- 1National Research Council of Italy (CNR) - Institute of Geosciences and Georesources (IGG), Pisa, Italy
- 2ENEA Italian National Agency for New Technologies, Italian National Agency for New Technologies (ENEA) - Energy and Sustainable Economic Development, Marine Environment Research Centre S.Teresa, La Spezia, Italy
We investigated helium isotopes on gas extracted by crushing from melt and fluid inclusions in minerals from Plinian and inter-Plinian tephra and lavas of Vesuvius, Italy. Erupted products of different ages were considered, from Avellino eruption (1995 BCE) to the last eruption of 1944, with special focus on the 79 AD Plinian eruption.
Introduction
The volcanoes of Monte Somma, Vesuvius, and Phlegraean Fields occupy the southern part of the Campania Plain, southern Italy, and border a densely populated region currently inhabited by more than two million people that also includes the metropolitan city of Naples. Starting in the 1980s’, the high volcanic risk related with the possible renewal of activity of one of these volcanoes fostered an intense scientific effort to increase knowledge on the eruptive history and mechanisms of these volcanoes (e.g., Barberi et al., 1984; De Vivo et al., 1993). Present volcanic activity mostly consists of active seismicity, ground deformation with alternation of deflation/inflation episodes (mostly in the Phlegraean Fields area), moderate fumarolic emissions, and hot spring discharges.
At Vesuvius, over the last decades, a large number of rock and fluid samples have been collected, and an extended database of mineralogical and geochemical analyses of various deposits is now available (De Vivo et al., 2010; Peccerillo, 2020). The historic activity of the Vesuvius volcanic system is characterized by occasional Plinian eruptions (Avellino, 79 AD, 472 AD, 1631) separated by periods of inactivity or semi-persistent activity (Arnò et al., 1987). During semi-persistent phases of activity, the volcano experienced an open-conduit behavior, and produced effusive, often from flank vents (Principe et al., 2004), and mixed (effusive and explosive) eruptions, from Strombolian, to Violent Strombolian and Sub-Plinian type (Arrighi et al., 2001).
As the last eruption occurred in 1944, most of the current scientific interest is in the possible correlation between ongoing observed dynamics and past geological record. In particular, the link between present-day fluid composition and magma degassing at depth is considered a key element in understanding the mechanisms that could control any possible future volcanic activity.
Due to its well-defined isotopic signature for different geological settings (e.g., Kurz et al., 1982; Ozima and Podosek, 1983; Mamyrin and Tolstikhin, 1984; Burnard, 2013), the helium isotope composition (expressed as the
At Vesuvius,
Geological-volcanological setting
Monte Somma is a complex volcanic structure affected by several calderas and structural collapses (e.g., Principe et al., 2021). In the middle of the resulting morphological depression, the volcanic cone of Vesuvius has grown. Along with the Phlegraean Fields volcanic area and the Roccamonfina stratovolcano, Monte Somma-Vesuvius volcano is hosted in a large graben within the Campania plain (Figure 1) that originated during the Upper Pliocene-Lower Pleistocene. This graben is part of a macro-regional extensional system that stretches along the Tyrrhenian margin of the Apennine mountainous chain, from southern Toscana to northern Calabria. This belt experienced widespread volcanism in Pleistocene (e.g., Scandone, 1979; Turco et al., 2006).
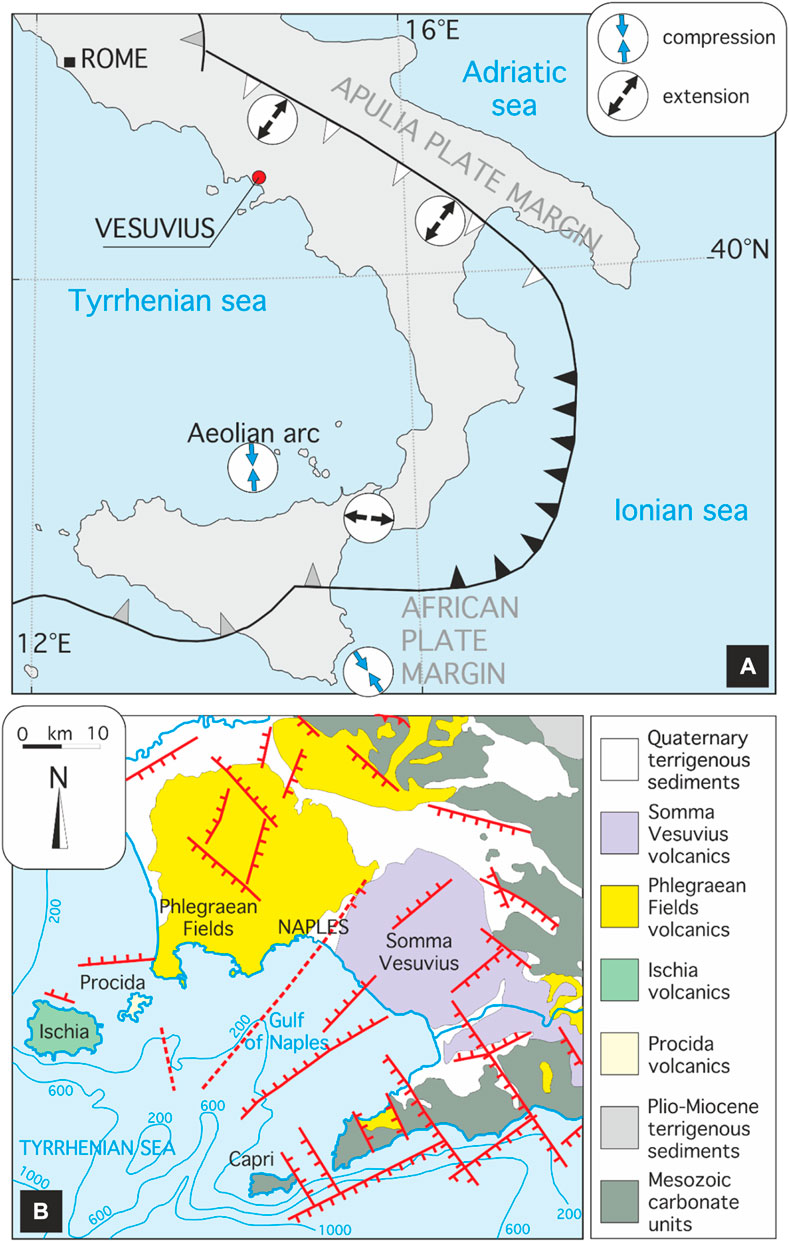
FIGURE 1. (A) Geodynamical sketch map of Southern Italy, with location of the Monte Somma and Vesuvius (modified after Montone et al., 1999). Bold lines = structural arcs; shaded triangles = active compressional fronts; solid triangles = active oceanic subduction; open triangles = front of the Plio-Pleistocene thrust, now prevalently affected by extension. (B) Simplified geological map of the Campania plain and volcanic districts.
