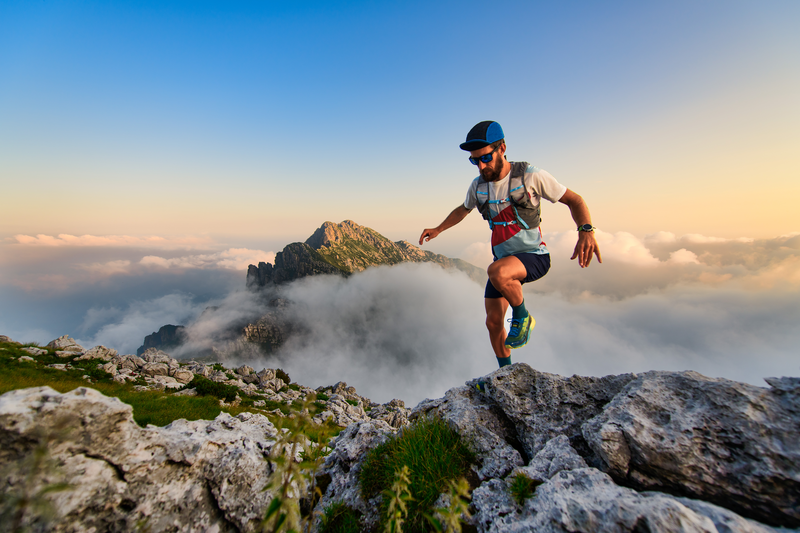
95% of researchers rate our articles as excellent or good
Learn more about the work of our research integrity team to safeguard the quality of each article we publish.
Find out more
ORIGINAL RESEARCH article
Front. Earth Sci. , 10 February 2022
Sec. Economic Geology
Volume 9 - 2021 | https://doi.org/10.3389/feart.2021.818475
This article is part of the Research Topic Advances in the Exploration and Development of Unconventional Oil and Gas: From the Integration of Geology and Engineering View all 32 articles
The potential of microbial carbonate rocks as hydrocarbon source rocks still remains uncertain. To evaluate the hydrocarbon generation and expulsion capability of microbial carbonate rocks, stromatolite obtained from the Xiaganchaigou formation of the western Qaidam Basin has been used for thermocompression experiments to simulate hydrocarbon generation and expulsion at temperature of 280 to 380°C. The results show that the generation potentials of total oil, residual oil, expelled oil, and hydrocarbon gases for stromatolite are 143.39 to 379.99 mg/g total organic carbon (TOC), 47.08 to 223.92 mg/gTOC, 71.12 to 170.81 mg/gTOC, and 6.60 to 101.74 mg/gTOC, respectively. A three-stage model of hydrocarbon generation and expulsion evolution has been constructed for stromatolite. The results show that the first stage is characterized by relatively slow oil generation with associated hydrocarbon gases, having limited capability of oil expulsion in the temperature range of 280 to 360°C (0.50% ≤ Ro ≤ 0.70%). The second stage is characterized by rapid oil generation with associated hydrocarbon gases, having incremental capability of oil expulsion in the temperature range of 320 to 360°C (0.70% ≤ Ro ≤ 1.15%). The third stage is characterized by hydrocarbon gas generation with associated oil, having efficient oil expulsion capability in the temperature range of 360 to 380°C (1.15% ≤ Ro ≤ 1.45%). The experimental data of argillaceous dolostone, gray mudstone, and marlstone, which were reported with the same hydrocarbon generation experimental method, was compared with the data for stromatolite. Compared with traditional source rocks, the oil generation potential of stromatolite is less than that of argillaceous dolostone and gray mudstone but greater than that that of marlstone in the same amount of organic carbon. Especially, the hydrocarbon gas generation potential of stromatolite is approximately double than that of traditional source rocks. Therefore, the experiments indicate that the microbial carbonate rocks in the western Qaidam Basin could be effective source rocks.
The Qaidam Basin is a large continental Meso-Cenozoic intermountain petroliferous basin in the northeastern Qinghai-Tibetan Plateau. It is bounded by the Qilian Mountain to the east, the East Kunlun Mountain to the west, and the Altyn Tagh Mountains to the north (Wu et al., 2013; Pan et al., 2015). The Cenozoic sediments in the basin are great thickness, which recorded the uplift and expansion of the northern Tibetan Plateau since the India–Eurasia collision (Tapponnier et al., 2001; Royden et al., 2008). In addition, the excellent source-reservoir-cap assemblages have been well developed in the Cenozoic sediments in this basin. Therefore, this basin has great potential for oil and gas resources (Zhang et al., 2018). The western Qaidam Basin is adjacent to the Kunlun Mountain in the south and the Altyn Tagh Mountains in the west. It is a depression lake basin formed during the Mesozoic and Cenozoic periods. The western Qaidam Basin is rich in hydrocarbon resources, with more than 20×108 t of oil geological resources and more than 170×104 t/km2 of oil reserves (Fu, 2010; Fu et al., 2016). However, the total organic carbon (TOC) content of source rocks found in the present study is generally in the range of 0.2 to 0.6 wt.%, with an average of less than 0.5 wt.%. Only a few samples with high organic matter abundance have been found (Zhang et al., 2017). This is not consistent with the discovered source rocks and high abundance of oil and gas reserves in the exploration of the western Qaidam Basin.
With the improvement in oil and gas exploration technologies, it has been found that traditional source rocks with high organic carbon content are not the unique hydrocarbon source rocks. Carbonate rocks with low TOC also have the potential to generate hydrocarbons (Yao et al., 2016; Liu W. H. et al., 2017). With their high biological productivity, the microbial carbonate rocks may serve as effective source rocks (Walter, 1994; Xie et al., 2007; Shi et al., 2008). For example, the microbial carbonate rocks in the Main Dolomite Formation deposited in the Permian Zechstein succession in Poland are good source rocks, with original TOC calculated values of 0.8−2.0 wt.% (Słowakiewicz and Mikołajewski, 2011; Słowakiewicz et al., 2013). Furthermore, oil and gas seepages related to bituminous carbonate rocks containing micro-stromatolites have been found in the Mesoproterozoic Gaozhuang Formation and Wumishan Formation in China, which are considered as good source rocks (Liang et al., 1995; Yang et al., 2007). The Cenozoic lacustrine microbial carbonate rocks have been found in the western Qaidam Basin (Zeng et al., 2017), including stromatolites, thrombolites, and dendrolites (Wen et al., 2005; Wang et al., 2020). The stromatolites in the upper part of the Lower Ganchaigou Formation are mainly near horizontal or gently undulating with a thickness of 30–50 cm and a maximum thickness of 4 m. The dark layers in stromatolites are organic enriched layers and have strong luminescence properties under fluorescence microscope (Li et al., 2021).
