- 1School of Geoscience and Technology, Southwest Petroleum University, Chengdu, China
- 2Natural Gas Geology Key Laboratory of Sichuan Province, Southwest Petroleum University, Chengdu, China
- 3Design and Planning Institute of SINOPEC Northwest Company, Urumqi, China
The deeply buried carbonate reservoir of Cambrian is an important target of oil and gas exploration in the Tarim Basin. Understanding the dissolution mechanism of the deep-buried carbonate reservoir is an urgent problem to be solved. In this study, 11 carbonate samples from three types of deep reservoirs in Tarim Basin were selected as experimental objects, and the dissolution process of carbonate reservoir was simulated by using an advanced reaction system of continuous flow at high temperature and high pressure. The dissolution test of continuous flow shows that the burial depth has an effect on carbonate dissolution, with the increasing of the depths from shallow to deep, the dissolution ability of acidic fluids in carbonate rocks increases first and then decreases. A comparison of dissolution results from different lithologic samples shows that the incomplete dolomitization calcite in the diagenetic stage provides the material basis for dissolution in the later stage. The relationship between reservoir type and physical property is discussed, and it is found that the permeability of the fracture-type and pore-fracture samples increase significantly after dissolution, indicating the pre-existing pores of carbonate rocks may be critical to the formation of high-quality reservoirs in deeply buried conditions. An interesting phenomenon was found by comparing the dissolution rates of experimental samples shows that the dissolution rate of dolomite reservoir has a trend of first increasing and then decreasing under the deeply buried environment, which proves that there is an optimal dissolution range of dolomite in the deeply buried environment, which is conducive to the formation of dolomite reservoir. The genetic model of burial dissolution in deep carbonate reservoirs was established and indicated that the search for dolostone reservoirs near the Himalayan fault may be a new idea for Cambrian deep oil and gas exploration.
1 Introduction
In recent years, global oil and gas exploration has made great breakthroughs in deep carbonate formation indicating deep dolostone is rich in oil and gas resources (Howarth and Alves, 2016; Zhu et al., 2019; Li and Goldberg, 2022; Ma et al., 2022). The abundant oil and gas displays have been found in the Cambrian super-deep dolomite reservoirs in the Tarim Basin of China (Huang et al., 2014; Valenciaa et al., 2020; Li and Goldberg, 2022). The high-porosity reservoirs of the TS1 well have been found at the depth of 8,848 m, which confirmed the good exploration prospects for ultra-deep reservoirs (Xiong et al., 2015; Zhu et al., 2018; Cheng et al., 2019; Jiao, 2019). Therefore, the cause of dissolution pores in deep carbonate reservoirs has become a hot study topic in petroleum geology. Many scholars believe that the dissolution of the quasi-synchronous period and supergene karst are of great significance to the carbonate reservoir (Yasuda et al., 2013; Luhmann et al., 2014; Li et al., 2019; Li et al., 2020; Tian et al., 2019). The main reason for the acceptance of this mechanism is that dissolution is easy to occur due to a large amount of fresh water on the surface (Cai et al., 2014). In contrast, some scholars believe that fluid activities in the deep burial stage have a great influence on the dissolution of carbonate reservoirs (Zhu et al., 2018; Jiao, 2019; Luo et al., 2022). But the source of acidic fluid dissolved in deep reservoirs is controversial. Some scholars believe that deep organic acids have a greater influence (Luhmann et al., 2014; Hao et al., 2015; Biehl et al., 2016; Valenciaa et al., 2020). Besides, the dissolution ability of dolomite is unclear (Hood et al., 2004; Tavakoli et al., 2019). Some scholars believe that the dolomite is not easy to dissolve and the reservoir space does not develop under the deep burial environment (Tutolo et al., 2015). Other scholars believe that dolomite is easily dissolved by deep fluids under the influence of faults at high temperatures and high pressure to form a high-quality reservoir (Howarth and Alves, 2016; Abarca et al., 2019; Lima et al., 2020; Valenciaa et al., 2020). From the perspective of petrology, thermodynamics, and dynamics, various simulated dissolution experiments show that the dissolution of dolomite is higher under burial conditions (Montes-Hernandez et al., 2016; Liu et al., 2018).
Given the above problems, this study designed the simulation experiment of high temperature and high-pressure dissolution dynamics. The theoretical mechanism for the deep-dissolved pore in the deep carbonate reservoirs was studied in this paper based on the dynamic properties of the reaction, the effect of deep dissolution method, and the microscopic observation and regional structural movement. It provides the reference for the formation mechanism research of deep dolomite reservoirs.
2 Experimental method
2.1 Experimental sample
To study the effect of dissolution transformation on different reservoir types and pore structures in a deep burial environment, the lithology of samples in the typical reservoirs of the Cambrian in the Tarim Basin is studied. Several wells, such as TS1, DG1, XH2, and YQ6 in the north, BT5 and MB1 in the northwest Tarim Basin (Figure 1). The mineral content and physical properties of each sample are shown in Table 1, and the microscopic characteristics are shown in Figure 2. The reservoir rock types are limestone and dolomite. The reservoir spaces are pore-type, pore-fracture type, and fracture type.
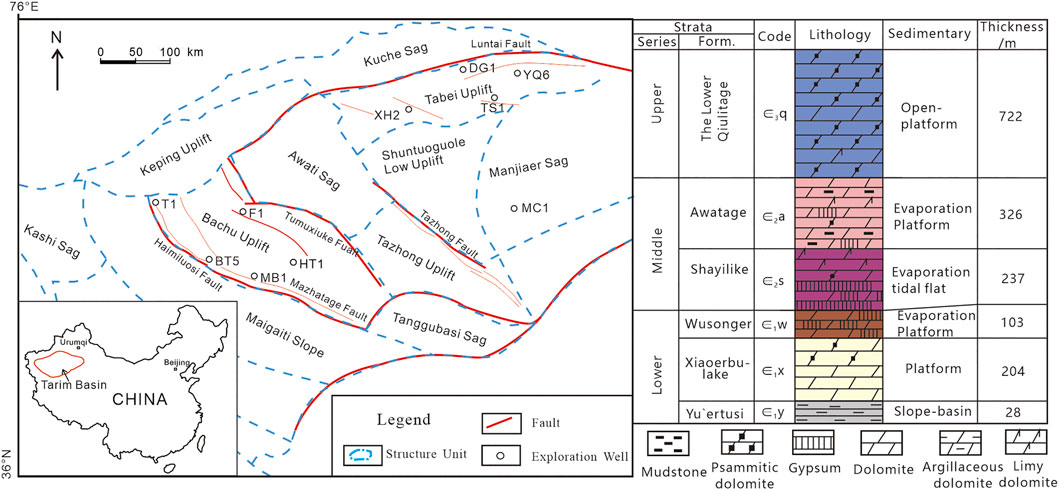
FIGURE 1. The distribution of Wells and Stratigraphic column of the Cambrian in the Tarim Basin (modified from Shen et al., 2016).
