- Key Laboratory of Western China’s Environmental Systems (Ministry of Education), College of Earth and Environmental Sciences, Lanzhou University, Lanzhou, China
Although the interactions between biotic and geomorphic processes usually occur on small spatial and short temporal scales, many of the mechanisms remain to be investigated. This study provides the first direct evidence of the interaction between biotic burrowing and loess cave formation in the Chinese Loess Plateau (CLP). The study area is the Qingshui Valley in the western CLP, near Lanzhou. We surveyed the target site (with an area of ∼13,367 m2) four times from Jul 2019 to Dec 2020, using an unmanned aerial vehicle (UAV). High resolution UAV images enabled us to determine the temporal and spatial dynamics of biotic burrowing and loess caves. The results show that loess caves tended to develop down valley below collapses, while animal burrows were preferentially located upslope away from collapses. Despite the distinct “topographic niches” for both biotic and abiotic processes, we observed an interaction between the two processes in space when tracking their temporal dynamics. Three out of seven new loess caves were in the process of formation at typical “topographic niches” of animal burrows and there was a significantly high animal burrow density around these three caves before their initiation. These results indicate that the three caves were directly initiated from animal burrows and/or developed under the influence of biotic activities. Therefore, biotic burrowing promotes the spatial heterogeneity of loess cave distribution. We also found significant decreases in animal burrow density surrounding the newly-formed loess caves after their initiation. This may reflect a risk avoidance strategy of animal burrowing, which causes animals to avoid areas of recent mass movement (i.e., collapses and new caves). The formation and expansion of loess caves can dictate the distribution of active areas of biotic disturbance. Our results demonstrate a clear interaction between biotic burrowing and loess cave formation, and they emphasize the role of biological agents as a mechanism for the formation of loess caves, which enrich the understanding of searching fingerprints of life during landscape evolution.
Introduction
The Chinese Loess Plateau (CLP) experiences some of the most serious soil erosion on Earth (Shi and Shao, 2000; Zhao et al., 2013; Chen et al., 2018). The loess structure is characterized by well-developed macropores and vertical joints (Feng et al., 2021), making it prone to underground processes such as seepage and piping erosion (Verachtert et al., 2013; Li et al., 2020). Consequently, loess caves, as a unique underground landform, are widely distributed in the CLP (Peng et al., 2018; Li et al., 2020). The frequent rainstorm events in the region significantly accelerate the development of loess caves (Hu et al., 2020) and also increase soil erosion (Shi and Shao, 2000; Wu et al., 2018). Previous studies have often emphasized the contribution of loess caves to the total soil erosion rate of the CLP (Zhu, 1997, 2003; Zhu et al., 2002; Li et al., 2020). For example, loess caves were shown to deliver at least 43% of the annual catchment outflow discharge and 57% of the annual basin sediment yield during 15 storm events in the upper Yangdaogou catchment (Zhu, 1997; Zhu et al., 2002). By inference, the net erosion by loess caves may contribute at least 25–30% of basin sediment yields (Zhu, 2003).
Biotic burrowing is a universal underground process, which can disturb soil and influence landscape evolution (Darwin, 1881; Gilbert, 1909; Gabet, 2000; Ballová et al., 2019). Many areas in the CLP have a large population of subterranean rodents (Su et al., 2013; Sui et al., 2014; Zhang et al., 2021), because of the habitable grassland environment (Yu et al., 2017) and unlimited burrowing potential of loess (Krasnov et al., 1997). This burrowing activity has both direct and indirect impacts on geomorphic processes (Hall et al., 1999; Hall and Lamont, 2003; Escapa et al., 2007; Germain et al., 2021; Sanders et al., 2021). The burrowing process may directly cause soil displacement (Black and Montgomery, 1991) and alter the slope micromorphology (Zhao et al., 2021) as well as soil compactness (Rogasik et al., 2014). These indirect impacts were previously emphasized in terms of changing the soil water holding capacity (Zhang et al., 2003), increasing erosion by overland flow (Li T. et al., 2019), and increasing slope instability (Harvey et al., 2019). As a result, animal burrowing can induce an equivalent soil erosion of ∼1 mm yr−1 (Winchell et al., 2016) and transport sediment at rates ranging from 0.01 t ha−1 y−1 to 2.40 t ha−1 y−1 (Voiculescu et al., 2019). Given their role in landscape evolution, animals such as subterranean rodents are known as “ecosystem engineers” (Huntly and Inouye, 1988; Jones et al., 1994; Reichman and Seabloom, 2002; Zhang et al., 2003; Davidson et al., 2008; Su et al., 2020).
Although many previous studies have proposed a potential relationship between loess cave development and animal burrowing (Pierson, 1983; Botschek et al., 2002; Verachtert et al., 2010; Bernatek-Jakiel et al., 2016; Wang et al., 2019), this biotic mechanism has not been demonstrated by direct observation. Since animal burrows and loess caves are difficult to detect, the most likely possibility is that both processes produce maze-like tunnel systems with a complex underground space (Vleck, 1981; Zhu, 1997; Voigt, 2014; Got et al., 2020). The tunnel systems excavated by subterranean animals can create preferential paths for subsurface water flow (Botschek et al., 2002), which will in turn enlarge the tunnel space (Wilson et al., 2015), increase soil erosion (Chen et al., 2021), and eventually promote loess cave formation (Verachtert et al., 2013). Regardless of the validity of this biotic mechanism, the reliability of the potential relationship between the two underground processes remains to be investigated. Therefore, a systematic examination of whether biotic burrowing could promote loess cave development may improve our understanding of the formation mechanism of loess caves.
Another issue raised by the foregoing is whether there is an interaction between biotic and geomorphic processes (Hall and Lamont, 2003; Bendix and Cowell, 2010; Corenblit et al., 2011; Zaitlin and Hayashi, 2012; Cienciala et al., 2020). For example, salmon migration upstream has a significant impact on the longitudinal profile of the stream bed and thus on the evolution of entire watersheds; and as a feedback, additional habitats may be created that promote the evolution of species including salmon (Fremier et al., 2018). Although ecologists focus on population dynamics, while geomorphologists are more concerned with the energy and stress effects of geomorphic processes (Yoo et al., 2005; Winchell et al., 2016), both biology and geomorphology may interact on a large spatial and long temporal scale (Butler, 1995; Winchell et al., 2016). However, the interactions between biotic and geomorphic processes usually occur at small spatial and short temporal scales, many mechanisms of which are uninvestigated (Dietrich and Perron, 2006). Therefore, an interesting question is how geomorphic processes influence the spatial distribution of burrowing activity and the temporal dynamics of biotic behaviors. Recent biological studies in the CLP have provided several clues. It has been found that subterranean rodents (such as zokors) have adapted to excavating tunnels in thick, loose soil with high air permeability, in order to provide a living space (Zhou and Dou, 1990; Zhou et al., 2010; Song et al., 2017). These subterranean rodents always prefer flat, open areas for excavation (Li and Wang, 2015), which could subsequently be affected or altered by geomorphic processes. These initial findings encouraged us to explore the potential interaction between biotic burrowing and the formation of loess caves in the Chinese Loess Plateau.
The specific objectives of the present study were: (1) to map the spatial distribution and track the temporal dynamics of both animal burrows and loess caves; (2) to examine the associated topographic conditions and soil properties; and (3) to compare the temporal dynamics of the two processes and to explore their potential connection. To this end, we used an unmanned aerial vehicle (UAV) to survey the study site (the Qingshui Valley in the western CLP) four times from Jul 2019 to Dec 2020. We located the animal burrows and loess caves each time and collected 12 soil samples from the study areas. Based on these data, we present the first direct evidence of the interaction between biotic burrowing and loess cave formation in the CLP.
Study Area
The Chinese Loess Plateau is located in the middle and upper reaches of the Yellow River and covers an area of ∼430,000 km2 (Liu, 1985) (Figure 1). The region has a continental climate and annual precipitation increases from northwest to southeast with the range of 200–800 mm (Feng et al., 2016). The vegetation cover also increases in the same direction, showing a gradual transition from grassland to forest (Chen et al., 2008). The loess particles have been transported from the northwestern desert by wind and have accumulated in the CLP since the beginning of the Quaternary (Zhang et al., 2016). The loess particle size becomes finer from northwest to southeast, with increasing distance from the desert sources, and over 50% of loess particles are silt (Liu and Zhang, 1962). The spatial distribution of loess thickness shows two depocenters, near Lanzhou and Qingyang, and the depth in the west is generally thicker than that in the east (Figure 1A). Previous qualitative research suggested that the density of loess caves has a striped distribution pattern, with the density decreasing from southwest to northeast (Figure 1B).