Since Connan (1974) proposed that temperature and time have complementary effects on hydrocarbon generation of organic matter, laboratory thermal simulation of source rocks has become an important means and basis for hydrocarbon generation evolution and petroleum resource evaluation (Waples, 1980; Horsfield et al., 1992; Behar et al., 1997; Lewan, 1997; Zhao et al., 2005; Le Bayon et al., 2011). To reasonably evaluate the hydrocarbon generation and expulsion capability of microbial carbonate rocks in the western Qaidam Basin, a hydrocarbon generation and expulsion thermocompression simulation instrument was used to model a semi-closed and semi-open system (Shi et al., 2018). The experimental samples were selected from the microbial carbonate rocks in the western Qaidam Basin, and a series of hydrocarbon generation simulation experiments have been carried out based on the burial evolution history of the Miocene strata in the western Qaidam Basin (Liu et al., 2016). With the same simulation experiment method, the hydrocarbon generation potential (GP) and expulsion efficiency of lacustrine argillaceous dolostone (Li et al., 2018), lacustrine gray mudstone (Ma et al., 2012), and marine marlstone (Fu et al., 2017) were previously simulated, and those results were compared with the results of stromatolite. An understanding about these issues is of greatcommercial interest and can lead to improvement in prediction of petroleum reservoirs in microbial carbonate rocks.
The Qaidam Basin is a large Meso-Cenozoic intermountain oil-bearing basin in western China, developed on the pre-Jurassic basement. It has experienced three tectonic evolution stages, including the early fault depression, the middle depression, and the late depocenter shift. Three oil and gas systems have been developed in the north margin of Qaidam Basin, west Qaidam Basin, and east Qaidam Basin on the plane, with three sets of source rocks, including the Middle to Lower Jurassic freshwater lacustrine source rocks, the Paleogene saline lacustrine source rocks, and Quaternary biogas source rocks. The stratigraphic division of the Cenozoic in the western Qaidam Basin is shown in Figure 1 (Liu Z. et al., 2017). It can be seen from Figure 1 that the Cenozoic consists of the Lulehe Formation (E1+2), lower Member of the anchaigou Formation (E31), upper Member of the Xiaganchaigou Formation (E32), Shangganchaigou Formation (N1), Xiayoushashan Formation (N21), Shangyoushashan Formation (N22), Shizigou Formation (N23), and Qigequan Formation (Q). The microbiolites including microbial carbonate rocks with stromatolites, thrombolites, laminites, and dendrolites are well preserved in the upper Member of the Lower Xiaganchaigou Formation (E32), the Shangganchaigou Formation (N1), the Xiayoushashan Formation (N21), and the Shangyoushashan Formation (N22).
FIGURE 1. Structural units of the western Qaidam Basin (A), stratigraphic column of the studied interval (B).
For the hydrocarbon generation and expulsion experiment, the experimental samples should be in the immature to low maturity stage currently, without the experience of a hydrocarbon generation peak in geological history (Ma et al., 2018). In this study, the microbial carbonate sample was taken from well Yue 84 in the western Qaidam Basin with a burial depth of 2,000.98 m. The microbial carbonate was identified as stromatolite (Figure 2). The organic geochemistry parameters of stromatolite are shown in Table 1. The TOC content of the stromatolite samples were measured with a LECO CS200 carbon sulfur analyzer. Rock-Eval 6 was used to analyze the free hydrocarbons present in the rock (S1), hydrocarbons generated by thermal cracking of non-extractable organic matter (S2), and hydrogen index (HI) of the stromatolite samples. The vitrinite reflectance (Ro) value of stromatolite samples indicates that in geological history, no hydrocarbon generation peak occurred for stromatolite samples. It is generally believed that the organic matter abundance of carbonate source rocks is relatively low. Many scholars proposed that the TOC threshold values of organic matter abundance of carbonate rocks for hydrocarbon generation range from 0.1 to 0.3 wt.% (Hunt, 1967; Fu and Liu, 1982; Palacas, 1984; Tissot and Welte, 1984). In the western Qaidam Basin, the content of organic matter in the Paleogene lacustrine carbonate source rocks is low, with the TOC generally below 0.4 wt.%, and the average value is only 0.32 wt.% (Tian et al., 2013). The TOC content of stromatolite (0.288 wt.%) falls within the threshold values of the Paleogene lacustrine carbonate source rocks in the western Qaidam Basin.
Experiments of hydrocarbon generation of stromatolite were conducted using the thermocompression simulation instrument in the Wuxi Research Institute of Petroleum Geology, SINOPEC. The principal structure of the instrument is shown in Figure 3, which is mainly composed of high-temperature and high-pressure reaction system, bidirectional hydraulic control system, automatic collection of hydrocarbon expulsion and fluid supplement system, data acquisition and automatic control system, and peripheral auxiliary equipment (Zheng et al., 2015). The hydrocarbon generation and expulsion simulation experiments on source rocks using this instrument have the following characteristics: 1) Cylindrical samples were used to preserve the original porosity structure, composition, and occurrence of organic matter. 2) The cylinder was sealed in the sample chamber and then placed in the high-temperature autoclave, so the hydrocarbon generation simulation was carried out in a closed system within the pore space of the rock, with almost no excess reaction space. 3) The process of hydrocarbon expulsion uses automatic control high-pressure valve, so it can not only simulate closed hydrocarbon generation under porosity fluid pressure, but also simulate episodic hydrocarbon expulsion under high fluid pressure.
FIGURE 3. Structure diagram of the thermocompression instrument for hydrocarbon generation and expulsion.
Considering the heterogeneity of the source rocks, the samples were crushed to crushed to particles smaller than 0.25 µm in size, fully mixed, and then divided into several portions to ensure the homogeneity and representativeness of the samples. For each simulated temperature point, one portion (about 120 g) was taken and loaded into the sample chamber, and pressed into a small cylinder core sample with a diameter of 3.5 cm under a certain mechanical pressure.