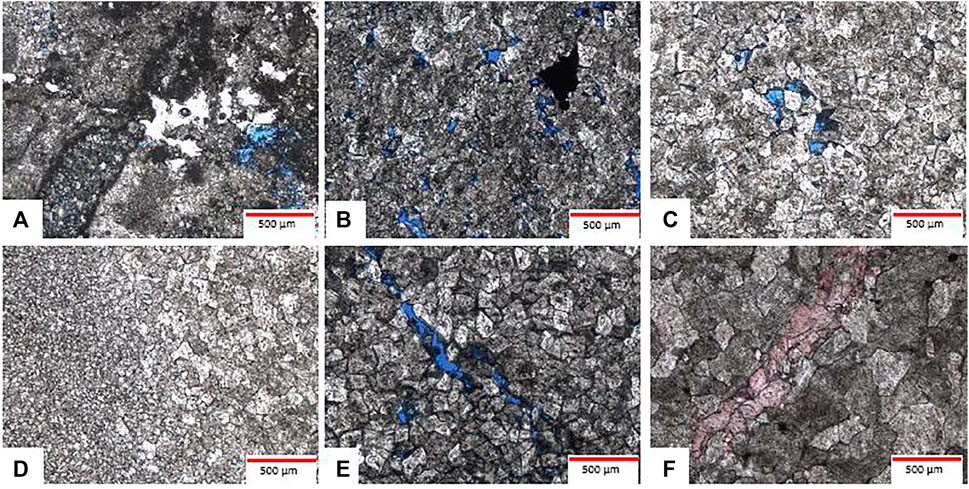
FIGURE 2. Microscopic petrological characteristics of experimental samples.Silty-crystalline dolomitic limestone, MB1,6007.32 m, ∈2, thin sections, plane polarized light, ×50 (B) residual fine-crystalline dolarenite, BT5, 5,785.95 m, ∈; (C) silty-crystalline dolomite, DG1, 6,260.1 m,∈, thin sections, plane polarized light,×50 (D) silty-crystalline dolomite, DG1,6260.1 m, ∈, thin sections, plane polarized light, ×50; (E) fine-grain dolomite, XH2,5353.93 m,∈3, thin sections, plane polarized light, ×50; (F) medium-crystalline dolomite, YQ6, 7,116.9, ∈3, dying cast thin sections, plane polarized light, ×50.
2.2 Experimental method
The pore structure characteristics of the experimental samples were observed by microscopic thin sections of the reservoir, and the identification results of reservoir space types were obtained by counting 300 points for each thin section. The dissolution experiments were carried out by the continuous flow diagenetic simulation system at the Key Laboratory of Carbonate Reservoirs of the Hangzhou Institute of Geology, as shown in Figure 3, PetroChina. The experimental equipment is a high-temperature and high-pressure dynamics simulation device independently designed by Hangzhou Institute. The laboratory is controlled at an initial temperature of 25°C and a humidity of 38%.
Since the deep carbonate rocks mainly experience the dissolution of fresh water in the supergenesis stage and the dissolution of organic acids and carbonic acid in the deep buried environment, the experiment was carried out in a continuous flow reaction system to simulate the flow dissolution process of acetic acid and CO2 solution in carbonate pores during the two processes of shallow burial to deep burial and deep burial to shallow burial. The temperature range of the experiment was 30–180°C, the pressure range of the experiment was 5–60°MPa, and the experimental flow rate was 3 ml/min. Combined with industrial CT digital core analysis method and gas pore-permeability analyzer to quantitatively analyze the effect of an acidic fluid on sample pore and connectivity. The concentration of calcium and magnesium ions in the fluid was tested by Leeman Prodigy’s full-spectrum direct reading spectrometer (4,015) and Thermo X Series II inductively coupled plasma mass spectrometry (SN01426C). The mineral composition was analyzed by X-ray diffractometer X’pert Pro, and the results were shown in Table 1. The porosity and permeability of the column sample were analyzed by FYKS-3under overburden pressure. The pore structure of samples was tested by Vltomelx S industrial CT. The test and analysis of the above items were carried out at a temperature of 25°C and a humidity of 40%.
2.3 Experimental process
Before the experiment, the prepared carbonate samples were loaded into the reaction kettle, and then the
Reactor was sealed. The prearranged 1 L reaction solution was poured into the high-pressure buffer vessel, and then the lid was tightened and closed to connect each valve interface. Firstly, carbonate samples were pretreated with reaction solution at room temperature, atmospheric pressure, and 3 ml/min flow rate for 0.5 h. Then the temperature and pressure are raised to the set value, respectively to carry on the experiment. Under the condition of setting the flow rate of the experiment, when the reaction system reaches a stable state, the samples of the solution after the reaction were collected. Generally, the two samples, each with a volume of about 6 ml were collected. The concentration of Ca2+ and Mg2+ was analyzed by ICP-OES.
3 Experimental result
3.1 Pre-existing constituents
To accurately understand the petrology and reservoir space characteristics of deep carbonate reservoirs. A total of 50 thin sections have been identified for rock structure in this study. The specific parameters are shown in Table 2. The carbonate constituents of the reservoir matrix are distributed between 0–72% with an average value of 11.49%. The main component of the rock is dolomite, with content ranging from 17.67 to.
97.33% and an average value of 76.32%. The quartz cement content was distributed at 0–23% with an average value of 3.08%. The pore types of reservoirs are mainly intercrystalline pores, intragranular pores, fracture pores, and vug pores, with average surface porosity of 5.44, 0.14, 1.40, and 1.83%, respectively. The total surface porosity is 0–39%, and the average value is 8.72%. The reservoir crystalline
Size is distributed between 0.015 and 0.6 mm with an average value of 0.11 mm. The reservoir is mainly dominated by silty-crystalline and fine-crystalline dolomite.
3.2 Dissolution results
3.2.1 Parameters analysis before and after the dissolution
The comparison of the data before and after the dissolution experiment can reflect the strength and effect of the dissolution. From Figure 4, it can be seen that the porosity and permeability of the carbonate reservoir increased after dissolution. The increase in permeability is larger than the increase of porosity, indicating that the dissolution is mainly connecting the internal pores of the rock with improved permeability accordingly (Figure 4A). As shown in Figure 4B, the growth of dolomitic limestone is significantly higher than that of the other three dolomite samples, indicating that the degree of dissolution of limestone is significantly higher than that of dolostone.