The volcanic activity in the area now occupied by Monte Somma and Vesuvius started about 400 ka BP (Brocchini et al., 2001). The outcropping deposits all belong to the volcanic activity following the Campanian Ignimbrite eruption (about 39 ka ago; Rosi and Sbrana, 1987). Dated to about 22 ka BP (Cioni et al., 2008), the Pomici di Base eruption—the oldest eruption considered in the present work—is the first one and the major Plinian eruption occurred in Vesuvius area (Bertagnini et al., 1998). Other major explosive eruptions followed, such as the Greenish Pumices Sub-Plinian eruption (about 20 ka BP; Cioni et al., 2003), and the Pomici di Mercato Plinian eruption (about 8,900 years BP; Mele et al., 2011). The activity occurred at Vesuvius during the last 4 ka BP has been subdivided into four synthematic units, on the basis of major geological events, marked by depositional unconformities (Paolillo et al., 2016): 1) Proto -Vesuvius, between the Plinian eruptions of Pomici di Avellino (about 4 ka ago) and 79 AD; 2) Ancient Vesuvius, between 79 AD Plinian eruption and 472 AD Sub-Plinian eruption; 3) Medieval Vesuvius, from the 472 AD Sub-Plinian eruption and the 1631 small-scale Plinian eruption; 4) Present Vesuvius, between 1631 and 1944 eruptions.
The Medieval Vesuvius activity is characterized by the emission of lava flows from flank vents opened on the slopes of the Vesuvius cone, and of Strombolian and Violent Strombolian scoriae fallout (Principe et al., 2004; Paolillo et al., 2016). During the Present Vesuvius period, the volcano passed from effusive and markedly Strombolian activity to episodes of violent Strombolian and Sub-Plinian eruptions with mixed effusive and explosive character (Arrighi et al., 2001).
Since the last eruption of 1944, Vesuvius entered a period of eruptive rest. In the crater area, temperatures between 600 and 800°C were recorded in the fumaroles during the period 1944–1960 (Chiodini et al., 2001), before starting to gently decrease close to the boiling point of water at the crater altitude (around 95°C) during the 1990s, down to present-day sub-boiling temperatures (about 72°C). The residual activity consists of fumaroles, diffuse degassing (e.g., Baubroun et al., 1991; Chiodini et al., 2001; Federico et al., 2002; Frondini et al., 2004), general subsidence and low-magnitude seismicity (e.g., Ricco et al., 2021; and references therein). The present-day, mildly fumarolic activity is concentrated near the crater only, whereas diffuse soil emissions widely occur around the flanks of the volcano, in correspondence of structural and volcano-tectonic elements (Paolillo et al., 2016). In this area, the extensive interaction between rising magmatic fluids and groundwater has been revealed by C-He systematics of dissolved species (Federico et al., 2002).
Methods
Bulk rocks samples were disaggregated and the mineral phases of interest separated with a magnet separator. Then, olivine and pyroxene crystals were carefully collected from the enriched fraction by handpicking under binocular microscope, and cleaned ultrasonically. Mineral samples successively underwent several hours under-vacuum degassing at 100–150°C to minimize air contamination on crystal surfaces. Fluid inclusions gases were then extracted by under-vacuum crushing of 1–2 g of the selected mineral fragments (grain size from 0.5 to 1 mm). The crushing efficiency was checked by verifying that the granulometric size of the powder was below 20
Both fumarolic gases and minerals were processed on a stainless-steel vacuum line equipped with cold and hot Ti getters to separate noble gases from the gaseous mixture. The extraction line was connected to both a magnetic mass spectrometer (MAP 215-50) equipped with ion counting detector, and a quadrupole mass spectrometer (Spectralab 200, VG-Micromass; Magro et al., 2003).
The
A standard volume of air at different pressures (from 1,013 to 10.13 mbar) was introduced into the extraction line and processed like the samples. The reproducibility of
The
Cross controls were performed to exclude C isotopic fractionation during crushing, and in particular, we verified that
Results and discussion
The concentration and the isotopic composition of He, and the
The
The
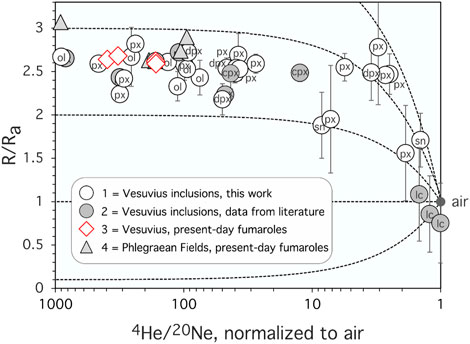
FIGURE 2.
