- 1School of Resources and Geosciences, China University of Mining and Technology, Xuzhou, China
- 2Key Laboratory of Unconventional Natural Gas Evaluation and Development in Complex Tectonic Areas, Ministry of Natural Resources, Guiyang, China
- 3Guizhou Research Institute of Oil and Gas Exploration and Development Engineering, Guiyang, China
In order to study the basic properties of coal seam thus to provide reference for coalbed methane (CBM) production, the porosity and permeability of coal from DH and WJZ regions of Dahebian syncline in Liupanshui Coalfield were systematically studied. The results shows that the porosity and permeability of coal samples in DH region are between 7.4–10.1% and 0.01 mD∼0.04 mD respectively. The permeability is obviously anisotropic, and it is distributed in a U-shape along bedding angle. The porosity and permeability in the WJZ region are between 12.4–24.85% and 0.3 mD–4.8 mD, but the correlation of permeability and bedding angle is not obvious. There are many primary cracks in coal matrix of WJZ region, while the coal of DH region is relatively complete. As a result, the porosity and permeability of WJZ coal samples are much higher than that of DH coal samples. With the change of confining pressure, obvious change has taken place in the permeability of coal in the two regions. When the confining pressure decreases from 2 to 8 MPa, the permeability loss rate of coal samples is close to 80%. The internal mechanism of the permeability loss is related to crack closure and plastic deformation caused by confining pressure. In contrast, the porosity and permeability of coal in WJZ region are much higher than those in DH region, which indicates that the permeability of the same coal seam in the same structural will have great differences and inhomogeneities. Therefore, the WJZ region is the preferred area, which can be included in the preferential exploitation plan of CBM. In addition, for the typical syncline gas reservoir in Liupanshui Coalfield, it is necessary to consider the block division according to the porosity and permeability indexes, and select the optimal block and give priority to development of CBM. Furthermore, we should pay attention to the difference and adaptability of CBM development in different regions.
Introduction
Natural gas is an important clean energy, which is of great significance for reducing carbon emissions, haze and acid rain hazards (Aguilera, 2014; Nduagu and Gates, 2016; Shen et al., 2016). At present, the annual consumption of natural gas in China has reached 300 billion m³, and it is expected to reach 500 billion m³ by 2030 (Liang et al., 2019; Liu et al., 2020a). Natural gas exploration and development (Wang et al., 2021), pipeline transportation (Liu et al., 2019a; Liu et al., 2020b), storage (Liu et al., 2019b; Zhang et al., 2019; Liu et al., 2020c; Liu et al., 2020d; Wan et al., 2021; Zhang et al., 2021) and peak shaving (Lin and Wang, 2012; Qiao et al., 2019; Qiao et al., 2020) have become important deployment and research work in the energy field of China. However, China’s conventional natural gas reserves are limited and its dependence on foreign countries is too high at present, which has become an important bottleneck restricting the future development of China’s natural gas (Wang and Lin, 2014). The total resources of unconventional natural gas (coal-bed methane, shale gas and tight gas) in China reach over 190 trillion m³, more than 5 times of the total geological reserves of conventional natural gas (Zheng et al., 2018). Therefore, in order to realize the large-scale utilization of natural gas in the future, it is particularly important to speed up the exploration and development of unconventional natural gas. However, compared with conventional natural gas reservoirs, unconventional natural gas reservoirs are generally characterized by low porosity and low permeability, so it is difficult to effectively form commercial industrial exploitation with the existing exploitation technologies (Zhou, 2015). Taking CBM resources as an example, the geological reserves of shallow CBM in China below 2,000 m are 368,10 billion m3 (Li et al., 2015). However, because most coal seams in China are soft and low permeability reservoirs, the surface and underground exploitation of CBM is not satisfactory (Regan and Chao, 2014; Tao et al., 2018; Men et al., 2021).
Since the “11th Five-Year Plan”, the central government and local governments have issued a series of support policies such as “Effective development and utilization of coalbed methane”, but the long-term development was not satisfactory. In the “12th Five-Year Plan” period, the output of CBM should reach 30 billion m³, including 16 billion m³ above ground and 14 billion m³ below ground. By 2015, both goals had failed, and only 4.4 billion m³ was extracted from the surfaces. During the “13th Five-Year Plan” period, the output of surface extraction was about 6 billion m3, while the target was 10 billion m3, just over half of which. In the beginning of the “13th Five-Year Plan”, the China’s CBM production was mainly concentrated in Jincheng and Liulin, Shanxi province, and the total utilization rate of China’s resources was less than 0.1%. So far, the situation has not improved significantly (Wang et al., 2019).
Guizhou Province is the province with the richest coal resources in South China, and its resource reserves exceed the sum of the fourteen provinces in South China. As an important energy supply base in South China, Guizhou Province plays an important role in ensuring energy security in South China. CBM is a semi-natural resource of coal mines, and the reserves of CBM in Guizhou Province are also very large. According to the shallow buried depth of 2000m, the total amount of CBM resources in Guizhou Province is 3,151 billion m3, accounting for about 10% of the total CBM resources in China, ranking the third in China, only after that of Xinjiang and Shanxi (Huang et al., 2010; Zhou et al., 2015). Therefore, most mines in the west of Guizhou Province belong to high gas mines, and gas safety accidents occur frequently (Zhong et al., 2016). Coal methane, like a sword hanging on miners’ heads, which directly affects miners’ life safety, and it is a big worry for the development of coal industry in Guizhou province. Therefore, realizing the safe and efficient exploitation of CBM resources in Guizhou Province can not only greatly alleviate the safety situation of coal mining in Guizhou Province, but also obtain a large amount of clean energy-CBM (methane as its main component), which is of great significance for improving the energy structure of Guizhou Province, which lacks gas and oil.
However, compared to other types of rock (Li et al., 2021a; Li et al., 2021b), coal have low strength. And compared with the coal seam conditions in Qinshui Basin, Shanxi Province, the coal reservoir conditions in Guizhou Province are more complex, and the exploitation is more difficult. Coal reservoirs in Guizhou Province have special conditions, such as high in-situ stress, low permeability and low water-content, and are composed of several thin-medium-thick coal seams (Yi et al., 2007). However, there are a few studies on the mechanical and physical properties of this kinds of coal reservoirs, which needs further improving. The geological conditions of CBM in Guizhou province are complex, and the mainstream CBM development technologies at home and abroad can not adopt to the complex geological conditions in Guizhou Province, which leads to the unsatisfactory gas production effect of most CBM extraction wells in Guizhou Province.
The characteristics and evolution of porosity and permeability of coal seam are the important basis for evaluating the recoverable potential of CBM and formulating corresponding development plans. In order to realize the efficient exploitation of CBM, scientific understanding of the porosity and permeability characteristics of coal seam is an important prerequisite. Scholars at home and abroad have made extensive research on the characteristics of porosity and permeability of coal reservoirs (Yang et al., 2019; Xue et al., 2020). For example, Ayers pointed out that the coal lithotypes rich in vitrinite tend to have an increased cleavage frequency, so they have a correspondingly higher grades than those with lower vitrinite (Ayers, 2002). Liu et al. studied the anisotropic characteristics of cubic coal sample under triaxial stress state (Liu et al., 2019c). Liu and Rutqvist put forward a new permeability model of coal, which can reflect the influences of internal swelling stress and fracture-matrix interaction (Liu and Rutqvist, 2010; Meng et al., 2011). Meng et al. studied the relationship between the permeability evolution process of coal and in-situ stress, pore pressure by using coal sample obtained from the southern Qinshui basin (Meng et al., 2011). Nie et al. studied the pore structure characteristics of different ranks by gas adsorption and SEM methods (Nie et al., 2015; Liu and He, 2017). Li et al. (Li et al., 2020) studied the mechanism of temperature and pore pressure on the evolution of coal permeability by taking the raw coal of Qianbei Coalfield, Guizhou Province as the research object. Yang et al. studied the anisotropic permeability of coal sample under cyclic load (Yang et al., 2018).
