. 2) Compared with the CS and COS, the root water absorption of summer maize under drought stress decreased. The research on the adaptation mechanism of summer maize has practical application value, it provides technical support for timely irrigation of farmland and improving the efficiency of water resources utilization, and provides strategic support for coping with drought disasters.
2 Materials and methods
2.1 Overview of the experimental area
The experimental site was selected at the Wudaogou hydrological experimental station (33°09′N, 117°21′E) in Anhui province, which is located in the northern Anhui plain and is the main crop producing area. The crop layout is mainly arid crops, and the farming system is mostly double cropping a year. The soil type is shajiang black soil, which has poor permeability and is characterized by “drought, waterlogging, barren, stiff and sticky,” and the bottom of the plow is thick. The return period of drought in this area is 2–3 years, and the summer is the growing season of maize in this area. Moreover, maize is not only the major crop in the northern Anhui plain, but also the dominant crop in this area.
2.2 Experimental setup
The experiment was carried out in the jointing-tasseling period in 2021. There have been eight experimental fields, including six fields inside the shed and two open-air fields outside the shed to observe the growth law of summer maize under different drought scenarios. Due to the limited experimental fields, two repeated fields were set, but the measurement data (soil moisture content, leaf area index) can ensure three or more repetitions. The size of each experimental field is 5.3 m × 3.7 m, there have been a partition around the field to block the lateral seepage and cross flow between the fields. The depth of the partition in the underground part is 2 m, and the above ground part is 0.4 m higher than the ground level. One soil moisture aluminum tube and two 90° and one 45° micro root tubes were buried in each experimental field to measure soil moisture and root morphology of summer maize at different depths. In this study, “Denghai 618” was taken as the summer maize variety. Before sowing, the experimental field needs to be applied with the same amount of fertilizer, based on 22500 kg·km−2 urea and 75000 kg km−2 maize compound fertilizer. Control the soil water content to reach the same level 5 days before sowing, and measure the soil water content the day before sowing. The summer maize in the field is 6.74 plants m−2, and the row spacing of summer maize in the field is 60 cm, and the plant spacing is 25 cm (Figure 1).
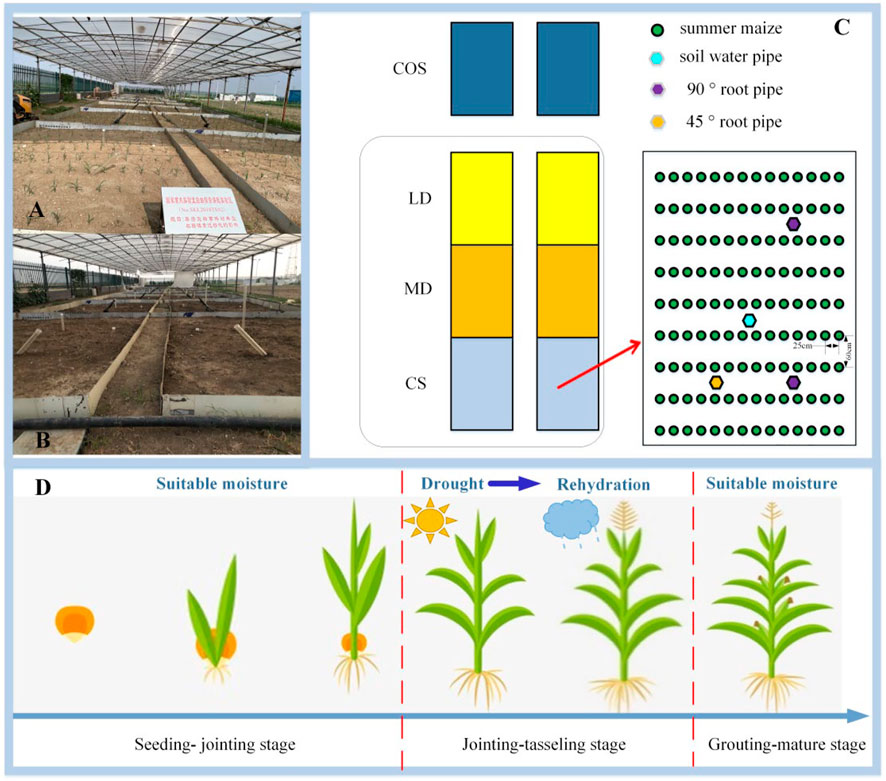
FIGURE 1. Design of experimental field. (A) and (B) is the photo of the experimental field in the shed, (C) is the plane design drawing of the experimental field, (D) is the simplified drawing of the drought experimental design.
The drought grade standard of this study is divided by the soil relative humidity of 10–20 cm soil layer. The calculation formula of soil relative humidity is shown in Eq. 1. In the jointing-tasseling stage in 2021, the experiments of light drought, moderate drought and rehydration after drought and the control group without drought inside and outside the shed are set up. The degree of drought grade is achieved by the number of consecutive days without rainfall. The specific experimental scheme is shown in Table 1.
Calculation formula of soil relative humidity:
where: R is relative humidity of soil (%); w is soil water content (%); f is soil field capacity (%); according to the test of the experimental station for many years, the soil field water capacity is about 30.7%.
2.3 Measurement items
2.3.1 Soil moisture content
In the experiment, soil water content was measured each day with an AIM-WiFi soil multi-parameters monitoring system (Beijing Aozuo Ecology Instrumentation Ltd., Beijing, China). The soil measurement accuracy of the instrument was ±2%, and the measurement repetition accuracy was ±0.3%. During the test, the soil water content was measured every morning. The measured value of each experimental field includes nine soil depths, measured from shallow to deep, starting from 10 cm layer, measuring every 10 cm layer, measuring to 90 cm. In the test, the measurement of each depth is repeated three times, and the test data is taken as the average value of three times. It shows the monitoring and measurement photos of soil moisture content (Figure 2A).
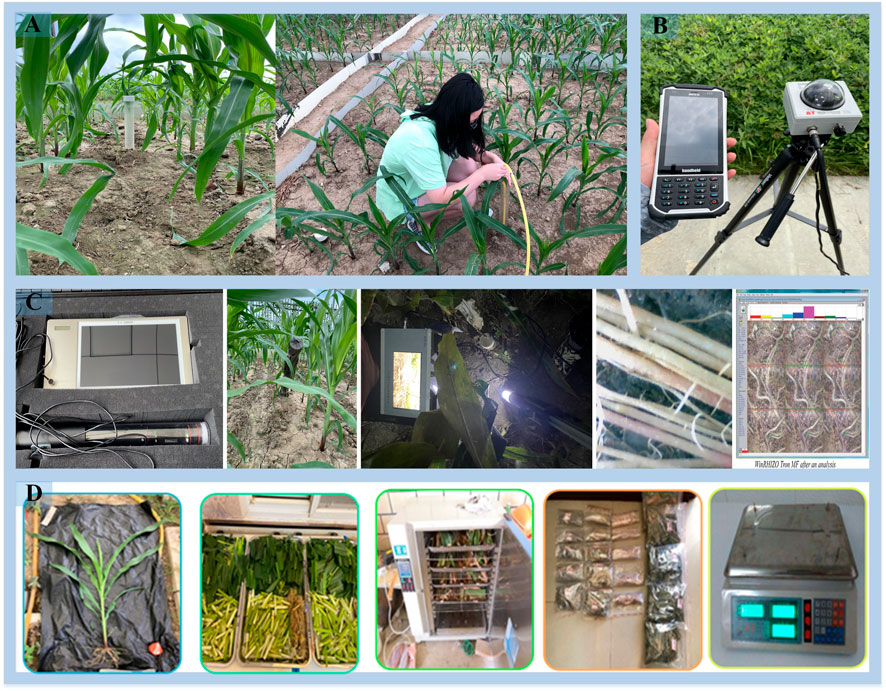
FIGURE 2. Experimental monitoring diagram of summer maize determination project. (A) is the monitoring and measurement photo of soil water content, (B) is the measurement instrument of leaf area index, (C) is the monitoring and measurement diagram of summer maize root system, and (D) is the sampling procedure diagram of summer maize biomass.
