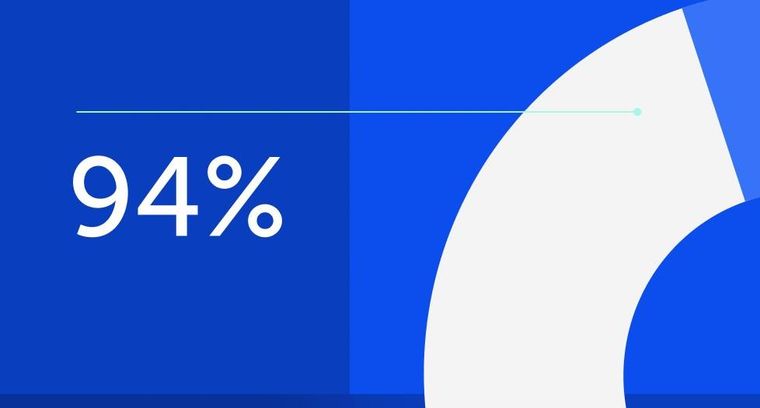
94% of researchers rate our articles as excellent or good
Learn more about the work of our research integrity team to safeguard the quality of each article we publish.
Find out more
OPINION article
Front. Earth Sci., 21 September 2022
Sec. Geochemistry
Volume 10 - 2022 | https://doi.org/10.3389/feart.2022.1007620
This article is part of the Research TopicMechanisms and Applications of Chemical Techniques for Effective Development of Unconventional ReservoirsView all 13 articles
Hydraulic fracturing was first developed in North America, resulting in a multi-stage horizontal well and multi-layer vertical well volume fracturing technology, and has been commonly used in reservoir reconstruction. However, hydraulic fracturing will cause a lot of waste of water resources, and the treatment of flowback fluids requires high cost (Wang et al., 2012; Lu et al., 2016; Rao et al., 2021). Supercritical CO2 has attracted much attention due to its high mobility and low intermolecular interaction. Using CO2 fracturing can effectively avoid the above problems by show more effectively increase the reservoir pressure and reduce the damage to the reservoir (King, 1983; Bryant and Monger, 1988; Yost et al., 1993; Xie and Hou, 2009). However, CO2 fracturing needs a large number of supercritical CO2, which is expensive and not easily accessible.
Since the first application of CO2 fracturing technology in the United States in the 1980s, CO2 fracturing technology has entered a stage of rapid development (Gupta and Bobier, 1998; Wei et al., 2019; Chen et al., 2020). A large number of CO2 pre-injection experiments during hydraulic fracturing have been carried out, and field applications have been carried out in oilfields, which have achieved good results in increasing production (Liu et al., 2014; Zhou et al., 2019). In 1990, CO2 sand fracturing, N2 foam fracturing and N2 fracturing were carried out in 15 gas wells in Kentucky, United States. The production data show that the cumulative gas production of CO2 sand fracturing wells in 37 months is 2 times that of N2 fracturing wells and 5 times that of N2 foam fracturing wells. In 2014, an oil well in Oklahoma was fractured by CO2. The daily oil production after fracturing was 2.7 t, and the oil production increased to 3.3 t/d after 1 month. CO2 fracturing technology has been applied in 25 wells of tight oil reservoir, and the average daily production of single well is 1.7 t higher than that of adjacent wells. But up to now, there is still a lack of discussion on the mechanisms of CO2 pre-injection to increase reservoir energy and production. Therefore, this paper aimed at investigating the interaction between pre-injected CO2 and the reservoir fluid/rock/energy, providing reference for further confirming the mechanism of enhancing production and improving the fracturing effect.
The study on the interaction of injected CO2 with reservoir rocks and fluids is of great significance for fracturing and reservoir reconstruction using CO2. The influence of injected CO2 on reservoirs is mainly reflected in four aspects, namely, improving physical properties of matrix, influence on fluid, improving stimulation effect and increasing reservoir pressure.
The improvement of reservoir properties by CO2 is mainly reflected in the influence of porosity and permeability of rock, the change of reservoir wettability, plugging removal, and inhibition of clay expansion.
