- 1University of Alabama, Tuscaloosa, AL, United States
- 2Key Laboratory of Watershed Geographic Sciences, Nanjing Institute of Geography and Limnology, Chinese Academy of Sciences, Nanjing, China
A major justification for building the Three Gorges Dam on the Changjiang is flood control. The dam can reduce peak discharge during severe floods. These floods, however, cannot be prevented by operation of the Three Gorges Dam alone. During the most severe floods abnormally high rainfall occurs throughout the Changjiang basin. The Three Gorges Dam can only affect discharge from the upper basin. Large tributaries on the middle Changjiang are unaffected by the dam. The cumulative impact of high discharge from large tributaries on the middle Changjiang increase flood severity with distance downstream. Also, Changjiang-lake interaction is affected by decreasing volume of Poyang and Dongting Lakes that occurred during the past few decades. These are the largest freshwater lakes in China. As a result of reduced floodwater storage capacity during summer high discharge periods, tributaries flow into the lakes with a higher base level than previously occurred. Instead of Changjiang back flowing into the lakes as previously expected during major floods, high Changjiang discharge occurs at the same time as high tributary discharge that increases peak river stage.
Introduction
Severe floods along the middle and lower Changjiang in southern China have been documented for centuries (Xu 1976; Sauer 1986; Min 1999). Extensive alluvial plains surrounding the Changjiang and its large tributaries flood most years during the summer months. During the most severe events floodwater can be meters above the surrounding plains. Levees have been used to protect against floods since large-scale settlement began. During major floods levees were over-topped or failed because of poor construction or maintenance resulting in extensive agricultural loss and many deaths (Duan et al., 2016). The frequency of extreme floods has increased during the past century (Min, 1999; Shankman et al., 2012). Since the 1950s, levees have greatly improved, but floods were progressively higher and catastrophic levee failures still occurred.
The Three Gorges Dam (TGD) spans the Changjiang at Yichang, Hubei Province.
The dam was designed for electric-power production, improved river navigation, and downstream flood control (see discussions by Edmonds 1992; Cheng 1997). Dam construction commenced 1994 and began pilot operation of 170 m in 2008. The Three Gorges Reservoir (TGR) extends upstream from the dam up to 600 km. Height of the dam is 180 m and increases water level at the downstream section of the (TGR) about 100 m when at maximum capacity. The reservoir is confined to the narrow width of the Three Gorges that averages 1.1 km. The reservoir stage is maintained at 175 m during winter months for electric power generation. The reservoir is lowered during spring to 145 m to increase floodwater storage capacity. This difference in water elevation creates a flood control capacity of 22.15 billion m3 (Zhang et al., 2017).
A severe flood occurred downstream on the middle Changjiang during 2020 when the dam was fully operational. The purpose of this paper is to examine the effects of the TGD on severe flood probability. This paper 1) reviews flood history of the middle Changjiang and discusses the influence of ENSO on flood events, 2) examines characteristics of the 2020 flood after the dam became operational, and 3) addresses how the dam’s reservoir capacity, tributary discharge, changes in sediment budget, and loss of floodwater storage will affect the probability of severe floods in the future.
Regional overview
Changjiang headwaters are in the rugged Tibetan and Qinghai Plateaus in western China. From there it flows eastward for 6,300 km before reaching the sea near Shanghai (Figure 1). The upper basin is mostly within Continental or Highland climate regions that are generally cold and dry. In contrast, the middle and lower sections of the drainage basin to the east have a humid subtropical climate with hot summers, long growing-season, and high annual precipitation. The rainy season in southern China begins during late spring when southerly monsoon winds move warm, humid air masses inland. Heavy rainfall continues through the summer months. River discharge increases during this time and peaks in June or July.
In the upper basin in western China the Changjiang and tributaries are deeply incised, mountain bedrock streams with little or no adjoining floodplain and, therefore, limited agriculture. Downstream of the Three Gorges the Changjiang is bounded by broad alluvial valleys. Fertile bottomland soils with abundant water and long growing season support intensive cultivation. This includes much of the country’s most productive farmland that is at the center of China’s rice growing region. It is a tectonically active region with areas of subsidence that result in many lakes along the main channel (Wang et al., 2005). The largest is Poyang Lake in Jiangxi Province that drains into the Changjiang at Hukou. It covers greater than 4,000 km2 during the summer wet season with a volume of 30 billion m3. Dongting Lake that also drains into the Changjiang in Hunan Province is slightly smaller with a volume of 22 billion m3. These lake basins are vast areas for floodwater storage.