From the above research, it can be seen that the characteristics of porosity and permeability of coal have always been the focus of research in this field. Generally speaking, the characteristics of porosity and permeability of coal reservoirs are greatly affected by coal rank, stress state, temperature and so on. The porosity and permeability characteristics of coal reservoirs usually have great anisotropy and regional differences. However, these studies mainly focus on the coalfields in the north China, such as the Qinshui coalfield, while there are few reports devoted to Liupanshui Coalfield in Guizhou Province. In particular, the research on the characteristics of porosity and permeability of the syncline gas-controlled coal reservoir in Liupanshui Coalfield is even more scarce. Therefore, in this study, the pore and permeability characteristics of typical coal in the Dahebian syncline area of Liupanshui Coalfield were studied in order to fill the research gap in this field and provide sufficient basis for the exploration and development of CBM and the improvement of the suitable technology.
In this paper, the coal of two typical regions of Dabebian Syncline with typical syncline gas-controlling structure in Liupanshui Coalfield, Guizhou Province was selected as the research object. A series of porosity tests and permeability evolution law studies were carried out for the underground raw coal cores in two typical areas (DH region and WJZ region) in the Dahebian Syncline region. The purpose is to provide theoretical reference and basic data for CBM reservoir evaluation, dessert spot screening, and rationale exploitation design of CBM in Liupanshui Coalfield and similar coal seams.
Testing Scheme
Region and Coring
The Liupanshui Coalfield is the most representative coalfield in Guizhou Province. The study area is named as Dahebian syncline, which is one secondary geo-structure in Liupanshui Coalfield. This syncline covers an area of about 50 km2, which is high in the west, and lower in the east, with its major axis in the north-south direction, and its minor axis in the east-west direction. And the position, shape, and boundary of Dahebian syncline are shown in Figures 1A, B. The regional distribution of methane content in coal seams in Liupanshui Coalfield is directly related to the scale of single syncline and the depth of “Depression”, and it has typical syncline gas-controlling structural characteristics. And there are dozens of coal seams with thick-to-medium thickness. Dahebian syncline is a typical syncline controlling gas structure in Liupanshui area, and it is also the preferred block for CBM exploration and development in Guizhou Province.
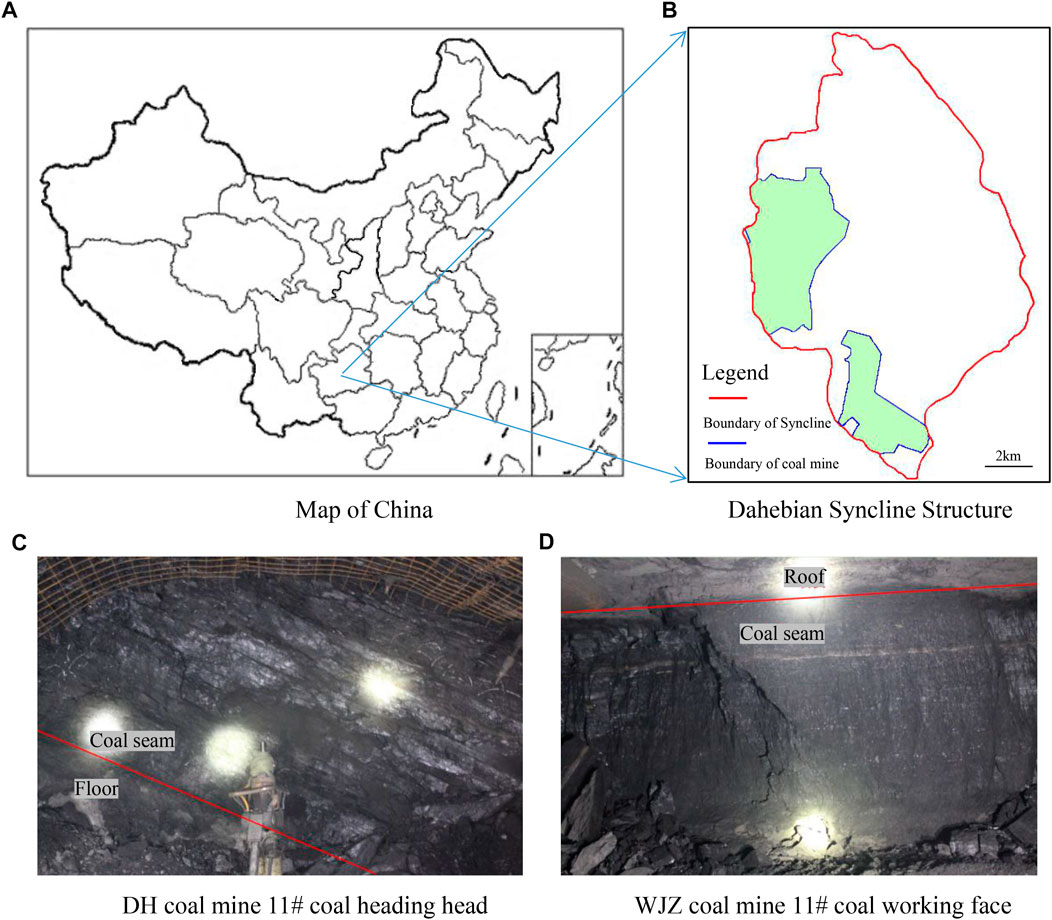
FIGURE 1. Dahebian syncline structure and the condition of underground coal seam in DH and WJZ regions. (A) Map of China. (B) Dahebian Syncline Structure. (C) DH coal mine 11# coal heading head. (D) WJZ coal mine 11# coal working face.
The study on the porosity and permeability characteristics of Dahebian syncline coal seam and its evolution law is of great representative significance to the mining of the whole Liupanshui Coalfield and even the coal seam with syncline gas-controlling structure in other coalfields of Guizhou Province. This study takes No.11 coal seam in DH and WJZ regions was taken as the research object, with a depth of about 800 m, and a layer thickness ranging from 1.77 to 5.7 m. This coal seam has the largest thickness and the highest gas content in this syncline, so it is chosen as the research target. As can be seen from Figures 1C, D, they belong to the same coal seam, but the apparent morphology of the coal seam is still different, which may be related to the tectonic evolution and stress conditions in different regions. At the same time, it is found that the coal exhibits strong bedding characteristics. Therefore, the influence of intersection angle should be fully considered when testing permeability.
Coal Sample Preparation
All coal cores are taken from underground coal mining face in the No.11 coal seam of DH and WJZ regions. The blocks with well integrity and low cracks are selected, and the large blocks with the size over 300 mm × 300 mm × 300 mm are obtained with crowbars, and transported to the surface (Figures 2A), and then packaged and transported to the laboratory. Because the cores contain many fissures and has low strength, so as to reduce the damage to the coal structure, all the samples in this study are processed by wire cutting (Figures 2B). Considering the obvious bedding characteristics of coal, five intersection angles of 0°, 30°, 45°, 60° and 90° are considered in sample preparation, which is defined as the intersection angle between the axis direction of sample and the bedding plane of coal seam. The influence of intersection angles on the permeability will be studied to reveal the anisotropy of permeability, which provides an important reference for the subsequent well-stability anaslysis and hydraulic fracturing designing.