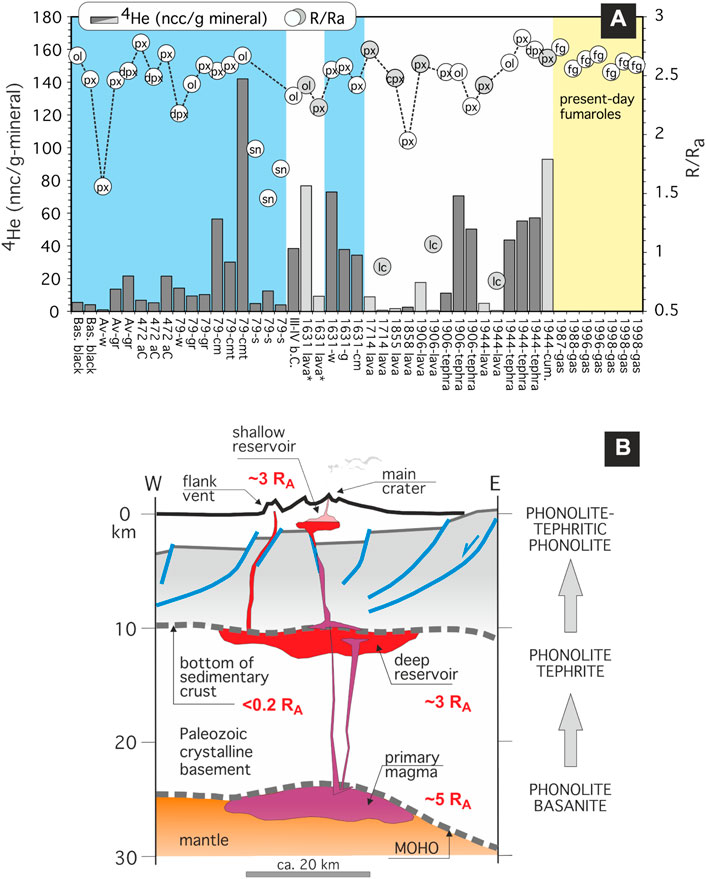
FIGURE 3. (A) R/RA ratios (dots) and 4He contents (bars) for different, individual eruptions (abundances are in ncc/grams of crushed mineral). Empty dots/grey bars: data from this work. Pale grey dots/pale grey bars: data after Graham et al. (1993). Leucites after Graham et al. (1993) were likely compromised by air contamination (low He concentrations, and air-like 4He/20Ne and 3He/4He ratios). The dashed line connects olivine and pyroxene samples. (*) 1,631 lava samples should be attributed to Medieval age activity Principe et al. (2004). Pale blue background: closed conduit activity; white background: open conduit activity; pale yellow background: present-day fumarolic activity. (B) Simplified conceptual model of Vesuvius, with indication of various hypothetical levels of magma accumulation and differentiation (modified after Stoppa et al., 2017). Volcanic activity is driven by the recurrent arrival of primitive mafic magma batches from the mantle into an extended deep-seated reservoir (“deep reservoir”), where homogenization occurs with resident, pre-existing magmas. From this reservoir, magmas can be directly emitted at the surface, or stored into shallow magma chambers (“shallow reservoir”), giving origin to the different eruptive styles and types of volcanic deposits of Vesuvius. Numbers in red are approximate 3He/4He signatures of different domains/reservoirs.
Data from literature suggest low
Data from this work point to a negligible effect (at least on He isotope distribution) of processes such as later crystallization and/or wall rock assimilation in magma chambers, because pyroxenes are not characterized by a
A tendency of
Low
Irrespective of these hypotheses, the correlation between He amounts and
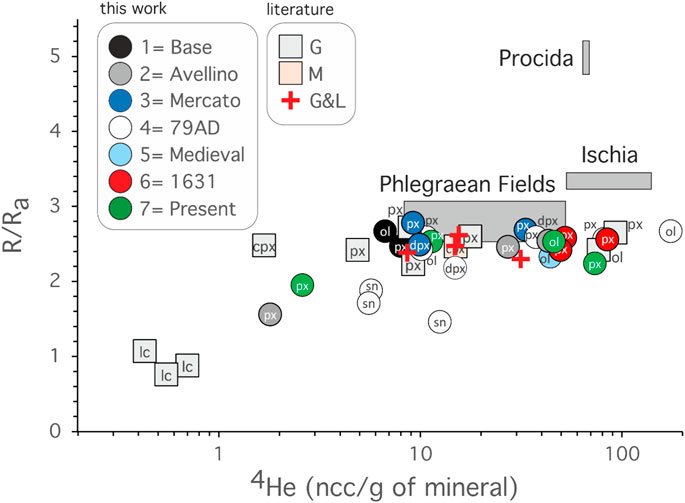
FIGURE 4. R/RA vs. 4He correlation diagram. Data from this work: 1 = Pomici di Base Plinian eruption black pumice; 2 = Pomici di Avellino Plinian eruption; 3 = Pomici di Mercato Plinian eruption; 4 = 79 AD Plinian eruption; 5 = Medieval Vesuvius, Calastro lava; 6 = 1,631 small-scale Plinian eruption; 7 = Present Vesuvius activity. Data from literature: G = Graham et al. (1993); M = Martelli et al. (2004); G&L = Graham and Lupton (1999). The compositional fields for Phlegraean Fields, Procida and Ischia islands are also shown for comparison (data from Martelli et al., 2004). Labels as in Figure 2.
