- 1State Key Laboratory of Tibetan Plateau Earth System Science, Resources and Environment (TPESRE), Institute of Tibetan Plateau Research, Chinese Academy of Sciences, Beijing, China
- 2Ministry of Education Key Laboratory of Western China’s Environmental Systems, College of Earth and Environmental Sciences, Lanzhou University, Lanzhou, Gansu, China
- 3Key Laboratory of Desert and Desertification, Northwest Institute of Eco-Environment and Resources, Chinese Academy of Sciences, Lanzhou, China
- 4Key Laboratory of Marine Geology and Environment, Institute of Oceanology, Chinese Academy of Sciences, Qingdao, China
- 5School of Geographical and Earth Sciences, University of Glasgow, Glasgow, United Kingdom
Editorial on the Research Topic
Tibetan Plateau uplift and environmental impacts: New progress and perspectives
Introduction
The Tibetan Plateau (TP) is the highest plateau on Earth, with an average elevation exceeding 4,500 m and covering over 2.5 × 106 km2. The plateau is therefore not only an iconic and unique region given its broad elevation and extent but whose creation is now considered one of the most significant geological events over the Cenozoic. Thus, the TP embodies an important natural laboratory for investigating the processes that helped to create it—continental convergence and global/regional climate—but for also testing and validating major hypotheses regarding how these forces interact to shape terrestrial environments in which we live (e.g., Molnar and England, 1990; Raymo and Ruddiman, 1992). Impacts of the TP are wide-ranging; it has played a vital role in global atmospheric circulation, from the inland Asian aridification, to the development of the Asian monsoon (Molnar et al., 2010; Wu et al., 2015), and in the evolution of Asian vegetation and fauna (Deng et al., 2019; Spicer et al., 2020).
The profound impact of TP uplift has inspired scholars worldwide to investigate its many facets over the past several decades. In recent years, the large-scale comprehensive scientific investigation of the TP, equipped with 21st century technologies and novel methods and led by multidisciplinary research groups, has brought exciting new perspectives. This Research Topic for Frontiers in Earth Science curating 27 papers (Figure 1) aims to provide an overview of recent findings that have aided progress in our understanding of the history of TP uplift, including the driving mechanisms, how such processes drove regional to global impacts, and how recent challenges have shifted our existing knowledge and established paradigms. It is the third year of the Second Tibetan Plateau Scientific Expedition, so we put together this volume as a partial summary for what has been achieved on this topic. We divide the 27 papers into the time intervals of their exploration and give a summary of their main findings, followed by our perspectives on future challenges in TP research.
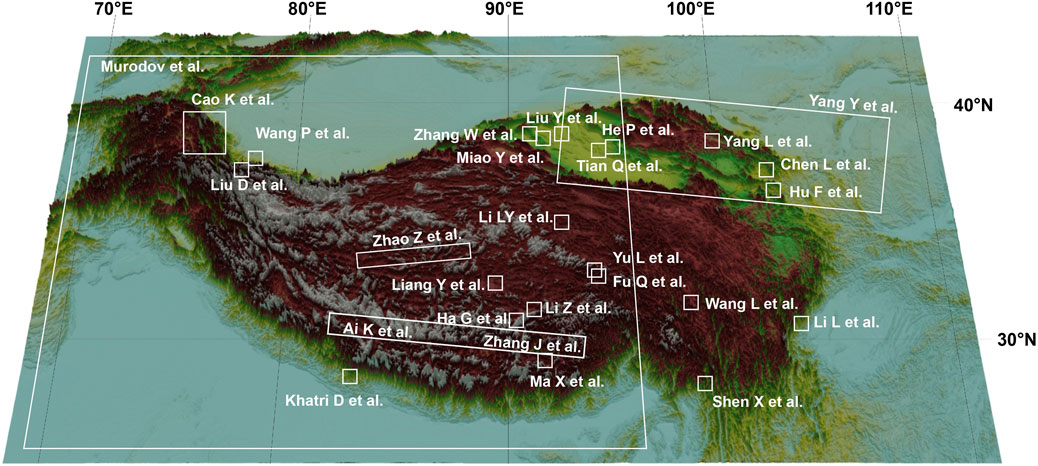
FIGURE 1. Locations of publications of this Research Topic on the Tibetan Plateau and surrounding regions.