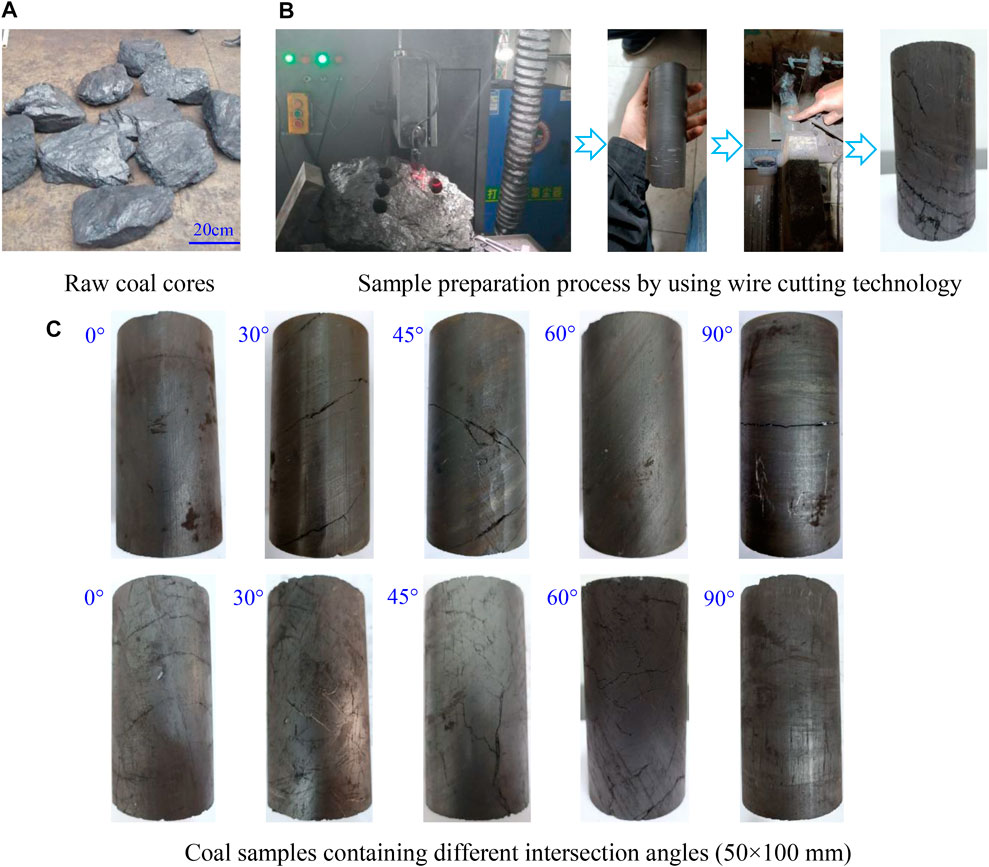
FIGURE 2. Coal cores and samples for porosity and permeability testing. (A) Raw coal cores (B) Sample preparation process by using wire cutting technology (C) Coal samples containing different intersection angles (50×100 mm).
Typical coal samples (50 mm × 100 mm, cylinder-shape) proceeding containing different intersection angles are shown in Figures 2C: the first row is the coal from the DH region. The coal samples have obvious bedding, good integrity, hard texture and a few initial cracks; the second row presents the coal from WJZ region, with many cracks and no obvious bedding, the integrity of which is worse than that of DH coal samples. This also fully shows that although they are in the same syncline tectonic area and the same coal seam, the structural characteristics of coal still show great differences with the different regional locations, which increases the difficulty in forming a unified coal-bed methane development technology.
Testing Principle and Scheme
1) Porosity test
In this experiment, the gas expansion method is used to measure the porosity of rock samples, which is an indirect method. The measuring principle is Boyle-Marius’s specific law: P1V1 = P2V2. First, open the valve of nitrogen bottle, under a certain pressure P1, and expand the gas into a standard chamber whose initial pressure equals the standard atmospheric pressure. The standard chamber has a volume of V1. measure the balance pressure at this moment. Then, open the vale to connect the standard chamber with the core holder. The core holder has a volume of V2. After reaching a new pressure balance, measure the new balance pressure of P2. after then, the gas volume in the system is V1+V2-VS.
According to Boyle-Mallot’s law:
The definition of porosity is:
Where:
Substitute Eqs 1, 2 into Eq. 3 to obtain:
The gas source is provided by the laboratory, including nitrogen, methane, carbon dioxide and helium. Considering the development of micro-pores in coal, the permeable gas medium is helium in this study. The corresponding program has been compiled fro the test system, which only needs to input the basic information of the sample, and the result can be read directly after the pressure becomes stable.
2) Routine permeability testing.
The permeability is measured by the steady-state method: first, an initial fluid pressure difference is applied to both ends of the rock, and the fluid will flow across the rock. After reaching a steady state, the measured flow rate and pressure difference are substituted into the Darcy seepage formula, and finally the corresponding permeability is calculated. The basic principle of the steady-state permeability measurement is Darcy’s law, that is:
Where: Q, unit seepage flow, unit m3/s; K, permeability, unit m2; A, Core cross-sectional area (m2); core length, unit m; h2-h1: Total head loss, unit m.
According to the actual needs of the research, liquid such as brine or gas such as nitrogen can be chosen as the permeation medium, and in some cases, mixed-phase fluid can also be used. When using gas as the permeation medium, the comprehensibility of the gas must be considered, and the calculation formula is as follows:
Where,
Porosity and permeability tests are carried out on the LW-1 porosity and permeability tester (Liu et al., 2020c), which was jointly developed by the State Key Laboratory of Coal Mine Disaster Dynamics and Control at Chongqing University and Jiangsu Tuochuang Science and Technology Co., Ltd. It can be used for porosity test by gas expansion, steady-state permeability test and unsteady-state permeability test. This device can fully meet the test requirements, which was shown in Figure 3.
3) Sample information.
See Table 1 for the test scheme. It mainly includes porosity, conventional permeability, and permeability tests at different confining/inlet pressures.
Pore and Permeability Testing
Results in DH Region
Figures 4A shows the test results of coal porosity in DH region. The porosity fluctuates between 7.38 and 11.22%, with an average value of 9.03%. It can be said that the porosity of DH region is good, which is beneficial to the exploitation of CBM in this area. Figures 4B shows the testing results of coal permeability in DH region. The permeability of coal sample in DH region ranges from 0.0131 mD to 6.5797 mD. It should be noted that the permeability of 6.5797 mD corresponds to the sample containing a penetrating crack. If this sample is excluded, the permeability of DH coal is between 0.0131 mD and 0.0843 mD, with an average value of 0.0381 mD. It can be seen that, the permeability of coal in DH region is very poor, which belongs to low permeability coal seam.
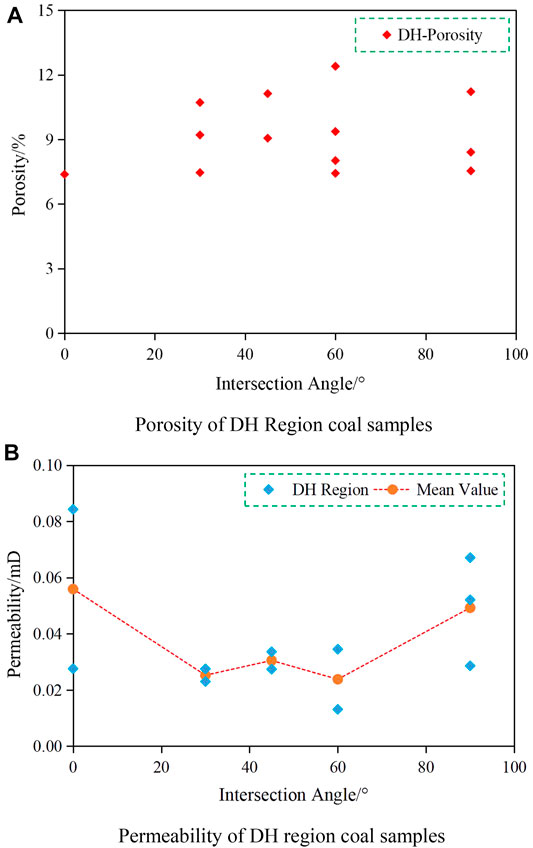
FIGURE 4. Relationship between permeability and Intersection angle of coal in DH region. (A) Porosity of DH Region coal samples. (B) Permeability of DH region coal samples.
Without artificial stimulation measures such as hydraulic fracturing, it is difficult to obtain effective exploitation and utilization of CBM. At the same time, it shows that the existence of cracks will increase the permeability of coal by over than two orders of magnitude. Therefore, it is necessary to pay close attention to the development of primary cracks in coal seams, and find potential favorable regions for the CBM extraction. In addition, it can also be seen in Figures 4B that the permeability of DH coal has obvious angle effect. The permeability of 0° sample (permeating along bedding direction) is the highest, while that of the middle angle samples (30°, 45° and 60°) is the lowest, indicating that the permeability through matrix is slightly poor.