First, the sample chamber with the core sample was installed in the reactor, sealed under pressure, and then the nitrogen of 5–10 MPa was injected to test for leakage. The gas was released until leakage was no longer detected, and the vacuum pump was used to evacuate the instrument. The above operation was repeated for 3 to 5 times so that the instrument was finally pumped into a vacuum state.
The high-pressure pump was used to inject 60–80 MPa of deionized water, so that the pore space of the pressed core sample was completely filled with water (during the process of water absorption of pressed core sample, the fluid pressure will continue to drop; when the fluid pressure of the system does not drop any more, it means that the porosity space has been filled with water). To ensure that the hydrocarbon generation space was filled with high-pressure liquid water during the entire process of hydrocarbon generation and expulsion, the fluid pressure was 2–3 MPa before heating up.
The pressure bar of the bidirectional hydraulic press was started to apply the set static pressure of the core sample for compaction. At the same time, the temperature controller was started so that the heating furnace can rise to the set temperature at a heating rate of 1°C/min. After reaching the set temperature, the temperature was kept constant for 48 h to carry out hydrocarbon generation in limited space.
When the temperature of the whole reaction system had been naturally cooled to 150°C, the hydrocarbon expulsion valves were opened to exhaust the mixture of oil, gas, and water from the hydrocarbon system. Oil, water and gas were separated by a liquid collection tube cooled by liquid nitrogen in which the oil and water mixture was frozen in the collection tube and the gas was collected in a metering tube to measure its volume. The gas composition was analyzed by a gas chromatograph and the amount of each gas substance was calculated.
The expelled oil was the sum of the oil that was expelled from the source rock into the void between the inner wall of the autoclave and the sample chamber and into the liquid-nitrogen cooled trap as well as the oil products in the liquid-nitrogen cooled trap that were frozen after the hydrocarbon expulsion valve was opened when the system had cooled to 150°C.
After weighing the simulated source rock residues, asphalt “A” was extracted with chloroform, which is residual oil. The sum of residual oil and expelled oil is total oil, and the sum of total oil and hydrocarbon gases is total hydrocarbons.
To reveal the process of hydrocarbon generation and expulsion of stromatolite, a series of thermal evolution simulation experiments have been carried out based on the burial evolution history of Paleogens strata in the western Qaidam Basin. During the experiments the corresponding simulation depth, anticipated Ro, formation pressure, and lithostatic pressure were set according to simulation experiment temperature. The specific experimental conditions are listed in Table 2. To obtain the simulation temperature corresponding to the peak of oil generation of the stromatolite, the initial temperature of the simulation experiment was set as 280°C, the maximum temperature was set as 380°C. Hydrocarbon generation experiments were carried out under closed conditions with deionized water. All temperature were raised to the set points at a heating rate of 1°C/min, and then kept at a constant temperature for 48 h for hydrocarbon generation in the limited space. Then, when the temperature had dropped to 150°C, hydrocarbon gases and inorganic gas products were collected. Gas composition was detected by gas chromatograph. The condensate oil expelled with gas was collected using the liquid-nitrogen cooled trap, and light oil was collected by washing reactor and inner wall of pipeline with dichloromethane after the reaction system cooled to room temperature. The combination of gas condensate and light oil is called expelled oil, which is equivalent to the expelled oil in the migration channels under geological conditions. The soluble organic matter obtained by chloroform extraction from the simulated stromatolite is the residual oil. The sum of the expelled oil and residual oil denotes the total oil in the simulation experment, and the sum of the total oil and hydrocarbon gas denotes the total hydrocarbons.
After the simulation of hydrocarbon generation and expulsion, the generated products mainly included hydrocarbon gases, non-hydrocarbon gases, liquid hydrocarbon, and solid residues. The analysis of products and corresponding output was completed by the Wuxi Research Institute of Petroleum Geology, SINOPEC. According to the literature reported (Castelli et al., 1990; Peng et al., 2008; Ross and Bustin, 2009; Eseme et al., 2012; Ma et al., 2012; Fu et al., 2017; Li et al., 2018), the hydrocarbon generation amount per gram TOC of the rock was used to quantify the hydrocarbon generation potential of the stromatolite in these experiments. The generation potentials of expelled oil, residual oil, and hydrocarbon gases of the stromatolite are given in Table 3.
The GPs of total oil, residual oil, and expelled oil with temperature during the formation porosity simulation experiment of for the stromatolite samples are respectively, 143.39 to 379.99 mg/gTOC, 47.08 to 223.92 mg/gTOC, and 71.12 to 170.81 mg/gTOC (shown in Figure 4 and Table 3). The total oil GP curves show that the oil generation process can be roughly divided into three stages: 1) At temperatures of 280–320°C (0.5% ≤ Ro ≤ 0.7%), only a small amount of oil was generated from stromatolite, the total oil GP from stromatolite changed slowly with the rise in temperature, and the total oil GP was about 143 to 168 mg/gTOC. 2) At temperatures of 320–360°C (0.7% ≤ Ro ≤ 1.15%), the total oil GP from the stromatolite increased rapidly with increasing temperature. The peak temperature of total oil GP was at 360°C, and the maximum total oil GP was about 379.99 mg/gTOC. 3) When the temperature exceeded 360°C (1.15% < Ro), the total oil GP from stromatolite decreased with increasing temperature.
A comparison of GPs of total oil, residual oil, and expelled oil for stromatolite shows when the temperature is below 320°C, the GP of expelled oil is lower than that of residual oil, which indicates that under the pore space and formation pressure experimental conditions, the oil expulsion capacity of stromatolite is relatively low before reaching the peak of hydrocarbon generation peak, due to the low oil saturation in the pore space. When the temperature is over 320°C, stromatolite enters into the oil generation window, and the oil GP increases rapidly, so does the expelled oil and residual oil. Although the GP of expelled oil is lower than that of the residual oil, the expelled oil GP increases more than that of the residual oil. Moreover, when the temperature is over 340°C, the expelled oil GP continues to increase, whereas the residual oil GP first enters a stable plateau and then decreases rapidly. The petroleum expulsion efficiency is a key parameter to evaluate the conventional and unconventional oil and gas resources (Cooles et al., 1986; Pepper and Corvi, 1995; Ritter, 2003; Eseme, 2007; Eseme et al., 2012; Liu et al., 2022). Cooles et al. (1986) defined the petroleum expulsion efficiency as a measure of the extent of expulsion from a given source rock section. In a similar manner, the oil expulsion efficiency indicates the extent of oil expulsion from source rock. According to the experiments, the oil expulsion efficiency (ratio of the expelled oil GP to the total oil GP, expressed as a percentage) of stromatolite is over 50% and reaches 68% at 380°C.