2.3.2 Root morphology
The root morphology monitoring instrument is AZR-100 root ecological detection system (Beijing Aozuo Ecology Instrumentation Ltd., Beijing, China). The main features of the instrument are to use the minirhizotron technology to observe the growth and development of maize roots at fixed points under the condition of ensuring that the roots are not damaged. It shows the experimental monitoring diagram of summer maize roots (Figure 2C). Summer maize was observed on 7/8/2021, 15/8/2021, 23/8/2021, 8/9/2021, 17/9/2021, and 27/9/2021 respectively. A total of eight directional images were monitored for the same layer of root system, and each image was obtained by rotating 45°. The obtained images are analyzed by professional software to obtain the root morphological parameters under different soil depths. The relevant calculation formula of root morphological parameters is as follows (Table 2).
2.3.3 Leaf area index
During the whole experiment, the leaf area index of summer maize was measured and recorded by using the Sunscan leaf area index measuring instrument (Figure 2B) from 9 to 11 a.m. or 1–3 p.m. (GMT+8) on a sunny day. Each experimental field was measured three times, once every 5–7 days.
2.3.4 Biomass
In this subject, in order to observe the change characteristics of growth morphology during the growth period of summer maize and determine the biomass of various organs of summer maize, first dig the whole maize plant with a shovel, then clean the root soil, wipe out the water stains, separate and sort out the various organs of maize, dry them in the drying box (the temperature is set to 105°C), and then weigh them, and finally get the biomass. It shows the sampling steps of summer maize biomass (Figure 2D).
2.3.5 Root water absorption
In order to understand the water absorption characteristics of summer maize roots under different drought conditions, we introduce a macro root water absorption model to reflect the water absorption of roots under different drought conditions. The model ignores the mechanism process of root water absorption and the hydraulic characteristics of roots. The basic principle of the model is Eq. 2:
where: θ is the soil moisture content; h is the pressure head, cm; T is the time, z is the length of water flow path, cm; S (z, t) is the root water absorption term; K is the unsaturated permeability coefficient.
Among them, root water absorption Eq. 3:
where: TP is the potential transpiration rate of plants; b(z) is the standardized root water absorption function; α(h) is the function of soil water stress.
VG (Genuchten and Th, 1980) model is used to describe the relationship between pressure head, soil moisture content and unsaturated permeability coefficient, Eqs 4–7:
where: θs is the saturated water content; θ is the soil moisture content; θr is residual water content; Se is relative saturation; Ks is the saturated permeability coefficient of soil; α, n, m is the empirical fitting coefficient, and l is taken as 0.5.
According to the data of soil particle gradation and soil bulk density in the study area (Wang et al., 2011), the characteristic parameters of soil moisture are predicted, as shown in Table 3.
Most researchers use Feddes model (Feddes et al., 1974) to simulate root water absorption. Combined with the situation of this study, this study also uses Feddes model to reflect root water absorption, and its formula is Eq. 8:
where: α(h) is a function reflecting the influence of soil water potential on root water absorption rate, 0 ≤ a ≤ 1; h1 is the anaerobic point of root water absorption; h2 is the soil matrix potential at the beginning of the optimal point of root water absorption; h3 is the soil base potential at the end of the optimal point of root water absorption; h4 is the soil matrix potential at the withering point of root water absorption.
The parameters provided by Hydrus-1D are selected as the water absorption parameters of maize roots in this paper (Table 4). The function of the effect of soil water potential on root water absorption rate (Figure 3).
The potential transpiration rate TP of plants refers to the soil water content absorbed by plants in unit time under ideal conditions. The potential transpiration rate of plants is calculated by using extinction coefficient and leaf area index (Allen et al., 1998), and its formula is Eqs 9, 10:
Where: ETc is the potential evapotranspiration of plants, cm d−1; Es is the potential evaporation of soil. Refer to relevant literature (Kang et al., 2009), and take 0.36 cm d−1; LAI is the crop leaf area index, and the measured value during the experiment is taken; k is the extinction coefficient, 0.4 for maize. Parameters of summer maize under different drought conditions as follow (Table 5).
The distribution of roots in the vertical direction can be constant, or expressed by linear or non-linear functions (Deng et al., 2015), exponential decline functions (Prasad, 1988) and piecewise functions (Wang et al., 2006). According to the relationship between measured root length density and soil depth, we choose piecewise linear function to reflect the distribution of roots in the profile (Figure 4). After linear interpolation fitting, the root length density distribution function is obtained, as shown in Table 6.
Where: b’(z) is the distribution of measured roots in the vertical direction, b(z) is the standardized root water absorption function.
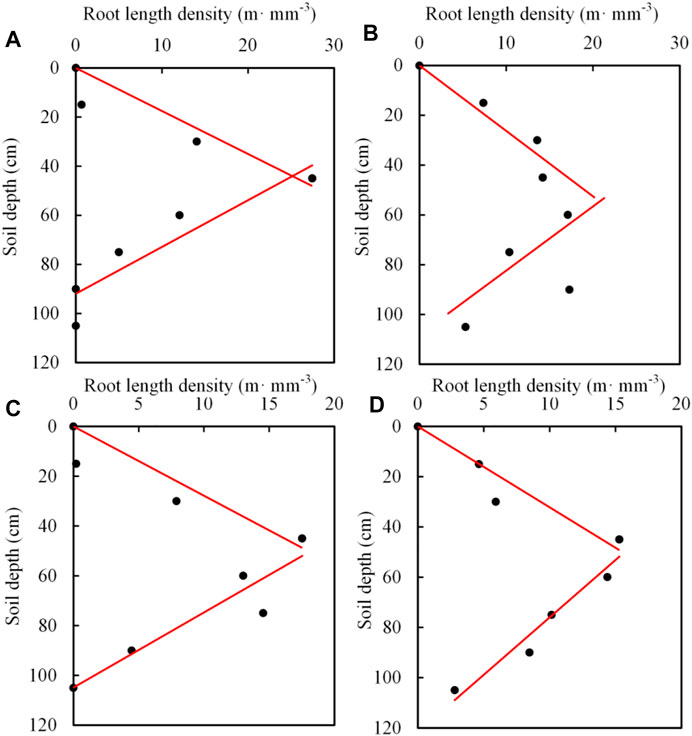
FIGURE 4. Distribution of root length density of summer maize under different drought conditions.(A) (B) (C), and (D) are the distribution map of root length density of LD, MD, CS, COS.

TABLE 6. Distribution function of root length density of summer maize under different drought conditions.
Set the relevant parameters in Hydrus-1D software. The specific steps can refer to the relevant literature (Wu and Huang, 2011; Ding et al., 2020), and finally obtain the simulated soil water content data. Relative deviation and overall deviation are used to measure the deviation distribution of soil water content in the vertical direction of soil (Table 7). The relative deviation and overall deviation are calculated according to Eqs 12, 13:
Where:
Where: β is overall deviation of soil moisture content; N is Total.
Through the calculation of the measured and simulated values of soil water content under different drought scenarios, it is found that the overall deviation between the simulated and measured values is small, and the distribution of the simulated and measured values is in good agreement. It can be used to simulate the water absorption characteristics of summer maize under different drought scenarios.
2.4 Statistical analysis
The obtained data were statistically calculated and processed by the Microsoft Excel 2010, and the data were analyzed by one-way ANOVA and LSD method with the IBM SPSS statistics 26 (α=0.05), the experimental data results are expressed as average values, and the graph is drawn in Origin 2018.