On the one hand, when the CO2 dissolution mechanism dominates, the porosity and permeability of rock will increase with the injection of CO2. When rocks and reservoir water contact with CO2, CO2 aqueous solution will produce new pores or broaden primary pores in the dissolution of organic matter or minerals, and the dissolution will increase with the increase of temperature and immersion time (Ross et al., 1981; Pokrovsky et al., 2005). Zou et al. (2021) conducted an experiment on the change of pore structure of shale reservoirs after immersion in CO2 aqueous solution for 24 h under simulated reservoir temperature/pressure conditions (80°C, 20 MPa), and the rock matrix sample was soaked for 24 h. A large number of dissolved pores appeared, the porosity was increased by 6.9%, and the permeability was increased from 0.23
On the other hand, when CO2 adsorption expansion is dominant, CO2 injection will reduce rock porosity and permeability. The interaction of CO2, brine, and rock will form mineral crystals, which grow and precipitate in pores (Xu et al., 2005; Lahann et al., 2013). An experimental study by Kumar et al. (2015) showed that the adsorption of CO2 in micropores may cause adsorption-induced swelling, thereby closing existing natural fractures and reducing fluid flow capacity. The change of pore structure is significantly affected by CO2, and it is necessary to conduct targeted research on shale in practical applications.
CO2 can change reservoir wettability (Chiquet et al., 2005; Zhang et al., 2018). The injection of CO2 will form carbonate in the reservoir, and the acid reacts with the minerals in the reservoir to generate new minerals, thereby changing the wettability of the solid wall of the liquid phase. The strong hydrophilicity of the reservoir is conducive to improving the injectivity of subsequent water flooding, and thus improving the recovery efficiency. Yao et al. (2017) proved that after injection of CO2 into the reservoir, the wetting contact angle decreased and the hydrophilicity of the reservoir increased.
In shale reservoirs, the reduction of the pH value of reservoir water can inhibit the expansion of clay. In carbonatite and sandstone, partial plugging can be relieved to restore oil well production. In the experiments by Zhang et al. (2020), the aqueous solution was slightly acidic owing to CO2 dissolution, and the formation water dissolved CO2 and interacted with the formation matrix to relieve plugging and inhibit clay swelling.
The influence of CO2 on fluid is mainly reflected in the influence of extraction rate, density, expansion, gas solubility, surface tension, and irreducible water saturation.
CO2 enhances the extraction capacity of crude oil (Ding et al., 2019). It is difficult for CO2 to get miscible with crude oil at first contact, so pre-injection of CO2 can achieve multi-contact with oil, and the extraction effect of CO2 on crude oil is continuously enriched to realize the miscibility. CO2 has strong extraction ability for C2-C5 components of crude oil, but weak extraction ability for heavy components and methane. Liu et al. (2021) determined the extraction rate of crude oil by CO2 at different pressures. Results show that CO2 density was positively correlated with pressure. When the pressure reaches 40 MPa, the extraction rate can reach 85.2%. The experiment showed that CO2 significantly enhanced the extraction rate of crude oil, as shown in Figure 1A.
Dissolving CO2 in crude oil will increase the density of crude oil. With the increase of CO2 content, more supercritical CO2 contacts with crude oil, and the density of crude oil increases with the increase of CO2 content (Abedini and Torabi, 2014). Su et al. (2021) injected 45% mole fraction of CO2 into crude oil in the oil expansion experiment, and the oil density was increased by 8.78% from 0.7341 to 0.7986 g/cm3 at 20 MPa.
CO2 has a high expansion effect on crude oil. The specific volume, reservoir volume factor and compressibility coefficient of crude oil increase after CO2 injection, which increases the compressibility of crude oil and further improves the productivity of oil wells (Nobakht et al., 2008), as shown in Figure 1B. Su et al. (2021) conducted crude oil expansion experiment, and found that when the mole fraction of CO2 in crude oil was increased from 0 to 0.45%, the specific volume, reservoir volume factor, and compressibility coefficient of crude oil were increased by 2.53, 30.06, and 41.54%, respectively. Zhang et al. (2020) injected CO2 into crude oil, and the expansion coefficient of crude oil increased from 1.00 to 1.19 after adding 45% CO2. The above experiments have demonstrated that CO2 can significantly enhance the elastic energy of reservoir.
The injection of CO2 can effectively improve the gas solubility of crude oil. The higher the viscosity of crude oil is, the more obvious the viscosity reduction effect will be. Lower viscosity can increase its mobility, which is conducive to the production of crude oil. Shi and Zhao (2020) found that the average dissolved gas-oil ratio of the oil samples increased from 13.5 to 18.05, an increase of 4.52, accounting for 33.41% of the dissolved gas-oil ratio of total oil samples. The data show that CO2 injection into crude oil can effectively enhance the gas solubility of crude oil and improve the gas solubility of crude oil.