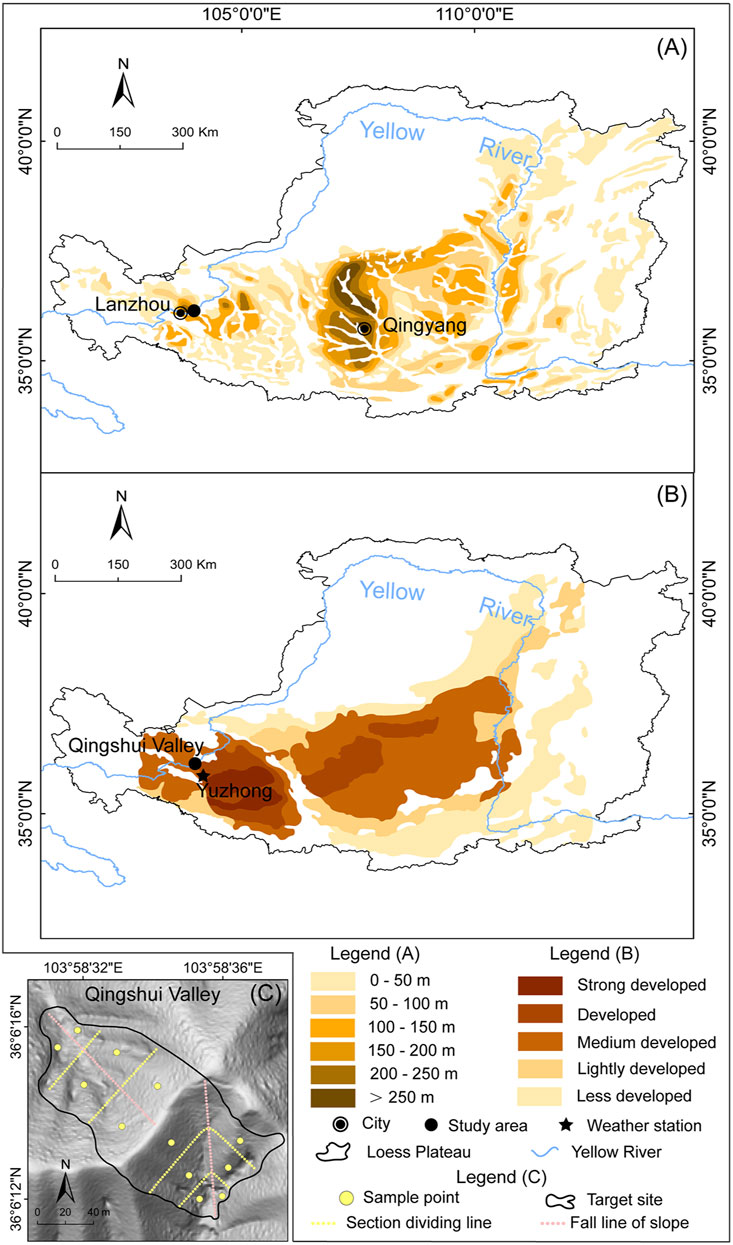
FIGURE 1. (A) The spatial distribution of loess thickness across the CLP (modified from Wang et al., 2010) (B) Development intensity map of loess caves in the CLP (modified from Peng et al., 2018) and (C) Shaded relief map of the target site in the Qingshui Valley showing the locations of the 12 sample points (yellow solid points). The two hillslopes were divided into three equal parts: slope top, slope middle, and slope toe (yellow dashed lines), according to their slope lengths in the direction of fall line (pink dashed lines).
The typical subterranean rodent species, the zokors (Chinese zokor Myospalax fontanierii and Gansu zokor Myospalax cansus) are widely distributed in the CLP (Lin et al., 2008; Sui et al., 2014). The population of Chinese zokors is ∼320–400 million across the entire CLP (Sui et al., 2014). The Chinese zokor density of Gansu province in the western CLP is ∼8 ha−1 (Chen et al., 2021). The Gansu zokor is a endemic rodent species in the CLP (Lin et al., 2021). The density of the Gansu zokor in Gansu province is ∼16–57 ha−1 (Cao and Wang, 1994).
The study area is located in Lanzhou City in Gansu Province in the western CLP. The mean annual precipitation is ∼310 mm and the mean annual temperature is ∼10°C; the natural vegetation is grassland (Feng and Wang, 2012; Zhang et al., 2016). The loess deposits in Lanzhou provide one of the most complete and continuous continental sediment archives, with maximum depths up to 400 m (Zhang et al., 2016; Guo et al., 2020). Loess caves in the study area are developed to an intermediate degree (Peng et al., 2018). The target site (Figure 1C) consists of two hillslopes with a total area of 13,367 m2, located in the Qingshui Valley (103.57°E–103.59°E, 36.05°N–36.07°N). The altitudinal range of the area is 1,549–2,010 m, and the average slope is 36.5°. Field investigations show a high degree of animal burrowing activity and negligible human influence.
Materials and Methods
Field Survey and UAV Mapping
We surveyed the animal burrows and loess caves in the target site a total of four times from Jul 2019 to Dec 2020 (Jul 2019, Nov 2019, Jul 2020 and Dec 2020). Animal burrows were identified in the field by their tunnel systems, including surface mounds and underground burrows (Miller, 1948). According to the characteristics of the mounds and burrows, we divided the burrows into active burrows and inactive burrows. The mounds of active burrows were clearly visible with fresh excavation traces (consisting of a mixture of loose material and grass, Figure 2A), or were partially visible with debris at the entrance (Figure 2B). If the mound debris was completely removed by geomorphic processes (Figure 2C), we checked for excavation traces on the tunnel sidewalls, which are usually smooth because the soil is compacted by the rodents. However, the inactive burrows had no evidence of current excavation, and grasses had regrown at the entrance (Figure 2D); also, the sidewall of the tunnel had partially collapsed and was no longer smooth. Here, we only focus on the active burrows. According to Peng et al. (2018), loess caves can be divided into vertical caves and parallel hidden caves, based on shape. The radius of the caves ranges from several centimeters to several meters. The depth of the caves varies from several tens of centimeters to tens of meters. Given that the diameter of the zokor burrow entrance is ∼8–12 cm (Chen et al., 2021), we only considered vertical loess caves with a diameter exceeding 12 cm and a depth of several tens of centimeters to tens of meters. In this study, we only considered caves with the above dimensions and which were usually exposed and clearly visible in the field (Figure 2E).
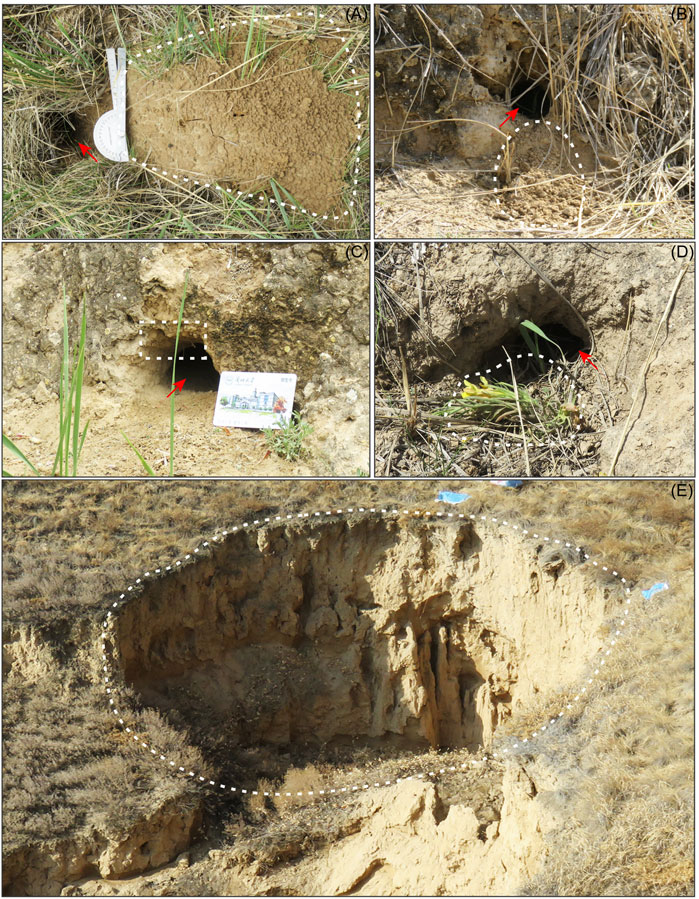
FIGURE 2. Photographs of typical active animal burrows (A, B and C), an inactive animal burrow (D), and a loess cave (E). The red arrows in panels (A, B, C and D) point to the entrance of the burrow. The white dashed line in panel (A) indicates the intact fresh mound of an active burrow. The white dashed line in panel (B) indicates a partially visible mound with debris at the entrance of an active burrow. The white dashed line in panel (C) indicates excavation traces on the tunnel sidewall of an active burrow. The white dashed line in panel (D) indicates the typical entrance of an inactive burrow with obvious grass regrowth and no evidence of current excavation. The white dashed line in panel (E) indicates a typical loess cave.
In the field, we used red flags (20 × 30 cm) to mark the animal burrows and blue flags (40 × 60 cm) to mark the loess caves. We then used an unmanned aerial vehicle (UAV, DJI Phantom 4 V2.0) to carry out photogrammetry. We reconstructed the 3D terrain in Pix4Dmapper and generated a DOM (digital orthophoto map) with a resolution of 8.1 cm/pixel and a DSM (digital surface model) with a resolution of 6.5 cm/pixel. We then mapped the spatial distribution of the animal burrows and loess caves based on the DOMs according to the colored flags.
The study area contains of two hillslopes with distinct aspects: a north-facing slope (N-slope) and a south-facing slope (S-slope). According to their slope lengths in the direction of fall line (116 m for the S-slope and 102 m for the N-slope; the pink dashed line in Figure 1C), we divided them into three equal sections (slope top, slope middle and slope toe; the yellow dashed lines in Figure 1C). We calculated the distribution density of the animal burrows and loess caves for the six sections for the four time periods, and the density changes in different sections were analyzed and listed in Table 1. Given the significant number and dynamics of animal burrows, we estimated the density distribution of animal burrows using the kernel density tool in ArcGIS 10.2, with a search radius of 20 m.