3 Results
3.1 Root morphology
3.1.1 Overall changes of root system
Through comparative analysis of root morphological parameters of summer maize under different drought and post drought rehydration scenarios, it was found that root length (Figure 5A), root surface area (Figure 5B), root average diameter (Figure 5C) and root volume (Figure 5D) of summer maize increased gradually with time in the whole growth period, peaked in late August and early September, and then gradually decreased with time, showing an inverted "V' shape change; The number of root tips (Figure 5E) showed a steady increasing trend with time throughout the measurement period.
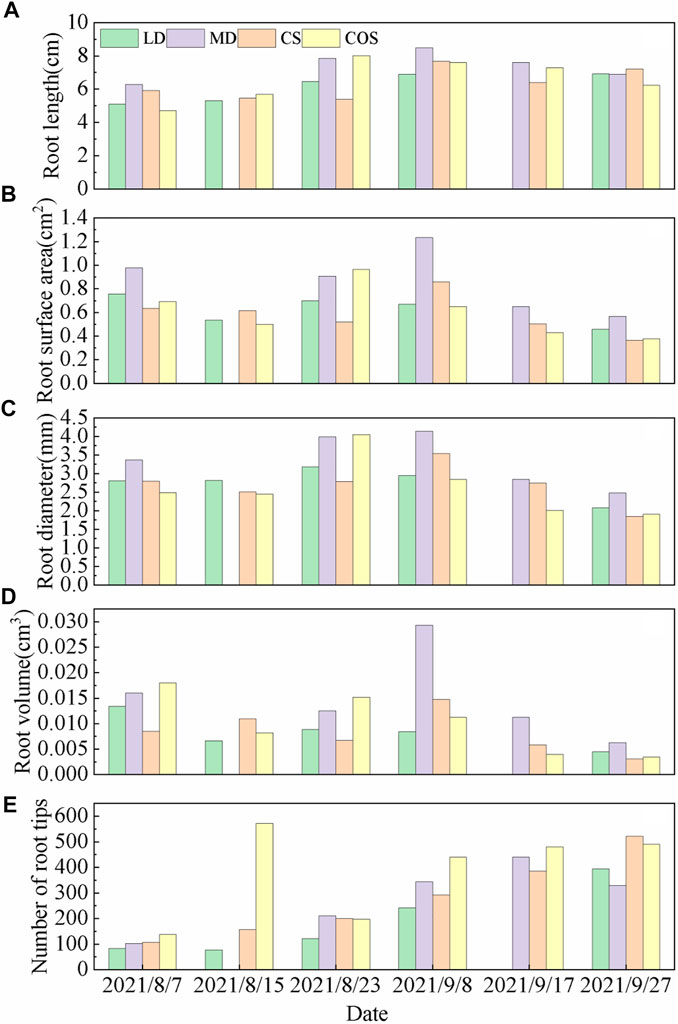
FIGURE 5. Dynamic changes of root morphological parameters of summer maize under different drought conditions. (A–E) are the dynamic changes of root length, root surface area, root diameter, root volume and number of root tips, respectively. (since only drought and post drought rehydration experiments were conducted, the middle drought group on 8/15 and the light drought group on 9/17 was not measured. The unmeasured data did not affect the experimental results).
Under the MD during jointing-tasseling stage, it is conducive to root growth, and the root morphology index is higher than that of the CS. The root length, root diameter, root surface area, root volume and root tip number of summer maize are 45.49%, 43.48%, 75.12%, 86.53%, and 5.25% higher than those of the CS respectively. The root length, root surface area, root diameter and root volume of the MD are lower than those of the COS, which are 2.15%, 6.03%, 1.42%, and 17.29% lower respectively. Under the LD, the root length, root surface area, root volume and number of root tips were 2.60%, 12.70%, 39.05%, and 50.48% lower than those of the CS, and 6.46%, 18.48%, and 86.36% less than those of the COS.
Rehydration after light drought (RLD), the root length, root surface area, root diameter and root volume of summer maize were higher than those of the CS, which were 19.73%, 34.97%, 14.38%, and 31.92% higher respectively, but the root length, root surface area, root volume, root diameter and number of root tips were lower than the COS, which were 19.48%, 27.58%, 41.51%, 21.41%, 38.13% less respectively. Rehydration after moderate drought (RMD), the root length, root surface area, root diameter, root volume and the number of root tips were higher than those of the CS and COS, which were 10.70%, 43.76%, 16.99%, 98.24%, and 17.98% higher than those of the CS respectively, which were 11.68%, 89.56%, 45.43%, 41.51%, and 160.63% higher than those of the COS.
3.1.2 Changes of root system in different layers
The root length distribution of summer maize at different depths under different drought scenarios is shown in Figure 6. It can be seen that the root length distribution law increases with the increase of depth, and then decreases with the increase of depth. The root length is mainly distributed in the 45–75 cm layer, and the 45–75 cm root length accounts for 48.91%–78.19% of the total root length. Under the action of drought, it is beneficial to improve the root length of 15–30 cm layer. The root length of 15–30 cm layer in LD (Figure 6A) and MD (Figure 6C) is 81.18% and 148.25% higher than that in the CS, and 39.39% and 37.89% higher than that in the COS respectively. The root length below 60 cm in the LD was lower than that in the CS and COS, which was 46.76% and 52.41% lower than that in the CS and COS, respectively. However, the root length below 60 cm in the MD was higher than that in the CS and COS, which was 5.18% and 5.15% lower than that in the CS and COS, respectively.
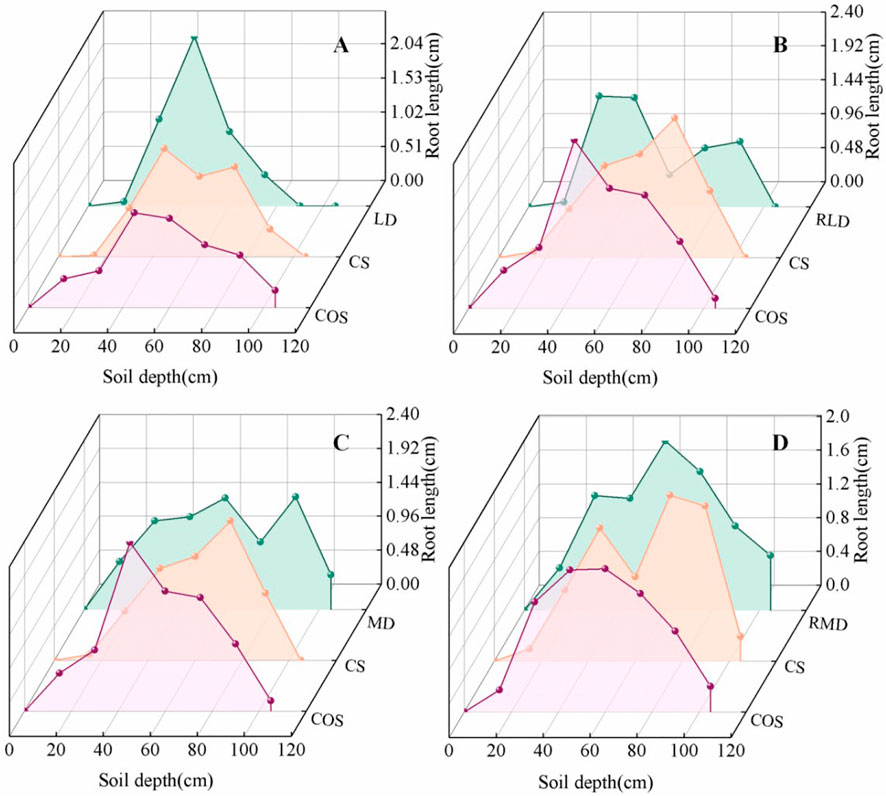
FIGURE 6. Vertical distribution characteristics of root length of summer maize under different drought and post drought rehydration. (A–D) are the vertical distribution characteristics of root length of LD, RLD, MD, and RMD, respectively.