Numerical models and laboratory experiments on site-specific rocks have emphasized the importance of crustal contamination/assimilation processes at mid-crustal depth (e.g., Iacono-Marziano et al., 2008; Iacono-Marziano et al., 2009; Pichavant et al., 2014), in agreement with the geology of the Vesuvius substratum, that comprises: 1) about 10 km of Mesozoic carbonates above the Paleozoic crystalline basement (e.g., Berrino et al., 1998; Brocchini et al., 2001; Improta and Corciulo, 2006; Di Renzo et al., 2007); 2) a possibly large (400
Supporting observations to this conjecture include the following points. 1) The occurrence of effective interactions between magma and rocks hosting the magma chamber, as testified by the mineralogical and geochemical characterization of cognate xenoliths and skarn fragments ejected during the different eruptions of the volcano (e.g., Barberi and Leoni, 1980; Belkin et al., 1985; Cioni et al., 1995; Fulignati et al., 1998; Gilg et al., 2001; Pascal et al., 2009; Stoppa et al., 2017). 2) The possibility that magma contamination by carbonate rocks widely occurs in the plumbing system of the volcano, as corroborated by Sr systematics (e.g., Civetta et al., 1991; Piochi et al., 2006), and quantitatively modelled by several authors (e.g., Pappalardo et al., 2004; Pappalardo and Mastrolorenzo, 2010). 3) The emission of large amounts of
However, other studies questioned the thermodynamic and geochemical grounds of conceptual models that consider extensive (i.e., >10%) country-rock assimilation processes active in the roots of Vesuvius (e.g., Bailey, 2005; Woolley et al., 2005; Bell and Kjarsgaard, 2006; Stoppa et al., 2017). Overall, despite the large amount of geoscientific data now available, there is no agreement on the actual effectiveness of carbonate assimilation, and whether the crustal component was involved at the time of melting of the source, or subsequently during the ascent of magma, or in what proportions the two processes can possibly overlap at Vesuvius.
In this complex picture, the main result of our work is to highlight the striking constancy of the He isotope signal of fluid/melt inclusions, and its close similarity to the composition of present-day gaseous emanations. This temporal stability points to a common deep source beneath the volcano, and to a mechanism of compositional homogenization that has the potential to smooth variations possibly related to the ascent of new magma batches. In the frame of the currently accepted multi-depth magma chamber (MDMC) model, this entails that magma ageing and interaction with crustal rocks could be effective in lowering the
The carbon-isotope composition of
The positive
Conclusion
With the aim of shedding light on the precursory path of a possible future reactivation of Vesuvius, we investigated helium isotope abundances in gases extracted by crushing from melt and fluid inclusions of volcanic products of different type and age, and we compared them with present-day fumarolic discharges.
For the first time, we analyzed pyroxene, olivine and sanidine crystals from both lavas and tephra coming from Plinian and inter-Plinian eruptions. In particular, we focused on volcanic products emitted after long periods of permanence in a magmatic chamber under closed conduit condition, by paying special attention to 79 AD Plinian eruption.
We measured
We related this homogenization mechanism to the existence of a deep-seated (about 10 km b.g.l.), extended magma reservoir filled with magma rising from the mantle. This reservoir directly fed lava flows emitted from flank vents during the Medieval Period, and the overlying magma chambers that produced mixed and explosive eruptions during other phases of Vesuvius history, through the ascent of pulses of mafic magma. Our data indicate that, independently of the effectiveness of carbonate assimilation processes, minor variations in
Overall, our data emphasize the fundamental role of the multiple-chamber structure in the magma dynamics of the Vesuvius volcanic system, and suggest that magma ponding at crustal depth could be considered a key mechanism that might have the potential to homogenize the helium isotope signal. Should this hypothesis be confirmed, the accuracy of future eruption forecasting through monitoring of
Data availability statement
The original contributions presented in the study are included in the article/Supplementary Material, further inquiries can be directed to the corresponding author.
Author contributions
FG: Noble gas analysis, data interpretation, writing of the original draft, review and editing; MB: Mineral separation, noble gas analysis, data interpretation, review and editing; CP: Field work, data interpretation, review and editing; GM: Conceptualization, methodology, noble gas analysis, data interpretation, writing the original draft, review and editing.
Acknowledgments
We warmly acknowledge the three reviewers for their constructive criticisms.
Conflict of interest
The authors declare that the research was conducted in the absence of any commercial or financial relationships that could be construed as a potential conflict of interest.
Publisher’s note
All claims expressed in this article are solely those of the authors and do not necessarily represent those of their affiliated organizations, or those of the publisher, the editors and the reviewers. Any product that may be evaluated in this article, or claim that may be made by its manufacturer, is not guaranteed or endorsed by the publisher.
Supplementary material
The Supplementary Material for this article can be found online at: https://www.frontiersin.org/articles/10.3389/feart.2022.1011203/full#supplementary-material
References
Arnò, V., Principe, C., Rosi, M., Santacroce, R., Sbrana, A., and Sheridan, M. F. (1987). “Eruptive history,” in Somma-vesuvius. Editor R. Santacroce (Roma: CNR Edizioni), Vol. 114, 53–104.8
Arrighi, S., Principe, C., and Rosi, M. (2001). Violent strombolian and subplinian eruption at Vesuvius during the post-1631 activity. Bull. Volcanol. 63, 126–150.