Levees protect extensive areas of highly productive farmland and a population of millions living in the low-lying alluvial valleys along the Changjiang and lower segments of its large tributaries. Most major levees are built on or very near riverbanks. For centuries the largest levees were sufficient to protect against normal yearly floods, but inadequate during severe flood events. Major levee construction projects beginning during the 1950s have increased levee height and the area of flood protection. Flood control policies were modified following the record high 1998 flood. This included permanent removal of some levees and the opening of others during severe floods to increase floodwater storage.
Flood history
Before operation of the Three Gorges dam
Sources for floods before the hydrology data recording period date back centuries and include inscriptions, monuments, and government documents. The 1849 flood was among the most severe in the 19th century (Jiangxi Province County Government, 1849). It occurred before first hydrology recording station on the middle Changjiang was established in 1865 at Hankou, which is 900 river km below the TGD. So, the exact water level is unknown. It is, however, well documented on both the middle and lower sections of the Changjiang (Zhao et al., 2004; Yang et al., 2021). E.L Oxenham (1875), who lived in Wuhan, Hubei Province, the largest city on the middle Changjiang, witnessed the 1849 flood. He noted that “many floods are recorded in the last and present century, but the highest one on record in modern times is that of 1849 … when the city was flooded to the depth of (4 m) … and innumerable houses swept away.”
Twentieth century floods set successive record highs in 1931, 1954, and 1998. The increasing severity of floods is attributed to 1) levee construction and the loss of floodwater storage and 2) sedimentation of large lakes that drain into the Changjiang. Higher and better constructed levees reduced flood risk in adjacent floodplains, but they constrict floodwater resulting in a higher water surface elevation during floods of equal discharge. Channel aggradation since the 1950s further increased flood risk. Changes in the sediment budget might have been related to land-use change and soil erosion, but this relationship was not well established. Although average annual peak stage has increased during this period, discharge has been almost constant indicating a smaller channel cross-section (Jun and Chen 2001; Chen and Lu 2003; Wang et al., 2007).
During the summer of 1931 the lower section of the Changjiang experienced a severe flood that ranks as one of the world’s greatest natural disasters. River stage at the flood peak was the highest recorded since the Hankou hydrology station was established. Many levees along the main channel of the Changjiang were overtopped or failed, and about 180,000 square kilometers of the surrounding alluvial plains were submerged. The British Naval Air Corps conducted aerial surveys to map flooded areas. This flood directly accounted for about 140,000 deaths. Millions of others died shortly afterwards from starvation and disease (Report of the National Flood Relief Commission. 1933). Although at the time the catastrophic nature of this event was well known, the disaster seems to be mostly forgotten both within China and the outside world.
The 1954 flood had the highest water level recorded until that time. Maximum river stage at Hukou was 21.68 m, well above the previous record high of 20 m during 1931. The 1954 flood was a calamity. Many of the major levees along the middle Changjiang and its tributaries were over-topped or failed. Vast areas adjacent to the rivers were submerged resulting in many deaths and an enormous economic loss (Shankman et al., 2012). Both the 1983 and 1995 floods reached a similar or slightly higher maximum stage at 21.71 m and 21.8 m, respectively. Forty-four years after the 1954 flood was the great flood of 1998 that had by far the highest river stage in recorded history. Water level at Hukou (22.59 m) exceeded the 1954 maximum by 0.9 m. The 1998 flood stage was much higher but less destructive than earlier floods because of improved levees.
2020 flood after completion of the Three Gorges dam
The 2020 flood was the most severe since completion of the TGD and ranked among the highest in recorded history on the middle Changjiang. The region was severely affected. About three million people were forced to temporarily relocate and 39,00 km2 of cropland was affected. The economic loss was 110 billion Yuan (17 billion US$) (Jia et al., 2022). This event, as with other severe floods, followed a winter El Nino. Rainfall during the preceding spring in much of southern China was near average. The period of intensive rainfall began in early June and continued until August. Many stations in the Changjiang basin experienced rainfall more than 300 mm above average (Zhou et al., 2021).