The 15–30 cm root length of RLD (Figure 6B) and RMD (Figure 6D) was 110.10% and 88.39% higher than that of the CS, and 17.19% and 19.24% higher than that of the COS, respectively. The root length of the RLD below 60 cm did not recover to the level of the CS and COS, which was lower 49.52% and 49.53% respectively. The root length of the RMD under 60 cm was 3.85% and 21.58% higher than that of the CS and COS respectively.
3.1.3 Changes of roots with different diameters
Through the observation of the whole growth period (Figure 7), it is found that the root length of summer maize is mainly 0–0.5 mm in diameter, and the root would become thinner and the proportion of fine roots (0–1.5 mm in diameter) would increase in the drought of jointing-tasseling period. Under the LD (Figure 7B), the root lengths of summer maize with root diameters of 0–0.5 mm, 0.5–1 mm, 1–1.5 mm and greater than 1.5 mm accounted for 84.65%, 12.78%, 2.37%, and 0.2% respectively. Under the LD, the proportion of fine roots accounted for 99.8%, which was 4.36% more than the CS in the same period and 1.91% more than the COS in the same period. Under the MD (Figure 7C), the root lengths of summer maize with root diameters of 0–0.5 mm, 0.5–1 mm, 1–1.5 mm and greater than 1.5 mm accounted for 74.95%, 20.28%, 4.24%, and 0.53% respectively, and the proportion of fine roots (0–1.5 mm diameter) accounted for 99.47%, 0.64% more than the CS in the same period and 1.61% more than the COS.
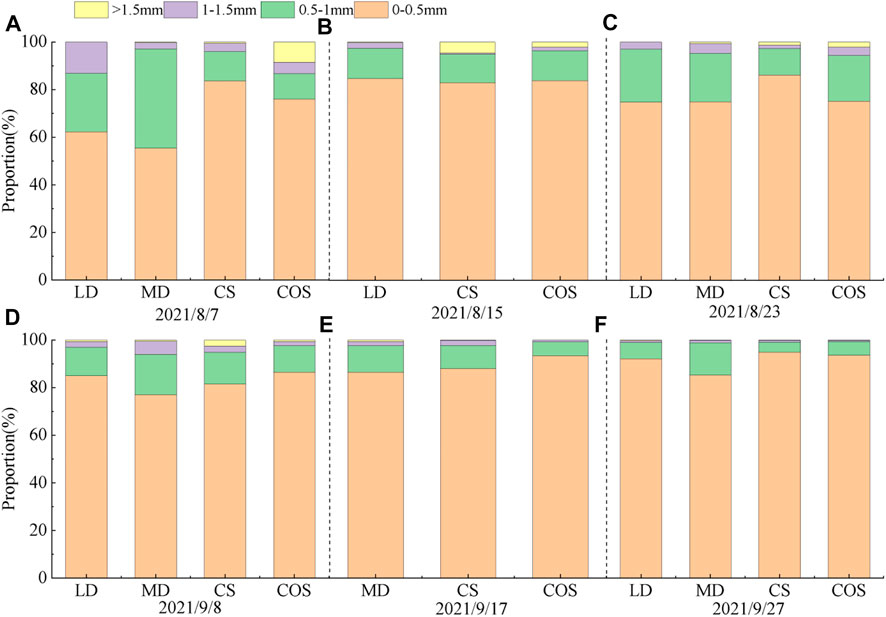
FIGURE 7. Proportion of root length of different diameter classes under different drought conditions. (A–F) are the proportion of root length with different diameters in 8/7, 8/15, 8/23, 9/8, 9/17, 9/27 in 2021, respectively.
Rehydration after drought, the proportion of fine roots increased and was higher than that of the control group. After RLD, the proportion of fine roots increased by 0.2% compared with that of drought, which was 1.17% and 2.14% higher than the CS and COS in the same period, respectively. The proportion of fine roots in RMD (Figure 7D) changed little compared with that during drought, which was 1.95% and 0.09% higher than that of the CS and COS in the same period, respectively.
3.1.4 Changes in root biomass
The root biomass of summer maize under different droughts during jointing-tasseling stage was compared and analysed (Figure 8). The two drought scenarios designed in this experiment led to higher root biomass than the CS, but there was no significant difference, which was significantly lower than the COS, the root biomass of LD and MD was 74.12% and 81.82% lower than that of the COS. The root biomass increased after rehydration. The root biomass in RLD and RMD increased by 5.16% and 71.48% respectively compared with that in drought.
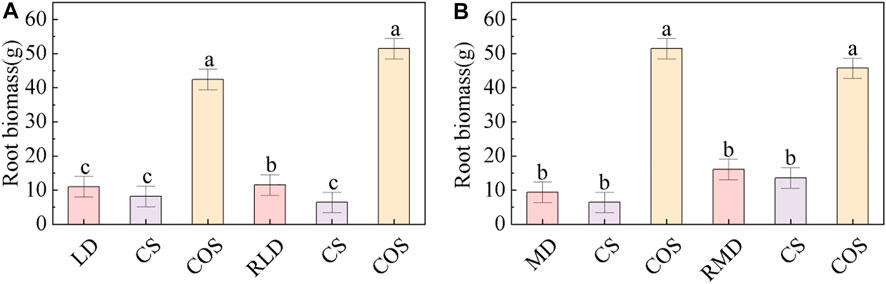
FIGURE 8. Comparison of root biomass of summer maize under different drought and drought rehydration conditions. (A) is the root biomass under LD and RLD, (B) is the root biomass under MD and RMD. Different small letters in the figure indicate that the difference between treatments is significant (p < 0.05).
3.2 Changes of root water absorption
The time-dependent changes of root water absorption under different scenarios are shown in Figure 9. The total root water absorption of the CS and COS increases steadily with time. This is because the control group has fewer days without rainfall, and the soil water content can be guaranteed within the appropriate range. The root water absorption of the CS (Figure 9C) and the COS (Figure 9D) is 3.4 mm d−1 and 3.6 mm d−1. In the LD (Figure 9A), the days without rainfall were more than 30 days, and the root water absorption was about 3.37 mm d−1 in the first 15 days. After 15 days, with the increase of the days without rainfall and effect of the root water absorption, the soil water content decreased, and the root water absorption decreased to 2.38 mm d−1. The MD (Figure 9B) continued 46 days without rainfall. The early stage of root water absorption was similar to that of LD, the root water absorption was 3.02 mm d−1 in the first 13 days, and decreased to 2.36 mm d−1 after 13 days. After 37 days, the total root water absorption increased little with time. At this time, the root water absorption was only 0.54 mm d−1. By comparing the root water absorption under different drought scenarios, it was found that the root water absorption decreased with the increase of drought degree, that is, COS > CS > LD> MD.