According to the gas component analyses, the hydrocarbon gases generated from the stromatolite simulation experiment are rich in composition, mainly containing C1 to C5+, and a small amount of olefin gases (Table 4).
Figure 5 shows the evolution characteristics of hydrocarbon gas GP from stromatolite in the thermocompression simulation experiment. Combined with the data in Table 3, it can be seen that within the experimental temperature range, the hydrocarbon gas GP increased with the increase of temperature, with a slow increase at first and then a rapid increase. The hydrocarbon gas GP increased from 6.60 to 101.74 mg/gTOC. With the increase in temperature, the ratio of hydrocarbon gas GP to total hydrocarbon GP gradually increased, reaching 23.5% at 380°C.
The group components of oil generated by the simulation of stromatolite were analyzed by column chromatography, and the data are shown in Table 5. During the whole process of hydrocarbon generation simulation, the group component of the residual oil and expelled oil obtained from stromatolite are characterized by high contents of non-hydrocarbon and asphaltene, and low contents of saturated hydrocarbon and aromatic hydrocarbon, in which the saturated hydrocarbon or aromatic hydrocarbon is about half of the non-hydrocarbon or asphaltene. Under the same thermal simulation method, the oil group compositions obtained from marlstone samples (Fu et al., 2017) also have high contents of asphaltene and non-hydrocarbon components, and the asphaltene content is mainly in the range of 20–50%. However, the expelled oil and residual oil obtained by conventional simulation are generally characterized by high contents of aromatic hydrocarbon, saturated hydrocarbon and non-hydrocarbon, and low content of asphaltene. Through comparison, it can be seen that different hydrocarbon generation simulation methods may lead to different crude oil group compositions of source rocks. The characteristic of oil group compositions of stromatolite with hydrocarbon and asphaltene in the high and low saturated hydrocarbon and aromatic hydrocarbon shows that the existence of high-pressure and high-temperature compressed water in thermocompression simulation experiments promotes the occurrence of “depolymerizing” hydrocarbon generation mode during the hydrocarbon generation process of stromatolite, and more soluble intermediate products was generated, such as asphaltene and colloid, improving the generation of “oil”, so that the content of asphaltene and non-hydrocarbon in the group compositions of crude oil is relatively high.
The triple stages of hydrocarbon generation and expulsion evolution model has been constructed for stromatolite. The results show that the first stage is characterized by relatively slow oil generation, with associated hydrocarbon gases, having limited capability of oil expulsion when the temperature is in the range of 280320°C (0.50% ≤ Ro ≤ 0.70%). The second stage is characterized by rapid oil generation with associated hydrocarbon gases, having incremental capability of oil expulsion when temperature is in the range of 320–360°C (0.70% ≤ Ro ≤ 1.15%). The third stage is characterized by hydrocarbon gas generation with associated oil, having efficient oil expulsion capability when the temperature is in the range of 360–380°C (1.15% ≤ Ro ≤ 1.45%).
The hydrocarbon generation potential and expulsion efficiency of microbial carbonate rocks have been characterized by comparing the experimental results of argillaceous dolostone (Li et al., 2018), gray mudstone (Ma et al., 2012), and marlstone (Fu et al., 2017) using the same simulation experiment, in combination with geochemical analysis data of the hydrocarbon gases and crude oil products of hydrocarbon generation simulation. Stromatolite, argillaceous dolostone, and gray mudstone are lacustrine rock samples, and marlstone is a marine rock sample. The argillaceous dolostone was taken from Well Zha-1 of Chuanjing Depression in western margin of Erlian Basin with a burial depth of 788.8m, TOC = 3.45 wt.%, S1 = 0.51 mg/g, S2 = 17.41 mg/g, HI = 505 mg/g, and Ro = 0.56%. The gray mudstone was taken from Well Bi-215 in Biyang Sag, with a burial depth of 1,523.0 m, with TOC = 2.64 wt.%, S2 = 15.83 mg/g, HI = 600 mg/g, and Ro = 0.38%. Marlstone was taken from D2 layer of Maoshan section in Luquan County, TOC = 3.33 wt.%, S1 = 1.11 mg/g, S2 = 13.9 mg/g, HI = 403 mg/g, and Ro = 0.42%.
Through the same thermocompression simulation experiment, the comparison results of total oil generation amount per gram TOC of the four lithologic samples are shown in Figure 6. Compared with the experimental data of total oil generation, with the increase of temperature, the total oil GP of the four lithologic samples increased first and then decreased. In the temperature range from 280 to 340°C, which was at low maturity stage, the total oil GP of the stromatolite was higher than that of gray mudstone and marlstone, although lower than that of the argillaceous dolostone. The total oil in the experimental results of stromatolite was 1.5–2.5 times that of gray mudstone, which may indicate that the hydrocarbon generation stage of the carbonate source rock was earlier than that of the gray mudstone. When the simulated temperature exceeded 320°C, the total oil GP of the four lithology samples increased rapidly. When the temperature reached 360°C or 370°C, the stromatolite, argillaceous dolostone, gray mudstone, and marlstone reached the oil generation peak, and the maximum total oil GP values were 379.99 mg/gTOC, 574.49 mg/gTOC, 611.11 mg/gTOC, and 392.56 mg/gTOC, respectively.
There are two stages in the relationship between the characteristics of residual oil generation amount per gram TOC of the four lithologic samples and temperature, as shown in Figure 7: 1) In the temperature range of 280–320°C, the residual oil GP of the four lithologic samples has little change with the increase of temperature, and the residual rate (the percentage value of residual oil production rate to the total oil production rate) was high. The maximum residual rate was 65.8% for stromatolite, 92.7% for argillaceous dolostone, 83.9% for gray mudstone, and 86.2% for marlstone. 2) In the temperature range of 320–380°C, the residual oil GP of the four lithologies increased first and then decreased with the increase of temperature, and the residual oil GP of stromatolite were much lower than that of gray mudstone and marlstone.