Heavy rainfall in the upper Changjiang basin resulted in a series of peak flows into the TGR exceeding 50,000 m3 per second (Figure 2). On August 20 the TGR experienced the highest inflow on record at 74,600 m3 per second. TGR stage reached 164.58 m on July 29, almost 20 m beyond the flood limit level (145 m) recorded on July 1 and the highest recorded during a summer flood since the completion of the TGD. Discharge from the TGR increased to 50,000 cubic meters per second lowering the TGR during the next 14 days to 153 m.
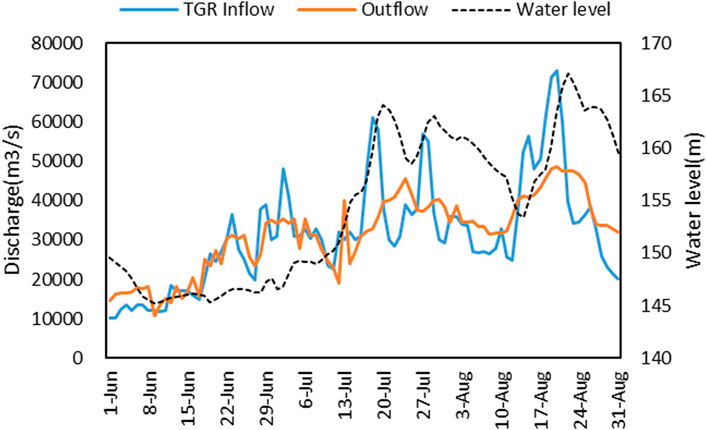
FIGURE 2. Three Gorges Reservoir inflow and downstream discharge, and stage upstream of the Three Gorges Dam during summer 2020.
The TGD reduced potential peak stage downstream. Flood peak at the Dongting Lake Chenglingji Station was 34.58 m, 1.36 m below the record high of 35.94 m in 1998 and the sixth highest water level in history. The Hankou Station flood peak was 28.77 m, 0.66 m below 1998. The maximum river stage at Hukou was 22.49 m, only 0.1 m below the record highs during 1998 (Table 1). All these peak values appeared in middle July when the outflow of the TGR was under 40,000 cubic meters per second. From June 20 to July 13, the reservoir had impounded about five billion m3 of floodwater. Yao et al. (2021) estimated that the TGD lowered potential maximum stage along the middle Changjiang at Hankou and Jiujiang (upstream of Hukou), by about 0.11 m and 0.09 m, respectively. During August, a period of high discharge from the upper basin, the TGR floodwater storage reduced potential downstream peak discharge from 74,600 m3/s to 50,000 m3/s. It also decreased potential stage at Yichang and Shashi by 1.64 m and 2.55 m, respectively, and greatly reduced flood risk for the 360 km river segment directly downstream of the dam. (Yao et al., 2021).

TABLE 1. Maximum river stage (m) during the 1931, 1954, 1998, and 2020 floods at three middle Changjiang gaging stations. Downstream distance from the Dam is shown (river km).
Discussion
Hydroclimate and El Nino
El nino is strongly linked to rainfall throughout the Changjiang basin (Ma et al., 2018). Subtropical high pressure over the western Pacific Ocean controls the summer monsoon in eastern Asia. This pressure system during summer moves warm moist air inland from the southeast. When colliding with cold air from the arid north, this airflow is lifted, causing abundant rainfall (Samel et al., 1995). During a normal or La Nina year, this frontal boundary is generally pushed northward causing below average rainfall throughout much of the Changjiang basin. In contrast, during El Nino the front is blocked from moving far north, which results in heavy precipitation that can result in severe Changjiang floods (Samel et al., 1995; Samel and Liang 2003; Shankman et al., 2006).
Several severe floods were recorded on the middle Changjiang during the second half of the 20th century (1954, 1973, 1983, 1995, 1998, 2020). Severe floods on the middle Changjiang were defined by Shankman et al. (2012) as Hukou stage 2 m above a maximum discharge time-series trend line. All these floods followed winter and spring El Nino events (Figure 3) that weakened toward the late spring and approached a neutral summertime position (1983, 1995, 1998, 2020) or moved into La Nina (1954, 1973). Winter and spring rainfall preceding these floods was generally near average. Rainfall during most summer months was far above average. During the 1973 flood June rainfall at some stations was 450 mm above average; 1954, 1995, 1998, and 2020 had at least one summer month with rainfall greater than 300 mm above average; and 1983 had two summer months with rainfall greater than 150 mm above the monthly average. (Shankman et al., 2012).