Pore and Permeability Test Results in WJZ Region
The porosity test results of WJZ coal are shown in Figure 5A as follows: the porosity ranges from 7.06 to 24.75%, with an average value of 18.67%. It can be said that the porosity of WJZ coal is high and good. Figure 5B shows the permeability results of the WJZ coal testing. Permeability ranges from 0.175 mD to 5.21 mD, with an average value of 1.36 mD. It can be said that the WJZ coal has good permeability, which is helpful for realizing the development of CBM directly or through simple reservoir reconstruction. Combined with Figure 5, it can be seen that there are many primary cracks in the WJZ coal, which may be an important reason for its good permeability. Due to the existence of a large number of primary cracks, the influence of bedding is weakened, so the relationship between the permeability and intersection angle of WJZ coal is not obvious. In addition, it is found that the permeability of WJZ coal is only several times higher than that of the same group of samples even if the coal contains perforating fractures, while the permeability of DH coal with perforated fracture has no significant difference. Considering the good porosity and permeability characteristics of WJZ coal, it is necessary to pay close attention to the recoverable potential of this region, and evaluate whether this region belongs to the preferential exploitation block of CBM.
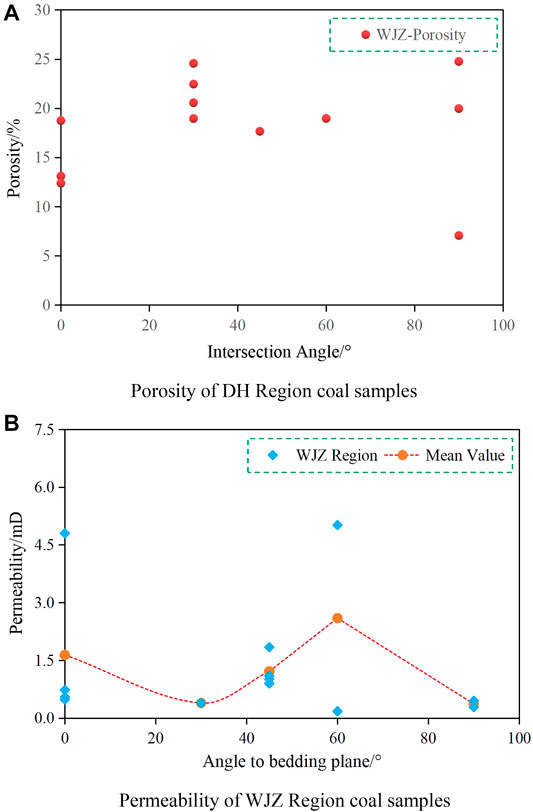
FIGURE 5. Relationship between permeability and intersection angle of coal. (A) Porosity of DH Region coal samples (B) Permeability of WJZ Region coal samples.
Comparison of Regional Results
DH region and WJZ region belong to two blocks in the same synclinal structure, and the coal cores used here belong to the same stratum. However, according to the above tests and analysis, there are obvious differences between them. Figure 6 shows the comparison results of porosity and permeability between these two regions.
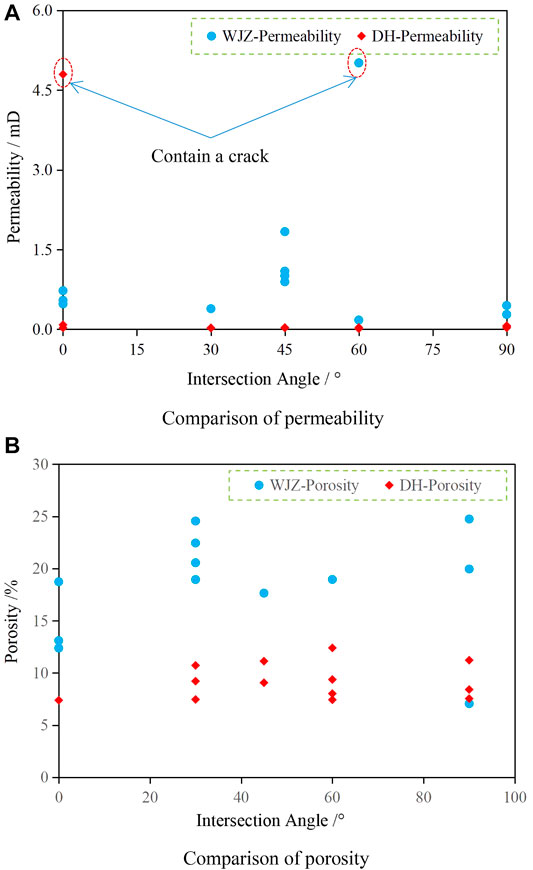
FIGURE 6. Variation of porosity/permeability with intersection angle. (A) Comparison of permeability. (B) Comparison of porosity.
The comparison shows that: 1) Due to the integrity of coal structure, the permeability of coal in DH region is as low as the order of 10−2mD, which belongs to the difficult-to-exploit coal seam. However, once there is a crack, the permeability will increase sharply. 2) The coal has good permeability, which is a favorable condition for methane exploitation. At the mD level, more attention should be paid to its exploitable potential and it should be included in the priority area of methane development. 3) The porosity of WJZ coal is almost twice that of coal in DH region, and the primary cracks are much more developed. From the perspective of CBM exploitation, the WJZ region should be given priority. 4) The comparative results fully show that even if the plane distance between the two study regions are very close, only 8 km, and the coal cores are taken from the same coal seam, the characteristics of porosity and permeability are quite different. Therefore, even in the same coalfield, some priority blocks should be founded and exploited first. In addition, it is necessary to further study the porosity and permeability characteristics of coal, and to find the development technology suitable for each region.
The comparison shows: 1) Because of the integrity of coal structure, the permeability of coal rock in DH region is low, which is in the order of 10−2mD, and without reservoir reconstruction, it is difficult to exploit CBM in this region. However, once there are cracks, the permeability will increase rapidly. 2) The permeability of WJZ coal is almost twice that of the DH coal, with extremely developed cracks. From the perspective of CBM exploitation, WJZ region should be given priority. 3) The comparison results fully show that even if the distance of the same coal seam in the same structural area is as close as 8km, the characteristics of porosity and permeability are quite different. Therefore, the exploitation of CBM in Liupanshui Coalfield must consider the priority regions, such as the WJZ region in Dahebian Syncline.
Influence of Cracks and Pressure
Influence of Cracks
Through the above research, it is found that the permeability test result of individual sample is much higher than other samples in the same group. For example, the permeability of DH-11-02 sample in DH region is as high as 6.5795 mD, which is 117.6 times of the permeability of coals in the same coal seam with the same intersection angle, and 172.8 times of the average permeability of all samples in the same coal seam. After observation, it is found that there is a crack connecting bedding on the surface of sample, as shown in Figure 7. The crack runs through the upper and lower ends of the sample, is almost parallel to the bedding, and bends locally, which is a typical bedding tensile fracture. Because of the existence of such crack, the permeability of the coal is increased by more than two orders of magnitude.
Meanwhile, the permeability of some coal samples in WJZ region is obviously higher than that of the same group. For example, the permeability of WHZ-11-60-06 sample is as high as 5.01mD, which is 8.63 times that of coal with the same intersection angle. This sample has an intersection angle of 60° to the coal seam bedding, but there is a crack on the side penetrating through the sample. The crack surface has a small opening and a relatively straight crack, which is characterized by shear crack. According to the analysis, the intersection angle of 60° is not the dominant direction of seepage migration, but it is this kind of crack that leads to the increase in permeability.
From the above comparison, it can be seen that the integrity of coal in DH region is better, and there are few cracks on the surface of the samples. However, once cracks appear, the increase in permeability is very obvious. From the observation of the sample, most of the cracks are tensile cracks along the bedding direction. However, in WJZ region, the integrity of coal is poor, and there are many primary cracks with poor regularity. These cracks should be formed along with the later tectonic process, including shear cracks and tensile cracks. Due to the existence of primary cracks, the permeability of WJZ coal samples is much higher than that of the DH coal samples. Therefore, when exploring and developing CBM in the WJZ region, the crisscrossing crack-network is a favorable factor for CBM extraction.
Influence of Pressure Condition
In the third part, the conventional permeability tests were carried out when the confining pressure was only 2.5 MPa. However, in the underground space, with the change of coal seam depth and CBM extraction depth, the stress state of coal will change. At present, the depth of coal seam is generally over 300m, and the reservoir pressure of the coal seam is much higher than 2.5 MPa. Therefore, it is still necessary to study the permeability of coal under different confining pressures and different gas pressure, so as to provide a basis for coal permeability evaluation and CBM extraction designing under the condition of field stress state. The fourth-level confining pressure is 2/4/6/8 MPa and the third-level inlet pressure is 0.4/0.6/0.8 MPa.