As shown in Figure 8, in the temperature range of 280–380°C, the expelled oil GP of stromatolite is higher than that of argillaceous dolostone, gray mudstone, and marlstone, and the expelled oil GPs of four lithologies all increases with the increase of temperature. The temperature at which the expelled oil GPs of stromatolite, argillaceous dolostone, gray mudstone, and marlstone increased rapidly were respectively at 320°C, 340°C, and 350°C. When the temperature reached 380°C, the expelled oil GPs of gray mudstone and marlstone approached that of stromatolite. In the temperature range from 280 to 380°C, the oil expulsion efficiency of stromatolite, argillaceous dolostone, and gray mudstone were about 34–68%, 8.2–70.6%, and 10.7–36.5%, respectively. The oil expulsion efficiency of stromatolite, argillaceous dolostone, and marlstone increased with the increase of temperature, but the oil expulsion efficiency of gray mudstone decreased first and then increased with the increase of temperature. The oil expulsion efficiency of marlstone was 13.8–63.2%. According to the residual oil GP, the expelled oil GP, and oil expulsion efficiency of these four kinds of lithologies, the oil expulsion capacity of stromatolite and argillaceous dolomite was higher than that of gray mudstone and marlstone. The main reason may be that the stromatolite and argillaceous dolomite have relatively fewer argillaceous components than that of argillaceous source rock. Therefore, the oil adsorption capacity of stromatolite and argillaceous dolomite is relatively weaker than that of argillaceous source rock, and the oil expulsion capacity of stromatolite and argillaceous dolomite is relatively stronger their oil adsorption capacity is relatively weak and the oil expulsion capacity is relatively stronger. The simulation results show that the oil expulsion efficiency of source rocks cannot be inferred simply from the type or abundance of organic matter.
The evolution comparison result of hydrocarbon gas GP of stromatolite, argillaceous dolostone, gray mudstone, and marlstone is shown in Figure 9, from which it can be seen that in the temperature range of 280–380°C, the hydrocarbon gas GP of four lithologies almost all increase with the increase of the degree of thermal evolution, but hydrocarbon gas GP of stromatolite is obviously the highest. The hydrocarbon gas GP of stromatolite is about 1.8–4.7 times that of argillaceous dolomite, 1.8 to 11 times that of gray mudstone, and 20 times that of marlstone The comparison of the simulation results of the four lithologies shows that stromatolite has a higher hydrocarbon gas GP than that of the traditional argillaceous source rock with the same amount of organic carbon. At present, the exploration of deep oil and gas mainly focuses on searching for natural gas, and the microbial carbonate rocks have higher hydrocarbon gas GP, making them favorable natural gas source rocks. Therefore, the potential of microbial carbonate rocks as natural gas source rocks should be emphasized in deep natural gas exploration.
By comparing the oil generation amount per gram TOC of stromatolite with argillaceous dolostone, gray mudstone, and marlstone, three points can be made about the characteristics of oil generation potential and expulsion efficiency of stromatolite: 1) Stromatolite has the same evolution path of hydrocarbon generation with argillaceous dolostone, gray mudstone, and marlstone. Thus, the total oil GPs of all four lithologic samples exhibit an initial increase followed by a decrease with the increase in temperature. 2) Stromatolite, argillaceous dolostone, and marlstone entered the oil generation window earlier than gray mudstone. 3) The oil expulsion efficiency of stromatolite is higher than that of gray mudstone and marlstone.
In this paper, a series of thermal evolution simulations from low maturity to high have been carried out for microbial carbonate rocks , using stromatolite as an example. The effects of temperature, lithostatic pressure, fluid pressure, and reaction space on hydrocarbon generation have been considered in the experimental conditions. The reaction conditions are close to geological environment. The data of oil generation potential and hydrocarbon gas generation potential of stromatolite have been obtained, and the maximum oil generation potential is 379.99 mg/gTOC. Peng et al. (2008) indicated that the maximum liquid hydrocarbon generation potential of type Ⅰ kerogen of marine carbonate rocks was 325.77 mg/gTOC, that of type Ⅱ kerogen was 235.84 mg/gTOC, and that of type Ⅲ kerogen was 56.04 mg/gTOC. In contrast, in this study, the capability of stromatolite to generate liquid hydrocarbon is equivalent to that of type kerogen in marine carbonate rocks. Through the same formation porosity simulation experiment, the maximum hydrocarbon generation potential of the four lithologies of stromatolite, argillaceous dolostone, gray mudstone, and marlstone was 470.59 mg/gTOC, 574.45 mg/gTOC, 611.11 mg/gTOC, and 450.10 mg/gTOC, respectively. The maximum hydrocarbon gas generation potential was 101.74 mg/gTOC, 57.76 mg/gTOC, 49.60 mg/gTOC, and 57.48 mg/gTOC, and the maximum oil expulsion efficiency was 68%, 71%, 36%, and 63%, respectively. According to the hydrocarbon generation simulation results of the four samples with the same amount of organic carbon, the maximum hydrocarbon generation potential of stromatolite is lower than that of the lacustrine argillaceous source rock, but higher than that of marine argillaceous carbonate source rock. In addition, stromatolite has higher hydrocarbon gas generation potential. The thermal evolution simulation results show that in western Qaidam Basin, the microbial carbonate rocks with stromatolite are effective hydrocarbon source rocks, especially natural gas source rocks.
Compared with the results of hydrocarbon generation and expulsion of stromatolite, argillaceous dolostone, gray mudstone, and marlstone in formation porosity simulation experiment, there are two differences between microbial carbonate rocks and traditional mudstone source rocks. First, the organic carbon content of stromatolite used in this hydrocarbon generation simulation experiment is low. Under the same TOC content as the traditional mudstone source rocks, stromatolite still has high oil generation potential and even higher hydrocarbon gas generation potential, so the hydrocarbon generation capacity of the microbial carbonate rocks cannot be eliminated because of the low organic carbon content. Second, microbial carbonate rocks have higher oil expulsion efficiency. In addition, microbial carbonate rocks themselves are good reservoirs, so the petroleum does not have to be migrated over long distances, but is mostly migrated over short distances or not migrated out of the source rocks and then accumulate into reservoirs.