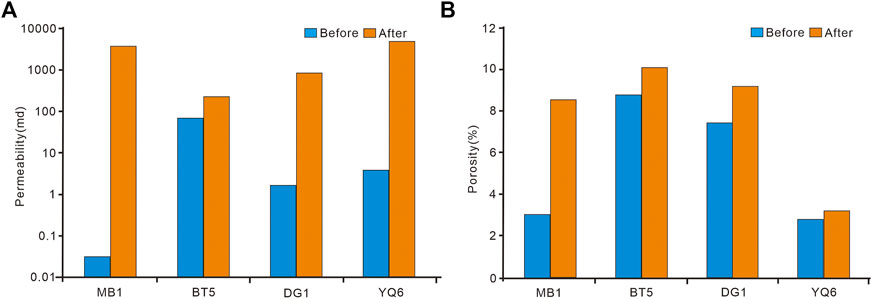
FIGURE 4. Histogram of carbonate porosity and permeability before and after dissolution (A) comparison of permeability before and after dissolution; (B) comparison of porosity before and after dissolution.
Based on the comparison of the parameters before and after the dissolution (Table 3), it can be seen that after the dissolution, the number of pores of the sample was reduced, but the volume was increased. Furthermore, the average radius of pores and throats was also increased from 21.84 to 40.15°μm, and from 15.22 to 40.2 μm.

TABLE 3. Pore-throat parameters of residual fine-crystalline dolarenite before and after Dissolution (CT Scanning Results).
Respectively. It also shows that the dissolution process could connect the pores of samples, which has a significant effect on the improvement of its permeability.
3.2.2 Comparison of CT scanning characteristics before and after the dissolution
CT scanning is an effective tool for the fine characterization of pore structure in rock. The dissolution is greatly affected by the heterogeneity of the original minerals, and the dissolution pores are developed in the areas with high calcite content. The dissolution fluid mainly corrodes along the microfracture of the original rock, which widens the fracture width and connects the interlayer pore system of the reservoir. Therefore, the dissolution effects of different reservoirs vary greatly.
From Figure 5A, the dissolution pores are unevenly distributed. The acidic fluid flows uniformly in the pores of the rock and then reacts with the minerals to transform the pores of the rock evenly. Therefore, the sample still pertains to the pore type after dissolution. As shown in Figure 5B, the dissolution occurs along with the pores, and fractures and dissolution pores increased. After dissolution, the pores and fractures expand to be connected, and the reservoir heterogeneity is significantly enhanced. Figure 5C showed that the dissolution pores increased along the fracture. The fracture is filled with calcite, and the acidic fluid mainly dissolves the calcite along the fracture. Besides, the dissolution greatly increases the permeability of the sample, but the porosity does not change much, and the fracture remains after the dissolution (Figure 5D).
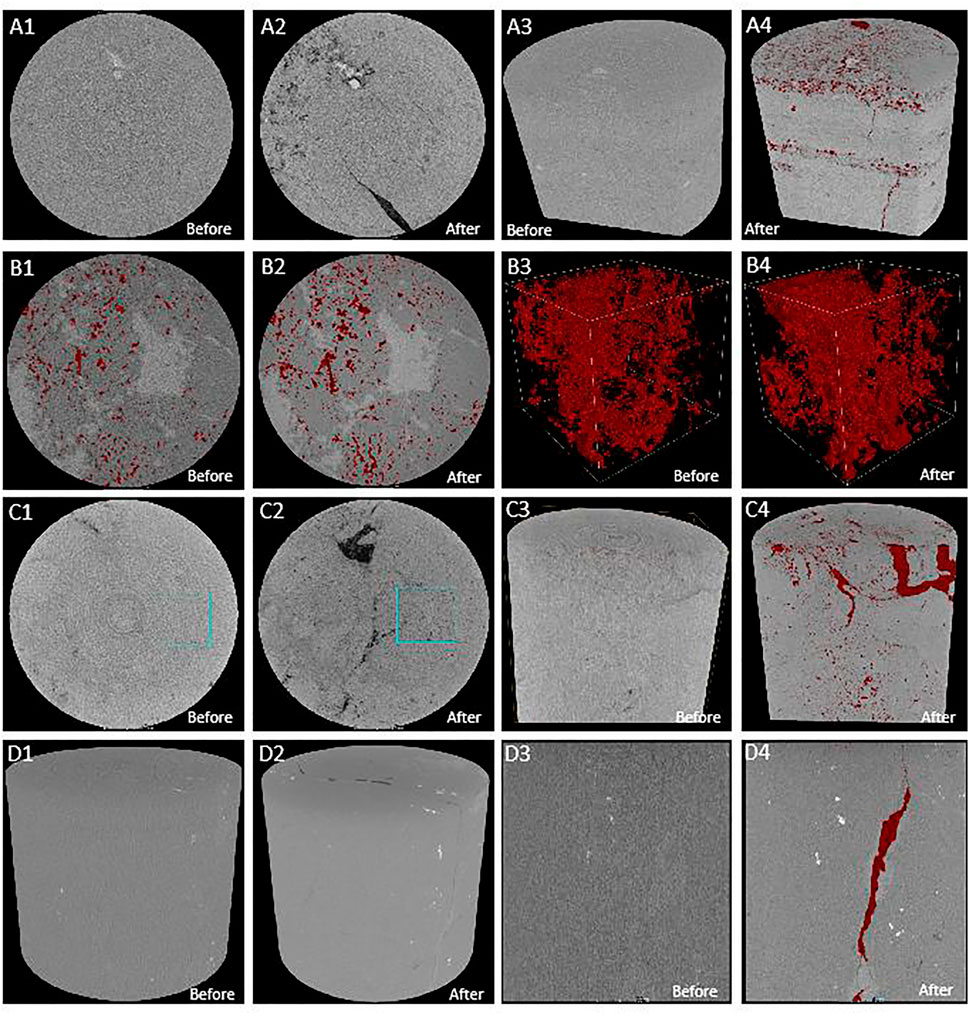
FIGURE 5. Comparison of CT images of the different reservoirs before and after dissolution (A) Comparison of pore structure of dolomitic limestone of MB1 well; (B) comparison of pore structure of residual fine-crystalline dolarenite of BT5 well (C) comparison of pore structure of fine-crystalline dolostone of XH2 well; (D) comparison of pore structure of medium-crystalline dolostone of YQ6 well.