1) Coal in DH region
Four coal samples in DH region were tested. The results show that with the increase of confining pressure, the permeability of coal shows a downward trend, indicating that the increase of confining pressure would lead to the compaction of coal, thus leading to the decrease of permeability.
The test results show that when the confining pressure increases from 2 to 8 MPa, the permeability of the three intact DH coal samples decreases by 75–93%, indicating that the confining pressure has a great influence on the permeability. When the confining pressure is in the range of 2–4 MPa, the permeability decreases rapidly, and then the decreasing trend slows down. When the confining pressure reaches 8 MPa, the permeability is basically stable, so no higher confining pressure test will be conducted. The influence of inlet pressure on permeability is still not clear. Taking DH-11-0-05 sample (intersection angle = 0°) as an example, the permeability increases with the increase of inlet pressure. However, DH-11-90-01 sample (intersection angle = 0°), the permeability decreases with the increase of inlet pressure. The testing results of three representative samples are selected as shown in Figure 8.
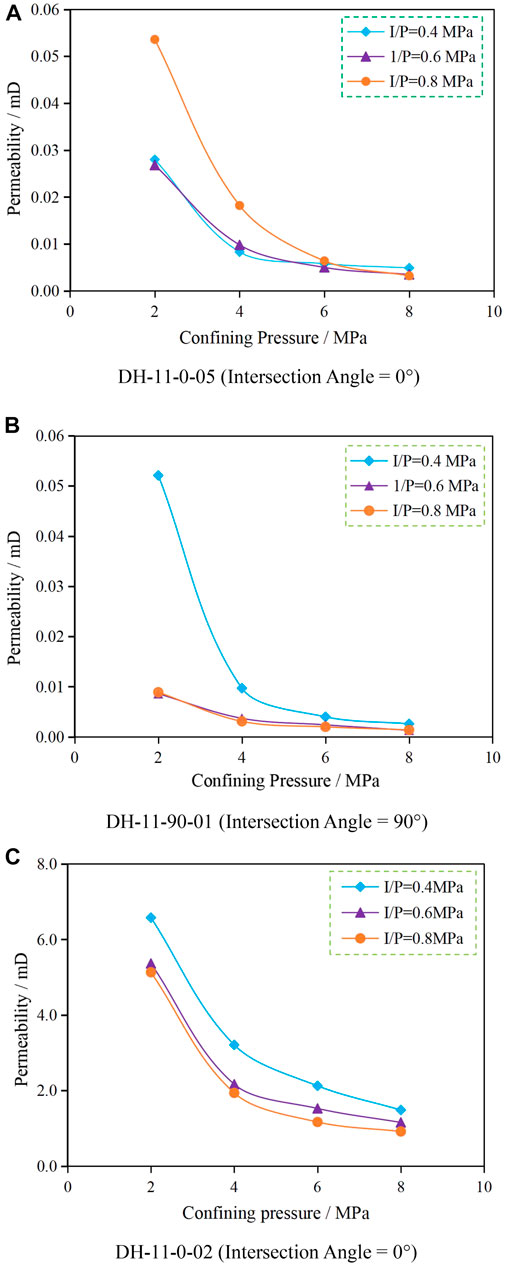
FIGURE 8. Permeability evolution of DH coal samples under different confining pressures and inlet pressures. (A) DH‐11‐0‐05 (Intersection Angle = 0°). (B) DH‐11‐90‐01 (Intersection Angle = 90=). (C) DH‐11‐0‐02 (Intersection Angle = 0°).
2) Coal in WJZ region
This paper studies the permeability evolution of coal in WJZ region under different confining pressures and inlet pressures. The results show that the confining pressure has a significant influence on the permeability of WJZ coal mass. When the confining pressure is increased from 2 to 8 MPa, the permeability decreases by 51.51–94.21%. Among them, the 60° sample (WJZ-11-6-06) shows the smallest decrease, only 47.43%. The 90° sample (WJZ-7-45-04) has the highest decrease rate, which is 13.74%. It should be noted that samples with intersection angles of 0°, 45° and 90° have the same decreasing trend and similar decreasing amplitude (13.74–19.91%). There are several penetrating cracks in the sampleWJZ-11-60-06, but with the increase of confining pressure, the decrease of permeability is limited, which indicates that the confining pressure only leads to partial closure of the crack. This further indicates that even under the action of underground stress, this crack are still the main channel for gas seepage. The inlet gas pressure shows the similar influence on the permeability of WJZ coal is basically the same. Under the same confining pressure, the higher the inlet pressure, the smaller the decrease of permeability. However, compared with confining pressure, the effect of the inlet pressure is very limited. See Figure 9 for the representative permeability test results under different confining pressures in the WJZ region.
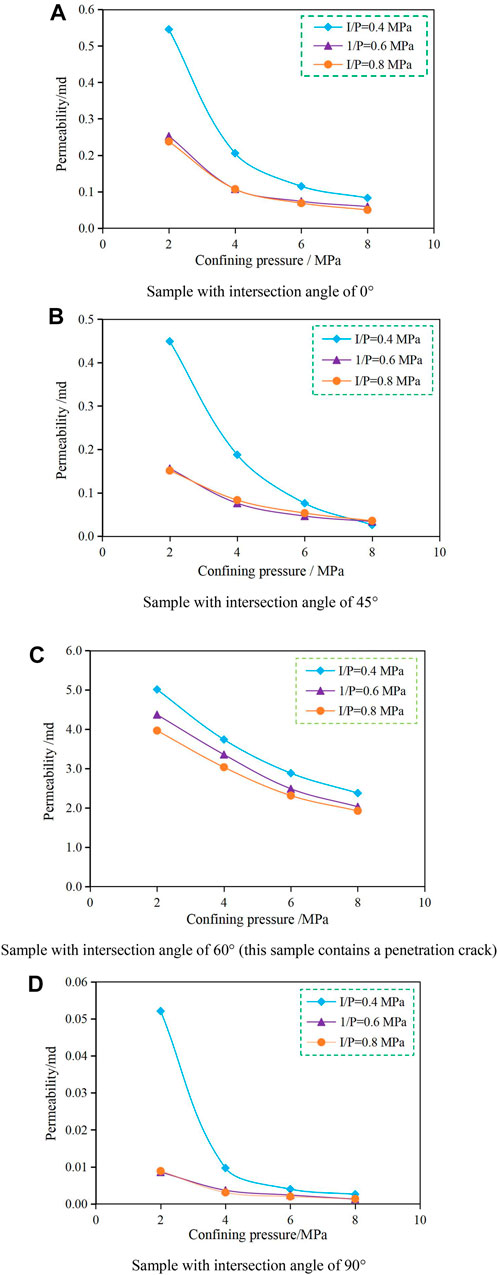
FIGURE 9. Permeability evolution of WJZ coal samples under different confining pressures and inlet gas pressures. (A) Sample with intersection angle of 0° (B) Sample with intersection angle of 45° (C) Sample with intersection angle of 60° (this sample contains a penetration crack) (D) Sample with intersection angle of 90°.
From the above analysis, it can be seen from the above analysis that the confining pressure has a very significant impact on permeability. However, it is also found that after the first test (confining pressure 2-4-6–8 MPa) and the second test (confining pressure 2-4-6–8 MPa), the inlet pressure increased from 0.4 to 0.6 MPa (average pressure increased from 0.25 to 0.35 MPa), and the permeability is lower than that of the first test. It seems that the inlet pressure causes the decrease in permeability. However, from the third round tests results (Confining pressure 2–4–6–8 MPa, Inlet gas pressure 0.8 MPa), it can be seen that the “Permeability-Confining pressure” curves of the seven samples in the second and third rounds are basically coincidental. This does not explain the influence of inlet pressure on coal permeability. As a matter of fact, this phenomenon has an important influence on the field applications.