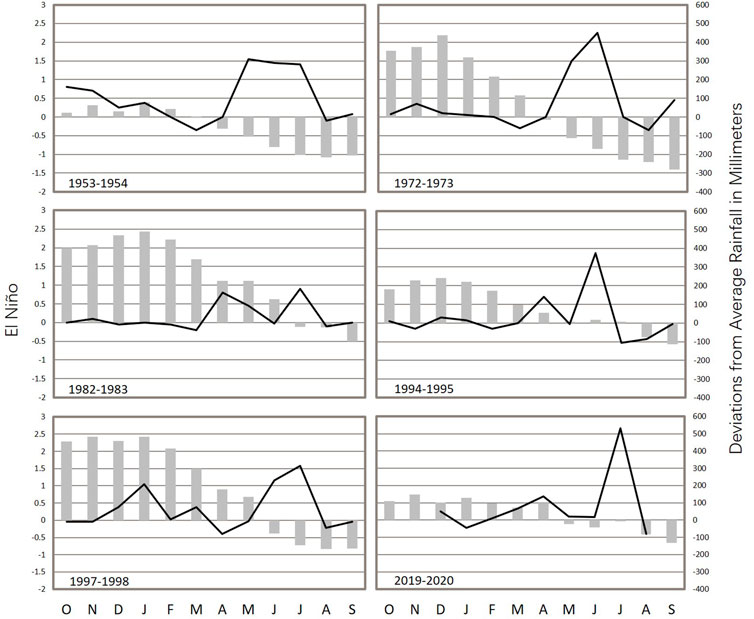
FIGURE 3. El Nino-3.4 and Nanchang, Jiangxi, rainfall deviations from average preceding and during severe floods occurring 1954–2020.
The El Nino–precipitation relationship is not entirely understood. Although El Nino events greatly increase the probability of above average rainfall, strength of l Nino is not directly related to rainfall intensity. For example, the 1953–1954 El Nino was the weakest preceding the severe floods mentioned above, but this was the only flood year with rainfall at least 250 mm above average for three consecutive summer months. The strongest El Nino among these five events preceded the 1983 and 1998 floods, but rainfall for these years, while still far above average, was less than during the 1954 flood. Conversely, during neutral or La Nina years, when rainfall is typically below average, the Changjiang sometimes reached flood stage, but these years the middle Changjiang never had a severe flood (Shankman et al., 2012).
Three Gorges reservoir storage capacity
Effective flood-control by the TGD depends partially on flood storage capacity exceeding upstream discharge during periods of high river stage downstream. Several studies before dam became operational concluded the TGD would not be effective during severe floods as occurred in recent decades (Hu and Zhu 1998; Liu and Wu 1999). These findings are consistent with later studies reporting the dam’s inability to have a substantial effect on Changjiang discharge during the most severe floods (Hartmann et al., 2004; Li et al., 2004). Studies completed after the TGD was built had access to data collected during normal operating conditions. In contrast to earlier investigations, these studies, as previously discussed, showed that the TGD during 2020 reduced downstream potential maximum discharge.
A study by Yan et al. (2021) determined that the TGR capacity can effectively withstand floods below a 100-years return interval. However, during 2020, only a few years after the TGD became operational, the middle Changjiang suffered from a major flood that was among the most severe in recorded history. Operation of upper basin reservoirs and the TGR, together with a floodwater storage capacity of 30 billion m3, reduced potential flood peaks on the middle Changjiang (Xia and Chen 2021). But when put to the test, the TGD did not meet the 100-years return interval standard expected for flood control. This is attributed to a large extent to high rainfall and tributary discharge on the middle Changjiang unaffected by the TGD.