3.2.3 The tendency of dissolution with temperature and pressure
The temperature and pressure are the important factors that affect the carbonate dissolution reaction under a deeply buried environment. Figure 6 reveals the variation of the concentrations of calcium and magnesium ions with temperature and pressure in the solution fluid. Under the same acidic fluid, temperature, and pressure conditions, the amount of dissolution of dolomitic limestone is larger than that of residual fine-crystalline dolarenite. With the increase in temperature and pressure, the amount of dissolution of dolomitic limestone first increased and then decreased. For higher temperature and pressure, the amount of dissolution of dolomitic limestone decreased sharply to the same amount as that of residual fine-crystalline dolarenite. It shows that the dissolution ability decreases with the increase in temperature and pressure.
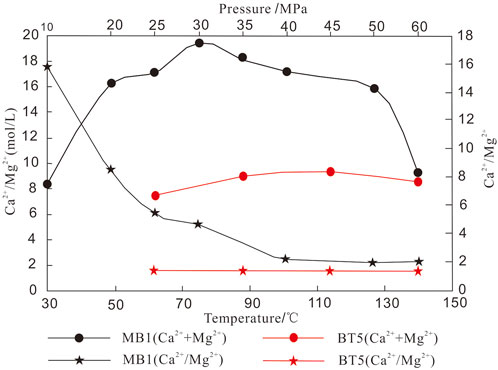
FIGURE 6. Variation trendy of dissolution results of residual fine-crystalline dolarenite and dolomitic limestone (Quoted from Peng et al., 2018).
To investigate the influence of buried history on the dissolution reaction of dolomite. In this paper, the reverse dissolution experiment of dolomite simulation was carried out at different temperatures and pressures from high to low. The amount of dissolution of the residual fine-crystalline dolarenite sample decreased with the decrease in temperature and pressure, and the dissolution rate peaked at 115°C and 45 MPa. According to the regional burial history (Xiong et al., 2015), with the uplift of the stratum, the dissolution of the dolomite reservoir increased at first and then decreased at about 4,000–6,000°m, which proves that there may be a dissolution range, which is referred to as the dissolution threshold in the deep dolomite reservoirs.
4 Discussion
4.1 Effect of original rock composition on dissolution
The dissolution ability of dolomite under a deeply buried environment is always the focus of carbonate reservoir research (Montes-Hernandez et al., 2016; Shen et al., 2016; Guo et al., 2020). Some scholars believed that the energy required for dolomite dissolution under burial conditions was less than that of calcite, and the automatic dissolution tendency was higher than that of calcite. Therefore, dolomite is more soluble than limestone under burial conditions (Zhu et al., 2015). Other scholars believed that in the shallow burial environment, limestone has a greater solubility in acetic acid solution than dolomite. But in a medium-deep burial environment (Dong et al., 2018), dolomite has a higher solubility in acetic acid solution than limestone (She et al., 2013; She et al., 2016), indicating temperature and pressure have a significant influence on the dissolution of the original rock. The results of this dissolution experiment (Figure 6) show that the dissolution in tensity of samples containing calcite is much higher. For example, the dolomitic limestone of the MB1 well has the largest amount of dissolution among the four samples. Under different temperature and pressure conditions, the dissolution of the dolomite sample is higher than that of BT5 well, indicating that calcite is more soluble than dolomite in a deep burial environment. Microscopic observation can be seen that the fine-medium crystal dolomite was subjected to dissolution and the intragranular pores developed (Figures 7A–C). The horizontal layer distribution is shown in the core of the MB1 well with the elongated dissolution pores (Figure 7D), the microfracture pores, and the interparticle pores of the BT5 well (Figures 7E,F), indicating that the pre-existing pores formed in the early diagenesis are favorable for the formation of secondary pores. From reservoir pore structure statistics (Table 2), it can also be seen that the dissolved pores, intercrystalline pores (Figure 8A), and the surface porosity (Figure 8B), that the dissolution pores have a good correlation with the reservoir pores. Considering that the relatively large dissolution pores are related to the early limestone dissolution, it can be speculated that the dissolution may be attributed to calcite fossils in the early diagenetic period, which contributes to a deeper dissolution.
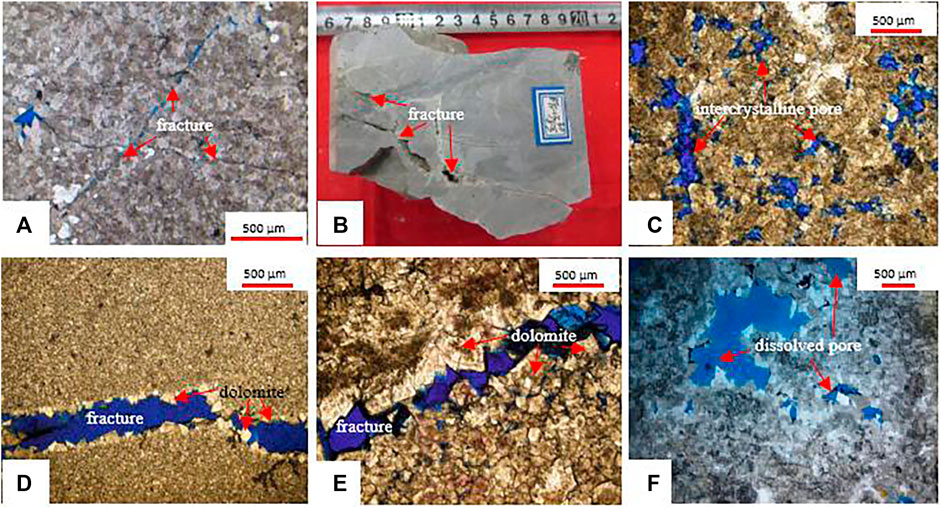
FIGURE 7. Characteristics of dissolution pores in Cambrian reservoirs. (A) medium-crystalline dolomite, BT5, ∈3, 4,812.9 m, cross-polarized light, ×50 (B) fine-crystalline dolomite, BT5, ∈3, 4,811.01–4,811.10 m; (C) residual fine-crystalline dolarenite, BT5, ∈1, 5,784.8 m, plane polarized light, ×50 (D) fracture filled with allochthonous dolomite, XH2, ∈35,595.761 m, blue thin sections, plane polarized light, ×50; (E) fine-crystalline dolostone, YQ6, ∈37,119.93 m, blue thin sections, plane polarized light, ×50; (F) fine-crystalline dolomite, TS1, ∈37,875.66–74 m, blue thin sections, plane polarized light, ×50.