Pre-Cenozoic
The pre-Cenozoic section contains four articles that study the processes preceding the India-Asia collision, providing an important and requisite geological framework for assessing later processes and impacts during and after India-Asia collision. Constraining the altitude of the Gangdese Mountains before India-Asia collision remains crucial in our understanding of the distribution of Asian environments. Unlike direct paleoelevation reconstructions, Zhang J et al. in this issue investigate the Late Cretaceous altitude of the Gangdese Mountains using climate models and test the coherence between assigned Gangdese altitudes and the observed extent of Asian aridity. They found that a height of ∼2 km for the Late Cretaceous Gangdese Mountains to be the most likely scenario. Paleomagnetic and rock magnetic methods have been applied to a wide variety of important geological questions regarding the evolution of the Tethys and India-Asia collision, but it remains crucial to determine the extent to which primary magnetic signals are faithfully preserved in rocks. Yu L et al. assess this through their comprehensive petrographic observations and rock magnetic and paleomagnetic analyses from early Carboniferous limestones within the East Qiangtang Terrane of central Tibet. Their results show that limestones were re-magnetized by hydrothermal activities after 53 Ma, due to far-field effects of the India–Eurasia collision. Fu Q. et al. further investigate paleomagnetism of an Early Cretaceous granite intruding into Jurassic sandstones within the East Qiangtang Terrane. Their findings constrain an earlier Cretaceous deformation event before granitic intrusion, thus suggesting that collision between the Lhasa and East Qiangtang terranes occurred before ∼126 Ma. Li Z et al. show new paleomagnetic results yielded from volcanic rocks in the northern Lhasa terrane. Their data indicate a paleolatitude of ∼13°N for the Lhasa Terrane at 115 ± 5 Ma, thus adding important constraints on the magnitude of crustal shortening between India and Asia, and on the reconstructed extent of the Neo-Tethys Ocean.
Paleogene-Neogene
The Paleogene-Neogene section contains 17 articles dealing with tectonics, erosion, weathering, and environment across sectors of the TP. India-Asia collision and TP uplift induced tectonic deformation that caused dramatic changes to dominant geomorphological regimes, drainage organization, and denudation patterns across the entire TP. In the western TP, Wang P. et al. present detrital zircon U-Pb and apatite fission-track ages from the southwest Tarim Basin that highlight rapid Eocene (45 Ma) denudation across the Central Pamir induced by abrupt uplift and paleo-geomorphological change. At the same time, Liu D et al. present an age-elevation profile across granites from the southern West Kunlun Mountains. Their apatite fission track ages document rapid late Oligocene (∼26 Ma) exhumation due to Cenozoic northward growth of the West Kunlun Mountains. In the northeastern TP, Chen L et al. reveal large-scale denudation from ranges surrounding the Xining Basin using detrital apatite fission track data from two late Neogene sections; they illustrate rapid denudation in this region since the Late Cretaceous and emphasize sediment recycling in the late Miocene (∼8 Ma) driven by deformation. He P et al. present new apatite fission track data from Paleogene strata in the northern Qaidam Basin that document an intensified period of thrusting and folding along with the Qilian Shan since the late Miocene (∼10 Ma). Tian Q et al. present a record of glycerol dialkyl glycerol tetraethers (GDGTs) from the same section as He P et al. in the Qaidam Basin. They show mean annual paleotemperatures of the basin were relatively higher (∼28°C) in the early Miocene (∼18 Ma) that suggests the mean paleoelevation of the basin was also much lower (∼1.5 km asl) at that time. Their results are largely in agreement with remarkable shortening recorded in the basin since the mid-late Miocene. Zhang W. et al. present a record of the anisotropy of magnetic susceptibility from the western Qaidam Basin. Their results surprisingly suggest tectonic events decreased in magnitude since the late Miocene (∼6.9 Ma), possibly because most geological units in the western Qaidam Basin released the majority of compressional strain during the mid-late Miocene. In the central TP, Zhao Z et al. combine the new apatite fission track data from the Qiangtang Terrane with isostatic modeling. They propose that the Qiangtang Terrane has experienced a two-stage uplift: an early uplift before the middle Eocene (∼40 Ma) caused by crustal thickening and a later Oligocene uplift caused by lithospheric mantle delamination. Li LY et al. present another record of the anisotropy of magnetic susceptibility from the late Eocene to early Miocene Tuotuohe foreland basin. They document active tectonic strain from the Tanggulashan uplift before ∼30 Ma and after 19.5 Ma. In the southern TP, Ai K et al. explore the sedimentology and chronology of the Gangdese Range. They infer that a westward-flowing axial palaeo-Yarlung-Zangbo River occupied the length of the Yarlung-Zangbo suture zone from the late Oligocene to early Miocene: the opposite of the current configuration of the Yarlung-Zangbo River. Ma X et al. investigate mesoscopic and microscopic magmatic structures of the early Eocene Quxu batholith, formed in the transitional regime between subduction of the Neotethyan oceanic slab beneath the Lhasa terrane and the continent-continent Indo-Asian collision. Results from the batholith suggest that magma mixing between a mantle-derived mafic endmember and felsic crustal material played a pivotal role in generation of the calc-alkaline pluton and its enclosed magmatic microgranular enclaves.