Auger, E., Gasparini, P., Virieux, J., and Zollo, A. (2001). Seismic evidence of an extended magmatic sill under Mt. Vesuvius. Science 294, 1510–1512. doi:10.1126/science.1064893
Avanzinelli, R., Casalini, M., Elliott, T., and Conticelli, S. (2018). Carbon fluxes from subducted carbonates revealed by uranium excess at Mount Vesuvius, Italy. Geology 46, 259–262. doi:10.1130/g39766.1
Ayuso, R. A., De Vivo, B., Rolandi, G., and Seal, R. R. (1998). Seal II R., Paone AGeochemical and isotopic (Nd-Pb-Sr-O) variations bearing on the Genesis of volcanic rocks from Vesuvius, Italy. J. Volcanol. Geotherm. Res. 82, 53–78. doi:10.1016/s0377-0273(97)00057-7
Bailey, D. K. (2005). Carbonate volcanics in Italy: Numerical tests for the hypothesis of lava-sedimentary limestone mixing. Period. Mineral. 74 (3), 205–208.
Barberi, F., Corrado, G., Innocenti, F., and Luongo, G. (1984). Phlegraean fields 1982–1984: Brief chronicle of a volcano emergency in a densely populated area. Bull. Volcanol. 47, 175–185. doi:10.1007/bf01961547
Barberi, F., and Leoni, L. (1980). Metamorphic carbonate ejecta from vesuvius plinian eruptions: Evidence of the occurrence of shallow magma chambers. Bull. Volcanol. 43, 107–120. doi:10.1007/bf02597615
Baubron, J.-C., Allard, P., Sabroux, J.-C., Tedesco, D., and Toutain, J.-P. (1991). Soil gas emanations as precursory indicators of volcanic eruptions. J. Geol. Soc. Lond. 148, 571–576. doi:10.1144/gsjgs.148.3.0571
Belkin, H. E., and De Vivo, B. (1993). Fluid inclusion studies of ejected nodules from plinian eruptions of Mt. Somma-Vesuvius. J. Volcanol. Geotherm. Res. 58, 89–100. doi:10.1016/0377-0273(93)90103-x
Belkin, H. E., De Vivo, B., Roedder, E., and Cortini, M. (1985). Fluid inclusion geobarometry from ejected Mt. Somma-vesuvius nodules. Am. Mineral. 70, 288–303.
Bell, K., and Kjarsgaard, B. (2006). Discussion of Peccerillo (2004) “carbonate-rich pyroclastic rocks from central apennines: Carbonatites or carbonate-rich rocks?” Period. Mineral. 75 (1), 85–92.
Berrino, G., Corrado, G., and Riccardi, U. (1998). Sea gravity data in the gulf of naples: A contribution to delineating the structural pattern of the vesuvian area. J. Volcanol. Geotherm. Res. 82, 139–150. doi:10.1016/s0377-0273(97)00061-9
Bertagnini, A., Landi, P., Rosi, M., and Vigliargio, A. (1998). The Pomici di Base plinian eruption of Somma-Vesuvius. J. Volcanol. Geotherm. Res. 83, 219–239. doi:10.1016/s0377-0273(98)00025-0
Brocchini, D., Principe, C., Castradori, D., Laurenzi, M., and Gorla, L. (2001). Quaternary evolution of the southern sector of the campanian plain and early somma–vesuvius activity: Insights from the trecase 1 well. Mineral. Pet. 73, 67–91. doi:10.1007/s007100170011
Chiodini, G., Cioni, R., Magro, G., Marini, L., Panichi, C., Raco, B., et al. (1996). Chemical and isotopic variations of bocca grande fumarole (solfatara volcano, phlegrean fields). Acta Vulcanol. 8, 228–232.
Chiodini, G., Marini, L., and Russo, M. (2001). Geochemical evidence for the existence of high-temperature hydrothermal brines at Vesuvio volcano, Italy. Geochim. Cosmochim. Acta 65, 2129–2147. doi:10.1016/s0016-7037(01)00583-x
Cioni, R., Civetta, L., Marianelli, P., Metrich, N., Santacroce, R., and Sbrana, A. (1995). Compositional layering and syn-eruptive mixing of a periodically refilled shallow magma chamber: The AD 79 plinian eruption of vesuvius. J. Petrology 36, 739–776. doi:10.1093/petrology/36.3.739
Cioni, R., D’Oriano, C., and Bertagnini, A. (2008). Fingerprinting ash deposits of small scale eruptions by their physical and textural features. J. Volcanol. Geotherm. Res. 177, 277–287. doi:10.1016/j.jvolgeores.2008.06.003
Cioni, R., Sulpizio, R., and Garruccio, N. (2003). Variability of the eruption dynamics during a subplinian event: The greenish pumice eruption of somma-vesuvius (Italy). J. Volcanol. Geotherm. Res. 124, 89–114. doi:10.1016/s0377-0273(03)00070-2
Civetta, L., Galati, R., and Santacroce, R. (1991). Magma mixing and convective compositional layering within the Vesuvius magma chamber. Bull. Volcanol. 53, 287–300. doi:10.1007/bf00414525
De Natale, G., Troise, C., Pingue, F., Mastrolorenzo, G., and Pappalardo, L. (2006). The Somma–Vesuvius volcano (southern Italy): Structure, dynamics and hazard evaluation. Earth. Sci. Rev. 74, 73–111. doi:10.1016/j.earscirev.2005.08.001
De Vivo, B., Petrosino, P., Lima, A., Rolandi, G., and Belkin, H. E. (2010). Research progress in volcanology in the neapolitan area, southern Italy: A review and some alternative views. Mineral. Pet. 99, 1–28. doi:10.1007/s00710-009-0098-6
B. De Vivo, R. Scandone, and R. R. Trigila (Editors) (1993). Mount Vesuvius: Special issue of J. Volcanol. Geotherm. Res., Vol. 58, 1–381.