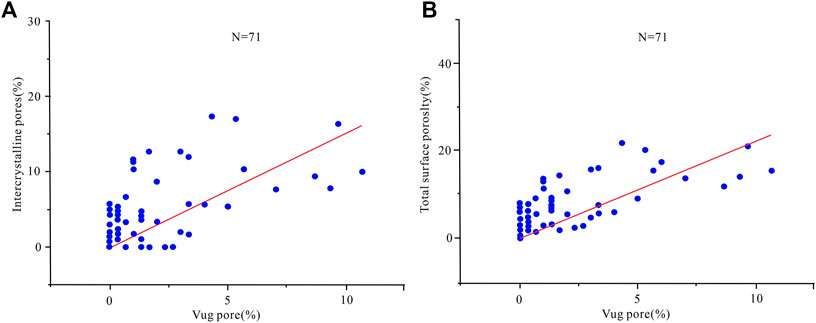
FIGURE 8. Relationship between dissolution pores and surface porosity of Cambrian reservoirs in Tarim Basin (A) Intercrystalline pore vs. dissolution pore; (B) total surface porosity vs. dissolution pore.
4.2 Influence of pore structure
The influence of preexisting pore structure on the burial dissolution of carbonate rocks is a problem worthy of attention (Luhmann et al., 2014). Due to the strong heterogeneity of the pore structure in deep reservoirs, it is difficult to draw effective conclusions and understandings. In this experiment two sets of comparative samples with a porosity of 2.027% and a permeability of 0.0148mD in the DG1 well were selected. The first set of early-developed pores and fractures were characterized by microscopic features as shown in Figure 2D. The second set had relatively denser lithology (Figure 2D), and early connected pores have not been fully developed. Under the temperature and pressure conditions of 40°C and 70 MPa, the acidic fluid never passed the second set of samples. After 4 hours of reaction, there was no obvious dissolution phenomenon observed on the surface of the sample. The experimental results show that the pre-existing pores and fractures stimulate the dissolution process, and the dissolution under buried conditions is caused by the dissolution and the adjustment of pre-existing pores.
According to the characteristics of the variations in porosity and permeability, the porosity of the sample increased after dissolution to some extent, while the permeability generally increased dramatically. It indicates that dissolution can connect pores and improve the seepage capacity. Especially for the samples with fractures, dissolution forms the dominant channels, which greatly increases the permeability. It is found that the increase in the permeability measured by the experiment can reach up to 1,257.26%.
The pore structure of the original rock controls the characteristics of the fluid flow and determines the result of the dissolution (Lambert et al., 2006; Beckert et al., 2016; Kirstein et al., 2016; Shen et al., 2016; Abarca et al., 2019). The Cambrian strata in the Tarim Basin have experienced multi-stage tectonic activities, and tectonic fractures developed, which provides a good seepage channel for the intrusion of diagenetic fluids (Figures 7A–F). It can be seen that the quartz content in the reservoir has a certain correlation with the intercrystalline pore (Figure 9A) and the total surface porosity (Figure 9B). It can be considered that the acidic fluid mostly percolates and dissolves along with the early pre-existing pore system, and precipitated.
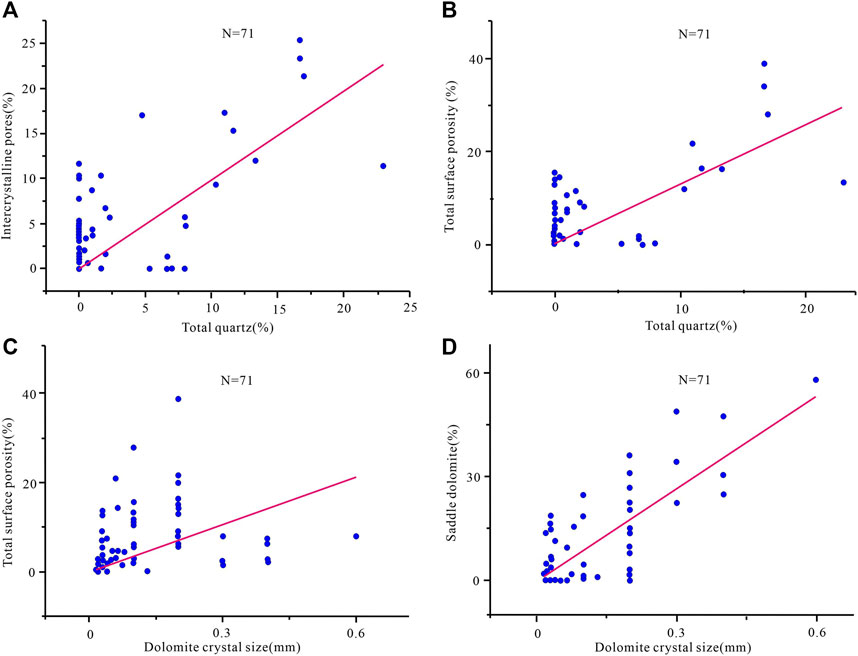
FIGURE 9. Relationship between reservoir composition and surface porosity of Cambrian in Tarim Basin (A) Quartz cement vs. intercrystalline pore; (B) quartz cement vs. total surface porosity (C) dolomite crystalline size vs. total surface porosity; (D) dolomite crystalline size vs. saddle dolomite content.
Based on the relationship obtained between the dolomite crystal size and the total surface porosity (Figure 9C) and the saddle dolomite content (Figure 9D), it can be seen that the pores formed in the early diagenetic period provide a seepage channel for the later dolomitization. It is of great significance and may be the main reason for the formation of high-quality deep carbonate reservoirs.
4.3 Burial dissolution
Burial dissolution is an important geologic process for the formation of deep carbonate reservoirs. The typical characteristics are that the dissolved pores in the Cambrian reservoir are distributed obviously along the stratum (Figure 7C). The part of the dissolved pores was filled by bitumen, which reflects that the reservoir was reformed by deep fluids (Figures 7D–F). The important condition for deep burial dissolution is the presence of a large number of acidic fluids. There is still no consensus on the source of acid fluid in a deeply buried environment. Some scholars believe that the acidic dissolution of large-scale reservoirs requires a large number of organic acid sources, but the existing source rocks cannot meet this condition under a closed environment (Ehrenberg et al., 2012; Shen et al., 2016). Therefore, the source of carbon dioxide buried deep in the environment is the key to explaining this phenomenon (Guo et al., 2020; Lima et al., 2020; Valenciaa et al., 2020). From the CO2 solution experiment, it can see that the amount of mineral dissolution increased at first and then decreased with the increase in temperature and pressure (Figure 10), and the amount of dissolution of the acetic acid solution was larger than that of the CO2 solution. However, the simulation experiment results show that there is a high-efficiency range of carbonic acid dissolution in a deep-buried environment, and the temperature is about 70–120°C.