To determine whether microbial carbonate rocks in the western Qaidam Basin are effective source rocks, the thermocompression simulation of hydrocarbon generation and expulsion of stromatolite has been performed under geological constraints in the present work. Moreover, the data from stromatolite have been compared with the experimental results of hydrocarbon generation and expulsion of argillaceous dolostone, marlstone, and gray mudstone. Overall, the following three understandings have been obtained with the database available in this study.
1) During the stage from 280 to 380°C, the generation potentials of total oil, residual oil, expelled oil, and hydrocarbon gases from stromatolite are 143.39 to 379.99 mg/gtotal organic carbon (TOC), 47.08 to 223.92 mg/gTOC, 71.12 to 170.81 mg/gTOC, and 6.60 to101.74 mg/gTOC, respectively.
2) The triple stages of hydrocarbon generation and expulsion evolution model has been constructed for stromatolite. The results show that the first stage is characterized by relatively slow oil generation with associated hydrocarbon gases, having limited capability of oil expulsion when the temperature is in the range of 280–320°C (0.50% ≤ Ro ≤ 0.70%). The second stage is characterized by rapid oil generation with associated hydrocarbon gases, having incremental capability of oil expulsion when temperature is in the range of 320–360°C (0.70% ≤ Ro ≤ 1.15%). The third stage is characterized by hydrocarbon gas generation, with associated oil, having efficient oil expulsion capability when the temperature is in the range of 360–380°C (1.15% ≤ Ro ≤ 1.45%).
3) The maximum hydrocarbon generation potential of the stromatolite is lower than that of lacustrine argillaceous source rocks, but higher than that of marine argillaceous carbonate source rocks. Stromatolite has higher hydrocarbon gas generation potential. The thermocompression simulation shows that the microbial carbonate rocks in the western Qaidam Basin can be effective hydrocarbon source rocks, especially the natural gas source rocks.
The original contributions presented in the study are included in the article/Supplementary Material, further inquiries can be directed to the corresponding author.
MS contributed as the major author of the article. AH and JL conceived the project and analyzed the samples. YL and WC collected the samples. LZ did the thermocompression experiment. All authors contributed to the article and approved the submitted version.
The authors declare that this study received funding from PetroChina. The funder had the following involvement in the study: study design and sample collection.
MS, AH, YL, and WC were employed by company Petrochina. Author LZ was employed by company SINOPEC.
The remaining authors declare that the research was conducted in the absence of any commercial or financial relationships that could be construed as a potential conflict of interest.
All claims expressed in this article are solely those of the authors and do not necessarily represent those of their affiliated organizations, or those of the publisher, the editors, and the reviewers. Any product that may be evaluated in this article, or claim that may be made by its manufacturer, is not guaranteed or endorsed by the publisher.
Behar, F., Vandenbroucke, M., Tang, Y., Marquis, F., and Espitalie, J. (1997). Thermal Cracking of Kerogen in Open and Closed Systems: Determination of Kinetic Parameters and Stoichiometric Coefficients for Oil and Gas Generation. Org. Geochem. 26 (5/6), 321–339. doi:10.1016/S0146-6380(97)00014-4
Castelli, A., Chiaramonte, M. A., Beltrame, P. L., Carniti, P., Del Bianco, A., and Stroppa, F. (1990). Thermal Degradation of Kerogen by Hydrous Pyrolysis. A Kinetic Study. Org. Geochem. 16 (1–3), 75–82. doi:10.1016/0146-6380(90)90027-W
Connan, J. (1974). Time-temperature Relation in Oil Genesis.. AAPG Bull.58 (12), 2516–2521. doi:10.1306/83D91BEB-16C7-11D7-8645000102C1865D
Cooles, G. P., Mackenzie, A. S., and Quigley, T. M. (1986). Calculation of Petroleum Masses Generated and Expelled from Source Rocks. Org. Geochem. 10, 235–245. doi:10.1016/0146-6380(86)90026-4
Eseme, E., Krooss, B. M., and Littke, R. (2012). Evolution of Petrophysical Properties of Oil Shales during High-Temperature Compaction Tests: Implications for Petroleum Expulsion. Mar. Pet. Geology. 31, 110–124. doi:10.1016/j.marpetgeo.2011.11.005
Fu, J. M., and Liu, D. H. (1982). Some Characteristics of the Evolution of Organic Matter in Carbonite Formation. Acta Petrol. Sinica 1, 1–9. [in Chinese, with English abstract].
Fu, S. T. (2010). Key Controlling Factors of Oil and Gas Accumulation in the Western Qaidam Basin and its Implications for Favorable Exploration Direction[J]. Acta Sedimentologica Sinica 28 (2), 373–379. doi:10.14027/j.cnki.cjxb.2010.02.019
Fu, S. T., Ma, D. D., Chen, Y., Zhang, G. Q., and Wu, K. Y. (2016). New advance of Petroleum and Gas Exploration in Qaidam Basin. Acta Petrolei Sinica 37 (S1), 1–10. doi:10.7623/syxb2016s1001
Fu, X. D., Qin, J. Z., Yao, G. S., Zheng, L. J., Zou, W. H., and Tenger, W. X. F. (2017). The Comparison of Hydrocarbon Generation and Evolution Characteristics between Two Temperature-Pressure Simulation Systems and its Geological Significance for Deep Reservoir Exploration. Geochimica 46, 262–275. [in Chinese, with English abstract].
Horsfield, B., Schenk, H. J., Mills, N., and Welte, D. H. (1992). An Investigation of the In-Reservoir Conversion of Oil to Gas: Compositional and Kinetic Finding from Closed-System Programmed-Temperature Pyrolysis. Org. Geochem. 19 (1-3), 191–204. doi:10.1016/0146-6380(92)90036-W
Hunt, J. M. (1967). “The Origin of Petroleum in Carbonate Rocks[C],” in Carbonate Rocks. Editors G V Chilingar, H J Biessll, and R W Fairbridge (New York: Elsevier), 225–251.