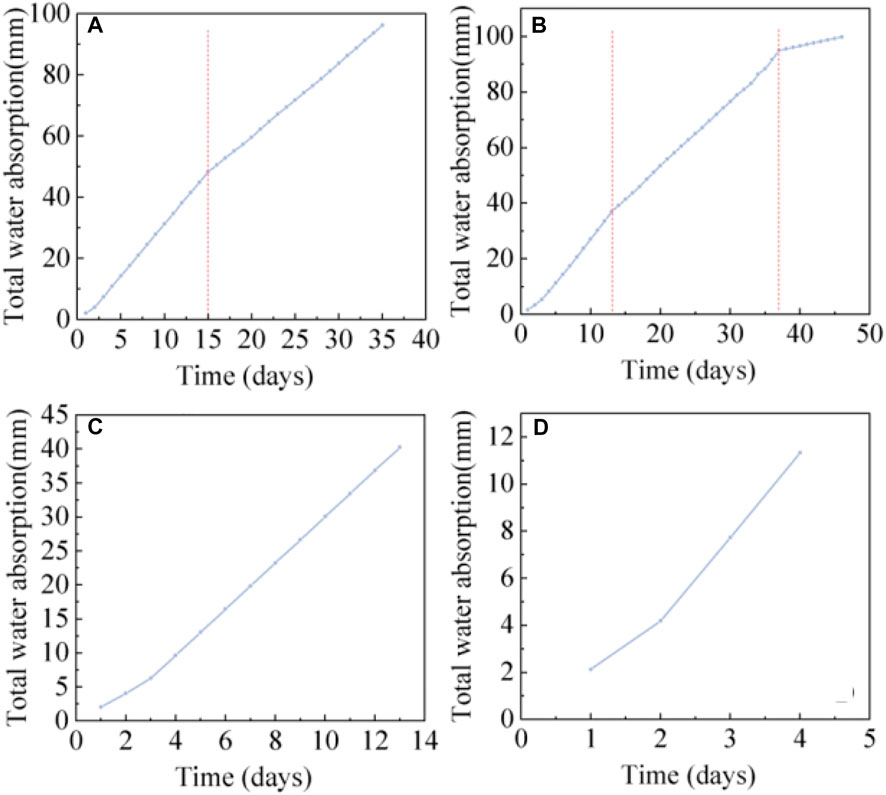
FIGURE 9. Variation of total water absorption of roots under different drought conditions. (A), (B), (C), and (D) are the total water absorption changes of roots in LD, MD, CS, COS, respectively.
4 Discussion
4.1 Adaptive ways of root morphology under drought stress
Soil moisture is very important for root growth (Mthandi et al., 2013). Under drought stress, crops themselves will actively adjust their roots to look for water in places with sufficient soil moisture. Crop roots mainly improve the water absorption of roots through two changes to ensure their own development and growth (Lal, 1979; Cairns et al., 2011). One is to reduce the consumption of photosynthetic accumulation by thinning and elongation, and increase the root length, which is conducive to striving for deeper soil moisture, so as to alleviate the burning of plants caused by water deficiency (Shan and Liang, 2007). The second is to ensure the normalization of aboveground morphology and function by increasing lateral roots and fine roots to absorb more water to adapt to stress (Tran et al., 2014; Ng et al., 2018). This study (Figure 5) found that MD promoted root growth, which is consistent with the research of some scholars: appropriate drought conditions are conducive to root growth (Dong et al., 2016; Wang et al., 2018). However, the root length of summer maize under the LD was not elongated compared with the CS. The reason may be that the number of days without rain in LD was less than that in MD, and the degree of water stress was insufficient to meet the requirement of root elongation. The root growth can reach the level of the control group after rehydration after drought in jointing-tasseling stage. The reason may be that the root activity is strong, the root adaptation is strong, and the recovery ability is strong. At this time, rehydration can compensate for the drought effect in the early stage. Through the analysis of root length of different diameter classes, this study (Figure 6) found that the proportion of fine roots of summer maize increases under drought stress, which is consistent with the research of some research scholars (Florian et al., 2019; Ahmed et al., 2020), under appropriate drought stress, the proportion of fine root length and root surface area will increase to strengthen water use efficiency, ensure the formation of a large underground system to support the normal operation of the whole plant, enhance adaptability to adverse environment (Smucker and Aiken, 1992; Lorenc et al., 2018).
The spatial distribution of root morphology is mainly affected by soil water content, so the root distribution of soil layers at different depths is different (Petek et al., 2013; Paz et al., 2015). For areas with sufficient rainfall or relatively humid, the root system is generally “shallow root type,” while for areas with water shortage, the surface soil water content is low, The root distribution is generally “deep rooted” (Lilley and Fukai, 1994; Oyanagi et al., 2004) Studies have shown (Qi et al., 2012) that the same crop has a certain amount of water absorption (Duan et al., 2003), but its root distribution is also different in different places. Maize planted in the North has deep roots, with a depth of 60–80 cm soil layer, while maize planted in the South has shallow roots, with a depth of 30–40 cm soil layer (Gao, 2013), or even shallower soil layer. This study (Figure 7) found that the root system first increased with the increase of depth, and then decreased with the increase of depth, mainly distributed in the 45–75 cm layer. This is because the soil type in the study area is shajiang black soil, the soil permeability is poor, the temperature during the growth period of summer maize is high, the soil surface is easy to evaporate, and the water content of the soil surface is more vulnerable to vegetation transpiration and soil evaporation (Kim and Mike, 1995). With the increase of drought degree, it will lead to the decrease of surface soil water content, which is not conducive to root growth and less root distribution. Although the water content of deep soil is high, it is not conducive to the survival of roots because of poor soil permeability and insufficient oxygen supply. In comparison, the evaporation of soil in the middle layer (45–75 cm) is smaller than that in the surface layer, the soil moisture content is relatively high, the oxygen supply is sufficient in the deeper soil, so the root system is distributed more.
Under the action of drought, it not only changes the root morphology and affects the water absorption capacity, but also has a great impact on its own dry matter accumulation. The thinning of the root system directly leads to the reduction of biomass. Biomass reflects the ability of crops to use resources and environment (Walne and Reddy, 2022). The distribution of crop biomass in various organs is not only affected by crop factors, such as crop varieties, crop genetic characteristics, but also affected by environmental factors (Pike et al., 2015). This study (Figure 8) found that root biomass of the drought treatment group was significantly lower than that of the COS due to the thinner root system, which is consistent with the conclusion of Chen et al. (2018): drought inhibits plant growth, root development and dry matter accumulation.
4.2 Adaptive ways of root water absorption under drought stress
Plants mainly rely on their roots to absorb water from the soil and supply physiological activities such as plant growth and development, metabolism and transpiration (Zhang et al., 2022). In arid environments, precipitation cannot meet evaporation, and there is less water in the upper layer of soil. In order to meet their own growth needs, plants are likely to use deep roots to absorb deep soil water or groundwater (Nie et al., 2010). Studies have shown that when crop transpiration is low, generally at night, the water absorbed by roots from deep soil can be released from shallow roots to the surface or sub surface dry soil through root transportation, thus changing the soil water status (Magistad and Breazeale, 1929; Corak et al., 1987). This study found that drought will weaken the ability of root water absorption and reduce the amount of root water absorption (Figure 9), the total root water absorption in the CS and COS increased steadily with the increase of days. In the drought treatment group, the root water absorption decreased with the increase of drought degree. The water absorption capacity of root system is reflected by the water conductivity of root system. Due to the water stress in the drought treatment group, the formation of exoplasmic resistance of water and ion transport was caused, and the radial resistance increased (radial resistance, that is, the resistance of water from the outside to the inside, soil water→ root epidermis→ cortex→ endoderm→ central column→ root xylem), the activity of aquaporin decreased, greatly reducing the root water conductivity (Wang, 2005). Water stress leads to the decrease of soil water potential, the signal material produced by the root system is transported to the aboveground part of the plant, and the leaves begin to reduce transpiration, and wilt and curl will occur, thus inhibiting the photosynthetic rate on the leaf surface, reducing the formation of photosynthetic products and their migration and transformation to the leaves (Bansal and Nagarajan, 1987; Liu and Stützel, 2002). The decrease of transpiration intensity will affect the hydraulic conductivity and lead to the decrease of root water absorption. Some studies (Yan et al., 1964) pointed out that the more roots per unit volume of soil layer, the more beneficial it is for crops to absorb water. Although this study has a certain promoting effect on roots under the MD, the root system in the deep soil is less distributed, and the efficiency of water absorption and utilization in deep soil is not very high, so the water absorption of the root system is lower than that of the CS and COS.