CO2 can reduce the oil-water interfacial tension and reduce the viscosity of crude oil. CO2 was injected into the reservoir in advance and CO2 was contacted with crude oil many times, which improved the physical properties of crude oil, enhanced the mobility of crude oil and finally reached the miscibility. This will greatly reduce crude oil viscosity, improve displacement efficiency and increase production. The high-pressure PVT experiments conducted by Yang et al. (2009) showed that when the oil-water mixed solution was saturated with CO2, the interfacial tension can be reduced by about 33%. Reducing the surface tension can reduce the adhesion work that needs to be overcome to strip oil from the rock surface, making the oil adhered to the rock surface and pores easier to be extracted.
Injecting CO2 increases irreducible water saturation (Liu et al., 2020). With the injection of CO2, the dissolved gas volume of reservoir water increase. Some of the irreducible water that occupies the oil flow channel becomes mobile water, which makes the oil flow out and thus improves the recovery efficiency. Zhao et al. (2011) conducted the reservoir water injection CO2 expansion experiment, and found that when the injection pressure was 27 MPa, irreducible water saturation increased from 36.3 to 41.11%.
Improving stimulated effect by CO2 is mainly reflected in reducing initiation pressure and increasing fracture complexity.
Supercritical CO2 can reduce the rupture pressure of rocks. Since supercritical CO2 has good diffusion and permeability, supercritical CO2 fracturing reduces the effective stress of surrounding rock by increasing pore pressure, which makes the initiation pressure lower than hydraulic fracturing (Tudor et al., 1994; Ito, 2008; Zou et al., 2018; Deng et al., 2022), as shown in Figure 1C. Ding et al. (2018) analyzed the fracturing mechanism of supercritical CO2 fracturing based on the rock fracture criterion of linear elastic model. The calculated data indicated that the rupture pressure by using supercritical CO2 was reduced by 75.5%. Wang et al. (2019) experimentally demonstrated that the initiation pressure of supercritical CO2 fracturing rock is 15% lower than that of liquid CO2 under the same conditions, which is about half of that of hydraulic fracturing.
Supercritical CO2 has the effect of slippage and diffusion, which makes its liquidity have certain nonlinear characteristics. CO2 can enter the tiny pores and fracture tips that water and fracturing fluid cannot enter during the propagation, promoting the opening of natural weak surface and increasing the complexity of fractures (Zou et al., 2018; Sheng et al., 2019; Tudor et al., 1994; Ito, 2008). Su et al. (2019) combined with physical simulation and numerical simulation, and found that the volume strain increment produced by supercritical CO2 fracturing is higher than that of hydraulic fracturing, the fracture conplexity and fracture surface roughness after fracturing are also larger, and the fracture morphology is more complex (Zhou et al., 2016).
Injection of CO2 can effectively increase reservoir pressure, which is conducive to production. CO2 has strong injection, good diffusion, strong production-increasing effect, wide range of pressure spread. Equal velocity injection of liquid carbon dioxide and water, the pressure-increasing effect of CO2 injection is twice that of water injection (Singh, 2018; Xiao, 2018). Zhang et al. (2020) found that with the increase of CO2 injection rate, the radius of miscible zone increased gradually. Due to the rapid propagation of CO2 pressure, the reservoir pressure increases rapidly, as shown in Figure 1D, and the pressure can be maintained above 29 MPa in the reservoir near the wellbore.
CO2 pre-injection during hydraulic fracturing can affect the physical properties of the reservoir, increase the porosity and permeability of the rock and improve the wettability of the reservoir, which is conducive to improving the subsequent water flooding injection capacity. CO2 injection enhances the flow capacity of crude oil by using the effect of CO2 injection on the fluid, making it easier for crude oil to be recovered. It also reduces initiation pressure, increases fracture complexity and increases formation pressure. Combined with water-based fracturing fluid, the fractures were further propagated and effectively supported.
At present, the mechanism of CO2 pre-injection during hydraulic fracturing on reservoir has not been comprehensive considered in the fracture propagation simulation and production dynamic simulation. In the future, all of the mechanism should be considered for the accuracy and efficiency of experimental and theory research.
CRediT author statement YY: Investigation and research, Conceptualization, Writing—Original draft preparation JL: Resources, Translation GS: Supervision, Modify analysis FD: Typesetting.