Generally speaking, from the external conditions, permeability is related to confining pressure, permeation gas pressure and gas type (Meng et al., 2017; Liu et al., 2020c). But from the internal mechanism, these factors essentially lead to the change of pore structure in rock matrix, which reflects the interaction between gas and pore structure, thus leading to the difference of permeability. The Kozeny-Carman equation is a famous theoretical formula for the response of permeability to pore structure and permeability channel characteristics inside rocks, and its revised formula is as follows (Kleinberg et al., 2003; Schneider et al., 2011):
Where:
It can be seen from Eq.6A that the porosity, specific surface area and tortuosity are all important factors affecting permeability. For coal sample, the internal porosity will inevitably decrease when subjected to external force. In the process of rock compression, the micro-pores and cracks are closed, and the total volume decreases, but the total surface area of particles remains unchanged, so (A/V)grain increases instead. The change in tortuosity was not significant. Generally speaking, the larger the confining pressure, the smaller the numerator and the bigger the denominator in Eq.6B. As a result, permeability is reduced. In addition, according to the research of Liu et al. (Liu et al., 2015; Fan et al., 2019; Fan et al., 2020), rocks with flat pores and cracks are more easier to close under pressure. Especially, in the process of increasing confining pressure, the smallest pore throat tends to close at first, so the permeability decreases rapidly in the early stage. Even if the confining pressure is increased, the permeability can not be reduced further. This explains why the permeability is almost unchanged when the confining pressure reaches 8 MPa. However, for samples with perforated cracks, because of the large crack size, the “closing pressure” required for complete crack closure will be very high. Therefore, when the confining pressure is 8 MPa, the decreasing trend of permeability is unchanged.
It should be pointed out that both the DH coal and WJZ coal have low strength and belongs to soft rock. After the first test (Confining pressure is 2–4–6–8 MPa), irreversible plastic deformation occurs in rock matrix (Liu et al., 2015). After entering the second and third tests (confining pressure 2–4–6–8 MPa), the porosity, specific surface area and tortuosity of the rock appear new situations due to the appearance of plastic deformation. Therefore, the “Permeability-Confining pressure” curves of the second round and third round are basically close, but there are also a significant difference from those of the first round. Hydro-fracturing and methane extraction is a process of loading and unloading, which inevitably leads to plastic deformation in coal. Therefore, the permeability of the coal reservoir is likely to be reduced and thus affect the productivity. In this study, the influence of plastic deformation under loading on permeability is only studied by conventional permeability test. In the follow-up study, according to the actual stress of coal and the loading and unloading situation of confining pressure, the downward trend of coal permeability will be further quantified, which will provide a favorable basis for on-site CBM extraction.
Discussion and Application
The coal samples used in this study were obtained from two different blocks of the same coal seam in Dahebian syncline. The plane distance of the coring point is only 8 km, but the porosity and permeability of the coal are quite different. This fully shows that there are obvious differences in physical and mechanical properties of coal in Liupanshui Coalfield. Therefore, even within the same structural area, it is necessary to understand the differences of coal in different blocks, which is the basis for further implementation of CBM exploitation plan.
In the previous exploitation of coal bed methane in Guizhou Province, the harvest was not great, and the commercial exploitation efficiency of coal bed methane has not yet been achieved. However, according to this study, this is mainly due to the complex coal structure, complex of coal occurrence environment, huge heterogeneity, and regional differences of coal in Guizhou Province. At the same time, the preliminary research work is still insufficient. If the characteristics of porosity and permeability of coal seam are not fully understood, the drilling work may be blind. Therefore, it is difficult to obtain commercial CBM extraction. There are still many blocks with high porosity, good permeability and developed primary fractures in coal seams in Guizhou Province (Figure 10). Although these blocks may be isolated in distribution, they may be the best places for methane extraction.
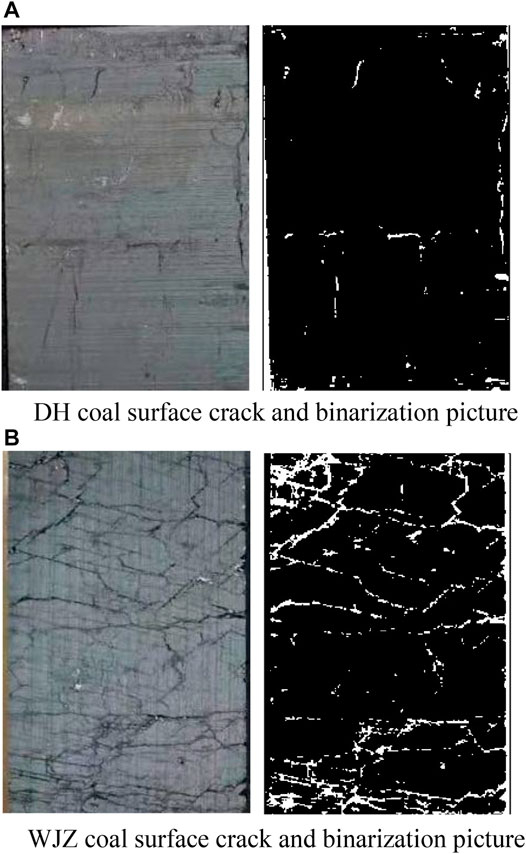
FIGURE 10. Primary fractures and binarization of WJZ coal (size 50 mm × 75 mm). (A) DH coal surface crack and binarization picture (B) WJZ coal surface crack and binarization picture.
From the Dahebian Syncline, the anisotropy and heterogeneity of coal in different areas are very obvious. Especially, the coals in the WJZ region are characterized by high porosity and relatively good permeability. These are the best regions for CBM exploitation, and should be listed as the first exploitation areas. Therefore, in the follow-up studies, even for different areas of the same structural area, it is necessary to explore the optimal blocks, find the areas with high porosity and high permeability (relative), and establish the optimal methane extraction areas. Zhou et al. (Zhou et al., 2009) studied the regional tectonic characteristics of Dahebian syncline. They pointed out that the proportion of reserve faults in WJZ regions is about 18%, and the argillaceous caprock in WZJ region accounts for 35.3%, while the sandstone only accounts for 32.2%. However, in the DH region, the proportion of normal faults in the DH region is as high as 96.9%. And the argillaceous caprock in DH region is as low as 3.57%, but the sandstone accounts for 69%. As a result, due to the higher proportion of reserve faults and tighter caprock, the coal seam in WJZ coal seams have subjected to structural compression, and the stress concentration becomes more obvious in this region. As a result, the integrity of coal seam in WJZ region has been weakened or even destroyed. Therefore, the coal in WJZ region have lower integrity but greater porosity and permeability than those of the DH region.
Due to the great differences in coal petro-physical properties, the methane extraction methods and technical parameters in different regions should also be different. Therefore, the implementation of the specific technologies of blocks may be a better method for CBM exploitation in Guizhou Province. In addition, Guizhou Province may not be suitable for the formation of a unified and standardized CBM exploitation method. It is suggested to study the relationship between the favorable blocks with high porosity and permeability, so as to provide some guidance for the selection of the favorable blocks from a macroscopic perspective.
Conclusions and Prospects
The porosity and permeability of underground coal samples in two blocks of No.11 coal seam in Dahebian Syncline of Liupanshui Coalfield were systematically tested and studied, and the following conclusions and suggestions are drawn.
1) The porosity of coal samples in DH region is 7.4–10.1%, with an average value of 9.2%. The range of permeability k ranges from 0.01 to 0.08 mD, with an average value of 0.04 mD. The permeability of DH coal samples has obvious bedding effects. The DH coal samples has good integrity and poor connectivity of matrix, so it still mainly depends on fracturing to increase productivity.
2) The porosity of the coal samples in WJZ region ranges from 7.06 to 24.8%, with an average of 18.67%. The permeability is between 0.2mD and 5.2mD, with an average of 1.4 mD. There is no obvious relationship between permeability and intersection angle. WJZ coal mass has good porosity and permeability, which is expected to be the optimal choice block for CBM development in the Dahebian Syncline.
3) Relatively speaking, the porosity of WJZ coal is twice that of DH coal, and the permeability is 1–2 orders of magnitude higher than that of DH coal. With the increase of confining pressure, the permeability of coal decreases rapidly, but there is a “Compaction confining pressure”. When this value is exceeded, the permeability will remain basically stable. In addition, with the occurrence of plastic deformation (irrecoverable deformation), the permeability of coal will be greatly decreased. The porosity and permeability of the same coal seam in different positions are quite different, which shows that the coal at different blocks have obvious heterogeneity and differences. Therefore, we should pay close attention to the differences in exploration and development so as to adopt appropriate technology.