Le Bayon, R., Brey, G. P., Ernst, W. G., and Mählmann, R. F. (2011). Experimental Kinetic Study of Organic Matter Maturation: Time and Pressure Effects on Vitrinite Reflectance at 400°C. Org. Geochem. 42 (4), 340–355. doi:10.1016/j.orggeochem.2011.01.011
Lewan, M. D. (1997). Experiments on the Role of Water in Petroleum Formation. Geochimica et Cosmochimica Acta 61 (17), 3691–3723. doi:10.1016/S0016-7037(97)00176-2
Li, X., Wang, J. G., Li, F., Wang, Y. L., Wu, K. Y., Li, Y. F., et al. (2021). Sedimentary Characteristics of Eocene Lacustrine Microbialites in Western Qaidam Basin: A Case Study from Xiaganchaigou Formation in Xichagou and Liangdong Areas. Lithologic Reservoirs 33 (3), 63–73. doi:10.12108/yxyqc.20210306
Li, Z. M., Zheng, L. J., Jiang, Q. G., Ma, Z. L., Tao, G. L., Xu, E. S., et al. (2018). Simulation of Hydrocarbon Generation and Expulsion for Lacustrine Organic-Rich Argillaceous Dolostone and its Implications for Shale Oil Exploration. Earth Science-Journal China Univ. Geosciences 43, 566–576. doi:10.3799/dqkx.2018.025
Liang, Y. Z., Zhu, S. X., Gao, Z. J., Du, R. L., and Qiu, S. Y. (1995). New Progress in the Study of Stratomalites—Microbialite. Regional Geology. China 1, 57–65. [in Chinese, with English abstract].
Liu, J., Liu, C. L., Dong, Q. Y., Sun, P., Zhang, L., and Guo, Z. Q. (2016). A Study of the Period of the Gas Accumulation of Paleogene-Neogene in Western Qaidam Basin. Earth Sci. Front. 23, 127–134. doi:10.13745/j.esf.2016.05.014
Liu, Q., Li, P., Jin, Z., Sun, Y., Hu, G., Zhu, D., et al. (2022). Organic-rich Formation and Hydrocarbon Enrichment of Lacustrine Shale Strata: A Case Study of Chang 7 Member. Sci. China Earth Sci. 52 (2), 270–290. doi:10.1007/s11430-021-9819-y
Liu, W. H., Tenger, B., Wang, X. F., Li, M. W., Hu, G., Wang, J., et al. (2017a). New Knowledge of Hydrocarbon Generating Theory of Organic Matter in Chinese marine Carbonates. Pet. Exploration Dev. 41 (1), 155–164. doi:10.11698/PED.2017.01.19
Liu, Z., Zhu, C., Li, S., Xue, J., Gong, Q., Wang, Y., et al. (2017b). Geological Features and Exploration fields of Tight Oil in the Cenozoic of Western Qaidam Basin, NW China. Pet. exploration Dev. 44 (2), 217–225. doi:10.1016/S1876-3804(17)30024-1
Ma, Z. L., Zheng, L. J., and Li, Z. M. (2012). The Thermocompression Simulation experiment of Source Rock Hydrocarbon Generation and Expulsion in Formation Porosity. Acta Sedimentologica Sinica 30, 955–963. doi:10.14027/j.cnki.cjxb.2012.05.006
Ma, Z. L., Zheng, L. J., Yu, X. L., Zhao, Z. X., and Li, Z. M. (2018). Effective Oil Expulsion Threshold of Argillaceous Source Rocks and Geological Significance of Shale Oil. J. China Univ. Pet. (Edition Nat. Science) 42 (1), 32–39. doi:10.3969/J.ISSN.1673-5005.2018.01.004
Palacas, J. G. (1984). Petroleum Geochemistry and Source Rocks Potential of Carbonate Rocks (AAPG Studies in Geology). Tulsa: AAPG, 71–96.
Pan, J. W., Li, H. B., Sun, Z. M., Liu, D. L., Wu, C., and Yu, C. Q. (2015). Tectonic Responses in the Qaidam Basin Induced by Cenozoic Activities of the Altyn Tagh Fault[J]. Acta Petrologica Sinica 31 (12), 3701–3712. [in Chinese, with English abstract].
Peng, P. A., Liu, D. Y., Qin, Y., Yu, C. L., Zhang, S. W., Sui, F. G., et al. (2008). Low Limits of Organic Carbon Content in Carbonate as Oil and Gas Source Rocks. Geochimica 3 (4), 415–422. [in Chinese, with English abstract].
Pepper, A. S., and Corvi, P. J. (1995). Simple Kinetic Models of Petroleum Formation. Part III: Modelling an Open System. Mar. Pet. Geology. 12 (4), 417–452. doi:10.1016/0264-8172(95)96904-5
Ritter, U. (2003). Solubility of Petroleum Compounds in Kerogen: Implications for Petroleum Expulsion. Org. Geochem. 34, 319–326. doi:10.1016/S0146-6380(02)00245-0
Ross, D. J. K., and Marc Bustin, R. (2009). The Importance of Shale Composition and Pore Structure upon Gas Storage Potential of Shale Gas Reservoirs. Mar. Pet. Geology. 26, 916–927. doi:10.1016/j.marpetgeo.2008.06.004
Royden, L. H., Burchfiel, B. C., and Van der Hilst, R. D. (2008). The Geological Evolution of the Tibetan Plateau. Science 321 (5892), 1054–1058. doi:10.1126/science.1155371
Shi, M., Yu, B., Zhang, J., Huang, H., Yuan, Y., and Li, B. (2018). Evolution of Organic Pores in marine Shales Undergoing Thermocompression: a Simulation experiment Using Hydrocarbon Generation and Expulsion. J. Nat. Gas Sci. Eng. 59, 406–413. doi:10.1016/j.jngse.2018.09.008
Shi, X. Y., Zhang, C. H., Jiang, G. Q., Liu, J., Wang, Y., and Liu, D. B. (2008). Microbial Mats from the Mesoproterozoic Carbonates of the north China Platform and Their Potential for Hydrocarbon-Generation. Geoscience 22, 669–682. [in Chinese, with English abstract].