Topographic Analysis
In order to reduce micro-scale noise in the topographic data, we resampled the UAV-derived DSMs to a spatial resolution of 3 m, which is an optimal resolution to capture geomorphic processes (Heimsath et al., 1999). Topographic variables of animal burrows and loess caves were calculated using ArcGIS 10.2, including slope angle, plan curvature, profile curvature, and upslope contributing area. We combined the data for the four periods to cover the maximum animal burrow set and calculated the distribution of burrows for all topographic variables. We calculated the loess cave distribution for topographic variables for the final period (Dec 2020) since it comprises the largest dataset. The plan curvature describes the hillslope planform, which influences the convergence and divergence of flow (i.e., >0 is divergent; < 0 is convergent). The profile curvature affects the acceleration or deceleration of flow and indicates the downslope morphology (i.e., >0 is concave; < 0 is convex; ≈0 indicates a straight slope). The upslope contributing area here enumerates all of the upslope grids that could potentially produce runoff to the location. We extracted the topographic variables corresponding to the locations of marked animal burrows and loess caves. We then calculated the frequency of animal burrows and loess caves under different topographic variables to obtain their topographic preference.
Soil Properties and Precipitation Records
Soil properties have been proposed to impact the development of loess caves (Peng et al., 2018) and the activity of burrowing animals (Vleck, 1981). To study the influence of soil properties on the distribution of animal burrows and loess caves, we collected 12 samples from the 6 sections across the study area (the yellow solid points in Figure 1C). Measured soil properties included soil bulk density, saturated hydraulic conductivity and soil porosity, all of which may physically affect geomorphic processes. Bulk density and soil porosity are important soil physical properties that influence soil water retention capacity and infiltration rate, while the saturated hydraulic conductivity is a critical soil hydraulic property that affects water flow (i.e., infiltration and evaporation) and soil water redistribution. We used metal cylinders (100 cm3 volume) to collect soil samples in the field. In the laboratory, the cylinders were wetted with water to saturation before measuring the saturated hydraulic conductivity using the constant head permeability test (Hu et al., 2012). The soil columns were then oven-dried at 105°C for 24 h to calculate the bulk density from the sample volume and mass. Soil porosity was estimated from the bulk density and soil particle density. Soil particle density is generally assumed to be 2.65 g cm−3 (Blanco-Canqui et al., 2006). Soil bulk density and the saturated hydraulic conductivity were measured in the Key Laboratory of Western China’s Environmental Systems (Ministry of Education), Lanzhou University.
We also selected precipitation from nearby weather station, Yuzhong station (35.87°N, 104.15°E, in Figure 1B), of which the records are available to cover our investigation period (downloaded from NOAA’s National Centers for Environmental Information (NCEI), https://www.ncei.noaa.gov). We prepared the records in monthly precipitation and showed the results in Figure 4 below.
Results
Spatial Distribution and Temporal Dynamics of Loess Caves and Animal Burrows
The spatial distribution of loess caves and animal burrows over the four mapping periods are presented in Figure 3. The density and numbers of caves and burrows for the six sections are listed in Table 1 and illustrated in Figure 3. The total density of loess caves on the N-slope is higher than on the S-slope and the density at the slope toes is higher than in the other sections (Table 1). The number of loess caves was relatively stable through time (Figure 3) and they were located mainly beneath the collapses (blue dashed lines in Figure 3). During the survey period, seven new caves started to develop; four were located below the collapse near the slope toe, while the other three were located above the collapse near the slope top. These three caves were respectively developed: 1) at the slope top near the ridge in Nov 2019 (Figure 3C); 2) in the middle of the S-slope in Jul 2020 (Figure 3E); and 3) at the slope top on the N-slope in Dec 2020 (Figure 3G).
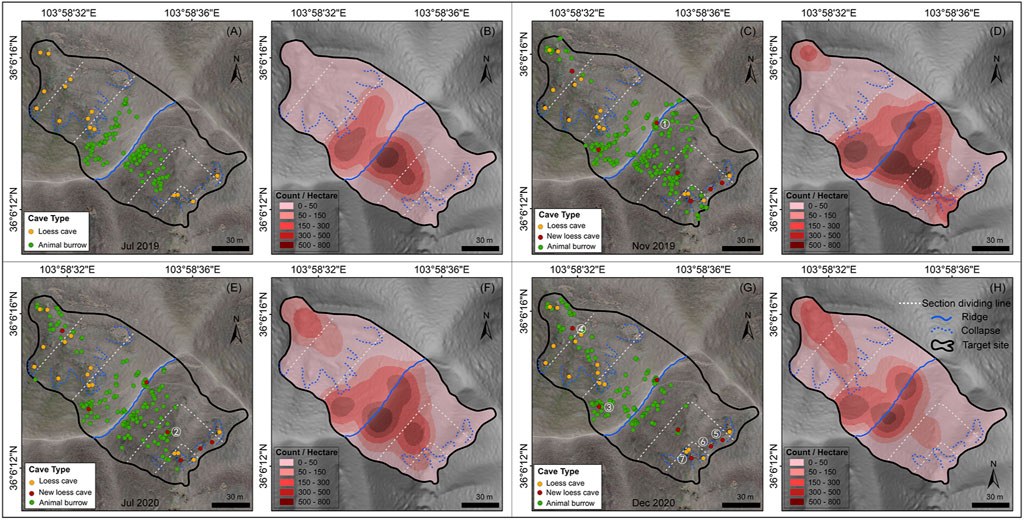
FIGURE 3. Spatial distribution and temporal dynamics of loess caves and animal burrows. Panels (A, C, E and G) show the distribution of loess caves (orange and red points) and animal burrows (green points) overlying the orthophotos of the target site for the four survey periods. The seven new loess caves are marked by red points and numbered in white (1–7). Panels (B, D, F and H) show the density (count·ha−1) of animal burrows. The blue dashed line indicates the collapse and the blue solid line is the ridge dividing the two slope aspects. The white lines are the boundary of the three equal sections (slope top, slope middle and slope toe) of each slope.
For the animal burrows, the total distribution density for the S-slope was always greater than that for the N-slope, except for Dec 2020 (period IV, in Table 1). In addition, with the exception of Nov 2019 (period II), the density at the slope top was always larger than that at the middle and toe, irrespective of slope aspect (Table 1). These results indicate that animal burrows have a significant topographic preference, like loess caves. However, unlike loess caves, the animal burrows show clear temporal dynamics (i.e., the counts of animal burrows at slope top increase from period I to period II then suddenly decrease from period II to period III) (Figure 4), which is further illustrated by the kernel density maps (Figures 3B,D,F,H). Furthermore, animal burrows at the slope top have a high density (101–263 ha−1) and 75–100% burrows are located above the collapses with a density of 122–170 ha−1 (Figure 3).
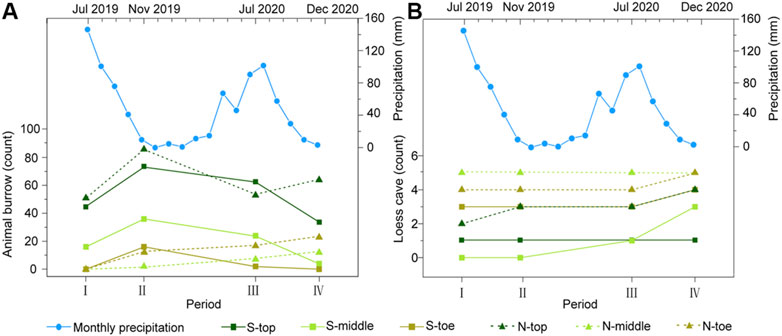
FIGURE 4. Counts of animal burrows (A) and loess caves (B) for the six slope sections over the four periods. The blue lines with dots are the monthly precipitation records during our investigation period.
In general, the loess caves and animal burrows show an opposite tendency in both time and space. The number of loess caves shows a uniform rate of increase, but the numbers of animal burrows fluctuate substantially over time (Figure 4). The loess caves tend to develop downslope below collapses, while animal burrows tend to be located upslope away from collapses. However, we did not find any unusual rainfall event during our investigation period (blue lines with dots in Figure 4). We then suggest that the opposite tendency of two processes in both time and space represents their dynamic status in nature. Therefore, it is noteworthy that the density of animal burrows surrounding new caves (30 m radius) decreases substantially (∼21–63%). This phenomenon suggests that the animal burrowing activities are influenced by geomorphic processes like loess cave formation.
Topographic Preferences of Loess Caves and Animal Burrows
The distribution of the loess caves and animal burrows reveals different topographic preferences, such as slope angle, upslope contributing area, plan curvature, and profile curvature (Figure 5). The results show that 86% of the animal burrows are located on slope angles from 10° to 40°, with a median of 36°; while 67% of the loess caves are located on slope angles from 40° to 60°, with a median of 44°. This means that animal burrows tend to be excavated on gentler slopes than loess caves (Figure 5A). The upslope contributing areas of loess caves are significantly larger than those of animal burrows (Figure 5B). Most of the animal burrows (92%) distributed in upslope contributing areas of <50 m2, but 71% of loess caves require an upslope contributing area >50 m2. The differences in topographic preference are also featured in the plan curvature and profile curvature (Figures 5C,D). The loess caves tend to develop in convergent landforms and concave topography, while the animal burrows have a strong dependency on convex topography, although there is no preference of plan curvature. Different from the four new caves below collapses near the slope toe, the three new caves located above the collapse near the slope top are all located in topographic contexts similar to animal burrows. These three loess caves have slope angles <40° and have upslope contributing areas <50 m2. In addition, they are all located in convex topographies and divergent landforms, which are unsuited to loess cave formation.
