- 1School of Earth and Environmental Sciences, Cardiff University, Cardiff, United Kingdom
- 2Institut de Physique du Globe de Paris, Université de Paris, CNRS, Paris, France
Lavas produced at subduction zones represent the integration of both source heterogeneity and an array of crustal processes, such as: differentiation; mixing; homogenisation; assimilation. Therefore, unravelling the relative contribution of the sub-arc mantle source versus these crustal processes is difficult when using the amalgamated end products in isolation. In contrast, plutonic xenoliths provide a complementary record of the deeper roots of the magmatic plumbing system and provide a unique record of the true chemical diversity of arc crust. Here, we present the δ56Fe record from well characterised plutonic xenoliths from two distinct volcanic centres in the Lesser Antilles volcanic arc–the islands of Martinique and Statia. The primary objective of this study is to test if the Fe isotope systematics of arc lavas are controlled by sub-arc mantle inputs or during subsequent differentiation processes during a magma’s journey through volcanic arc crust. The Fe isotopic record, coupled to petrology, trace element chemistry and radiogenic isotopes of plutonic xenoliths from the two islands reveal a hidden crustal reservoir of heavy Fe that previously hasn’t been considered. Iron isotopes are decoupled from radiogenic isotopes, suggesting that crustal and/or sediment assimilation does not control the Fe systematics of arc magmas. In contrast to arc lavas, the cumulates from both islands record MORB-like δ56Fe values. In Statia, δ56Fe decreases with major and trace element indicators of differentiation (SiO2, Na2O + K2O, Eu/Eu*, Dy/Yb), consistent with fractionating mineral assemblages along a line of liquid descent. In Martinique, δ56Fe shows no clear relationship with most indicators of differentiation (apart from Dy/Yb), suggesting that the δ56Fe signature of the plutonic xenoliths has been overprinted by later stage processes, such as percolating reactive melts. Together, these data suggest that magmatic processes within the sub-arc crust overprint any source variation of the sub-arc mantle and that a light Fe source is not a requirement to produce the light Fe isotopic compositions recorded in volcanic arc lavas. Therefore, whenever possible, the complimentary plutonic record should be considered in isotopic studies to understand the relative control of the mantle source versus magmatic processes in the crust.
Introduction
Subduction zone magmas represent the integrated result of both source heterogeneity and an array of crustal processes, such as differentiation, mixing, homogenisation and assimilation. Therefore, unravelling the relative contribution of the sub-arc mantle source versus subsequent passage through the crust is difficult when using the erupted end products (lavas) alone, which represent the amalgamation of all these processes. In contrast, plutonic xenoliths provide a complementary record of the deeper roots of the magmatic plumbing system and provide a unique record of the true chemical diversity of arc crust. In the Lesser Antilles volcanic arc (LAA) it has been shown that vertically extensive mush-dominated reservoirs make up the majority of sub-volcanic crust (Melekhova et al., 2019). Plutonic xenoliths are direct portions of this crystal mush and therefore represent a volumetrically greater proportion of the crust than the melt dominant magmas which reach the surface in eruptions. Thus, the formation of crystal mush in the deeper portions of volcanic plumbing systems likely plays a key role in governing the geochemical and isotopic composition of arc magmas (Cooper et al., 2016; Cooper et al., 2019; Brown et al., 2021). In this study, we present the first δ56Fe record from plutonic xenoliths, in order to test if the Fe isotope systematics of sub-arc mantle inputs can be inferred from arc lavas, or are modified through subsequent differentiation processes in the sub-arc crust during storage and ascent.