Supported by Open Fund of Hubei Key Laboratory of Drilling and Production Engineering for Oil and Gas (Yangtze University) (ID: YQZC202203).
The authors declare that the research was conducted in the absence of any commercial or financial relationships that could be construed as a potential conflict of interest.
All claims expressed in this article are solely those of the authors and do not necessarily represent those of their affiliated organizations, or those of the publisher, the editors and the reviewers. Any product that may be evaluated in this article, or claim that may be made by its manufacturer, is not guaranteed or endorsed by the publisher.
Abedini, A., and Torabi, F. (2014). On the CO2 storage potential of cyclic CO2 injection process for enhanced oil recovery. Fuel 124, 14–27. doi:10.1016/j.fuel.2014.01.084
Bryant, D. W., and Monger, T. G. (1988). Multiple-contact phase behavior measurement and application with mixtures of CO2 and highly asphaltic crude. SPE Reserv. Eng. 3 (02), 701–710. doi:10.2118/14438-pa
Chen, H., Liu, X., and Jia, N. (2020). Prospects and key scientific issues of CO2 near-mis cible flooding. Petroleum Sci. Bull. 03, 392–401. doi:10.3969/j.issn.2096-1693.2020.03.033
Chiquet, P., Broseta, D., and Thibeau, S. (2005). “Capillary alteration of shaly caprocks by carbon dioxide (SPE94183),” in 67th EAGE Conference & Exhibition, Madrid, Spain, June 13–16, 2005 (European Association of Geoscientists & Engineers). cp-1-00041.
Deng, H., Sheng, G., Zhao, H., Meng, F., Zhang, H., Ma, J., et al. (2022). Integrated optimization of fracture parameters for subdivision cutting fractured horizontal wells in shale oil reservoirs. J. Petroleum Sci. Eng. 212, 110205. doi:10.1016/j.petrol.2022.110205
Ding, M., Wang, Y., Han, Y., Gao, M., and Wang, R. (2019). Interactions in bypassed oil-CO2 systems and their utilization in enhancing the recovery of bypassed oil[J]. Fuel 237, 1068–1078. doi:10.1016/j.fuel.2018.10.019
Ding, Y., Ma, X., and Ye, L. (2018). Rock breaking mechanism of CO2 and fracturing technology. Qingdao, China: Elsevier BV.
Gupta, D. V. S., and Bobier, D. M. (1998). “The history and success of liquid CO2 and CO2/N2 fracturing system,” in SPE Gas Technology Symposium, Calgary, Alberta, Canada, March 15–18, 1998. SPE 40016: Society of Petroleum Engineers.
Ha, S. J., Choo, J., and Yun, T. S. (2018). Liquid CO2 fracturing: Effect of fluid permeation on the breakdown pressure and cracking behavior. Rock Mech. Rock Eng. 51 (11), 3407–3420. doi:10.1007/s00603-018-1542-x
Ito, T. (2008). Effect of pore pressure gradient on fracture initiation in fluid saturated porous media: Rock. Eng. Fract. Mech. 75 (7), 1753–1762. doi:10.1016/j.engfracmech.2007.03.028
King, S. R. (1983). “Liquid CO2 for the stimulation of low-permeability reservoirs,” in SPE/DOE Low Permeability Gas Reservoirs Symposium, Denver, Colorado, March 14–16, 1983. OnePetro.