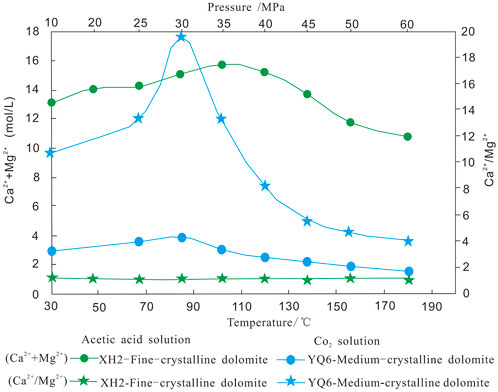
FIGURE 10. Variation trend of dissolution results of fine-grained dolomite and medium-dolomite with temperature and pressure.
The combined analysis of the experimental results and the burial history indicates that in the shallow to middle burial depth (3000–5000 m) if sufficient acidic fluid exists, the carbonate reservoir may develope.
Large-scale dissolution pore (Feng et al., 2013; Shen et al., 2016). Fracture is an important factor affecting burial dissolution. Considering the importance of shallow burial on the dissolution of dolomite reservoirs, some scholars have suggested that the dissolution and precipitation of carbonates have a reverse dissolution mode (Valenciaa et al., 2020), and the variation of fluid saturation caused by tectonic uplift or basin subsidence is an important mechanism for controlling the formation of secondary pores (Shen et al., 2016). The CO2 dissolved in the hydrothermal solution at high temperature and high pressure, and the carbonic acid that formed corroded and altered the residual pores along the seepage channels, such as fractures and intergranular solution pores (Figure 7). Due to the influence of tectonics, acidic fluid connected some pre-existing residual pores and expanded primary pores and fractures along with fractures and intercrystalline pores (Figure 11), which forms a secondary pore system with good local connectivity. This indicates that late-stage tectonics is important for the formation of high-quality deep reservoirs. Therefore, according to the distribution of extensional faults and the characteristics of hydrocarbon migration, it is of great concern to search for the Cambrian dolostone reservoirs near the Himalayan faults.
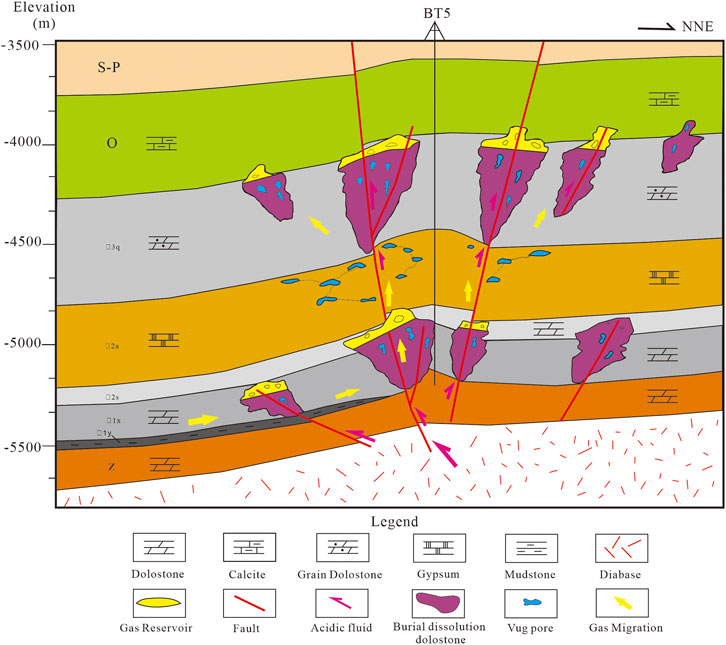
FIGURE 11. The schematic model showing that faults and host facies control the fluid flow in the formation of dissolution pores in the Cambrian reservoir in the Tarim Basin.
5 Conclusion
1) The high temperature and high-pressure carbonate simulation experiment show that the organic acid (acetic acid solution) has a higher ability to dissolve the carbonate rock than the carbon dioxide solution. In the shallow-middle depth stage, the dissolution ability of acidic fluids on carbonate rocks tends to increase first and then decrease, indicating that there is a favorable range of dissolution pores in carbonate reservoirs.
2) The pore structure of the original rock has a great influence on the dissolution of deep reservoirs. The porosity and permeability of the porous reservoir increased correspondingly after dissolution, and the permeability of the fracture-type and pore-fracture samples was improved after dissolution, indicating that the pre-existing pores formed in shallow-middle depth may be the key to the formation of high-quality reservoirs in a deeply buried environment.
3) In this study, a dolomite regression dissolution simulation experiment was designed to confirm that the dissolution of dolomite reservoirs tended to increase first and then decrease, corresponding to the shallow-middle buried stage and ranging from 3,000 to 5,000 m.
4) The genetic model of burial dissolution in deep carbonate reservoir is established and shows that the dissolution of CO2 to form carbonic acid in the hydrothermal fluid can promote an increase of porosity in carbonate reservoirs. Therefore, according to the distribution of the extensional fault and the characteristics of hydrocarbon migration, it is important to search for the Cambrian dolostone reservoir near the Himalayan faults.
Data availability statement
The original contributions presented in the study are included in the article/Supplementary Material, further inquiries can be directed to the corresponding author.
Author contributions
XZ: data curation. BL: conceptualization, methodology. JP: visualization. FQ: conception, design and consult. KZ: supervision. SY: validation. QX: data.
Funding
This research was supported by the Regional innovation Cooperation Project from the Science and Technology Department of Sichuan Province (grant number No.21QYCX0048).
Acknowledgments
We would like to acknowledge Design and Planning Institute of SINOPEC Northwest Company for their assistance. We are grateful to Shuai Yin and two reviewers for the constructive reviews of the manuscript.
Conflict of interest
Authors SY and QX were employed by the company Design and Planning Institute of SINOPEC Northwest Company.
The remaining authors declare that the research was conducted in the absence of any commercial or financial relationships that could be construed as a potential conflict of interest.
Publisher’s note
All claims expressed in this article are solely those of the authors and do not necessarily represent those of their affiliated organizations, or those of the publisher, the editors and the reviewers. Any product that may be evaluated in this article, or claim that may be made by its manufacturer, is not guaranteed or endorsed by the publisher.