Middle basin rainfall and tributary discharge
Major floods cannot be avoided by operation of the TGD alone. During the most severe floods such as 1954, 1998, and 2020, abnormally high rainfall occurred throughout the Changjiang basin. The TGD can only affect discharge from the upper basin. Large tributaries on the middle Changjiang unaffected by the TGD include the Hanjiang in Hubei that is 1,500 km in length, in addition to Hunan rivers that flow into Dongting Lake and Jiangxi rivers that drain into Poyang Lake. Several others can be added to this list. The total drainage area of the middle Changjiang is about 680,000 km2, which is 38 percent of the total river basin. Figure 4 shows total Changjiang and tributary discharge downstream of the Three Gorges during the 1998 flood, which occurred before operation of the TGD. Tributary discharge was more than twice the volume from the upper Changjiang basin. Poyang and Dongting Lakes retain floodwater and, therefore, increasing Changjiang discharge with distance downstream is not proportional to tributary discharge. Despite that, the cumulative impact of high tributary discharge increased flood severity with distance downstream. Peak stage during 2020 on the river section immediately downstream of the TGD was well below the record high during 1998. In comparison, maximum stage at Hukou, which is downstream of the Hanjiang and Dongting Lake, and at the mouth of Poyang Lake, was only 0.1 m below the record high during 1998.
Studies on Dongting Lake (Lai and Wang 2017) and Poyang Lake (Shankman et al., 2012) completed after the TGD was operational but before the 2020 flood independently concluded that these areas along the middle Changjiang are still susceptible to sever floods. These findings were based mostly on 1) tributary discharge and 2) loss of floodwater storage that affects river-lake interaction. The 2020 flood showed these studies to be correct. Although rainfall and discharge from the upper Changjiang basin during 2020 was similar to 1998, in some areas in the middle Changjiang basin it was higher. Several stations at Poyang Lake during 2020 recorded the highest water levels in the recorded history.
River-lake interaction
Poyang and Dongting Lakes are the largest freshwater lakes in China. These lake basins are, by far, the largest areas for floodwater storage on the middle Changjiang (Figure 4). Poyang and Dongting Lakes have surface area of about 3,800 and 2,700 km2 respectively during summer floods. Large rivers flow into both lakes that for most of the year drain into the Changjiang through narrow outlets. Historically, as the Changjiang discharge and stage increased as expected during early to mid-summer months, the Changjiang back-flowed into these lakes, resulting in significantly lower Changjiang stage than would otherwise occur.
Changjiang-lake interaction can be affected by decreasing lake floodwater storage capacity and timing of lake tributary peak discharge. Decreasing lake capacity during the past few decades is a result of improvements or new construction of levees and land reclamation, and to a lesser extent lake sedimentation (Shankman et al., 2006; Lai and Wang 2017). From the early 1950s to 1998 Poyang Lake surface area decreased from 5,160 to 3,860 km2. Dongting Lake during the same period decreased 4,350 to 2,700 km2 (Jiang et al., 2008). Total loss of floodwater storge was 21.1 billion m3 (Min 1999; Dou and Jiang 2000). To understand the magnitude of this loss, consider that this is only slightly less than floodwater storage capacity of the TGR.
As a result, during summer high discharge periods 1) tributaries are flowing into the lakes with a higher base level than previously occurred and 2) less floodwater storage is available during the most severe Changjiang floods. Further, lake changes might affect the timing of peak flows. Instead of Changjiang backflows into the lakes as previous expected, high Changjiang discharge occurs at the same time as high tributary discharge that increases peak stage. During the 2020 flood, higher lake base stage and coincidence of high Changjiang and tributary discharge contributed to some Changjiang stations reaching near their historic highs. Altered timing of peak TGD discharge during severe floods so that maximum Changjiang stage and lake discharge no longer coincide could effectively mitigate flood intensity (Li et al., 2017; Zhang et al., 2022).
Channel morphology and discharge capacity
Change in downstream Changjiang sediment load can affect flood risk, but the long-term effects are uncertain. Decrease in sediment after dam construction resulted in channel bed incision to varying degrees downstream of the dam to the Chenglingji river section near Dongting Lake (Xu and Milliman, 2009; Xu et al., 2020). Large floodwater storage areas are designated along this section of Changjiang. A larger channel with higher discharge capacity will lower river stage so that during major floods water surface elevation will be below the level needed for the river to spread into the planned retention areas. In this case, an unimpeded discharge continues downstream.
Studies have predicted channel aggradation downstream of Chenglingji that will reduce channel capacity. The Changjiang Science Academy estimates 0.34 m channel aggradation at Hukou 22 years after dam completion. The China Academy of Science estimates a much higher rate of 1.46 m,1.69 m, and 1.78 m channel aggradation 10, 22, and 46 years, respectively, after dam completion (Li et al., 2004). Zhou et al. (2021) presented similar conclusions. If the Changjiang aggrades as these studies project, river stage will be higher than would otherwise occur. In contrast, Wang et al. (2007) addressing sediment budgets on the middle and lower Changjiang suggested reduced sediment delivery in the middle and lower reaches of the Changjiang because of sediment storage in tributaries. This suggests channel bed stability or incision during the coming decades as opposed to aggradation.