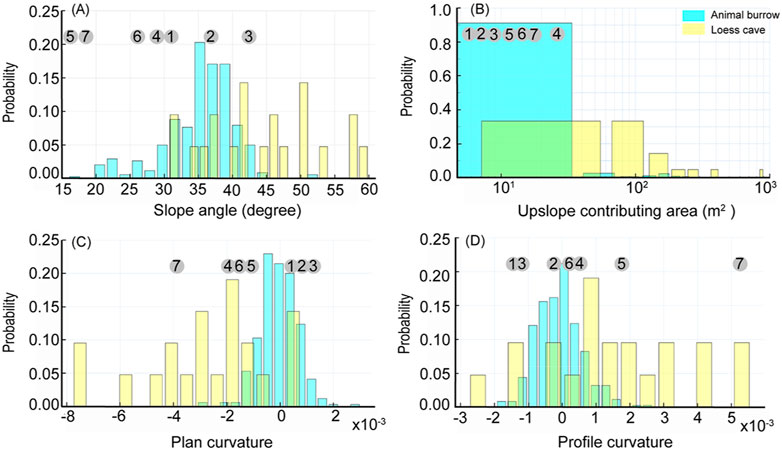
FIGURE 5. Probability histograms comparing the distribution of topographic conditions (A): Slope angle, (B): Upslope contributing area, (C): Plan curvature and (D): Profile curvature) for loess caves (yellow) and animal burrows (blue). The numbers in gray circles (1–7) correspond to the topographic conditions of the seven new loess caves.
Influence of Soil Properties on Loess Cave Density and Burrowing Activity
Having examined the topographic preferences of loess caves and animal burrows, we now consider the potential influence of soil properties on their spatial distribution. The six sections of the study area differ only slightly in bulk density and porosity but show significant differences in saturated hydraulic conductivity (Table 2). The results show that the average porosity and saturated hydraulic conductivity on the S-slope are both lower than on the N-slope. The porosity and saturated hydraulic conductivity at the slope tops are both generally lower than in the remaining parts. Although the bulk density on the S-slope is systematically higher than that on the N-slope, there is no significant difference within the two slope aspects. We therefore correlated the density of loess caves and animal burrows with porosity and saturated hydraulic conductivity. The results indicate that there is no correlation between loess cave density and porosity (Figure 6A), while there is a slight positive correlation between loess cave density and saturated hydraulic conductivity (Figure 6B). The density of animal burrows has no correlation with either porosity and saturated hydraulic conductivity (Figures 6C,D). These results suggest that the variation of soil properties at the slope scale has little influence on loess cave development and animal burrowing activity. Therefore, we conclude that soil properties are not responsible for the temporal dynamics of loess caves and animal burrows.
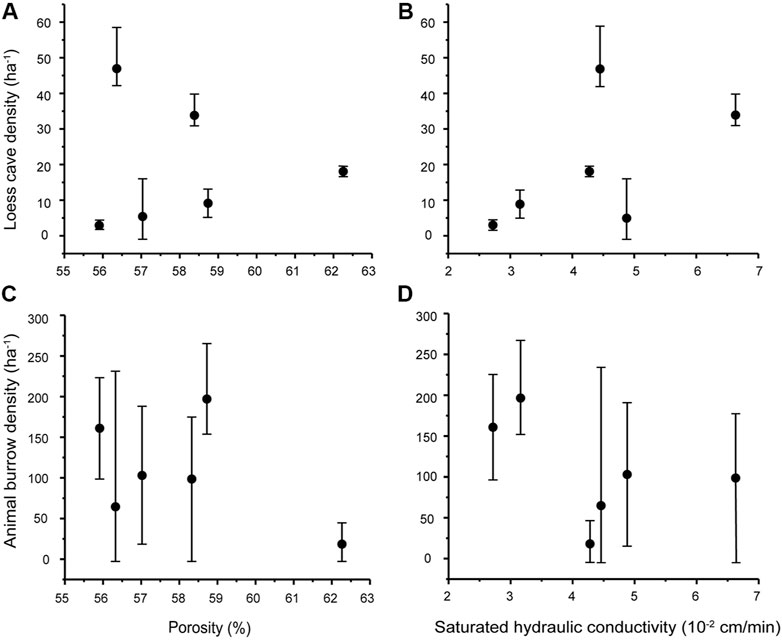
FIGURE 6. Scatter plots of loess cave density and animal burrow density versus porosity (A and C) and saturated hydraulic conductivity (B and D). The black dots are the average values of loess cave density/animal burrow density for the six slope sections, and the error bars represent the corresponding minimum and maximum.
Discussion
Topographic Controls on Loess Cave Development and Burrowing Activity
The distribution of loess caves in the Qingshui Valley shows topographic preferences in terms of slope angle and upslope contributing area. More importantly, loess caves tend to develop in convergent landforms and areas of concave topography (Figure 5), from which we infer that the loess cave formation processes depend on surface runoff. This mechanism may explain the higher loess cave density at the slope toe compared to the other slope sections (Table 1). Runoff-induced loess cave development might be expected given the sparse vegetation and storm-dominated rainfall in the area, where land cover has a limited influence on overland flow processes (Hessel and Van Asch, 2003; Geng et al., 2015). Therefore, the preferred topographic areas of loess caves are conducive to forming concentrated flow (Garland and Humphrey, 1992; Faulkner, 2006). The flow could easily erode the well-developed vertical joints (Xu, 1999) and then cause the expansion and disaggregation of the surrounding loess particles (Li X.-A. et al., 2019b), by inference, forming loess caves (Hu et al., 2020). This is probably why previous studies found that loess cave formation tended to occur at gully heads or on hillslopes with furrows and depressions (Zhu, 2006). Thus, the observed topographic preferences of loess caves in the study area are consistent with previous research.
Animal burrows also have a topographic preference, tending to occur on gentle slopes (Figure 5A), with a small upslope contributing area (Figure 5B) and convex topography (Figure 5D). We consider it likely that the preferred burrowing locations of rodents are closely related to their living habits. The most important aspect of selecting a habitable environment is a spatial unit that provides the necessary conditions for survival (Morris et al., 2008). Besides avoiding predators and seeking an adequate food supply (Forsman and Martin, 2009), topographic conditions are crucial in determining a habitable environment on the slope scale (Bailey, 2005). The preferred burrowing topography is a location that minimizes the formation of concentrated flow. A gentle slope angle could facilitate infiltration and decrease the amount of overland flow (Fox et al., 1997). In addition, a convex topography is concentrated at the slope top where the upslope contributing area is small and there is a limited opportunity to form concentrated flow. Biotic behavior is another reason guiding the selection of a gentle slope. For example, Seabloom et al. (2000) found that a steep slope angle will lead to the falling back of excavated material, which may be the reason why the excavation angle to the horizontal plane was smaller than the angle of repose of the loose mound of excavated soil; therefore, a gentle slope will reduce the cost of excavation (Vleck, 1981). Accordingly, the topographic preference of animal burrows reflects the survival needs and minimum excavation cost for the typical subterranean rodent species in the study area.
We found that both loess cave development and animal burrowing activity demonstrate significant topographic controls, although they have different topographic preferences. However, the variation of soil properties at the slope scale has little influence on preferred locations. Loess caves are dominantly developed in the valley below the collapse, while animal burrows are dominantly located upslope away from collapses. The varied topographic preferences lead to distinct “topographic niches” for both biotic and abiotic processes, with little probability for overlapping in space.
Interaction Between Loess Cave Development and Animal Burrowing Activity
We observed the initiation of seven new loess caves during the survey period. Four caves are still located below the collapse near the slope toe, which is consistent with the concept of “topographic niches” and with the observations of a previous study (Zhu, 2012). However, the remaining three caves (No. 1–3 in Figure 3) are all located above the collapse near the slope top, which is an unexpected location for loess cave formation based on our statistics. It is surprising that these three new caves are forming in the typical “topographic niches” of animal burrows, and we suggest the possibility that these caves were directly initiated (or “inherited”) from animal burrows and/or developed under the influence of biotic activities.
Our observations suggest that loess caves can inherit animal burrows, and one example is new cave No. 2, in a mid-slope location and with an average diameter of ∼28 cm and depth of ∼20 cm, that was initiated in Jul 2020 (Figure 7A). In this case there were visible animal tunnel remnants near the bottom of the cave (red arrow in Figure 7A). Interestingly, the average diameter of the entrance of new cave No. 2 (Figure 7B) was enlarged to 35 cm and the depth deepened to 40 cm by the time of our final survey (Oct 2021), which provides direct evidence for the role of animal burrowing in initiating loess cave formation.