4.3 Adaptive mechanism of summer maize under different drought stress
Based on the analysis of root morphological parameters and root water absorption of summer maize under different drought stress, the explanatory diagram of adaptive mechanism and process of summer maize under different levels of drought was drawn (Figure 10). Different levels of drought stress will cause soil water content to decrease. The greater the degree of drought, the greater the decrease of soil water content, and the soil water content will increase with the increase of depth in the vertical level. The change of soil moisture content makes the root morphology change. In the vertical level, the root system increases with the depth and then decreases. Under the LD (Figure 10B), the root system growth is inhibited, and the root system morphological parameters are smaller than those of the control group. However, under the MD (Figure 10A), the root system of summer maize was more adapted to this environment, which promoted root growth and root morphological parameters were higher than those of the control group. Under drought stress, not only the underground part would change to adapt to this environment, but also the aboveground part would change to adapt to drought. Under drought stress, the aboveground part would reduce transpiration, the wilting and curling of leaves would reduce the leaf area index, affecting the photosynthesis of the aboveground part. The changes of the underground part and the aboveground part would affect the water absorption capacity of the root system, the higher the degree of drought, the lower the water absorption of roots. Under the LD, the decrease of root length, leaf area index and transpiration rate led to the decrease of root water uptake. Under the MD, root length increased, leaf area index and transpiration rate decreased, and root water absorption decreased.
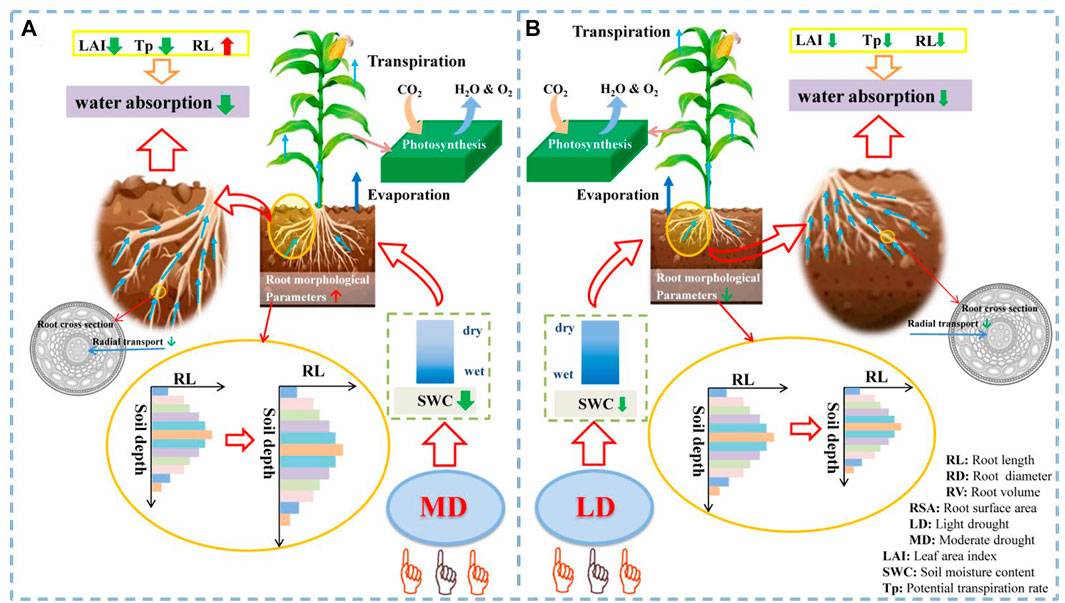
FIGURE 10. Explanation of adaptive mechanism process of summer maize under drought stress. (A) (B) is the process chart of root morphology change and root water absorption change under the MD and LD. The red up arrow indicates the positive impact, the green down arrow indicates the negative impact, and the width of the arrow indicates the strength.
5 Conclusion
This study mainly discussed adaptive changes in root morphology and root water absorption of summer maize under different drought stress: 1) Under drought stress, the root system of maize adapts to drought by increasing the proportion of fine roots to improve the water absorption. The MD during jointing-tasseling stage is beneficial to the growth of root system. The length of root system in 15–30 cm layer is increased by LD and MD. Root biomass was no significant difference between the drought treatment group and the CS. The root biomass of the drought treatment group was significantly lower than that of the COS due to the thinner root system. 2) The total amount of root water absorption in the CS and COS increased steadily with the increase of time. The drought treatment group was affected by soil moisture and the adaptive changes of root morphology, with the increase of the days without rainfall, the root water absorption capacity would become weak and the root water absorption would decrease: COS > CS > LD > MD. The results basically verified our hypothesis. In this study, only two drought treatment groups are set in the jointing and heading stage of 2021. Further research can add different drought treatment groups and carry out control tests on different growth stages. Thus, the adaptability of crop root system under different drought stress can be explored in a deeper level, which provides a theoretical basis for improving crop water use efficiency.
Data availability statement
The original contributions presented in the study are included in the article/Supplementary Material, further inquiries can be directed to the corresponding author.
Ethics statement
Written informed consent was obtained from the individual(s) for the publication of any potentially identifiable images or data included in this article.
Author contributions
SY and LJ collected basic data, SY performed the statistical analysis and wrote the first draft of the manuscript. BW provides the working concept. DY confirmed and guided the study. The manuscript was critically revised by BW, DY, and WB provided a revised version of the manuscript. All authors contributed to this article and approved this version for submission.
Funding
This work was supported the National Natural Science Foundation of China (No. 52022110), the IWHR Research & Development Support Program (No. MK0145B022021), and the Representative Achievements and Cultivation Project of State Key Laboratory of Simulation and Regulation of Water Cycle in River Basin (No. SKL2020TS02). The Open Fund of Key Laboratory of Flood & Drought Disaster Defense, the Ministry of Water Resources (No. KYFB202112071044).
Acknowledgments
Many thanks to Wudaogou Experimentation Research Station for their full support and help during this study.
Conflict of interest
The authors declare that the research was conducted in the absence of any commercial or financial relationships that could be construed as a potential conflict of interest.
Publisher’s note
All claims expressed in this article are solely those of the authors and do not necessarily represent those of their affiliated organizations, or those of the publisher, the editors and the reviewers. Any product that may be evaluated in this article, or claim that may be made by its manufacturer, is not guaranteed or endorsed by the publisher.
References
Ahmed, F., Arthur, E., Liu, H., and Andersen, M. H. (2020). New rootsnap sensor reveals the ameliorating effect of biochar on in situ root growth dynamics of maize in sandy soil. Front. Plant Sci. 11, 949. doi:10.3389/fpls.2020.00949
Allen, R., Pereira, L., Raes, D., Smith, M., Allen, R. G., and Pereira, L. S. (1998). Cropevapotranspiration Guidelines for computing crop water requirements-FAO irrigation and drainage paper 56. Food Agric. Organ. U. N. Rome 300 (9), 20–36.