Słowakiewicz, M., and Mikołajewski, Z. (2011). Upper Permian Main Dolomite Microbial Carbonates as Potential Source Rocks for Hydrocarbons (W Poland). Mar. Pet. Geology. 28 (8), 1572–1591. doi:10.1016/j.marpetgeo.2011.04.002
Słowakiewicz, M., Tucker, M. E., Pancost, R. D., Perri, E., and Mawson, M. (2013). Upper Permian (Zechstein) Microbialites: Supratidal through Deep Subtidal Deposition, Source Rock, and Reservoir Potential. Bulletin 97 (11), 1921–1936. doi:10.1306/06181312179
Tapponnier, P., Zhiqin, X., Roger, F., Meyer, B., Arnaud, N., Wittlinger, G., et al. (2001). Oblique Stepwise Rise and Growth of the Tibet Plateau. Science 294 (5547), 1671–1677. doi:10.1126/science.105978
Tian, C. T., Ma, S. P., Yang, Y., Meng, J., and Xia, Y. Q. (2013). Comparison of Hydrocarbon Generation Conditions between Lacustrine and marine Carbonate Source Rocks. Oil Gas Geology. 35, 336–341. doi:10.11743/ogg201406
Tissot, B. P., and Welte, D. H. (1984). Petroleum formation and occurrence (second revised and enlarged edition). Berlin: Springer Verlag, 160–198. doi:10.1007/978-3-642-87813-8_10
Walter, M. R. (1994). “Stromatolites: The Main Geological Source of Information on the Evolution of Early Benthos,” in Early Life on Earth. Editor S Bengtson (New York: Columbia U. P.), 270–286.
Wang, J. G., Zhang, D. W., Bai, Y. D., Sun, X. J., Li, X., Wang, A. P., et al. (2020). Saline Lacustrine Palustrine Sediments and Microbialite in the Upper Youshashan Formation in the Western Qaidam basin. Geologica Sinica 94 (11), 3228–3248. doi:10.19762/j.cnki.dizhixuebao.2020169
Waples, D. (1980). Time and Temperature in Petroleum Formation: Application of Lopatin's Method to Petroleum Exploration. Bulletin 64 (6), 916–926. doi:10.1306/2F9193D2-16CE-11D7-8645000102C1865D
Wen, Z. F., Zhong, J. H., Zhang, Y. Z., Guo, Z. Q., Liu, Y. T., and Li, Y. (2005). Distribution Characteristics of Reef Reservoir in the Western Qaidam Basin. Acta Petrologica Sinica 26 (6), 30–35. [in Chinese, with English abstract].
Wu, C., Yan, C. F., Li, H. B., Sun, Z. M., Liu, D. L., Yu, C. Q., et al. (2013). Cenozoic Tectonic Evolution of the Western Qaidam Basin and its Constrain on the Growth of the Northern Tibetan Plateau. Acta Petrologica Sinica 29 (6), 2211–2222. [in Chinese, with English abstract].
Xie, S. C., Yin, H. F., Xie, X. N., Qin, J. Z., Shi, X. Y., Hu, C. Y., et al. (2007). On the Geobiological Evaluation of Hydrocarbon Source Rocks. Earth Science-Journal China Univ. Geosciences 32, 727–740. [in Chinese, with English abstract].
Yang, H., Wang, Y. B., Chen, L, and Dong, M. (2007). Calci-microbialite as a Kind of Potential Hydrocarbon Source Rock and its Geomicrobiological Processes. Earth Science-Jounal China Univ. Geosciences 32, 797–802. [in Chinese, with English abstract].
Yao, J. L., Wang, C. C., Chen, J. P., Gao, G., Wang, Y. F., Li, X. F., et al. (2016). Distribution Characteristics of Subsalt Carbonate Source Rocks in Majiagou Formation, Ordos Basin[J]. Nat. Gas Geosci. 27 (12), 2115–2126. doi:10.11764/j.issn.1672-1926.2016.12.2115
Zeng, L. Q., Yi, H. S., Xia, G. Q., and Yuan, T. (2017). Sedimentary Sequences and Implications for Paleoenvironment of Cenozoic Lacustrine Stromatolites, Qaidam Basin. Geoscience 31 (6), 1251–1260. [in Chinese, with English abstract].
Zhang, B., He, Y. Y., Chen, Y., Meng, Q. Y., and Yuan, L. (2017). Geochemical Characteristics and Oil Accumulation Significance of the High Quality saline Lacustrine Source Rocks in the Western Qaidam Basin, NW China. Acta Petrolei Sinica 38 (10), 1158–1167. doi:10.7623/syxb201710006
Zhang, Y. S., Wu, K. Y., Jiang, Y. H., Wang, P., Cai, Z. H., Gao, F. R., et al. (2018). Geological Characteristics of Deep Carbonate Hydrocarbon-Bearing Pool in the Western Yingxiongling Area in Qaidam Basin. Nat. Gas Geosci. 29 (3), 358–369. doi:10.11764/j.issn.1672-1926.2018.02.005
Zhao, Z., Zhong, N. N., and Hong, Z. L. (2005). Pattern of Pressurization from Hydrocarbon Generation in Carbonate Source Rocks and its Significance. Oil Gas Geology. 26 (3), 344–355. [in Chinese, with English abstract].
Keywords: lacustrine microbiolites, hydrocarbon generation and expulsion, oil generation potential, hydrocarbon gas generation potential, Qaidam Basin
Citation: She M, Hu A, Lv Y, Chen W, Liang J and Zheng L (2022) Hydrocarbon Generation Potential of Microbial Carbonate in the Western Qaidam Basin, China: Implication From Thermocompression Experiment. Front. Earth Sci. 9:818475. doi: 10.3389/feart.2021.818475
Received: 19 November 2021; Accepted: 21 December 2021;
Published: 10 February 2022.
Edited by:
Rui Liu, Southwest Petroleum University, ChinaReviewed by:
Huaguo Wen, Chengdu University of Technology, ChinaCopyright © 2022 She, Hu, Lv, Chen, Liang and Zheng. This is an open-access article distributed under the terms of the Creative Commons Attribution License (CC BY). The use, distribution or reproduction in other forums is permitted, provided the original author(s) and the copyright owner(s) are credited and that the original publication in this journal is cited, in accordance with accepted academic practice. No use, distribution or reproduction is permitted which does not comply with these terms.
*Correspondence: Min She, bXNuMDQyMEAxNjMuY29t
Disclaimer: All claims expressed in this article are solely those of the authors and do not necessarily represent those of their affiliated organizations, or those of the publisher, the editors and the reviewers. Any product that may be evaluated in this article or claim that may be made by its manufacturer is not guaranteed or endorsed by the publisher.
Research integrity at Frontiers
Learn more about the work of our research integrity team to safeguard the quality of each article we publish.