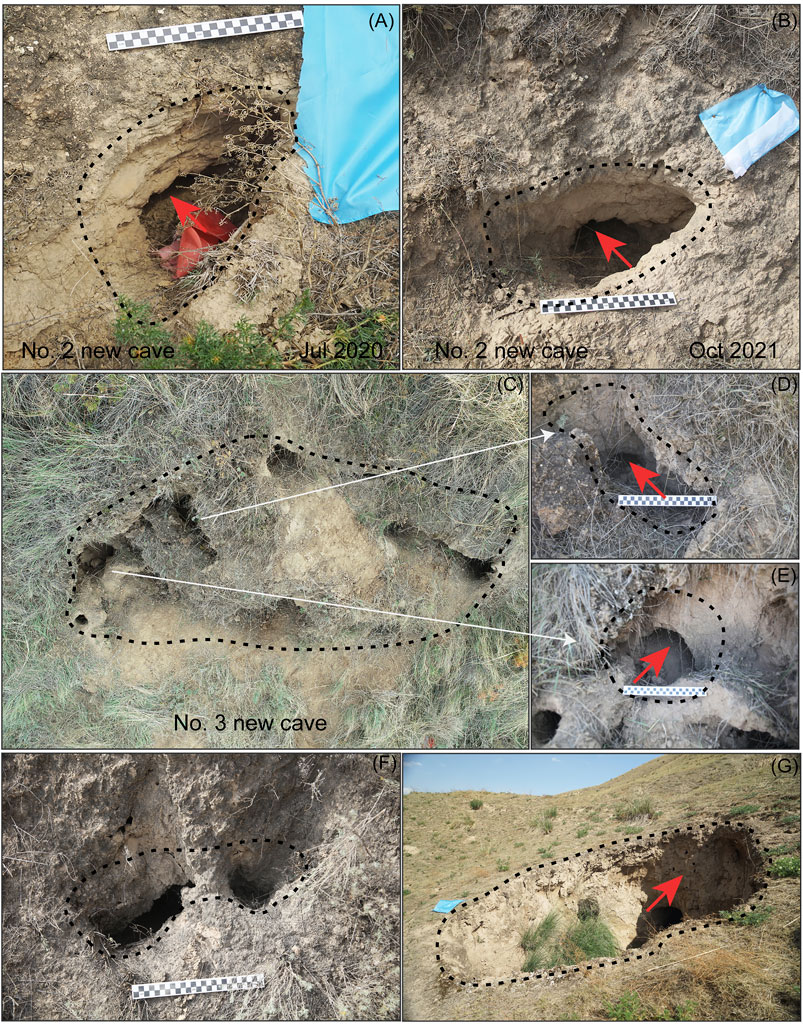
FIGURE 7. Evidence that loess caves can “inherit” animal burrows (A, B, D, E and F) and develop under the influence of biotic activities (C and G). Both panel (A and B) are the No.2 new cave, which were photographed in Jul 2020 and Oct 2021 respectively. Panel (C) is the No.3 new cave, which consists of eight sub-caves [such as sub-cave in panel (D) and sub-cave in panel (E)]. The new cave in panel (F) and the mature cave in panel (G) are observed near our study area. The black dashed lines indicate the entrances of loess caves. The red arrows in panels (A, B, D, E, F, and G) indicate the remaining animal burrows in loess caves.
Other new caves may also have developed under the influence of biotic activity. New cave No. 3 (Figure 7C) at the slope top on the N-slope consists of eight sub-caves; one sub-cave had an average diameter of 30 cm and depth of 20 cm (Figure 7D) and another sub-cave had an average diameter of 35 cm and depth of 30 cm (Figure 7E). There are also remnant animal burrows near the base of these sub-caves (indicated by the red arrows in 7D and 7E). There were many signs of collapse in new cave No. 3, which were in the process of expansion. Field observation indicates that animal burrowing can enhance water infiltration, either by altering the microtopography and then extending the runoff path, or by supplying loose material to the surface which promotes water penetration (Chen et al., 2021). Given the abundant joints and macropores in the loess (Zhang et al., 2018), the enhanced infiltration will cause the rapid expansion and collapse of the loess during rainfall (Zhuang and Peng, 2014), thus accelerating the development of new loess caves.
Our results also show a high level of animal burrow density around the three new caves before their initiation. For example, the density for cave No. 1 is 184 ha−1 (30 m radius); that for cave No. 2 is 279 ha−1; and that for cave No. 3 is 149 ha−1. Thus, there is both direct and indirect evidence supporting our speculation that biotic activities can induce and accelerate the development of loess caves. This phenomenon is actually very common in the CLP. For example, of two loess caves observed near our study area, one is a new cave (Figure 7F) with an average diameter of 28 cm and depth of 50 cm, consisting of two animal burrow entrances; while the inner part of the cave has collapsed and expanded to form an integrated cave, suggesting inheritance from animal burrows. Moreover, there were numerous signs of animal burrowing on the wall of a mature cave (Figure 7G), which further verifies the role of animal burrowing in accelerating the development of loess caves. Overall, our findings underline the potential role of biological activity in initiating and developing underground geomorphic phenomena (e.g., piping). In loess areas in northern Mississippi, Wilson et al. (2015) suggested that old roots or other biological channels are highly susceptible to the formation of soil pipes due to strong internal erosion. In addition, the burrows of moles and mice enable immediate water infiltration and direct vertical and lateral water movement; and earthworms were found to provide a high transport capacity for soil water via creating abundant macropores in a loess-rich soil in Germany (Botschek et al., 2002). Pipe formation resulting from biological activity can modify soil properties and soil texture, making the soil prone to erosion by runoff or groundwater (Verachtert et al., 2013). The existence of animal burrows as a condition for pipe development has also been reported in areas without loess (Czeppe, 1960; Bernatek-Jakiel et al., 2016).
Animal burrowing is a dynamic phenomenon on the annual scale and is influenced by geomorphic processes such as loess cave formation. For example, we observed an abrupt decrease in animal burrow density on the S-slope in Dec 2020, after the initiation of cave No. 2. We speculate that the formation and expansion of loess caves may dictate the location of active areas of biotic disturbance. This is indicated by the significant decrease in animal burrow density surrounding the newly formed loess caves after their initiation, which reflects a risk avoidance strategy for animals in the long-term natural selection process, which requires that organisms inherit behaviors in order to avoid risks and enhance survival rates (Lima et al., 1985; Blanchard et al., 2001; Nemati et al., 2013). Migration to a safe location is a type of inherited activity, developed via a long process of random mutation and natural selection (Kirschvink, 2000). Rodents such as burrowing animals may have a greater capacity for risk prediction than animals above ground, because their hearing is extremely acute (Heffner and Masterton, 1980). We therefore infer that the excavation activities of animals are indeed restricted by recent mass movement processes (i.e., cave formation and collapses), thus promoting their migration.
The proposed interaction between biotic burrowing and loess cave formation in the Chinese Loess Plateau has significant implications for landscape evolution. First, the biotically-induced loess cave formation mechanism is an important supplement to runoff-induced cave formation. This biotic mechanism could promote the spatial heterogeneity of the distribution of loess caves. In this study, we found that three out of seven new loess caves were developing via a biotic mechanism. We also found that 14% of loess caves were located in areas with a convex topography and divergent landforms (Figure 5), which are typical “topographic niches” for animal burrows. We propose tentatively that these caves were all induced by animal burrowing. Second, the formation of loess caves promotes the migration of animals, which in turn will extend the area of burrowing activity and increase the rate of soil loss via frequent underground excavation. Although biotic disturbance has been proposed as an important agent of surface erosion (Reichman and Seabloom, 2002; Gabet et al., 2003; Stallins, 2006; Winchell et al., 2016). It also could introduce bias to the sediment flux calculated by geomorphic transport laws and promote the spatial heterogeneity of hillslope processes (Roering et al., 1999; Dietrich et al., 2003). However, this process has not been comprehensively investigated in the CLP. Further quantitative studies are needed to determine the geomorphic contribution of different animals to soil erosion across the Chinese Loess Plateau.
Conclusion
We have investigated the spatial distribution of animal burrows and loess caves based on field investigations including UAV mapping of a site in the Qingshui Valley. We surveyed the site four times, from Jul 2019 to Dec 2020, in order to track the temporal dynamics of both processes. We found that both loess cave development and animal burrowing activity show a significant topographic control, although they have different topographic preferences in term of slope angle, plan curvature, profile curvature, and upslope contributing area. The preferred topographic areas of loess caves are conducive to forming concentrated flow, supporting a runoff-induced mechanism of loess cave development. Animals prefer topographic contexts that are unlikely to form concentrated flows. These topographic preferences lead to distinct “topographic niches” for both biotic and abiotic processes with little chance for overlapping in space.
Seven new loess caves started to develop during the survey period. Four of the caves were consistent with their “topographic niches”, but the other three new caves were developing in the typical “topographic niches” of animal burrows. We also observed a significantly high animal burrow density around three new caves before their initiation. Thus, we conclude that these three caves were directly initiated from animal burrows and/or develop under the influence of biotic activities. Animal burrowing activity is seemingly arbitrary but with a clear risk avoidance strategy, which leads them to avoid areas of recent mass movement (e.g., caves and collapses), concentrated flow paths, and newly formed loess caves. The formation and expansion of loess caves can dictate the active areas of biotic disturbance, while biotic burrowing in turn promotes the spatial heterogeneity of the loess cave distribution. Our study emphasizes the role of biological agents in formation of loess caves and provides the first direct evidence of the interaction between biotic burrowing and loess cave formation in the CLP.
Data Availability Statement
The original contributions presented in the study are included in the article/supplementary material, further inquiries can be directed to the corresponding author.
Author Contributions
This manuscript was written by HG and RL. This manuscript was designed by HG and BP, HG, RL, and WZ analyzed the topographic data. RL and YZ measured the soil properties. RL, WZ, YZ, RX, YG, attended the field work.