4) Generally speaking, when there are cracks in the coal, the permeability will increase greatly. In contrast, the integrity of coal in DH region is better, and the porosity and permeability are lower, while WJZ coal contains more primary cracks, which has higher porosity and permeability. Therefore, when developing CBM similar to the Dahebian Syncline, it is suggested to choose the first dessert area and give priority to CBM development, and pay attention to the application of appropriate technology in different blocks to gradually promote the commercialization and scale of CBM development.
Dahebian Syncline, a representative syncline in Liupanshui Coalfield, has the characteristics of strong porosity-permeability heterogeneity and regional particularity, which must be paid special attention to in the future exploration and development. It also shows that there are still many high permeability blocks in the coalfield. How to grasp the structural characteristics and occurrence characteristics of these dessert areas will provide important guidance for the establishment of commercial CBM base in the next step, which is also the research direction for our further study.
Data Availability Statement
The original contributions presented in the study are included in the article/Supplementary Material, further inquiries can be directed to the corresponding author.
Author Contributions
FZ orgainized and wrote this paper, YW proposed the idea and did the experiments and analysis.
Funding
This research was supported by the Guizhou Provincial Geological Exploration Fund (Grant no. 208-9912-JBN-UTS0) and by the Fundamental Research Funds for the Central Universities (Grant no. 2021CDJQY-030).
Conflict of Interest
The authors declare that the research was conducted in the absence of any commercial or financial relationships that could be construed as a potential conflict of interest.
Publisher’s Note
All claims expressed in this article are solely those of the authors and do not necessarily represent those of their affiliated organizations, or those of the publisher, the editors and the reviewers. Any product that may be evaluated in this article, or claim that may be made by its manufacturer, is not guaranteed or endorsed by the publisher.
References
Aguilera, R. F. (2014). The Role of Natural Gas in a Low Carbon Asia Pacific. Appl. Energ. 113, 1795–1800. doi:10.1016/j.apenergy.2013.07.048
Ayers, W. B. (2002). Coalbed Gas Systems, Resources, and Production and a Review of Contrasting Cases from the San Juan and Powder River Basins. AAPG Bull. 86 (11), 1853–1890. doi:10.1306/61eeddaa-173e-11d7-8645000102c1865d
Fan, J., Jiang, D., Liu, W., Wu, F., Chen, J., and Daemen, J. (2019). Discontinuous Fatigue of Salt Rock with Low-Stress Intervals. Int. J. Rock Mech. Mining Sci. 115, 77–86. doi:10.1016/j.ijrmms.2019.01.013
Fan, J., Liu, W., Jiang, D., Chen, J., Tiedeu, W. N., and Daemen, J. J. K. (2020). Time Interval Effect in Triaxial Discontinuous Cyclic Compression Tests and Simulations for the Residual Stress in Rock Salt. Rock Mech. Rock Eng. 53, 4061–4076. doi:10.1007/s00603-020-02150-y
Huang, H., Sang, S., Yi, T., Xu, H., Li, L., and Li, J. (2010). Analysis of Coal Resource Effective Supply Ability Based on Grey Clustering in Guizhou Province Province. Coal Geology. China 22 (2), 19–23. doi:10.39690.issn.1674-1803.2010.02.05
Kleinberg, R. L., Flaum, C., Griffin, D. D., Brewer, P. G., Malby, G. E., Peltzer, E. T., et al. (2003). Deep Sea NMR: Methane Hydrate Growth Habit in Porous Media and its Relationship to Hydraulic Permeability, Deposit Accumulation, and Submarine Slope Stability. J. Geophys. Research-Solid Earth 108 (B10), 2508. doi:10.1029/2003jb002389
Li, B., Ren, C., Wang, Z., Li, J., Yang, K., and Xu, J. (2020). Experimental Study on Damage and the Permeability Evolution Process of Methane-Containing Coal under Different Temperature Conditions. J. Pet. Sci. Eng. 184, 106509. doi:10.1016/j.petrol.2019.106509
Li, S., Tang, D., Pan, Z., Xu, H., and Guo, L. (2015). Evaluation of Coalbed Methane Potential of Different Reservoirs in Western Guizhou and Eastern Yunnan, China. Fuel 139, 257–267. doi:10.1016/j.fuel.2014.08.054
Li, X., Peng, K., Peng, J., and Xu, H. (2021). Effect of Cyclic Wetting-Drying Treatment on Strength and Failure Behavior of Two Quartz-Rich Sandstones under Direct Shear. Rock Mech. Rock Eng. 54, 5953–5960. doi:10.1007/s00603-021-02583-z
Li, X., Wang, Y., Yang, S., Jun, X., and Zhao, K. (2021). Research Progress in the Mining Technology of the Slowly Inclined, Thin to Medium Thick Phosphate Rock Transition from Open-Pit to Underground Mine. Appl. Maths. Nonlinear Sci. 6 (1), 319–334. doi:10.2478/amns.2021.2.00017
Liang, T., Chai, J., Zhang, Y.-J., Zhang, Z. G., and Zhang, Z. (2019). Refined Analysis and Prediction of Natural Gas Consumption in china. J. Manage. Sci. Eng. 4 (2), 91–104. doi:10.1016/j.jmse.2019.07.001
Lin, B., and Wang, T. (2012). Forecasting Natural Gas Supply in china: Production Peak and Import Trends. Energy Policy 49, 225–233. doi:10.1016/j.enpol.2012.05.074
Liu, E., Guo, B., Lv, L., Qiao, W., and Azimi, M. (2020). Numerical Simulation and Simplified Calculation Method for Heat Exchange Performance of Dry Air Cooler in Natural Gas Pipeline Compressor Station. Energy Sci Eng 8 (6), 2256–2270. doi:10.1002/ese3.661
Liu, E., Lv, L., Yi, Y., and Xie, P. (2019). Research on the Steady Operation Optimization Model of Natural Gas Pipeline Considering the Combined Operation of Air Coolers and Compressors. IEEE ACCESS 7, 83251–83265. doi:10.1109/access.2019.2924515
Liu, H.-H., and Rutqvist, J. (2010). A New Coal-Permeability Model: Internal Swelling Stress and Fracture-Matrix Interaction. Transp Porous Med. 82 (1), 157–171. doi:10.1007/s11242-009-9442-x
Liu, W., Li, Y., Yang, C., Daemen, J. J. K., Yang, Y., and Zhang, G. (2015). Permeability Characteristics of Mudstone Cap Rock and Interlayers in Bedded Salt Formations and Tightness Assessment for Underground Gas Storage Caverns. Eng. Geology. 193, 212–223. doi:10.1016/j.enggeo.2015.04.010
Liu, W., Zhang, X., Fan, J., Li, Y., and Wang, L. (2020). Evaluation of Potential for Salt Cavern Gas Storage and Integration of Brine Extraction: Cavern Utilization, Yangtze River delta Region. Nat. Resour. Res. 29 (5), 3275–3290. doi:10.1007/s11053-020-09640-4
Liu, W., Zhang, X., Fan, J., Zuo, J., Zhang, Z., and Chen, J. (2020). Study on the Mechanical Properties of Man-Made Salt Rock Samples with Impurities. J. Nat. Gas Sci. Eng. 84, 103683. doi:10.1016/j.jngse.2020.103683
Liu, W., Zhang, Z., Chen, J., Fan, J., Jiang, D., Jjk, D., et al. (2019). Physical Simulation of Construction and Control of Two Butted-Well Horizontal Cavern Energy Storage Using Large Molded Rock Salt Specimens. Energy 185, 682–694. doi:10.1016/j.energy.2019.07.014
Liu, W., Zhang, Z., Fan, J., Jiang, D., Li, Z., and Chen, J. (2020). Research on Gas Leakage and Collapse in the Cavern Roof of Underground Natural Gas Storage in Thinly Bedded Salt Rocks. J. Energ. Storage 31, 101669. doi:10.1016/j.est.2020.101669
Liu, X., and He, X. (2017). Effect of Pore Characteristics on Coalbed Methane Adsorption in Middle-High Rank Coals. Adsorption 23 (1), 3–12. doi:10.1007/s10450-016-9811-z
Liu, Y., Yin, G., Li, M., Zhang, D., Deng, B., Liu, C., et al. (2019). Anisotropic Mechanical Properties and the Permeability Evolution of Cubic Coal under True Triaxial Stress Paths. Rock Mech. Rock Eng. 52 (8), 2505–2521. doi:10.1007/s00603-019-01748-1
Men, X. Y., Tao, S., Liu, Z. X., Tian, W. G., and Chen, S. D. (2021). Experimental Study on Gas Mass Transfer Process in a Heterogeneous Coal Reservoir. Fuel Process. Technol. 216, 106779.