Kumar, H., Elsworth, D., Liu, J., Pone, D., and Mathews, J. P. (2015). Permeability evolution of propped artificial fractures in coal on injection of CO2. J. Petroleum Sci. Eng. 133, 695–704. doi:10.1016/j.petrol.2015.07.008
Lahann, R., Mastalerz, M., Rupp, J. A., and Drobniak, A. (2013). Influence of CO2 on new albany shale composition and pore structure. Int. J. Coal Geol. 108, 2–9. doi:10.1016/j.coal.2011.05.004
Liu, H., Wang, F., Zhang, J., and Meng, S. (2014). Fracturing with carbon dioxide:Application and development trend. Petroleum Explor. Dev. 41 (4), 513–519. doi:10.1016/S1876-3804(14)60060-4
Liu, H., Zhu, Z., Patrick, W., Liu, J., Lei, H., and Zhang, L. (2020). Pore-scale numerical simulation of supercritical CO2 migration in porous and fractured media saturated with water. Adv. Geo-Energy Res. 4 (4), 419–434. doi:10.46690/ager.2020.04.07
Liu, J., Li, L., and Gao, M. (2021). Interaction between CO2 and crude oil in low permeability reservoirs. Oilfield Chem. 38 (03), 464–469. doi:10.19346/j.cnki.1000-4092.2021.03.015
Lu, J., Nicot, J. P., Mickler, P. J., Ribeiro, L. H., and Darvari, R. (2016). Alteration of bakken reservoir rock during CO2-based fracturing—an autoclave reaction experiment. J. Unconv. Oil Gas Resour. 14, 72–85. doi:10.1016/j.juogr.2016.03.002
Nobakht, M., Moghadam, S., and Gu, Y. (2008). Mutual interactions between crude oil and CO2 under different pressures. Fluid phase equilibria 265 (1-2), 94–103. doi:10.1016/j.fluid.2007.12.009
Pokrovsky, O. S., Golubev, S. V., and Schott, J. (2005). Dissolution kinetics of calcite, dolomite and magnesite at 25 C and 0 to 50 atm pCO2. Chem. Geol. 217 (3-4), 239–255. doi:10.1016/j.chemgeo.2004.12.012
Rao, X., Xu, Y., Liu, D., and Hu, Y. (2021). A general physics-based data-driven framework for numerical simulation and history matching of reservoirs. Adv. Geo-Energy Res. 5 (4), 422–436. doi:10.46690/ager.2021.04.07
Ross, G. D., Todd, A. C., and Tweedie, J. A. (1981). “The effect of simulated CO2 flooding on the permeability of reservoir rocks,” in Enhanced oil recovery (Amsterdam: Elsevier), 351–366.
Sheng, G., Su, Y., and Wang, W. (2019). A new fractal approach for describing induced-fracture porosity/permeability/compressibility in stimulated unconventional reservoirs. J. Petroleum Sci. Eng. 179, 855–866. doi:10.1016/j.petrol.2019.04.104
Shi, J., and Zhao, X. (2020). Effect of CO_2 on physical properties of crude oil. China Petroleum Chem. Stand. Qual. 40 (8), 117–118,120. doi:10.3969/j.issn.1673-4076.2020.08.056
Singh, H. (2018). Impact of four different CO2 injection schemes on extent of reservoir pressure and saturation. Adv. Geo-Energy Res. 2 (3), 305–318. doi:10.26804/ager.2018.03.08
Su, Y., Wang, C., and Li, L. (2021). Behavior of CO2 pre-fracturing fluid in tight reservoir. Sci. Technol. Eng. 21 (8), 3076–3081. doi:10.3969/j.issn.1671-1815.2021.08.014
Su, Z., Li, F., and Zhou, D. (2019). Hydraulic fracture propagation behavious and geometry under supercritical CO2 fracturing in shale reservoirs. Oil Gas Geol. 40 (3), 616–625. doi:10.11743/ogg20190316
Tudor, R., Vozniak, C., and Peters, W. (1994). “Technical advances in liquid CO2 fracturing,” in Annual Technical Meeting, Calgary, Alberta, June 11–14, 1994. OnePetro.
Wang, H., Li, G., and Shen, Z. (2012). A feasibility analysis on shale gas exploitation with supercritical carbon dioxide. Energy Sources, Part A Recovery, Util. Environ. Eff. 34 (15), 1426–1435. doi:10.1080/15567036.2010.529570
Wang, X., Sun, X., and Luo, P. (2019). Progress and application of CO2 fracturing technology for unconventional oil and gas[J]. Lithologic Reservoirs 31 (2), 1–7
Wei, Q., Hou, J., and Hao, H. (2019). Laboratory study of CO2 channeling characteristics in ultra-low-permeability oil reservoirs. Petroleum Sci. Bull. 02, 145–153. doi:10.3969/j.issn.2096-1693.2019.02.013
Xiao, S. (2018). Research on carbon dioxide flooding technology in Fu Yang oilfield. Daqing: Northeast Petroleum University.
Xie, P., Hou, G., and Han, J. (2009). Application of CO, fracturing technology in sulige gas field. Fault-Block Oil Gas Field 16 (5), 104–106.