Annicchiarico, P., Pecetti, L., and Tava, A. (2012). Physiological and morphological traits associated with adaptation of lucerne (Medicago sativa) to severely drought-stressed and to irrigated environments. Ann. Appl. Biol. 162 (1), 27–40. doi:10.1111/j.1744-7348.2012.00576.x
Bansal, K. C., and Nagarajan, S. (1987). Reduction of leaf growth by water stress and its recovery in relation to transpiration and stomatal conductance in some potato (Solanum tuberosum L.) genotypes. Potato Res. 30 (3), 497–506. doi:10.1007/bf02361929
Cairns, J. E., Impa, S. M., O’Toole, J. C., Jagadish, S. V. K., and Price, A. H. (2011). Influence of the soil physical environment on rice (Oryza sativa L.) response to drought stress and its implications for drought research. Field Crops Res. 121 (3), 303–310. doi:10.1016/j.fcr.2011.01.012
Campos, H., Cooper, M., Habben, J. E., Edmeades, G. O., and Schussler, J. R. (2004). Improving drought tolerance in maize: A view from industry. Field Crops Res. 90 (1), 19–34. doi:10.1016/j.fcr.2004.07.003
Chen, P. S., Ji, R. P., Xie, Y. B., Shi, K. Q., Yang, Y., Zhang, H., et al. (2018). Effects of drought stress on root growth of Northeast Spring Maize at different developmental stages. Agric. Res. arid areas 36 (01), 156–163. doi:10.7606/j.issn.1000-7601.2018.01.24
Corak, S. J., Blevins, D. G., and Pallardy, S. G. (1987). Water transfer in an alfalfa/maize association. Plant Physiol. 84 (3), 582–586. doi:10.1104/pp.84.3.582
Deng, F., Chen, G. S., Huang, C. C., Xiong, D. C., and Huang, J. X. (2015). Fine root diameter distribution, spatial variation and sampling quantity estimation of subtropical evergreen broad-leaved forest based on micro root canal method. J. Subtropical Resour. Environ. 10 (04), 24–30.
Ding, C. M., Wu, P. N., Yue, L., Li, Y. H., Guan, X. K., and Wang, T. C. (2020). Based on HYDRUS-1D simulation, the effect of Tillage Methods on the field moisture of summer maize was studied. J. irrigation drainage 39 (12), 24–31. doi:10.13522/j.cnki.ggps.2019431
Dong, T., Duan, B., Zhang, S., Helena, K., Niinemets, Ü., and Li, C. Y. (2016). Growth, biomass allocation and photosynthetic responses are related to intensity of root severance and soil moisture conditions in the plantation tree cunninghamia lanceolata. Tree Physiol. 36 (7), 807–817. doi:10.1093/treephys/tpw025
Duan, H. P., Bian, X. M., Xie, X. L., and Wang, K. R. (2003). Farmland water cycle: Advances in the study of water transport at the surface - atmosphere interface. China agrometeorol. 24 (01), 37–41. doi:10.3969/j.issn.1000-6362.2003.01.011
Egamberdiyeva, D. (2007). The effect of plant growth promoting bacteria on growth and nutrient uptake of maize in two different soils. Appl. Soil Ecol. 36 (2-3), 184–189. doi:10.1016/j.apsoil.2007.02.005
FAO (2022). FAOSTAT. Food and agriculture organisation of the united Nations. https://www.fao.org/statistics/databases/en/.
Feddes, R. A., Bresler, E., and Neuman, S. P. (1974). Field test of a modified numerical model for water uptake by root systems. Water Resour. Res. 10 (6), 1199–1206. doi:10.1029/WR010i006p01199
Florian, K., Florian, V., Li, X., Hinrich, B., Günter, N., Benjamin, N., et al. (2019). Estimating the importance of maize root hairs in low phosphorus conditions and under drought. Ann. Bot. 6, 961–968. doi:10.1093/aob/mcz011
Gan, Y. W., and Zha, X. L. B. (2020). The effect of drought stress on crop growth mechanism. Tibet Agric. Sci. Technol. 42 (02), 31–33.
Gao, Y. (2013). Study on water dynamics of red soil sloping farmland based on hydras model, doctoral dissertation. Hubei, China: Huazhong Agricultural University. doi:10.7666/d.Y2394210
Genuchten, V., and Th, M. (1980). A closed-form equation for predicting the hydraulic conductivity of unsaturated soils. Soil Sci. Soc. Am. J. 44 (5), 892–898. doi:10.2136/sssaj1980.03615995004400050002x
Hasanuzzaman, M., Nahar, K., and Hossain, M. A. (2019). Wheat production in changing environments responses, adaptation and tolerance: Responses, adaptation and tolerance: ResearchGate.
Jiang, Y., Wang, B. Q., Xie, B. T., Zhang, H. Y., Duan, W. W., and Wang, Q. M. (2016). Analysis of root antioxidant characteristics of sweet potato seedlings with different drought tolerance under drought conditions. Agric. Sci. Technol. 17 (02), 245–250+255. doi:10.16175/j.cnki.1009-4229.2016.02.002
Kadioglu, A., and Terzi, R. (2007). A dehydration avoidance mechanism: Leaf rolling. Botanical Rev. 73 (4), 290–302. doi:10.1663/0006-8101(2007)73[290:adamlr]2.0.co;2
Kang, Y. X., Lu, G. H., Wu, Z. Y., and He, H. (2009). Changes of reference crop evapotranspiration and its response to climate in the Huaihe River Basin. Hydropower energy Sci. 27 (06), 12–14+44. doi:10.3969/j.issn.1000-7709.2009.06.004
Kim, J., and Mike, E. (1995). A simulation of the surface energy budget and soil water content over the hydrologic atmospheric pilot experiments-modélisation du bilan hydrique forest site. J. Geophys. Res. 100 (D10), 20845. doi:10.1029/95JD01469
Lal, R. (1979). Plant root systems: Their function and interaction with the soil. Field Crops Res. 2, 177–179. doi:10.1016/0378-4290(79)90020-0
Li, Y., Li, Z., Cui, S., Chang, S. X., Jia, C., and Zhang, Q. (2019). A global synthesis of the effect of water and nitrogen input on maize (Zea mays) yield, water productivity and nitrogen use efficiency. Agric. For. Meteorology 268, 136–145. doi:10.1016/j.agrformet.2019.01.018
Lilley, J. M., and Fukai, S. (1994). Effect of timing and severity of water deficit on four diverse rice cultivars I. Rooting pattern and soil water extraction. Field Crops Res. 37 (3), 205–213. doi:10.1016/0378-4290(94)90099-X
Liu, F. H., and Stützel, H. (2002). Leaf expansion, stomatal conductance, and transpiration of vegetable amaranth (amaranthus sp.) in response to soil drying. J. Am. Soc. Hortic. Sci. 127 (5), 878–883. doi:10.21273/jashs.127.5.878
Liu, J. B. (2012). Corn cultivation in northern China. Beijing, China: China Agricultural Science and Technology Press.
Liu, X., Wang, P., and Zhou, Q. P. (2021). Advances in research on plant root apoplast barrier. Acta Bot. Sin. 66 (06), 761–773.
Lorenc, F., Peková, V., Modlinger, R., Mrnka, L., Turcani, M., Senfeld, P., et al. (2018). Fine roots of Picea abies compensate for drought stress in the rainfall reduction experiment. Dendrobiology 80, 91–100. doi:10.12657/denbio.080.009
Lu, Z. X. (2021). China's corn industry development, trade changes and future prospects in the past 60 years. Heilongjiang grain 09, 9–14. doi:10.3969/j.issn.1671-6019.2021.09.003
Magistad, O. C., and Breazeale, J. F. (1929). Plant and soil relations at and below the wilting percentage. Ariz. Agric. Exp. Sta Tech. Bull. P 25, 1–36.