Funding
This work was co-supported by the National Natural Science Foundation of China (42041006, 41971001 and 41501002), and the Fundamental Research Funds for the Central Universities (lzujbky-2020-70).
Conflict of Interest
The authors declare that the research was conducted in the absence of any commercial or financial relationships that could be construed as a potential conflict of interest.
Publisher’s Note
All claims expressed in this article are solely those of the authors and do not necessarily represent those of their affiliated organizations, or those of the publisher, the editors and the reviewers. Any product that may be evaluated in this article, or claim that may be made by its manufacturer, is not guaranteed or endorsed by the publisher.
Acknowledgments
We thank Pro. Lixun Zhang for his guidance on the identification of the animal burrows. Thanks to Wenqian Yang and Ke Yan for their assistance with field work, and Dr. Jan Bloemendal for revising the language.
References
Bailey, D. W. (2005). Identification and Creation of Optimum Habitat Conditions for Livestock. Rangel. Ecol. Manag. 58, 109–118. doi:10.2111/03-147.1
Ballová, Z., Pekárik, L., Píš, V., and Šibík, J. (2019). How Much Do Ecosystem Engineers Contribute to Landscape Evolution? A Case Study on Tatra Marmots. Catena 182, 104121. doi:10.1016/j.catena.2019.104121
Bendix, J., and Cowell, C. M. (2010). Fire, Floods and Woody Debris: Interactions between Biotic and Geomorphic Processes. Geomorphology 116, 297–304. doi:10.1016/j.geomorph.2009.09.043
Bernatek-Jakiel, A., Kacprzak, A., and Stolarczyk, M. (2016). Impact of Soil Characteristics on Piping Activity in a Mountainous Area under a Temperate Climate (Bieszczady Mts., Eastern Carpathians). Catena 141, 117–129. doi:10.1016/j.catena.2016.03.001
Black, T. A., and Montgomery, D. R. (1991). Sediment Transport by Burrowing Mammals, Marin County, California. Earth Surf. Process. Landf. 16, 163–172. doi:10.1002/esp.3290160207
Blanchard, R. J., Yudko, E., Dulloog, L., and Blanchard, D. C. (2001). Defense Changes in Stress Nonresponsive Subordinate Males in a Visible Burrow System. Physiol. Behav. 72, 635–642. doi:10.1016/S0031-9384(00)00449-2
Blanco-Canqui, H., Lal, R., Post, W. M., Izaurralde, R. C., and Shipitalo, M. J. (2006). Organic Carbon Influences on Soil Particle Density and Rheological Properties. Soil Sci. Soc. Am. J. 70, 1407–1414. doi:10.2136/sssaj2005.0355
Botschek, J., Krause, S., Abel, T., and Skowronek, A. (2002). Hydrological Parameterization of Piping in Loess-Rich Soils in the Bergisches Land, Nordrhein-Westfalen, Germany. J. Plant Nutr. Soil Sci. 165, 506–510. doi:10.1002/1522-2624(200208)165:4<506:aid-jpln506>3.0.co;2-7
Butler, D. R. (1995). Zoogeomorphology: Animals as Geomorphic Agents. Cambridge, UK: Cambridge University Press, 231.
Cao, Z., and Wang, M. (1994). Study on the Biological Characteristics of Gansu Zokor (In Chinese). Gansu Agricl Sci.Tech. 5, 32–33.
Chen, H., Shao, M., and Li, Y. (2008). Soil Desiccation in the Loess Plateau of China. Geoderma 143, 91–100. doi:10.1016/j.geoderma.2007.10.013
Chen, H., Zhang, X., Abla, M., Lü, D., Yan, R., Ren, Q., et al. (2018). Effects of Vegetation and Rainfall Types on Surface Runoff and Soil Erosion on Steep Slopes on the Loess Plateau, China. Catena 170, 141–149. doi:10.1016/j.catena.2018.06.006
Chen, M., Ma, L., Shao, M., Wei, X., Jia, Y., Sun, S., et al. (2021). Chinese Zokor (Myospalax Fontanierii) Excavating Activities Lessen Runoff but Facilitate Soil Erosion - A Simulation experiment. Catena 202, 105248. doi:10.1016/j.catena.2021.105248
Cienciala, P., Nelson, A. D., Haas, A. D., and Xu, Z. (2020). Lateral Geomorphic Connectivity in a Fluvial Landscape System: Unraveling the Role of Confinement, Biogeomorphic Interactions, and Glacial Legacies. Geomorphology 354, 107036. doi:10.1016/j.geomorph.2020.107036
Corenblit, D., Baas, A. C. W., Bornette, G., Darrozes, J., Delmotte, S., Francis, R. A., et al. (2011). Feedbacks between Geomorphology and Biota Controlling Earth Surface Processes and Landforms: A Review of Foundation Concepts and Current Understandings. Earth-Sci. Rev. 106, 307–331. doi:10.1016/j.earscirev.2011.03.002
Czeppe, Z. (1960). Suffosional Phenomena in Slope Loams of the Upper Sau Drainage basin. Inst. Geol. Biuletin (Warsaw) 9, 232–297.
Darwin, C. (1881). The Formation of Vegetable Mould: Through the Action of Worms, with Observations on Their Habits: London John Murray Press.
Davidson, A. D., Lightfoot, D. C., and McIntyre, J. L. (2008). Engineering Rodents Create Key Habitat for Lizards. J. Arid Environ. 72, 2142–2149. doi:10.1016/j.jaridenv.2008.07.006
Dietrich, W. E., and Perron, J. T. (2006). The Search for a Topographic Signature of Life. Nature 439, 411–418. doi:10.1038/nature04452
Dietrich, W. E., Bellugi, D. G., Sklar, L. S., Stock, J. D., Heimsath, A. M., and Roering, J. J. (2003). Geomorphic Transport Laws for Predicting Landscape Form and Dynamics. Geophys. Monogr. Geophys. Union. 135, 103–132. doi:10.1029/135GM09
Escapa, M., Minkoff, D. R., Perillo, G. M. E., and Iribarne, O. (2007). Direct and Indirect Effects of Burrowing Crab Chasmagnathus Granulatus Activities on Erosion of Southwest Atlantic Sarcocornia -dominated Marshes. Limnol. Oceanogr. 52, 2340–2349. doi:10.4319/lo.2007.52.6.2340
Faulkner, H. (2006). “Piping hazard on collapsible and dispersive soils in Europe,” in Soil Erosion in Europe. Editors J. Boardman, and J. Poesen (Hoboken: John Wiley and Sons, Ltd Press), 537–562.
Feng, X., and Wang, S. (2012). Influence of Different Weather Events on Concentrations of Particulate Matter with Different Sizes in Lanzhou, China. J. Environ. Sci. 24, 665–674. doi:10.1016/S1001-0742(11)60807-3
Feng, X., Fu, B., Piao, S., Wang, S., Ciais, P., Zeng, Z., et al. (2016). Revegetation in China's Loess Plateau Is Approaching Sustainable Water Resource Limits. Nat. Clim Change. 6, 1019–1022. doi:10.1038/nclimate3092
Feng, L., Zhang, M., Jin, Z., Zhang, S., Sun, P., Gu, T., et al. (2021). The Genesis, Development, and Evolution of Original Vertical Joints in Loess. Earth-Sci. Rev. 214, 103526. doi:10.1016/j.earscirev.2021.103526
Forsman, J. T., and Martin, T. E. (2009). Habitat Selection for Parasite-free Space by Hosts of Parasitic Cowbirds. Oikos 118, 464–470. doi:10.1111/j.1600-0706.2008.17000.x
Fox, D. M., Bryan, R. B., and Price, A. G. (1997). The Influence of Slope Angle on Final Infiltration Rate for Interrill Conditions. Geoderma 80, 181–194. doi:10.1016/S0016-7061(97)00075-X
Fremier, A. K., Yanites, B. J., and Yager, E. M. (2018). Sex that Moves Mountains: The Influence of Spawning Fish on River Profiles over Geologic Timescales. Geomorphology 305, 163–172. doi:10.1016/j.geomorph.2017.09.033
Gabet, E. J., Reichman, O. J., and Seabloom, E. W. (2003). The Effects of Bioturbation on Soil Processes and Sediment Transport. Annu. Rev. Earth Planet. Sci. 31, 249–273. doi:10.1146/annurev.earth.31.100901.141314
Gabet, E. J. (2000). Gopher Bioturbation: Field Evidence for Non-linear Hillslope Diffusion. Earth Surf. Process. Landforms 25, 1419–1428. doi:10.1002/1096-9837(200012)25:13<1419:aid-esp148>3.0.co;2-1
Garland, G., and Humphrey, B. (1992). Field Measurements of Discharge and Sediment Yield from a Soil Pipe in the Natal Drakensberg, South Africa. zfg 36, 15–23. doi:10.1127/zfg/36/1992/15