Meng, T., You, Y., Chen, J., and Hu, Y. (2017). Investigation on the Permeability Evolution of gypsum Interlayer under High Temperature and Triaxial Pressure. Rock Mech. Rock Eng. 50 (8), 2059–2069. doi:10.1007/s00603-017-1222-2
Meng, Z., Zhang, J., and Wang, R. (2011). In-Situ Stress, Pore Pressure and Stress-dependent Permeability in the Southern Qinshui Basin. Int. J. Rock Mech. Mining Sci. 48 (1), 122–131. doi:10.1016/j.ijrmms.2010.10.003
Nduagu, E. I., and Gates, I. D. (2016). Economic Assessment of Natural Gas Decarbonization Technology for Carbon Emissions Reduction of Bitumen Recovery from Oil Sands. Int. J. Greenhouse Gas Control. 55, 153–165. doi:10.1016/j.ijggc.2016.10.011
Nie, B., Liu, X., Yang, L., Meng, J., and Li, X. (2015). Pore Structure Characterization of Different Rank Coals Using Gas Adsorption and Scanning Electron Microscopy. Fuel 158, 908–917. doi:10.1016/j.fuel.2015.06.050
Qiao, W., Huang, K., Azimi, M., and Han, S. (2019). A Novel Hybrid Prediction Model for Hourly Gas Consumption in Supply Side Based on Improved Whale Optimization Algorithm and Relevance Vector Machine. IEEE ACCESS 7, 88218–88230. doi:10.1109/access.2019.2918156
Qiao, W., Yang, Z., Kang, Z., and Pan, Z. (2020). Short-Term Natural Gas Consumption Prediction Based on Volterra Adaptive Filter and Improved Whale Optimization Algorithm. Eng. Appl. Artif. Intelligence 87, 103323. doi:10.1016/j.engappai.2019.103323
Regan, T., and Chao, Z. (2014). Twenty Five Years of Coal Bed Methane Development in china. The J. World Energ. L. Business 7 (5), 423–447. doi:10.1093/jwelb/jwu030
Schneider, J., Flemings, P. B., Day-Stirrat, R. J., and Germaine, J. T. (2011). Insights into Pore-Scale Controls on Mudstone Permeability through Resedimentation Experiments. Geology 39 (11), 1011–1014. doi:10.1130/g32475.1
Shen, X., Tu, Y., Wang, J., and Chen, J. (2016). Strategic Direction and Policy Suggestions on Promoting Utilization of Natural Gas in China. Int. Pet. Econ. 24 (10), 69–78. doi:10.3969/j.issn.1004-7298.2016.10.010
Tao, S., Chen, S., Tang, D., Zhao, X., Xu, H., and Li, S. (2018). Material Composition, Pore Structure and Adsorption Capacity of Low-Rank Coals Around the First Coalification Jump: A Case of Eastern Junggar Basin, China. Fuel 211, 804–815. doi:10.1016/j.fuel.2017.09.087
Wan, J., Peng, T., Yuan, G., Ban, F., Jurado, M. J., and Xia, Y. (2021). Influence of Tubing/oil-Blanket Lifting on Construction and Geometries of Two-Well-Horizontal Salt Caverns. Tunnelling Underground Space Technol. 108, 103688. doi:10.1016/j.tust.2020.103688
Wang, X., Liu, Y., Wang, F., and Li, H. (2019). Study on CBM Industrial Policy Status Quo in China. Coal Geol. China 31 (12), 102–107. doi:10.3969/j.issn.1674-1803.2019.12.20
Wang, L., Guo, Y., Zhou, J., Yang, H., Yang, C., and Xiao, J. (2021). Rock Mechanical Characteristics of Deep marine Shale in Southern china, a Case Study in Dingshan Block. J. Pet. Sci. Eng. 204, 108699. doi:10.1016/j.petrol.2021.108699
Wang, T., and Lin, B. (2014). Impacts of Unconventional Gas Development on China׳s Natural Gas Production and Import. Renew. Sustain. Energ. Rev. 39, 546–554. doi:10.1016/j.rser.2014.07.103
Xue, Y., Teng, T., Dang, F., Ma, Z., Wang, S., and Xue, H. (2020). Productivity Analysis of Fractured wells in Reservoir of Hydrogen and Carbon Based on Dual-Porosity Medium Model. Int. J. Hydrogen Energ. 45 (39), 20240–20249. doi:10.1016/j.ijhydene.2019.11.146
Yang, D. S., Qi, X. Y., Chen, W. Z., Wang, S. G., and Yang, J. P. (2018). Anisotropic Permeability of Coal Subjected to Cyclic Loading and Unloading. Int. J. Geomech. 18 (8), 04018093. doi:10.1061/(asce)gm.1943-5622.0001229
Yang, Z., Qin, Y., Yi, T., Tang, J., Zhang, Z., and Wu, C. (2019). Analysis of Multi-Coalbed CBM Development Methods in Western Guizhou, China. Geosci. J. 23 (2), 315–325. doi:10.1007/s12303-018-0037-9
Yi, T., Zhang, J., and Li, X. (2007). Development Geology Assessment on Coalbed Methane in Panguan Syncline of Liupanshui Coal Field. Nat. Gas Industry 27 (5), 29–31.
Zhang, X., Liu, W., Jiang, D., Qiao, W., Liu, E., Zhang, N., et al. (2021). Investigation on the Influences of Interlayer Contents on Stability and Usability of Energy Storage Caverns in Bedded Rock Salt. Energy 231, 120968. doi:10.1016/j.energy.2021.120968
Zhang, Z., Jiang, D., Liu, W., Chen, J., Li, E., Fan, J., et al. (2019). Study on the Mechanism of Roof Collapse and Leakage of Horizontal Cavern in Thinly Bedded Salt Rocks. Environ. Earth Sci. 78 (10), 292. doi:10.1007/s12665-019-8292-2
Zheng, M., Li, J., Wu, X., Wang, S., Guo, Q., Yu, J., et al. (2018). China's Conventional and Unconventional Natural Gas Resources: Potential and Exploration Targets. J. Nat. Gas Geosci. 3 (6), 295–309. doi:10.1016/j.jnggs.2018.11.007
Zhong, D., Liu, Y., Kang, X., and Gao, L. (2016). Analysis and the Prevention Advice of Gas Accidents of Guizhou Province Coal Mines 2007-2015. Coal Eng. 48 (11), 69–72.
Zhou, P., Liu, W., and Li, W. (2009). Effect of Dahebian Syncline on Coa1 and Gas Outburst. J. Mining Saf. Eng. 26 (1), 55–59.
Zhou, S. (2015). Briefly Introduce of Coal Bed Methane Resources Estimation. Acta Geologica Sinica - English Edition 89 (s1), 230. doi:10.1111/1755-6724.12304_4
Keywords: permeability, porosity, primary cracks, effect of confining pressure, intersection angle between bedding
Citation: Zhao F and Wei Y (2022) Regional Characteristics of Porosity and Permeability of Dahebian Syncline Coal and Its Application. Front. Earth Sci. 9:822322. doi: 10.3389/feart.2021.822322
Received: 25 November 2021; Accepted: 08 December 2021;
Published: 27 January 2022.
Edited by:
Jie Chen, Chongqing University, ChinaReviewed by:
Jinyang Fan, Chongqing University, ChinaHuabin Zhang, Liaoning Technical University, China
Xianjie Hao, China University of Mining and Technology, China
Copyright © 2022 Zhao and Wei. This is an open-access article distributed under the terms of the Creative Commons Attribution License (CC BY). The use, distribution or reproduction in other forums is permitted, provided the original author(s) and the copyright owner(s) are credited and that the original publication in this journal is cited, in accordance with accepted academic practice. No use, distribution or reproduction is permitted which does not comply with these terms.
*Correspondence: Yuanlong Wei, eXVhbmxvbmd3ZWkxOTg4QDEyNi5jb20=