India-Asia collision and resultant TP uplift instigated significant and diverse environmental changes across the entire Asian region. In the northern TP, Yang Y. et al. provide the first complete, long (53 Myr) continental silicate chemical weathering record based on clay mineral data. Their weathering record documents Paleogene weakening followed by long-term Neogene strengthening, with three significant transitions at ∼26–23 Ma, ∼16 Ma, and ∼8 Ma. Their results suggest that global climate modulated the Paleogene variations in silicate chemical weathering, while regional monsoon rainfall controlled Neogene variability, including the three critical transitions. Miao Y et al. uses microcharcoal data in the Qaidam Basin to reconstruct a first record of microcharcoal-based wildfire in the northern TP (Inner Asia) spanning the Oligocene-Miocene. Their results show that wildfires before ∼26 Ma remained relatively frequent, then reduced gradually, coinciding with global temperature changes. Liu Y et al. present a high-resolution continuous carbonate manganese record reconstructed from Qaidam Basin lake sediments from 7.3 Ma. They argue their manganese record is a sensitive proxy of climate change that shows long-term variability consistent with global climate, especially with high latitudes. Hu F et al. present carbon and oxygen isotopic compositions from ∼9 Myr Hipparion fauna tooth enamel from the Xunhua Basin. Enamel carbon isotope data indicate that Hipparion fauna fed mostly on C3 plants. Their reconstructed paleo-meteoric oxygen isotopes suggest a wetter climate or stronger monsoonal influence than today. In the eastern TP, Wang L et al. use sporomorphs assemblages, sedimentology, and evaporite geochemistry to reconstruct the paleoclimate and paleovegetation for the middle Eocene. They infer that warm and humid deciduous broad-leaved forests transitioned to cool and arid temperate forests by ∼44 Ma. In the central TP, Liang Y. et al. used multiple records of n-alkane biomarker indices to trace mid-Miocene lake levels fluctuations in the Lunpola Basin. They argue that global climatic changes played a dominant role in dictating lake fluctuations whereas tectonic uplift only played a subordinate role. In the south TP, Khatri et al. explore how active tectonics dictated rock magnetic properties of sediments from Late Cretaceous-Eocene Lesser Himalayan strata from western Nepal.
Quaternary
The Quaternary section contains six papers dealing with geophysical observations, erosion, and weathering processes across the TP. In the northern TP, Murodov et al. review and analyze receiver function data beneath the Pamir–Tibetan Plateau to observe variations in depth of the Moho and crustal Vp/Vs ratios. They find that lower crustal channel flow might contribute to the crustal thickening in the central plateau but that crustal thickening and shortening provide the main mechanism of uplift for other areas of the Pamir–Tibetan Plateau. Cao K et al. apply multiple geomorphic parameters to study how normal faulting and surface erosion influence Quaternary exhumation across the Pamir. They evaluate the relative contributions from tectonics, glacial/fluvial erosion, and lithology in driving focused exhumation of domes in the region. Yang L et al. present ion geochemistry from weekly water sampling of the upper Yellow River to quantify chemical weathering inputs. They propose that runoff, rather than erosion, plays a central role in chemical weathering within the Yellow River Basin. In the eastern TP, Li L et al. compile dissolved radiogenic uranium isotopes in river water samples across catchments with variable landsliding in the Minjiang River Basin. Based on their results, they propose that the riverine dissolved 234U/238U ratio is a sensitive tracer of weathering fluxes driven by landslides. Shen X et al. report apatite (U-Th)/He data from an age-elevation transect along the Dulong batholith of the central Three Rivers Region and suggest that uplift due to high-strain around the eastern syntaxis of India-Eurasia convergence is responsible for enhanced Quaternary tectonism in the region. In the southern TP, Ha G et al. apply 14C dating to peat layers and snail shells to constrain the emplacement age of a rock avalanche. Their results question a sole seismic trigger for the avalanche because the accuracy of the commonly used age determination cannot rule out other possibilities.