Di Renzo, V., Di Vito, M. A., Arienzo, I., Carandente, A., Civetta, L., D'Antonio, M., et al. (2007). Magmatic history of Somma–Vesuvius on the basis of new geochemical and isotopic data from a deep borehole (Camaldoli della Torre). J. Petrology 48 (4), 753–784. doi:10.1093/petrology/egl081
Federico, C., Aiuppa, A., Allard, P., Bellomo, S., Jean-Baptiste, P., Parello, F., et al. (2002). Magma-derived gas influx and water-rock interactions in the volcanic aquifer of Mt. Vesuvius, Italy. Geochim. Cosmochim. Acta 66, 963–981. doi:10.1016/s0016-7037(01)00813-4
Frondini, F., Chiodini, G., Caliro, S., Cardellini, S., Granieri, D., and Ventura, G. (2004). Diffuse CO2 soil degassing at Vesuvio, Italy. Bull. Volcanol. 66, 642–651. doi:10.1007/s00445-004-0346-x
Fulignati, P., Gioncada, A., and Sbrana, A. (1998). The 79AD vesuvius magma chamber: A SEM-EDS study of doughter minerals in hypersaline fluid inclusions from cognate syenites. N. Jb. Mineral. Abh. 9, 403–416.
Fulignati, P., Panichi, C., Sbrana, A., Caliro, S., Gioncada, A., and Del Moro, A. (2005). Skarn formation at the walls of the 79AD magma chamber of Vesuvius (Italy): Mineralogical and isotopic constraints. njma. 181, 53–66. doi:10.1127/0077-7757/2005/0181-0007
Gasperini, D., Blichert-Toft, J., Bosch, D., Del Moro, A., Macera, P., and Albarède, F. (2002). Upwelling of deep mantle material through a plate window: Evidence from the geochemistry of Italian basaltic volcanics. J. Geophys. Res. 107 (B12), ECV 7-1–ECV 7-19. doi:10.1029/2001jb000418
Gilg, H. A., Lima, A., Somma, R., Belkin, H. E., De Vivo, B., and Ayuso, R. A. (2001). Isotope geochemistry and fluid inclusion study of skarns from Vesuvius. Mineral. Pet. 73, 145–176. doi:10.1007/s007100170015
Graham, D. W., Allard, P., Kilburn, C. R. J., Spera, F. J., and Lupton, J. E. (1993). Helium isotopes in some historical lavas from Mount Vesuvius. J. Volcanol. Geotherm. Res. 58, 359–366. doi:10.1016/0377-0273(93)90117-a
Graham, D. W., and Lupton, J. E. (1999). Helium isotopes in historical lavas from Mount Vesuvius. Earth Planet. Sci. Lett. 174, 241–244. doi:10.1016/s0012-821x(99)00268-x
Hilton, D. R., Barling, J., and Wheller, G. E. (1995). Effect of shallow-level contamination on the helium isotope systematics of ocean-island lavas. Nature 373, 330–333.
Hilton, D. R., Fischer, T. P., and Marty, B. (2002). “Noble gases and volatile recycling at subduction zones,” in Noble gases in geochemistry and cosmochemistry. Editors D. P. Porcelli, C. J. Ballentine, and R. Wieler (Washington, DC: Reviews in Mineralogy and Geochemistry), Vol. 47, 319–370.
Hilton, D. R., Hammerschmidt, K., Loock, G., and Friedrichsen, H. (1993a). Helium and argon isotope systematics of the central Lau Basin and Valu Fa Ridge: Evidence of crust/mantle interactions in a back-arc basin. Geochim. Cosmochim. Acta 57, 2819–2841. doi:10.1016/0016-7037(93)90392-a
Hilton, D. R., Hammerschmidt, K., Teufel, S., and Friedrichsen, H. (1993b). Helium isotope characteristics of Andean geothermal fluids and lavas. Earth Planet. Sci. Lett. 120, 265–282. doi:10.1016/0012-821x(93)90244-4
Iacono-Marziano, G., Gaillard, F., and Pichavant, M. (2008). Limestone assimilation by basaltic magmas: An experimental re-assessment and application to Italian volcanoes. Contrib. Mineral. Pet. 155, 719–738. doi:10.1007/s00410-007-0267-8
Iacono-Marziano, G., Gaillard, F., Scaillet, B., Pichavant, M., and Chiodini, G. (2009). Role of non-mantle CO2 in the dynamics of volcano degassing: The Mount Vesuvius example. Geology 37, 319–322. doi:10.1130/g25446a.1
Improta, L., and Corciulo, M. (2006). Controlled source nonlinear tomography: A powerful tool to constrain tectonic models of the southern apennines orogenic wedge, Italy. Geol. 34, 941–944. doi:10.1130/g22676a.1
Joron, J. L., Metrich, N., Rosi, M., Santocroce, R., and Sbrana, A. (1987). “Chemistry and petrography,” in Somma-vesuvius. Editor R. Santacroce (Rome, Italy: CNR, Quaderni De La Ricerca Scientifica), Vol. 114, 105–174.
Kurz, M. D., JenkinsSchilling, W. J, J. G., and Hart, S. R. (1982). Helium isotopic variations in the mantle beneath the central North Atlantic Ocean. Earth Planet. Sci. Lett. 58, 1–14. doi:10.1016/0012-821x(82)90099-1
Macdonald, R., Baginski, B., Rolandi, G., De Vivo, B., and Kopczynska, A. (2016). Petrology of parasitic and eccentric cones on the flanks and base of Somma-Vesuvius. Min. Pet. 110, 65–85. doi:10.1007/s00710-015-0410-6
Magro, G., Ruggieri, G., Gianelli, G., Bellani, S., and Scandiffio, G. (2003). Helium isotopes in paleofluids and present-day fluids of the Larderello geothermal field: Constraints on the heat source. J. Geophys. Res. 108, ECV 3-1–ECV 3-12. doi:10.1029/2001jb001590
Mamyrin, B. A., and Tolstikhin, I. N. (1984). Helium isotopes in nature. Amsterdam, Netherlands: Elsevier, 273.