Levees and floodwater storage
The probability of severe floods can be affected by changes in floodwater storage.
As previously discussed, alluvial valleys along the middle and lower Changjiang and large tributaries are protected by levees. The total length of levees in this region is more 30,000 km (Wang and Plate 2002; Li et al., 2003). Most major levees are built on or immediately adjacent to the riverbanks, which detaches river channels from the adjoining floodplain. This reduces the area available for floodwater storage and results in a higher water surface elevation during floods of equal discharge. Enlargement and improvement in levee quality during the past few decades has made them better able to withstand severe floods. Many levees were overtopped or failed during the 1954 flood. Water diversion onto the surrounding floodplains was greater than 100 billion m3 submerging 50,000 km2 of the surrounding alluvial plains. Maximum river stage was much higher during 1998, but because of levee improvement total water diversion was about 10 billion m3, only ten percent of that during 1954 (Li et al., 2003).
Because of increasing severity of floods during the 20th century, flood planning and management priorities in China moved away from flood control based mostly on levee construction to give greater floodplain protection and towards flood management with a focus on increasing floodwater storage. Some smaller levees were removed, and formerly protected areas are now designated for floodwater storage. Also, some levees that protect large areas during normal flood years are opened when critical flood stage is reached, and additional floodwater storage is most needed to lower river stage and reduce the probability of levee failures. This is, at least partially offset by improvements in the major river levees since 1998 that reduce levee failures and water diversion into surrounding floodplains.
Conclusion
We conclude that the Three Gorges Dam cannot prevent the most severe floods that will continue to occur regularly on a decadal time scale for the following reasons:
1) The 2020 flood occurred within a few years of the TGD becoming operational and 22 years after the great flood during 1998. It was among the highest in recorded history on some stations along the middle Changjiang. The TGD in some studies has been considered effective against floods with a 100-years discharge return interval. This conclusion, however, is inconsistent with the hydrologic record.
2) Flood risk downstream of the TGD depends on both (a) upstream discharge and (b) rainfall in the middle Changjiang basin and tributary discharge, which is independent of the TGD. During the most severe floods, such as 1954, 1998, and 2020, abnormally high rainfall occurs throughout the Changjiang basin, both upstream and downstream of the Three Gorges. Total tributary discharge on the middle Changjiang during severe floods is much greater than that from the upper basin.
3) Floodwater storage for Poyang Lake and Dongting Lake decreased during past decades. Instead of Changjiang backflows into the lakes as previous expected during severe floods, Changjiang discharge coincides with high tributary discharge and lake stage.
Data availability statement
The data analyzed in this study is subject to the following licenses/restrictions: The data that support this study are available from the corresponding author upon reasonable request. Requests to access these datasets should be directed to XL, xjlai@niglas.ac.cn.
Author contributions
DS: Writing-original draft, Writing-review and editing, Supervision. XL: Writing-original draft, Writing-review and editing, Supervision, Funding acquisition.
Funding
This work was supported by the National Natural Science Foundation of China (No. 42171012).
Conflict of interest
The authors declare that the research was conducted in the absence of any commercial or financial relationships that could be construed as a potential conflict of interest.
Publisher’s note
All claims expressed in this article are solely those of the authors and do not necessarily represent those of their affiliated organizations, or those of the publisher, the editors and the reviewers. Any product that may be evaluated in this article, or claim that may be made by its manufacturer, is not guaranteed or endorsed by the publisher.
References
Chen, Y. C., and Lu, H. J. (2003). An analysis of the variation of maximum flood stage of the Lower Yangtze River. J. Hydraulic Res. 41, 235–238.
Cheng, B. (1997). “The effect of the project on the lake area environment along the middle section of the Changjiang,” in Research on the effects of the three Gorges dam on the ecosystem and environment. Editor L. Weng (Beijing: Scientific Press), 103–120.
Dou, H. S., and Jiang, J. H. (2000). Dongting Lake. China: Chinese Scientific and Technology University Press ((in Chinese).