Perspective and remaining challenges
This Research Topic presents studies across a diverse spectrum of geoscience. However, there are still several long-standing issues in the Tibetan Plateau that warrant further research and we summarize a few below:
Applying novel methods to acquire environmental boundary conditions in the rock record
One of the ongoing primary challenges in the geoscience community is the quantitative reconstruction of environmental parameters from the rock record, such as elevation, temperature, precipitation, and seasonality. In part, improvements in our ability to measure boundary conditions utilizing novel (bio)geochemical markers, such as clumped isotopes, have shed light on paleoevelation reconstructions in recent years. However, rigorous debate still remains concerning paleoelevation records for the TP, especially in context to findings from traditional isotopic paleoaltimetry (Quade et al., 2011, 2020). In particular, the northern TP region displays a hydrologically complex setting with multiple meteoric water sources through the Cenozoic; this hinders the application of traditional paleoaltimetry studies based on oxygen and hydrogen isotopes of meteoric waters. Paleoaltimetry estimates independent of meteoric sources may be valid prospects for future study. For example, recent investigations in the northern TP show that temperature- and paleovegetation-based paleoelevations reconstructions could have a great potential, e.g., GDGTs from Tian Q et al. in this Research Topic and carbonate clumped-isotopes in lacustrine authigenic carbonates (ostracod) (Li et al., 2021; Song et al., 2022), as well as Climate-Leaf Analysis Multivariate Program (CLAMP) and moist enthalpy method based on the shape of fossil leaves (Song et al., 2020).
Linking surface and deep processes to define triggers, thresholds, and tipping points.
Over the last few decades, considerable time and effort have gone into determining the relative roles of earth surface processes, tectonics, and mantle dynamics in driving large events in Earth evolution. In terms of the wider TP and Himalaya research community, many agree now that this is a somewhat paradoxical approach given the tight links amongst all these processes over geological time (i.e., Molnar and England, 1990). Often, deeper processes can inform on earth surface evolution, and vice versa. For example, work by Hu et al. (2020) suggested a direct correlation exists between crustal thickness and elevation in both subduction-related and collision-related mountain belts due to isostatic equilibrium. Through the application of Sr/Y and (La/Yb)N ratios from magmatic rocks, Hu et al. (2020). reconstructed Tibetan crustal thickness and paleoelevation since the Cretaceous. Similarly, Zhao Z et al. (in this Research Topic) utilised isostatic models to constrain uplift histories for the Qiangtang Terrane, central Tibet. Further, a comprehensive assessment of the carbon budget for the TP requires a full understanding of the atmospheric carbon dioxide uptake by weathering processes and organic carbon on earth’s surface (e.g., Raymo and Ruddiman, 1992; France-Lanord and Derry, 1997), and the release of carbon dioxide from the deep portion beneath the TP (e.g., Becker et al., 2008; Guo et al., 2021). The next—perhaps current—chapter in this overarching challenge involves better characterization of surface-tectonic-mantle linkages, especially in terms of their magnitude and directionality (positive or negative feedbacks). Identifying unique tipping points in TP paleoenvironments due to tectonic or atmospheric triggers may be especially key for mitigating future climate projections.
Bridging gaps between modern processes and reconstructions.