Martelli, M., Nuccio, P. M., Stuart, F. M., Burgess, R., Ellam, R. M., and Italiano, F. (2004). Helium–strontium isotope constraints on mantle evolution beneath the Roman Comagmatic Province, Italy. Earth Planet. Sci. Lett. 224, 295–308. doi:10.1016/j.epsl.2004.05.025
Martelli, M., Nuccio, P. M., Stuart, F. M., Di Liberto, V., and Ellam, R. M. (2008). Constraints on mantle source and interactions from He-Sr isotope variation in Italian Plio-Quaternary volcanism. Geochem. Geophys. Geosyst. 9, Q02001. doi:10.1029/2007GC001730
Marty, B., Trull, T., Lussiez, P., Basile, I., and Tanguy, J. C. (1994). He, Ar, O, Sr and Nd isotope constraints on the origin and evolution of Mount Etna magmatism. Earth Planet. Sci. Lett. 126, 23–39. doi:10.1016/0012-821x(94)90240-2
Mele, D., Sulpizio, R., Dellino, P. F., and La Volpe, L. (2011). Stratigraphy and eruptive dynamics of a pulsating plinian eruption of somma-vesuvius: The Pomici di Mercato (8900 years B.P.). Bull. Volcanol. 73, 257–278. doi:10.1007/s00445-010-0407-2
Montone, P., Amato, A., and Pondrelli, S. (1999). Active stress map of Italy. J. Geophys. Res. 104, 25595–25610. doi:10.1029/1999jb900181
Morgan, D. J., Blake, S., Rogers, N. W., DeVivo, B., Rolandi, G., Macdonald, R., et al. (2004). Time scales of crystal residence and magma chamber volume from modelling of diffusion profiles in phenocrysts: Vesuvius 1944. Earth Planet. Sci. Lett. 222, 933–946. doi:10.1016/j.epsl.2004.03.030
Ozima, M., and Podosek, F. A. (1983). Noble gas geochemistry. Cambridge: Cambridge University Press.
Paolillo, A., Principe, C., Bisson, M., Gianardi, R., Giordano, D., and La Felice, S. (2016). Volcanology of the southwestern sector of Vesuvius volcano, Italy. J. Maps 12, 425–440. doi:10.1080/17445647.2016.1234982
Pappalardo, L., and Mastrolorenzo, G. (2010). Short residence times for alkaline Vesuvius magmas in a multi-depth supply system: Evidence from geochemical and textural studies. Earth Planet. Sci. Lett. 296, 133–143. doi:10.1016/j.epsl.2010.05.010
Pappalardo, L., Piochi, M., and Mastrolorenzo, G. (2004). The 3800 yr BP–1944 AD magma plumbing system of somma-vesuvius: Constraints on its behaviour and present satte through a review of isotope data. Ann. Geophys. 47, 1363–1375.
Parello, F., Allard, P., D’Alessandro, W., Federico, C., Jean-Baptiste, P., and Catani, O. (2000). Isotope geochemistry of Pantelleria volcanic fluids, sicily channel rift: A mantle volatile end-member for volcanism in southern europe. Earth Planet. Sci. Lett. 180, 325–339. doi:10.1016/s0012-821x(00)00183-7
Pascal, M. L., Di Muro, A., Fonteilles, M., and Principe, C. (2009). Zirconolite and calzirtite in banded fosterite-spinel-calcite skarn ejecta from vesuvius 1631 eruption: Inferences for the magma-wallrock interactions. Mineral. Mag. 73 (1), 149–172.
Peccerillo, A. (2020). “Campania volcanoes: Petrology, geochemistry, and geodynamic significance.” in Vesuvius, campi flegrei, and campanian volcanism. Editor B. De Vivo, H. E. Belkin, and G. Rolandi (Amsterdam, Netherlands: Elsevier), 79–120.
Pichavant, M., Scaillet, B., Pommier, A., Iacono-Marziano, G., and Cioni, R. (2014). Nature and evolution of primitive vesuvius magmas: An experimental study. J. Petrology 55, 2281–2310. doi:10.1093/petrology/egu057
Pineau, F., Shilobreeva, S., Hekinian, R., Bideau, D., and Javoy, M. (2004). deep-sea explosive activity on the mid-atlantic ridge near 34°50′N: A stable isotope (C, H, O) study. Chem. Geol. 211, 159–175. doi:10.1016/j.chemgeo.2004.06.029
Piochi, M., Ayuso, R. A., De Vivo, B., and Somma, R. (2006). Crustal contamination and crystal entrapment during polybaric magma evolution at Mt. Somma–Vesuvius volcano, Italy: Geochemical and Sr isotope evidence. Lithos 86, 303–329. doi:10.1016/j.lithos.2005.05.009
Porcelli, D., Ballentine, C. J., and Wieler, R. (2002). An overview of noble gas geochemistry and cosmochemistry. Rev. Mineralogy Geochem. 47, 1–19. doi:10.2138/rmg.2002.47.1
Poreda, R., and Craig, H. (1989). Helium isotope ratios in circum-Pacific volcanic arcs. Nature 338, 473–478. doi:10.1038/338473a0
Principe, C., Paolillo, A., La Felice, S., and Arrighi, S. (2021). “Forma vesuvii- 2 volcanic morphology at the time of the 79AD plinian eruption,” in Physis. Editor L. Olschki, 289–303. Firenze. 2021/1-2 (LVI).