Duan, W., He, B., Nover, D., Fan, J., Yang, G., Chen, W., et al. (2016). Floods and associated socioeconomic damages in China over the last century. Nat. Hazards (Dordr). 82 (1), 401–413. doi:10.1007/s11069-016-2207-2
Edmonds, R. L. (1992). The Sanxia (Three Gorges) project: The environmental argument surrounding China’s super dam. Glob. Ecol. Biogeogr. Lett. 2, 105–125. doi:10.2307/2997637
Hartmann, K., Becker, S., and King, L. (2004). “The Three-Gorge project: Its influence on the flood risk along the middle reaches of the Yangtze River and on the economy of the region surrounding the reservoir,” in Climate change and Yangtze floods. Editor T. Jiang (Science Press), 396–404.
Hu, X., and Zhu, H. (1998). Three-Gorges project and flood protection of important cities in the Poyang Lake region. J. Jiangxi Normal Univ. 22 (4), 365–370.
Jia, H. C., Chen, F., Pan, D., Du, E., Wang, L., Wang, N., et al. (2022). Flood risk management in the Yangtze River basin - comparison of 1998 and 2020 events. Int. J. Disaster Risk Reduct. 68, 102724. doi:10.1016/j.ijdrr.2021.102724
Jiang, J., Lai, X., and Huang, Q. (2007). The characteristics of flood responses to the restoration of polders on Dongting Lake, China. Hydrological Sci. J. 52, 671–685. doi:10.1623/hysj.52.4.671
Lai, X., and Wang, Z. (2017). Flood management of Dongting Lake after operation of three Gorges dam. Water Sci. Eng. 10 (4), 303–310. doi:10.1016/j.wse.2017.12.005
Li, G. X., Jie, Y. X., and Li, Q. Y. (2003). Yangtze Dyke and its strengthening. Lowl. Technol. Int. 5, 39–46.
Li, R. Z., Xiong, D. K., and Liu, Y. (2004). “Future change in the Poyang Lake wetland ecosystem,” in Poyang Lake wetland ecosystem evaluation. Editor X. H. Wang (Science Press), 151–173.
Li, X., Zhang, Q., Hu, Q., Zhang, D., and Ye, X. (2017). Lake flooding sensitivity to the relative timing of peak flows between upstream and downstream waterways: A case study of Poyang Lake, China. Hydrol. Process. 31, 4217–4228. doi:10.1002/hyp.11362
Liu, X. D., and Wu, D. Y. (1999). Brief analysis of the effect of the Poyang Lake water level in flood period by the Three-Gorges project. Jiangxi Hydraul. Sci. Technol. 25, 71–75.
Ma, F., Ye, A., You, J., and Duan, Q. (2018). 2015-16 Floods and droughts in China and its response to the strong El Niño. Sci. Total Environ. 627, 1473–1484. doi:10.1016/j.scitotenv.2018.01.280
Min, Q. (1999). Impact evaluation of Poyang Lake reclamation on floods (in Chinese). Yangtze River 7, 30–32.
Oxenham, E. L. (1875). On the inundations of the Yang-tse-kiang. J. R. Geogr. Soc. Lond. 45, 170–184. doi:10.2307/1798708
Report of the National Flood Relief Commission (1933). Report of the National flood Relief Commission, 1931-1932. Shanghai: Comacrib Press.
Samel, A. N., and Liang, X. Z. (2003). Understanding relationships between the 1998 Yangtze River flood and northeast Eurasian blocking. Clim. Res. 23, 149–158. doi:10.3354/cr023149
Samel, A. N., Wang, S. W., and Wang, W. C. (1995). A comparison between observed and GCM-simulated summer monsoon characteristics over China. J. Clim. 8, 1690–1696. doi:10.1175/1520-0442(1995)008<1690:acboag>2.0.co;2
Sauer, V. B. (1986). “Flood-frequency analysis with historical data in China,”. Editor V. J. Singh (Louisiana State University). in Hydrologic Frequency Modeling; Proceedings of the International Symposium on Flood Frequency and Risk Analysis. 1986. Baton Rouge, USA.