Mahdid, M., Kameli, A., Ehlert, C., and Simonneau, T. (2011). Rapid changes in leaf elongation, ABA and water status during the recovery phase following application of water stress in two durum wheat varieties differing in drought tolerance. Plant Physiology Biochem. 49 (10), 1077–1083. doi:10.1016/j.plaphy.2011.08.002
Monika, B., Dana, H., Luká, F., Jedelsky, P. L., František, H., Naďa, W., et al. (2012). The physiology and proteomics of drought tolerance in maize: Early stomatal closure as a cause of lower tolerance to short-term dehydration? PLoS ONE 7 (6), e38017. doi:10.1371/journal.pone.0038017
Mthandi, J., Kahimba, F. C., Tarimo, A., Salim, B. A., and Lowole, M. W. (2013). Root zone soil moisture redistribution in maize (<i>Zea mays</i> L.) under different water application regimes. Agric. Sci. 4 (10), 521–528. doi:10.4236/as.2013.410070
Nezhadahmadi, A., Prodhan, Z. H., and Faruq, G. (2013). Drought tolerance in wheat. Sci. World J. 2013, 1–12. doi:10.1155/2013/610721
Ng, C. W. W., Tasnim, R., Capobianco, V., and Coo, J. L. (2018). Influence of soil nutrients on plant characteristics and soil hydrological responses. Geotech. Lett. 8 (1), 19–24. doi:10.1680/jgele.17.00104
Nie, Y. P., Chen, H. S., Wang, K. L., Tan, W., Deng, P. Y., and Yang, J. (2010). Seasonal water use patterns of woody species growing on the continuous dolostone outcrops and nearby thin soils in subtropical China. Plant Soil 341 (1-2), 399–412. doi:10.1007/s11104-010-0653-2
Oyanagi, A., Kiribuchi-Otobe, C., Yanagisawa, T., Miura, S., and Muranaka, S. (2004). Growth and grain yield of wheat experimental lines with deep and shallow root system in wet paddy fields. Jpn. J. Crop Sci. 73 (3), 300–308. doi:10.1626/jcs.73.300
Paz, H., Pineda-Garcia, F., and Pinzon-Perez, L. F. (2015). Root depth and morphology in response to soil drought: Comparing ecological groups along the secondary succession in a tropical dry forest. Oecologia 179 (2), 551–561. doi:10.1007/s00442-015-3359-6
Petek, M., Toth, N., Pecina, M., Karažija, T., Lazarević, B., Palčić, I., et al. (2013). Effects of different fertilization on root surface area of turfgrass. Glas. Zatite Bilj 6, 22–30.
Pike, C. C., Warren, J. C., and Montgomery, R. A. (2015). Allometry of early growth in selected and wild sources of white spruce, Picea glauca (Moench) Voss. New For. 47 (1), 131–141. doi:10.1007/s11056-015-9498-0
Prasad, R. (1988). A linear root water uptake model. J. Hydrology 99 (3-4), 297–306. doi:10.1016/0022-1694(88)90055-8
Prince, S., Anower, M. R., Motes, C. M., Hernandez, T. D., Liao, F., Putman, L., et al. (2022). Intraspecific variation for leaf physiological and root morphological adaptation to drought stress in alfalfa (medicago sativa L). Front. Plant Sci. 13, 795011. doi:10.3389/fpls.2022.795011
Qi, W. Z., Liu, H. H., Li, G., Shao, L. J., and Wang, F. F. (2012). Temporal and spatial distribution characteristics of super high yield summer maize roots. J. plant Nutr. fertilizer 18 (01), 69–76. doi:10.11674/zwyf.2012.11223
Qiao, S. (2018). Diversity of maize root traits and its response to drought stress. Shanxi, China: Northwest A & F University. https://kns.cnki.net/KCMS/detail/detail.aspx?dbname=CMFD201901&filename=1018973998.
Ren, B. Z., Zhang, J. W., Xia, L., Xia, F., Dong, S. T., Peng, L., et al. (2014). Effects of waterlogging on the yield and growth of summer maize under field conditions. Can. J. Plant Sci. 94 (1), 23–31.
Shan, C. J., and Liang, Z. S. (2007). Effects of soil drought on root growth and physiological characteristics of winter wheat seedlings. Chin. J. Ecol. Agric. 15 (05), 38–41.
Shemi, R., Wang, L., Zhang, K., Gheith, E., Hussain, S., Irfan, M., et al. (2021). Effects of salicylic acid, zinc and glycine betaine on morphophysiological growth and yield of maize under drought stress. Sci. Rep. 11 (1), 3195. doi:10.1038/s41598-021-82264-7
Smucker, A. J. M., and Aiken, R. M. (1992). Dynamic root responses to water deficits. Soil Sci. 154, 281–289. doi:10.1097/00010694-199210000-00004
Sposito, G. (2013). Green water and global food security. Vadose Zone J. 12 (4). doi:10.2136/vzj2013.02.0041
Stephanie, S., and Smet, I. D. (2012). Root growth and branching | introduction: Root system architecture: Insights from arabidopsis and cereal crops. Philos. Trans. Biol. Sci. 367 (1595), 1441–1452. doi:10.2307/23250439
Tran, T. T., Kano-Nakata, M., Suralta, R. R., Menge, D., Yamauchi, A., Inukai, Y., et al. (2014). Root plasticity and its functional roles were triggered by water deficit but not by the resulting changes in the forms of soil N in rice. Plant Soil 386 (1-2), 65–76. doi:10.1007/s11104-014-2240-4
Walne, C. H., and Reddy, K. R. (2022). Temperature effects on the shoot and root growth, development, and biomass accumulation of corn (Zea mays L). Agriculture 12 (4), 443. doi:10.3390/agriculture12040443
Wang, F., Wang, M. J., Su, S. H., Wang, Y. Y., Su, Y. H., Meng, G. D., et al. (2018). Effects of straw returning on maize yield and root system spatial distribution under water stress. J. Appl. Ecol. 29, 3643–3648. doi:10.13287/j.1001-9332.201811.023
Wang, S. F., Zhang, X. Y., and Pei, D. (2006). Effects of different water supply conditions on root distribution, yield and water use efficiency of winter wheat. J. Agric. Eng. 22 (02), 27–32. doi:10.3321/j.issn:1002-6819.2006.02.007
Wang, Z. F., Zhang, S. Q., and Liu, X. F. (2005). Analysis on the physiological and ecological reasons for the difference of water conductivity of maize root system. Acta Botanica Sinica 25 (08), 1607–1611.
Wang, Z. L., Zhang, Q. B., and Li, R. (2011). Experimental study on hydrology in huaibei plain. Beijing, China: China University of science and Technology Press.
Wu, Y. Z., and Huang, M. B. (2011). Based on HYDRUS-1D model, the influencing factors of maize root water absorption were analyzed. J. Agric. Eng. 27 (S2), 66–73. doi:10.3969/j.issn.1002-6819.2011.z2.014
Yan, Z. D., Zhu, C. W., and Sheng, J. L. (1964). Studies on drought tolerance and identification methods of sweet potato varieties. J. crops 3 (2), 183–189.
Zhang, Z. Y., Guo, W., and Yang, S. J. (2022). Research progress of plant root pressure. Guangxi plants 42 (04), 714–727.
Keywords: drought, rehydration after drought, root length, diameter class, root water absorption
Citation: Yan S, Weng B, Jing L, Bi W and Yan D (2022) Adaptive pathway of summer maize under drought stress: Transformation of root morphology and water absorption law. Front. Earth Sci. 10:1020553. doi: 10.3389/feart.2022.1020553
Received: 16 August 2022; Accepted: 08 September 2022;
Published: 26 September 2022.
Edited by:
Jun Niu, China Agricultural University, ChinaCopyright © 2022 Yan, Weng, Jing, Bi and Yan. This is an open-access article distributed under the terms of the Creative Commons Attribution License (CC BY). The use, distribution or reproduction in other forums is permitted, provided the original author(s) and the copyright owner(s) are credited and that the original publication in this journal is cited, in accordance with accepted academic practice. No use, distribution or reproduction is permitted which does not comply with these terms.
*Correspondence: Baisha Weng, YmFpc2hhd2VuZ0AxNjMuY29t