Principe, C., Tanguy, J. C., Arrighi, S., Paiotti, A., Le Goff, M., and Zoppi, U. (2004). Chronology of Vesuvius’ activity from AD 79 to 1631 based on archeomagnetism of lavas and historical sources. Bull. Volcanol. 66, 703–724. doi:10.1007/s00445-004-0348-8
Ricco, C., Petrosino, S., Aquino, I., Cusano, P., and Madonia, P. (2021). Tracking the recent dynamics of Mt. Vesuvius from joint investigations of ground deformation, seismicity and geofluid circulation. Sci. Rep. 11, 965. doi:10.1038/s41598-020-79636-w
M. Rosi, and A. Sbrana (Editors) (1987). Phlegraean fields (Roma: Collana Quaderni della Ricerca Scientifica, Consiglio Nazionale delle Ricerche), 175.
Sano, Y., Wakita, H., Italiano, F., and Nuccio, M. P. (1989). Helium isotopes and tectonics in southern Italy. Geophys. Res. Lett. 16, 511–514. doi:10.1029/gl016i006p00511
Scaillet, B., Pichavant, M., and Cioni, R. (2008). Upward migration of Vesuvius magma chamber over the past 20, 000 years. Nature 455, 216–219. doi:10.1038/nature07232
Scandone, P. (1979). Origin of the Tyrrhenian Sea and calabrian arc. Boll. Soc. Geol. It. 98, 27–34.
Scheibner, B., Heumann, A., and Wörner, C. L. (2008). Crustal residence times of explosive phonolite magmas: U–Th ages of magmatic Ca-garnets of Mt. Somma-vesuvius (Italy). Earth Planet. Sci. Lett. 276, 293–301. doi:10.1016/j.epsl.2008.09.028
Shaw, A. M., Hilton, D. R., Fisher, T. P., Walker, J. A., and de Leew, G. A. M. (2006). Helium isotope variations in mineral separates from Costa Rica and Nicaragua: Assessing crustal contributions, timescale variations and diffusion-related mechanisms. Chem. Geol. 230, 124–139. doi:10.1016/j.chemgeo.2005.12.003
Stoppa, F., Principe, C., Schiazza, M., Liu, Y., Giosa, P., and Crocetti, S. (2017). Magma evolution inside the 1631 Vesuvius magma chamber and eruption triggering. Open Geosci. 9, 24–52. doi:10.1515/geo-2017-0003
Tedesco, D., Allard, P., Sano, Y., Wakita, H., and Pece, R. (1990). Helium-3 in subaerial and submarine fumaroles of Campi Flegrei caldera, Italy. Geochim. Cosmochim. Acta 54, 1105–1116. doi:10.1016/0016-7037(90)90442-n
Tedesco, D. (1997). Systematic variations in the 3He/4He ratio and carbon fumarolic fluids from active volcanic areas in Italy: Evidence for radiogenic 4He and crustal carbon addition by the subducting African plate? Earth and Planetary Science Letters 151, 255–269.
Tedesco, D., and Scarsi, P. (1999). Chemical (He, H2, CH4, Ne, Ar, N2) and isotopic (He, Ne, Ar, C) variations at the solfatara crater (southern Italy): Mixing of different sources in relation to seismic activity. Earth Planet. Sci. Lett. 171, 465–480. doi:10.1016/s0012-821x(99)00137-5
Trull, T. W., and Kurz, M. D. (1993). Diffusivity of 3He and 4He in olivine and clinopyroxene at magmatic and mantle temperatures. Geochim. Cosmochim. Acta 57, 1313–1324. doi:10.1016/0016-7037(93)90068-8
Turco, E., Schettino, A., Pierantoni, P. P., and Santarelli, G. (2006). “The Pleistocene extension of the Campania plain in the framework of the southern tyrrhenian tectonic evolution: Morphotectonic analysis, kinematic model and implications for volcanism,” in Developments in volcanology. Developments in volcanology. Editor B. De Vivo, Vol. 9, 27–51.
Valley, J. W. (1986). “Stable isotope geochemistry of metamorphic rocks,” in Stable isotopes in high temperature geological processes. Reviews in mineralogy. Editors J. W. Valley, H. P. TaylorJr., and J. R. O’Neil, Vol. 16, 445–489.
Keywords: Helium isotopes, Carbon isotopes, melt and fluid inclusions, Plinian eruptions, Sub-Plinian eruptions, Violent Strombolian eruptions, flank eruptions, Vesuvius
Citation: Gherardi F, Barsanti M, Principe C and Magro G (2022) Helium isotopes in Plinian and inter-Plinian volcanic products of Vesuvius, Italy. Front. Earth Sci. 10:1011203. doi: 10.3389/feart.2022.1011203
Received: 03 August 2022; Accepted: 21 October 2022;
Published: 07 November 2022.
Edited by:
Ying Li, Institute of Earthquake Forecasting, China Earthquake Administration, ChinaReviewed by:
David Graham, Oregon State University, United StatesIan Ernest Masterman Smith, The University of Auckland, New Zealand
Eemu Ranta, Faculty of Science, University of Helsinki, Finland
Copyright © 2022 Gherardi, Barsanti, Principe and Magro. This is an open-access article distributed under the terms of the Creative Commons Attribution License (CC BY). The use, distribution or reproduction in other forums is permitted, provided the original author(s) and the copyright owner(s) are credited and that the original publication in this journal is cited, in accordance with accepted academic practice. No use, distribution or reproduction is permitted which does not comply with these terms.
*Correspondence: Fabrizio Gherardi, f.gherardi@igg.cnr.it