Shankman, D., Keim, B. D., Nakayama, T., Li, R. F., Wu, Y. D., and Remington, W. C. (2012). Hydroclimate analysis of severe Floods in China’s Poyang Lake region. Earth Interact. 16, 1–16. doi:10.1175/2012ei000455.1
Shankman, D., Keim, B. D., and Song, J. (2006). Flood frequency in China’s Poyang Lake region: Trends and teleconnections. Int. J. Climatol. 26, 1255–1266. doi:10.1002/joc.1307
Wang, S. J., Chen, Z. Y., and Smith, D. G. (2005). Anastomosing river system along the subsiding middle Yangtze River basin, southern China. Catena 60, 147–163. doi:10.1016/j.catena.2004.11.007
Wang, Z. Y., Li, Y. T., and He, Y. P. (2007). Sediment budget of the Yangtze River. Water Resour. Res. 43, W04401. doi:10.1029/2006WR005012
Wang, Z. Y., and Plate, E. J. (2002). Recent flood disasters in China. Proc. Institution Civ. Eng. - Water Marit. Eng. 154, 177–188. doi:10.1680/wame.2002.154.3.177
Xia, J., and Chen, J. (2021). A new era of flood control strategies from the perspective of managing the 2020 Yangtze River flood. Sci. China Earth Sci. 64 (1), 1–9. doi:10.1007/s11430-020-9699-8
Xu, J. Z. (1976). Floods and Droughts in Jiangsu Province and Shanghai. Nanjing Institute of Geography and Limnology. Chinese Academy of Science.
Xu, K., and Milliman, J. (2009). Seasonal variations of sediment discharge from the Yangtze River before and after impoundment of the three Gorges dam. Geomorphology 104, 276–283. doi:10.1016/j.geomorph.2008.09.004
Xu, Z., Xu, X., Li, A., Zhang, L., and Hu, C. (2020). Flood control effect of the fluvial process in the middle and lower reaches of the Yangtze River. Adv. Water Sci. 31, 366–376.
Yan, Q., Jing, Z., Xia, Z., and Zhang, S. (2021). Flood control capacity of the three Gorges project for different frequency floods. Environ. Eng. Sci. 38, 1195–1205. doi:10.1089/ees.2021.0003
Yang, Y., Xu, Z., Zheng, W., Wang, S., and Kang, Y. (2021). Rain Belt and flood peak: A study of the extreme precipitation event in the Yangtze River basin in 1849. Water 13, 2677. doi:10.3390/w13192677
Yao, S., Guo, X., and Chen, D. (2021). Analysis of the flood process and characteristics in the middle and lower reaches of the Yangtze River during the 2020 flood season. China Flood Drought Manag. 31 (2), 5–10.
Zhang, C., Ji, C., Wang, Y., and Xiao, Q. (2022). Flood hydrograph coincidence analysis of the upper Yangtze River and Dongting Lake, China. Nat. Hazards (Dordr). 110 (2), 1339–1360. doi:10.1007/s11069-021-04993-2
Zhang, S., Jing, Z., Yi, Y., Wu, Y., and Zhao, Y. (2017). The dynamic capacity calculation method and the flood control ability of the Three Gorges Reservoir. J. Hydrology 555, 361–370. doi:10.1016/j.jhydrol.2017.10.043
Zhao, W., Zheng, J., and Ge, Q. (2004). The reconstruction of 1755 and 1849 severe flood events in Jiangsu and Anhui Provinces. Sci. Meteorol. Sin. 24, 460–466.
Keywords: River-lake system, Yangtze River, flood, water safety, Dam impact, ENSO (El Nino, southern oscillation), Three Gorges Dam
Citation: Shankman D and Lai X (2022) Severe flood probability on the middle Changjiang (Yangtze River) after completion of the Three Gorges Dam. Front. Earth Sci. 10:1006004. doi: 10.3389/feart.2022.1006004
Received: 28 July 2022; Accepted: 07 September 2022;
Published: 29 September 2022.
Edited by:
Weili Duan, Xinjiang Institute of Ecology and Geography (CAS), ChinaReviewed by:
Jingqiao Mao, Hohai University, ChinaHui Tao, Xinjiang Institute of Ecology and Geography (CAS), China
Copyright © 2022 Shankman and Lai. This is an open-access article distributed under the terms of the Creative Commons Attribution License (CC BY). The use, distribution or reproduction in other forums is permitted, provided the original author(s) and the copyright owner(s) are credited and that the original publication in this journal is cited, in accordance with accepted academic practice. No use, distribution or reproduction is permitted which does not comply with these terms.
*Correspondence: Xijun Lai, xjlai@niglas.ac.cn