Geng, H., Pan, B., Milledge, D. G., Huang, B., and Zhang, G. (2015). Quantifying Sheet Wash Erosion Rates in a Mountainous Semi-arid basin Using Environmental Radionuclides and a Stream Power Model. Earth Surf. Process. Landforms 40, 1814–1826. doi:10.1002/esp.3761
Germain, D., Ianăş, A.-N., and Voiculescu, M. (2021). Sediment Transport by the Burrowing Activity of Snow Vole (Chionomys Nivalis) in Subalpine Prairies of the Parâng Mountains (Romania): Quantitative Modelling and Methodological Perspectives. Geomorphology 374, 107519. doi:10.1016/j.geomorph.2020.107519
Got, J. B., Bielders, C. L., and Lambot, S. (2020). Characterizing Soil Piping Networks in Loess‐derived Soils Using Ground‐penetrating Radar. Vadose Zone J. 19, e20006. doi:10.1002/vzj2.20006
Guo, B., Peng, T., Yu, H., Hui, Z., Ma, Z., Li, X., et al. (2020). Magnetostratigraphy and Palaeoclimatic Significance of the Late Pliocene Red Clay‐Quaternary Loess Sequence in the Lanzhou Basin, Western Chinese Loess Plateau. Geophys. Res. Lett. 47, 1–10. doi:10.1029/2019GL086556
Hall, K., and Lamont, N. (2003). Zoogeomorphology in the Alpine: Some Observations on Abiotic-Biotic Interactions. Geomorphology 55, 219–234. doi:10.1016/S0169-555X(03)00141-7
Hall, K., Boelhouwers, J., and Driscoll, K. (1999). Animals as Erosion Agents in the alpine Zone: Some Data and Observations from Canada, Lesotho, and Tibet. Arctic, Antarctic, Alp. Res. 31, 436–446. doi:10.1080/15230430.1999.12003328
Harvey, G. L., Henshaw, A. J., Brasington, J., and England, J. (2019). Burrowing Invasive Species: An Unquantified Erosion Risk at the Aquatic‐Terrestrial Interface. Rev. Geophys. 57, 1018–1036. doi:10.1029/2018RG000635
Heffner, H., and Masterton, B. (1980). Hearing in Glires: Domestic Rabbit, Cotton Rat, Feral House Mouse, and Kangaroo Rat. The J. Acoust. Soc. Am. 68, 1584–1599. doi:10.1121/1.385213
Heimsath, A. M., E. Dietrich, W. W., Nishiizumi, K., and Finkel, R. C. (1999). Cosmogenic Nuclides, Topography, and the Spatial Variation of Soil Depth. Geomorphology 27, 151–172. doi:10.1016/S0169-555X(98)00095-6
Hessel, R., and Van Asch, T. (2003). Modelling Gully Erosion for a Small Catchment on the Chinese Loess Plateau. Catena 54, 131–146. doi:10.1016/S0341-8162(03)00061-4
Hu, W., Shao, M. A., and Si, B. C. (2012). Seasonal Changes in Surface Bulk Density and Saturated Hydraulic Conductivity of Natural Landscapes. Eur. J. Soil Sci. 63, 820–830. doi:10.1111/j.1365-2389.2012.01479.x
Hu, S., Qiu, H., Wang, N., Cui, Y., Wang, J., Wang, X., et al. (2020). The Influence of Loess Cave Development upon Landslides and Geomorphologic Evolution: A Case Study from the Northwest Loess Plateau, China. Geomorphology 359, 107167. doi:10.1016/j.geomorph.2020.107167
Huntly, N., and Inouye, R. (1988). Pocket Gophers in Ecosystems: Patterns and Mechanisms. BioScience 38, 786–793. doi:10.2307/1310788
Jones, C. G., Lawton, J. H., and Shachak, M. (1994). “Organisms as Ecosystem Engineers,” in Ecosystem Management (New York, NY: Springer), 130–147. doi:10.1007/978-1-4612-4018-1_14
Kirschvink, J. L. (2000). Earthquake Prediction by Animals: Evolution and Sensory Perception. Bull. Seismol. Soc. Am. 90, 312–323. doi:10.1785/0119980114
Krasnov, B. R., Shenbrot, G. I., Medvedev, S. G., Vatschenok, V. S., and Khokhlova, I. S. (1997). Host-habitat Relations as an Important Determinant of Spatial Distribution of Flea Assemblages (Siphonaptera) on Rodents in the Negev Desert. Parasitology 114, 159–173. doi:10.1017/S0031182096008347
Li, Q., and Wang, X. (2015). Into Tibet: An Early Pliocene Dispersal of Fossil Zokor (Rodentia: Spalacidae) from Mongolian Plateau to the Hinterland of Tibetan Plateau. Plos One 10, e0144993–18. doi:10.1371/journal.pone.0144993
Li, T., Jia, Y., Shao, M. a., and Shen, N. (2019a). Camponotus Japonicus Burrowing Activities Exacerbate Soil Erosion on Bare Slopes. Geoderma 348, 158–167. doi:10.1016/j.geoderma.2019.04.035
Li, X. A., Wang, L., Yan, Y., Hong, B., and Li, L. (2019b). Experimental Study on the Disintegration of Loess in the Loess Plateau of China. Bull. Eng. Geol. Environ. 78, 4907–4918. doi:10.1007/s10064-018-01434-6
Li, X. A., Wang, L., Hong, B., Li, L. C., Liu, J., and Lei, H. n. (2020). Erosion Characteristics of Loess Tunnels on the Loess Plateau: A Field Investigation and Experimental Study. Earth Surf. Process. Landforms. 45, 1945–1958. doi:10.1002/esp.4857
Lima, S. L., Valone, T. J., and Caraco, T. (1985). Foraging-efficiency-predation-risk Trade-Off in the Grey Squirrel. Anim. Behav. 33, 155–165. doi:10.1016/S0003-3472(85)80129-9
Lin, G. H., Cai, Z. Y., Zhang, T. Z., Su, J. P., and Thirgood, S. J. (2008). Genetic Diversity of the Subterranean Gansu Zokor in a Semi-natural Landscape. J. Zoolog. 275, 153–159. doi:10.1111/j.1469-7998.2008.00423.x
Lin, J., Fan, L., Han, Y., Guo, J., Hao, Z., Cao, L., et al. (2021). The mTORC1/eIF4E/HIF-1α Pathway Mediates Glycolysis to Support Brain Hypoxia Resistance in the Gansu Zokor, Eospalax Cansus. Front. Physiol. 12, 626240. doi:10.3389/fphys.2021.626240
Miller, M. A. (1948). Seasonal Trends in Burrowing of Pocket Gophers (Thomomys). J. Mammal. 29, 38–44. doi:10.2307/1375279
Morris, D. W., Clark, R. G., and Boyce, M. S. (2008). Habitat and Habitat Selection: Theory, Tests, and Implications. Isr. J. Ecol. Evol. 54, 287–294. doi:10.1560/IJEE.54.310.1560/ijee.54.3-4.287
Nemati, F., Kolb, B., and Metz, G. A. (2013). Stress and Risk Avoidance by Exploring Rats: Implications for Stress Management in Fear-Related Behaviours. Behav. Process. 94, 89–98. doi:10.1016/j.beproc.2012.12.005
Peng, J., Sun, P., Igwe, O., and Li, X. A. (2018). Loess Caves, a Special Kind of Geo-hazard on Loess Plateau, Northwestern China. Eng. Geol. 236, 79–88. doi:10.1016/j.enggeo.2017.08.012
Pierson, T. C. (1983). Soil Pipes and Slope Stability. Q. J. Eng. Geol. Hydrogeol. 16, 1–11. doi:10.1144/gsl.qjeg.1983.016.01.01
Reichman, O. J., and Seabloom, E. W. (2002). The Role of Pocket Gophers as Subterranean Ecosystem Engineers. Trends Ecol. Evol. 17, 44–49. doi:10.1016/S0169-5347(01)02329-1
Roering, J. J., Kirchner, J. W., and Dietrich, W. E. (1999). Evidence for Nonlinear, Diffusive Sediment Transport on Hillslopes and Implications for Landscape Morphology. Water Resour. Res. 35, 853–870. doi:10.1029/1998WR900090
Rogasik, H., Schrader, S., Onasch, I., Kiesel, J., and Gerke, H. H. (2014). Micro-scale Dry Bulk Density Variation Around Earthworm (Lumbricus Terrestris L.) Burrows Based on X-ray Computed Tomography. Geoderma 213, 471–477. doi:10.1016/j.geoderma.2013.08.034
Sanders, H., Rice, S. P., and Wood, P. J. (2021). Signal Crayfish Burrowing, Bank Retreat and Sediment Supply to Rivers: A Biophysical Sediment Budget. Earth Surf. Process. Landforms. 46, 837–852. doi:10.1002/esp.5070
Seabloom, E. W., Reichman, O. J., and Gabet, E. J. (2000). The Effect of Hillslope Angle on Pocket Gopher (Thomomys bottae) Burrow Geometry. Oecologia 125, 26–34. doi:10.1007/PL00008888
Shi, H., and Shao, M. (2000). Soil and Water Loss from the Loess Plateau in China. J. Arid Environ. 45, 9–20. doi:10.1006/jare.1999.0618
Song, Y. C., Liu, Y., Lin, Y. Y., Liang, T., and Shi, L. (2017). Burrow Characteristics and Microhabitat Use of the Turpan Wonder Gecko Teratoscincus Roborowskii (Squamata, Gekkonidae). Asian Herpetol. Res. 8, 61–69. doi:10.16373/j.cnki.ahr.160028
Stallins, J. A. (2006). Geomorphology and Ecology: Unifying Themes for Complex Systems in Biogeomorphology. Geomorphology 77, 207–216. doi:10.1016/j.geomorph.2006.01.005