Building from our two previously posed challenges, this final issue encompasses the pervasive problem of scale (extent, magnitude, and rates) in the rock record. There still exist considerable gaps in our knowledge for how modern processes can be appropriately applied to paleoenvironments in the past. This, unfortunately, often leads to the simple fact that many observed relationships cannot be appropriately applied in our paleo-reconstructions. One of the best examples in current TP research is the issue of atmospheric CO2, global temperatures, and chemical weathering. For example, TP uplift has been argued to drive Cenozoic global cooling through the consumption of atmospheric CO2 via the chemical weathering of silicates (e.g., Raymo and Ruddiman, 1992). There are emerging studies on modern weathering dynamics and the associated atmospheric carbon dioxide consumption (e.g., Huh, 2010; Isson et al., 2020; Hilton and West, 2020) as well as past weathering dynamics under differing environmental conditions (e.g., Caves Rugenstein et al., 2019; Clift et al., 2014; Yang et al., 2021). However, combining modern observations with paleoreconstructions from the geological record to yield rates of chemical weathering, let alone chemical weathering fluxes, remains challenging (Clift and Jonell, 2021; Yang et al., 2021). Recent studies from the TP especially highlight that more studies with robustly-resolved erosional records and chemical weathering fluxes across the Himalayan-TP and wider Asia-Oceania are required.
Author contributions
All authors listed have made a substantial, direct, and intellectual contribution to the work and approved it for publication.
Funding
This work was co-supported by the Second Tibetan Plateau Scientific Expedition and Research (STEP) program (Grant No. 2019QZKK0707).
Acknowledgments
We deeply thank all the authors and reviewers who have participated in this Research Topic, and the Frontiers in Earth Science editorial office for assistance in production.
Conflict of interest
The authors declare that the research was conducted in the absence of any commercial or financial relationships that could be construed as a potential conflict of interest.
Publisher’s note
All claims expressed in this article are solely those of the authors and do not necessarily represent those of their affiliated organizations, or those of the publisher, the editors and the reviewers. Any product that may be evaluated in this article, or claim that may be made by its manufacturer, is not guaranteed or endorsed by the publisher.
References
Becker, J. A., Bickle, M. J., Galy, A., and Holland, T. J. (2008). Himalayan metamorphic CO2 fluxes: Quantitative constraints from hydrothermal springs. Earth Planet. Sci. Lett. 265 (3-4), 616–629. doi:10.1016/j.epsl.2007.10.046
Caves Rugenstein, J. K., Ibarra, D., and von Blanckenburg, F. (2019). Neogene cooling driven by land surface reactivity rather than increased weathering fluxes. Nature 571 (7763), 99–102. doi:10.1038/s41586-019-1332-y
Clift, P. D., and Jonell, T. N. (2021). Himalayan‐Tibetan erosion is not the cause of Neogene Global Cooling. Geophys. Res. Lett. 48 (8), e2020GL087742. doi:10.1029/2020GL087742
Clift, P. D., Wan, S., and Blusztajn, J. (2014). Reconstructing chemical weathering, physical erosion and monsoon intensity since 25 Ma in the northern south China sea: A review of competing proxies. Earth. Sci. Rev. 130, 86–102. doi:10.1016/j.earscirev.2014.01.002
Deng, T., Wang, X., Wu, F., Wang, Y., Li, Q., Wang, S., et al. (2019). Review: Implications of vertebrate fossils for paleo-elevations of the Tibetan Plateau. Glob. Planet. Change 174, 58–69. doi:10.1016/j.gloplacha.2019.01.005
France-Lanord, C., and Derry, L. A. (1997). Organic carbon burial forcing of the carbon cycle from Himalayan erosion. Nature 390 (6655), 65–67. doi:10.1038/36324
Guo, Z., Wilson, M., Dingwell, D. B., and Liu, J. (2021). India-Asia collision as a driver of atmospheric CO2 in the Cenozoic. Nat. Commun. 12 (1), 3891. doi:10.1038/s41467-021-23772-y
Hilton, R. G., and West, A. J. (2020). Mountains, erosion and the carbon cycle. Nat. Rev. Earth Env. 1 (6), 284–299. doi:10.1038/s43017-020-0058-6