References
Abarca, E., Idiart, A., Grandia, F., Rodríguez-Morillas, N., Pellan, C., Zen, M., et al. (2019). 3D reactive transport modeling of porosity evolution in a carbonate reservoir through dolomitization. Chem. Geol. 513, 184–199. doi:10.1016/j.chemgeo.2019.03.017
Beckert, B., Vandeginste, V., and John, C. M. (2016). Relationship between karstification and burial dolomitization in Permian platform carbonates (Lower Khuff-Oman. Sediment. Geololgy 342, 165–179. doi:10.1016/j.sedgeo.2016.07.001
Biehl, B. C., Reuning, L., Schoenherr, J., Lewin, A., Leupold, M., and Kukla, P. A. (2016). Do CO2-charged fluids contribute to secondary porosity creation in deeply buried carbonates? Mar. Petroleum Geol. 76, 176–186. doi:10.1016/j.marpetgeo.2016.05.005
Cai, C. F., He, W. X., Jiang, L., Li, K. K., Xiang, L., and Jia, L. (2014). Petrological and geochemical constraints on porosity difference between Lower Triassic sour- and sweet-gas carbonate reservoirs in the Sichuan Basin. Mar. Petroleum Geol. 56, 34–50. doi:10.1016/j.marpetgeo.2014.04.003
Cheng, B. H., Liu, Z., Cao, X., Wu, Z., and Chen, Z. (2020). Origin of deep oil accumulations in carbonate reservoirs within the north Tarim Basin: Insights from molecular and isotopic compositions. Org. Geochem. 139, 103931. doi:10.1016/j.orggeochem.2019.103931
Dong, K. D., Zhu, A. D., and Hill, A. D. (2018). The role of temperature on optimal conditions in dolomite acidizing: An experimental study and its applications. J. Petroleum Sci. Eng. 165, 736–742. doi:10.1016/j.petrol.2018.03.018
Ehrenberg, S. N., Walderhaug, O., and Bjørlykke, K. (2012). Carbonate porosity creation by mesogenetic dissolution: Reality or illusion? Bulletin 96, 217–233. doi:10.1306/05031110187
Feng, J. J., Cao, K., Hu, X., Peng, Y., Chen, Y., Wang, M., et al. (2013). Dissolution and its impacts on reservoir formation in moderately to deeply buried strata of mixed siliciclastic-carbonate sediments, northwestern Qaidam Basin, northwest China. Mar. Petroleum Geol. 39, 124–137. doi:10.1016/j.marpetgeo.2012.09.002
Guo, R. S., Zhang, X., Bai, K., Wang, X., Sun, X., and Liu, X. (2020). Hydrothermal dolomite reservoirs in a fault system and the factors controlling reservoir formation-A case study of Lower Paleozoic carbonate reservoirs in the Gucheng area, Tarim Basin. Mar. Petroleum Geol. 120, 104506. doi:10.1016/j.marpetgeo.2020.104506
Hao, F., Zhang, X. F., Wang, C. W., Li, P. P., Guo, T. L., Zou, H. Y., et al. (2015). The fate of CO2 derived from thermochemical sulfate reduction (TSR) and effect of TSR on carbonate porosity and permeability, Sichuan Basin, China. Earth-Science Rev. 141, 154–177. doi:10.1016/j.earscirev.2014.12.001
Hood, S. D., Campell, S., Kamp, P. J. J., and Nelson, S. (2004). Burial dolomitisation in a non-tropical carbonate petroleum reservoir: The oligocene tikorangi formation, taranaki basin, New Zealand. Sediment. Geol. 172, 117–138. doi:10.1016/j.sedgeo.2004.08.005
Howarth, V., and Alves, T. M. (2016). Fluid flow through carbonate platforms as evidence for deep-seated reservoirs in Northwest Australia. Mar. Geol. 380, 17–43. doi:10.1016/j.margeo.2016.06.011
Huang, T. Z., Jiang, H. S., and Ma, Q. Y. (2014). Hydrocarbon accumulation characteristics in lower paleozoic carbonate reservoirs of Tarim Basin. Petroleum Nat. Gas Geol. 35, 780–787. doi:10.1007/BF02875312
Jiao, F. Z. (2019). Practice and knowledge of volumetric development of deep fractured-vuggy carbonate reservoirs in Tarim Basin, NW China. Petroleum Explor. Dev. 46, 576–582. doi:10.1016/s1876-3804(19)60037-6
Kirstein, J. H., Hellevang, B. G., Haile, G., and Gaupp, G, R. (2016). Experimental determination of natural carbonate rock dissolution rates with a focus on temperature dependency. Geomorphology 261, 30–40. doi:10.1016/j.geomorph.2016.02.019
Lambert, L., C., Durlet, J., Loreau, G., and Marnier, R. (2006). Burial dissolution of micrite in Middle East carbonate reservoirs (Jurassic-Cretaceous) keys for recognition and timing. Mar. Petroleum Geol. 23, 79–92. doi:10.1016/j.marpetgeo.2005.04.003
Li, B., and Goldberg, K. (2022). Diagenesis and reservoir quality of Cambrian carbonates in the Tarim Basin, northwestern China. J. Asian Earth Sci. 223, 104972. doi:10.1016/j.jseaes.2021.104972
Li, B., Xia, Q. S., Peng, J., Yang, S. J., Xu, Q. Q., and Zeng, L. (2019). Characteristics and dolomitization of the lower qiulitage group reservoir in bachu uplift, Tarim Basin, China. Earth Sci. Res. J. 23, 199–208. doi:10.15446/esrj.v23n3.81914
Li, H., Qin, Q. R., Zhang, B. J., Ge, X. Y., Hu, X., Fan, C. H., et al. (2020). Tectonic fracture formation and distribution in ultradeep marine carbonate gas reservoirs: A case study of the maokou formation in the jiulongshan gas field, sichuan basin, southwest China. Energy & Fuels 34 (11), 14132–14146. doi:10.1021/acs.energyfuels.0c03327
Lima, B. E. M., Tedeschi, A. L. S., Pestilho, R. V., Santos, J. C., Vazquez, J. V. P., Guzzo, L. F., et al. (2020). Deep-burial hydrothermal alteration of the Pre-Salt carbonate reservoirs from northern Campos Basin, offshore Brazil: Evidence from petrography, fluid inclusions, Sr, C and O isotopes. Mar. Petroleum Geol. 113, 104143. doi:10.1016/j.marpetgeo.2019.104143
Liu, W., Liu, N. S., Qiu, Q. C., and Xu, Q. (2018). The evolution of pore-fluid pressure and its causes in the Sinian-Cambrian deep carbonate gas reservoirs in central Sichuan Basin, southwestern China. J. Petroleum Sci. Eng. 169, 96–108. doi:10.1016/j.petrol.2018.05.057