Xu, T., Apps, J. A., and Pruess, K. (2005). Mineral sequestration of carbon dioxide in a sandstone–shale system. Chem. Geol. 217 (3-4), 295–318. doi:10.1016/j.chemgeo.2004.12.015
Yang, S., Hang, D., Sun, R., et al. (2009). CO2 extraction for crude oil and its effect on crude oil viscosity. J. Petroleum Sci. Eng. Nat. Sci. Ed. 33 (4), 85–88. doi:10.3321/j.issn:1673-5005.2009.04.016
Yao, Z., Huang, C., and Ma, Y. (2017). Physical property variation law of CO2 flooding reservoir, Yanchang Oilfield. Fault-block Oilfield 24 (1), 60–62. doi:10.6056/dkyqt201701014
Yost, A. B., Mazza, R. L., and Gehr, J. B. (1993). “CO2/Sand fracturing in devonian shales,” in SPE Eastern Regional Meeting, Pittsburgh, Pennsylvania, November 2–4, 1993. OnePetro.
Zhang, K., Bai, X., and Liu, S. (2020). Energy enhancement effect and parameters optimization of CO2 injection in tight oil reservoir. Sci. Technol. Eng. 20 (26), 10751–10758. doi:10.3969/j.issn.1671-1815.2020.26.029
Zhang, X., Wei, B., Shang, J., Gao, K., Pu, W., Xu, X., et al. (2018). Alterations of geochemical properties of a tight sandstone reservoir caused by supercritical CO2-brine-rock interactions in CO2-EOR and geosequestration. J. CO2 Util. 28, 408–418. doi:10.1016/j.jcou.2018.11.002
Zhao, M., Li, J., and Wang, Z. (2011). Study on mechanism of CO_2 immiscible flooding in ultra-low permeability reservoir [J]. Sci. Tech. Engg. 11 (7), 1438–1440
Zhou, J., Hu, N. A. N., Xian, X., Zhou, L., Tang, J., Kang, Y., et al. (2019). Supercritical CO2 fracking for enhanced shale gas recovery and CO2 sequestration: Results, status and future challenges. Adv. Geo-Energy Res. 3 (2), 207–224. doi:10.26804/ager.2019.02.10
Zhou, J., Liu, G., Jiang, Y., Xian, X., Liu, Q., Zhang, D., et al. (2016). Supercritical carbon dioxide fracturing in shale and the coupled effects on the permeability of fractured shale: An experimental study. J. Nat. Gas Sci. Eng. 36, 369–377. doi:10.1016/j.jngse.2016.10.005
Zou, Y., Li, S., Ma, X., Zhang, S., Li, N., and Chen, M. (2018). Effects of CO2–brine–rock interaction on porosity/permeability and mechanical properties during supercritical-CO2 fracturing in shale reservoirs. J. Nat. Gas Sci. Eng. 49, 157–168. doi:10.1016/j.jngse.2017.11.004
Keywords: hydraulic fracturing, CO2 pre-injection, reservoir pressure, stimulated reservoir volume, fracturing stimulation
Citation: Yang Y, Liu J, Sheng G, Du F and Zheng D (2022) Analysis of the influence of CO2 pre-injection during hydraulic fracturing on enhanced oil recovery in shale reservoirs. Front. Earth Sci. 10:1007620. doi: 10.3389/feart.2022.1007620
Received: 30 July 2022; Accepted: 02 September 2022;
Published: 21 September 2022.
Edited by:
Qingbang Meng, China University of Geosciences, ChinaReviewed by:
Zhiming Chen, China University of Petroleum, ChinaCopyright © 2022 Yang, Liu, Sheng, Du and Zheng. This is an open-access article distributed under the terms of the Creative Commons Attribution License (CC BY). The use, distribution or reproduction in other forums is permitted, provided the original author(s) and the copyright owner(s) are credited and that the original publication in this journal is cited, in accordance with accepted academic practice. No use, distribution or reproduction is permitted which does not comply with these terms.
*Correspondence: Jinghua Liu, bGl1amgyMDE5QHlhbmd0emV1LmVkdS5jbg==; Guanglong Sheng, c2hlbmdnbDIwMTlAeWFuZ3R6ZXUuZWR1LmNu
Disclaimer: All claims expressed in this article are solely those of the authors and do not necessarily represent those of their affiliated organizations, or those of the publisher, the editors and the reviewers. Any product that may be evaluated in this article or claim that may be made by its manufacturer is not guaranteed or endorsed by the publisher.
Research integrity at Frontiers
Learn more about the work of our research integrity team to safeguard the quality of each article we publish.