Su, J., Wang, J., Hua, L., Gleeson, D., and Ji, W. (2013). Complete Mitochondrial Genome of the Gansu zokor,Eospalax cansus(Rodentia, Spalacidae). Mitochondrial DNA 24, 651–653. doi:10.3109/19401736.2013.772166
Su, J., Ji, W., Li, H., Yao, T., Wang, J., and Nan, Z. (2020). Zokor Disturbances Indicated Positive Soil Microbial Responses with Carbon Cycle and mineral Encrustation in alpine Grassland. Ecol. Eng. 144, 105702. doi:10.1016/j.ecoleng.2019.105702
Sui, D.-D., Wu, J.-L., Zhang, H., Li, H., Zhou, Z.-M., Zhang, D.-H., et al. (2014). Molecular Cloning, Structural Analysis, and Expression of Zona Pellucida Glycoprotein ZP3 Gene from Chinese Zokor, Myospalax Fontanierii. Mol. Biol. 48, 646–654. doi:10.1134/S0026893314050148
Verachtert, E., Van Den Eeckhaut, M., Poesen, J., and Deckers, J. (2010). Factors Controlling the Spatial Distribution of Soil Piping Erosion on Loess-Derived Soils: A Case Study from central Belgium. Geomorphology 118, 339–348. doi:10.1016/j.geomorph.2010.02.001
Verachtert, E., Van Den Eeckhaut, M., Poesen, J., and Deckers, J. (2013). Spatial Interaction between Collapsed Pipes and Landslides in Hilly Regions with Loess-Derived Soils. Earth Surf. Process. Landforms. 38, 826–835. doi:10.1002/esp.3325
Vleck, D. (1981). Burrow Structure and Foraging Costs in the Fossorial Rodent, Thomomys bottae. Oecologia 49, 391–396. doi:10.1007/BF00347605
Voiculescu, M., Ianăş, A.-N., and Germain, D. (2019). Exploring the Impact of Snow Vole (Chionomys Nivalis) Burrowing Activity in the Făgăraș Mountains, Southern Carpathians (Romania): Geomorphic Characteristics and Sediment Budget. Catena 181, 104070. doi:10.1016/j.catena.2019.05.016
Voigt, C. (2014). An Example of Burrow System Architecture of Dispersing Damaraland Mole-Rats. Afr. Zoolog. 49, 148–152. doi:10.1080/15627020.2014.11407627
Wang, T., Wu, J., Kou, X., Oliver, C., Mou, P., and Ge, J. (2010). Ecologically Asynchronous Agricultural Practice Erodes Sustainability of the Loess Plateau of China. Ecol. Appl. 20, 1126–1135. doi:10.1890/09-0229.1
Wang, L., Li, X.-A., Li, L.-C., Hong, B., and Liu, J. (2019). Experimental Study on the Physical Modeling of Loess Tunnel-Erosion Rate. Bull. Eng. Geol. Environ. 78, 5827–5840. doi:10.1007/s10064-019-01495-1
Wilson, G. V., Rigby, J. R., and Dabney, S. M. (2015). Soil Pipe Collapses in a Loess Pasture of Goodwin Creek Watershed, Mississippi: Role of Soil Properties and Past Land Use. Earth Surf. Process. Landforms 40, 1448–1463. doi:10.1002/esp.3727
Winchell, E. W., Anderson, R. S., Lombardi, E. M., and Doak, D. F. (2016). Gophers as Geomorphic Agents in the Colorado Front Range Subalpine Zone. Geomorphology 264, 41–51. doi:10.1016/j.geomorph.2016.04.003
Wu, L., Jiang, J., Li, G.-x., and Ma, X.-y. (2018). Characteristics of Pulsed Runoff-Erosion Events under Typical Rainstorms in a Small Watershed on the Loess Plateau of China. Sci. Rep. 8, 1–12. doi:10.1038/s41598-018-22045-x
Xu, J. (1999). Erosion Caused by Hyperconcentrated Flow on the Loess Plateau of China. Catena 36, 1–19. doi:10.1016/S0341-8162(99)00009-0
Yoo, K., Amundson, R., Heimsath, A. M., and Dietrich, W. E. (2005). Process-Based Model Linking Pocket Gopher (Thomomys bottae) Activity to Sediment Transport and Soil Thickness. Geology 33, 917–920. doi:10.1130/G21831.1
Yu, C., Zhang, J., Pang, X. P., Wang, Q., Zhou, Y. P., and Guo, Z. G. (2017). Soil Disturbance and Disturbance Intensity: Response of Soil Nutrient Concentrations of alpine Meadow to Plateau Pika Bioturbation in the Qinghai-Tibetan Plateau, China. Geoderma 307, 98–106. doi:10.1016/j.geoderma.2017.07.041
Zaitlin, B., and Hayashi, M. (2012). Interactions between Soil Biota and the Effects on Geomorphological Features. Geomorphology 157-158, 142–152. doi:10.1016/j.geomorph.2011.07.029
Zhang, Y., Zhang, Z., and Liu, J. (2003). Burrowing Rodents as Ecosystem Engineers: The Ecology and Management of Plateau Zokors Myospalax Fontanierii in alpine Meadow Ecosystems on the Tibetan Plateau. Mammal Rev. 33, 284–294. doi:10.1046/j.1365-2907.2003.00020.x
Zhang, J., Li, J., Guo, B., Ma, Z., Li, X., Ye, X., et al. (2016). Magnetostratigraphic Age and Monsoonal Evolution Recorded by the Thickest Quaternary Loess deposit of the Lanzhou Region, Western Chinese Loess Plateau. Quat. Sci. Rev. 139, 17–29. doi:10.1016/j.quascirev.2016.02.025
Zhang, Y., Hu, Z., Li, L., and Xue, Z. (2018). Improving the Structure and Mechanical Properties of Loess by Acid Solutions - an Experimental Study. Eng. Geol. 244, 132–145. doi:10.1016/j.enggeo.2018.07.023
Zhang, X., Zou, Y., Nan, X., and Han, C. (2021). DNA Metabarcoding Uncover the Diet of Subterranean Rodents in China. bioRxiv [Preprint]. Available at: https://www.biorxiv.org/content/10.1101/2021.09.20.461124v1.full.(Accessed September 20, 2021).
Zhao, G., Mu, X., Wen, Z., Wang, F., and Gao, P. (2013). Soil Erosion, Conservation, and Eco-Environment Changes in the Loess Plateau of China. Land Degrad. Develop. 24, 499–510. doi:10.1002/ldr.2246
Zhao, J., Tian, L., Wei, H., Zhang, T., Bai, Y., Li, R., et al. (2021). Impact of Plateau Pika (Ochotona Curzoniae) Burrowing-Induced Microtopography on Ecosystem Respiration of the alpine Meadow and Steppe on the Tibetan Plateau. Plant Soil 458, 217–230. doi:10.1007/s11104-019-04122-w
Zhou, W. Y., and Dou, F. M. (1990). Studies on Activity and home Range of Plateau Zokor. Aacta Theriol. Sin. 10, 31–39. doi:10.16829/j.slxb.1990.01.00610.1016/0956-053x(90)90069-w
Zhou, X. R., Guo, Z. G., and Guo, X. H. (2010). The Role of Plateau Pika and Plateal Zokor in alpine Meadow. Pratacultural ence 27, 38–44. doi:10.3724/SP.J.1011.2010.01351
Zhu, T. X., Luk, S. H., and Cai, Q. G. (2002). Tunnel Erosion and Sediment Production in the Hilly Loess Region, North China. J. Hydrol. 257, 78–90. doi:10.1016/S0022-1694(01)00544-3
Zhu, T. X. (1997). Deep-seated., Complex Tunnel Systems - a Hydrological Study in a Semi-arid Catchment, Loess Plateau, China. Geomorphology 20, 255–267. doi:10.1016/s0169-555x(97)00027-5
Zhu, T. X. (2003). Tunnel Development over a 12 Year Period in a Semi-arid Catchment of the Loess Plateau, China. Earth Surf. Process. Landforms. 28, 507–525. doi:10.1002/esp.455
Zhu, T. X. (2006). Initiation and Development Processes of Tunnel Systems in the Hilly Loess Region of Northern China. Int. J. Sediment. Res. 21, 171–179. doi:10.3724/SP.J.1011.2010.01351
Zhu, T. X. (2012). Gully and Tunnel Erosion in the Hilly Loess Plateau Region, China. Geomorphology 153-154, 144–155. doi:10.1016/j.geomorph.2012.02.019
Keywords: loess cave, biotic burrowing, Chinese Loess Plateau, biotic disturbance, forming mechanism, landscape evolution
Citation: Geng H, Liu R, Zheng W, Zhang Y, Xie R, Guo Y and Pan B (2021) Interaction Between Animal Burrowing and Loess Cave Formation in the Chinese Loess Plateau. Front. Earth Sci. 9:806921. doi: 10.3389/feart.2021.806921
Received: 01 November 2021; Accepted: 12 November 2021;
Published: 03 December 2021.
Edited by:
Mark Allen, Durham University, United KingdomCopyright © 2021 Geng, Liu, Zheng, Zhang, Xie, Guo and Pan. This is an open-access article distributed under the terms of the Creative Commons Attribution License (CC BY). The use, distribution or reproduction in other forums is permitted, provided the original author(s) and the copyright owner(s) are credited and that the original publication in this journal is cited, in accordance with accepted academic practice. No use, distribution or reproduction is permitted which does not comply with these terms.
*Correspondence: Haopeng Geng, hpgeng@lzu.edu.cn