Luhmann, A. J., Kong, B. M., Tutolo, N., Garapati, B. C., Bagley, M. O., Saar, W. E., et al. (2014). Experimental dissolution of dolomite by CO2-charged brine at 100°C and 150bar: Evolution of porosity, permeability, and reactive surface area. Chem. Geol. 380, 145–160. doi:10.1016/j.chemgeo.2014.05.001
Luo, C., Chen, X., Shi, Z., Chen, J., Wu, T., Shi, C., et al. (2022). Effects of precipitation and dissolution of carbonate cements on the quality of deeply buried high-temperature and overpressured clastic reservoirs: XD 10 block, yinggehai basin, south China sea. Mar. Petroleum Geol. 139, 105591. doi:10.1016/j.marpetgeo.2022.105591
Ma, Y., Cai, X., Yun, L., Li, Z., Li, H., Deng, S., et al. (2022). Practice and theoretical and technical progress in exploration and development of Shunbei ultra-deep carbonate oil and gas field, Tarim Basin, NW China. Petroleum Explor. Dev. 49 (1), 1–20. doi:10.1016/s1876-3804(22)60001-6
Montes-Hernandez, G., N., Findling, F., and Renard, F. (2016). Dissolution-precipitation reactions controlling fast formation of dolomite under hydrothermal conditions. Appl. Geochem. 73, 169–177. doi:10.1016/j.apgeochem.2016.08.011
Peng, J., Wang, X., and Han, H. (2018). Simulation experiment for the dissolution mechanism of carbonate rock of Cambrian in the Tarim Basin. Petroleum Exploration and Development 45, 1–11. doi:10.11698/PED.2018.03.00
She, M., Shou, J. F., He, X. Y., Wang, Y., and Zhu, Y. (2013). Experiment of dissolution mechanism of carbonate rocks:surface dissolution and internal dissolution. Mar. oil gas Geol. 18, 55–61. doi:10.1155/2017/8439259
She, M., Shou, J. F., Sheng, A. J., and Pan, L. Y. (2016). Experimental simulation of dissolution law and porosity evolution of carbonate rock. Petroleum Explor. Dev. 43 (4), 564–573. doi:10.1016/s1876-3804(16)30072-6
Shen, A. J., Zheng, J. F., Chen, Y. Q., Ni, X. F., and Huang, L. L. (2016). Characteristics, origin and distribution of dolomite reservoirs in LowerMiddle cambrian, Tarim Basin, NW China. Pet. Explor Dev. 43 (3), 375–385. doi:10.1016/s1876-3804(16)30044-1
Tavakoli, V., and Jamalian, A. (2019). Porosity evolution in dolomitized Permian–Triassic strata of the Persian Gulf, insights into the porosity origin of dolomite reservoirs. J. Petroleum Sci. Eng. 181, 1–12. doi:10.1016/j.petrol.2019.106191
Tian, F., X., Luo, W., and Zhang, S. (2019). Integrated geological-geophysical characterizations of deeply buried fractured-vuggy carbonate reservoirs in Ordovician strata, Tarim Basin. Mar. Petroleum Geol. 99, 292–309. doi:10.1016/j.marpetgeo.2018.10.028
Tutolo, B. M., Kong, X. Z., Seyfried, W. E., and Saar, M. O. (2015). High performance reactive transport simulations examining the effects of thermal, hydraulic, and chemical (THC) gradients on fluid injectivity at carbonate CCUS reservoir scales. Int. J. Greenh. Gas Contro 39, 285–301. doi:10.1016/j.ijggc.2015.05.026
Valenciaa, F. L., and Layab, J. C. (2020). Deep-burial dissolution in an oligocene-miocene giant carbonate reservoir (perla limestone), gulf of Venezuela basin: Implications on microporosity development. Mar. Petroleum Geol. 113, 1–21. doi:10.1016/j.marpetgeo.2019.104144
Xiong, Y. X., Chen, Y. Q., Guan, B. Z., Deng, L. P., Ni, X. F., and Xiong, R. (2015). Distribution of northern platform margin and implications to favorable exploration regions on lower cambrian xiaoerbulake formation, Tarim Basin. J. Sedimentology 33, 408–415. doi:10.14027/j.cnki.cjxb.2015.02.020
Yasuda, E., Ronaldo Gonçalves, S., and Santos, O. (2013). Kinetics of carbonate dissolution and its effects on the porosity and permeability of consolidated porous media. J. Petroleum Sci. Eng. 112, 284–289. doi:10.1016/j.petrol.2013.11.015
Zhu, D., Q., Meng, Z., Jin, Q., Liu, W., and Hu, J. (2015). Formation mechanism of deep Cambrian dolomite reservoirs in the Tarim basin, northwestern China. Mar. Petroleum Geol. 59, 232–244. doi:10.1016/j.marpetgeo.2014.08.022
Zhu, G., Zhang, Y., Zhou, X., Zhang, Z., Du, D., Shi, S., et al. (2019). TSR, deep oil cracking and exploration potential in the Hetianhe gas field, Tarim Basin, China. Fuel 236, 1078–1092. doi:10.1016/j.fuel.2018.08.119
Keywords: deep carbonate reservoir, simulation experiment, burial dissolution, Tarim Basin, Cambrian
Citation: Zhang X, Li B, Peng J, Qu F, Zhang K, Yang S and Xu Q (2022) Experimental analysis of dissolution reconstruction of deep dolomite reservoirs: A case study of the Cambrian dolomite reservoirs in the Tarim Basin. Front. Earth Sci. 10:1015460. doi: 10.3389/feart.2022.1015460
Received: 09 August 2022; Accepted: 08 September 2022;
Published: 29 September 2022.
Edited by:
Shuai Yin, Xi’an Shiyou University, ChinaReviewed by:
Yingquan Zhao, Chengdu University of Technology, ChinaChuanxin Li, China University of Geosciences, China
Copyright © 2022 Zhang, Li, Peng, Qu, Zhang, Yang and Xu. This is an open-access article distributed under the terms of the Creative Commons Attribution License (CC BY). The use, distribution or reproduction in other forums is permitted, provided the original author(s) and the copyright owner(s) are credited and that the original publication in this journal is cited, in accordance with accepted academic practice. No use, distribution or reproduction is permitted which does not comply with these terms.
*Correspondence: Bin Li, libinxnsy@outlook.com