Hu, F., Wu, F., Chapman, J. B., Ducea, M. N., Ji, W., and Liu, S. (2020). Quantitatively tracking the elevation of the Tibetan Plateau since the cretaceous: Insights from whole‐rock Sr/Y and La/Yb ratios. Geophys. Res. Lett. 47 (15), e2020GL089202. doi:10.1029/2020GL089202
Huh, Y. (2010). Estimation of atmospheric CO2 uptake by silicate weathering in the himalayas and the Tibetan plateau: A review of existing fluvial geochemical data. Geol. Soc. Lond. Spec. Publ. 342 (1), 129–151. doi:10.1144/sp342.10
Isson, T. T., Planavsky, N. J., Coogan, L. A., Stewart, E. M., Ague, J. J., Bolton, E. W., et al. (2020). Evolution of the global carbon cycle and climate regulation on Earth. Glob. Biogeochem. Cycles 34, e2018GB006061. doi:10.1029/2018GB006061
Li, H., Liu, X., Arnold, A., Elliott, B., Flores, R., Kelley, A. M., et al. (2021). Mass 47 clumped isotope signatures in modern lacustrine authigenic carbonates in Western China and other regions and implications for paleotemperature and paleoelevation reconstructions. Earth Planet. Sci. Lett. 562, 116840. doi:10.1016/j.epsl.2021.116840
Molnar, P., Boos, W. R., and Battisti, D. S. (2010). Orographic controls on climate and paleoclimate of Asia: Thermal and mechanical roles for the Tibetan Plateau. Annu. Rev. Earth Planet. Sci. 38 (1), 77–102. doi:10.1146/annurev-earth-040809-152456
Molnar, P., and England, P. (1990). Late cenozoic uplift of mountain ranges and global climate change: Chicken or egg? Nature 346 (6279), 29–34. doi:10.1038/346029a0
Quade, J., Breecker, D. O., Daëron, M., and Eiler, J. (2011). The paleoaltimetry of Tibet: An isotopic perspective. Am. J. Sci. 311 (2), 77–115. doi:10.2475/02.2011.01
Quade, J., Leary, R., Dettinger, M. P., Orme, D., Krupa, A., DeCelles, P. G., et al. (2020). Resetting Southern Tibet: The serious challenge of obtaining primary records of Paleoaltimetry. Glob. Planet. Change 191, 103194. doi:10.1016/j.gloplacha.2020.103194
Raymo, M. E., and Ruddiman, W. F. (1992). Tectonic forcing of late Cenozoic climate. Nature 359 (6391), 117–122. doi:10.1038/359117a0
Song, B., Spicer, R. A., Zhang, K., Ji, J., Farnsworth, A., Hughes, A. C., et al. (2020). Qaidam Basin leaf fossils show northeastern Tibet was high, wet and cool in the early Oligocene. Earth Planet. Sci. Lett. 537, 116175. doi:10.1016/j.epsl.2020.116175
Song, B., Zhang, K., Farnsworth, A., Ji, J., Algeo, T. J., Li, X., et al. (2022). Application of ostracod-based carbonate clumped-isotope thermometry to paleo-elevation reconstruction in a hydrologically complex setting: A case study from the northern Tibetan plateau. Gondwana Res. 107, 73–83. doi:10.1016/j.gr.2022.02.014
Spicer, R. A., Farnsworth, A., and Su, T. (2020). Cenozoic topography, monsoons and biodiversity conservation within the Tibetan Region: An evolving story. Plant Divers. 42 (4), 229–254. doi:10.1016/j.pld.2020.06.011
Wu, G., Duan, A., Liu, Y., Mao, J., Ren, R., Bao, Q., et al. (2015). Tibetan plateau climate dynamics: Recent research progress and outlook. Natl. Sci. Rev. 2 (1), 100–116. doi:10.1093/nsr/nwu045
Keywords: Asian monsoon, Asian inland drying, global change, paleoaltimetry, chemcial weathering, carbon cycle and budget, paleoecology, provenance
Citation: Yang Y, Nie J, Miao Y, Wan S and Jonell TN (2022) Editorial: Tibetan Plateau uplift and environmental impacts: New progress and perspectives. Front. Earth Sci. 10:1020354. doi: 10.3389/feart.2022.1020354
Received: 16 August 2022; Accepted: 16 August 2022;
Published: 09 September 2022.
Edited and reviewed by:
Derek Keir, University of Southampton, United KingdomCopyright © 2022 Yang, Nie, Miao, Wan and Jonell. This is an open-access article distributed under the terms of the Creative Commons Attribution License (CC BY). The use, distribution or reproduction in other forums is permitted, provided the original author(s) and the copyright owner(s) are credited and that the original publication in this journal is cited, in accordance with accepted academic practice. No use, distribution or reproduction is permitted which does not comply with these terms.
*Correspondence: Yibo Yang, eWFuZ3lpYm9AaXRwY2FzLmFjLmNu