The stable isotopes of Fe have been shown to be an effective tracer of petrogenetic processes in magmatic systems (Johnson et al., 2004; Dauphas et al., 2017). As a redox sensitive major element, it is predicted that Fe equilibrium isotope fractionation occurs due to changes in oxidation state, with the Fe3+ bearing phases being more enriched in the heavier isotopes of Fe. In addition, the coordination chemistry of Fe between different mineral phases and melts/fluids will drive an isotopic fractionation, with high-fold coordinated sites forming shorter and stiffer bonds, preferentially incorporating the lighter isotopes. Therefore, owing to their more oxidised nature (Kelley and Cottrell, 2009), it is predicted that subduction zone melts (FMQ = +1) would exhibit Fe isotope compositions that are heavier than MORB (FMQ = 0). Despite this the inverse is observed (e.g., Nebel et al., 2015; Foden et al., 2018; Nebel et al., 2018). For example, Foden et al. (2018) studied 130 primitive arc lavas from 15 different global arcs and found them to be systematically lighter (δ56Fe = 0.044 ± 0.12‰ 2sd) than the suggested MORB value (δ56Fe = 0.11 ± 0.04‰ 2sd) (Teng et al., 2013). It is suggested a possible mechanism for the light δ56Fe values observed within the lavas could be the repeated re-melting of a DMM like source. Other studies have sought to examine the Fe isotope systematics of subduction zones by analysing metamorphosed slab serpentinites and crustal lithologies (Debret et al., 2016; El Korh et al., 2017; Inglis et al., 2017; Debret et al., 2018; Gerrits et al., 2019). While the mafic oceanic crust remains isotopically unfractionated during subduction zone metamorphism, the devolatilization of hydrous slab serpentinites is suggested to release an isotopically light component into dehydration fluids. Recent work has demonstrated covariation between δ56Fe values and indicators of slab fluid addition in sub-arc peridotite xenoliths (δ56Fe vs. U/Th, 206Pb/204Pb), thus suggesting that isotopically light slab fluids can serve an important role in metasomatising the sub arc mantle (Turner et al., 2018). This notion is further reinforced by the recent study of Fe isotopes in forearc serpentinite clasts, which are shown to preserve light Fe isotope compositions, thought to reflect metasomatism by isotopically light fluids (Debret et al., 2020). Despite this, it is argued by Foden et al. (2018) that the wide range of δ56Fe values observed for different arcs cannot be explained solely by the influx of light Fe from the slab, and that other processes must be evoked to account for the δ56Fe values of arc lavas, such as magmatic differentiation (Li et al., 2020; Chen et al., 2021). It is now widely accepted that the mid-lower crust is a primary location for a wide variety of magmatic differentiation processes. Deep Crustal Hot Zones (Annen et al., 2006; Solano et al., 2012), where mantle derived magmas stall and differentiate could therefore be a key site of isotope fractionation that has previously been overlooked.
Here, we present δ56Fe values from well characterised (petrology, geochemistry, radiogenic isotopes) plutonic xenoliths from two distinct volcanic centres. These samples represent direct portions of the deeper plutonic roots of magmatic plumbing systems. By combining δ56Fe compositions with radiogenic isotopes, we have an opportunity to see through any variations in the mantle source to reveal the role of the crust in governing the Fe isotope systematics of arc lavas.
The Lesser Antilles Arc and Sample Selection
The Lesser Antilles volcanic arc (LAA) is an ideal location to investigate the relative contributions of subduction zone inputs versus subsequent crustal differentiation processes due to a very large range in elemental and isotopic compositions in the volcanic record. The Lesser Antilles (Figure 1) is an example of an oceanic arc with ‘continental’ geochemical and isotopic signatures (White and Dupré 1986: Davidson 1987; Macdonald et al., 2000) in the central-southern segments. In contrast, the islands in the northern segment have typical oceanic arc compositions. Current plate reconstructions (Müller et al., 2019; Braszus et al., 2021) show that the northern LAA is underlain by ∼90 Ma Equatorial Atlantic lithosphere, whereas the subducted lithosphere beneath the southern LAA is formed at the proto-Caribbean mid-ocean ridge at ∼120 Ma (Figure 1; Cooper et al., 2020). This suggests that the inputs sourced from the slab in the north LAA versus the central-south LAA may have distinct geochemical signatures. Therefore, we have undertaken an isotopic study of plutonic xenoliths and volcanics from Martinique (central LAA) and Statia (northern LAA) to compare the relative role of the crust versus the mantle source beneath two distinct volcanic systems. These islands contrast in age, volume and predicted volcanic production rates (Cooper et al., 2020). Statia is relatively young with all deposits <1 Ma (Roobol and Smith, 2004). All samples used in this study are inferred to have been erupted from the Quill, which was active from 22.24 to 1.55 kyr (Roobol and Smith, 2004). Martinique has a longer volcanic record spanning at least the last 25 Myr (Germa et al., 2011) and this is reflected in the largest range of radiogenic isotopes of any island in the Lesser Antilles (Davidson 1986; Labanieh et al., 2010). The plutonic xenoliths sampled for this study are inferred to have been erupted from Mt Pelée (126–0 ka) and Mount Conil (550–127 ka) (Labanieh et al., 2012).
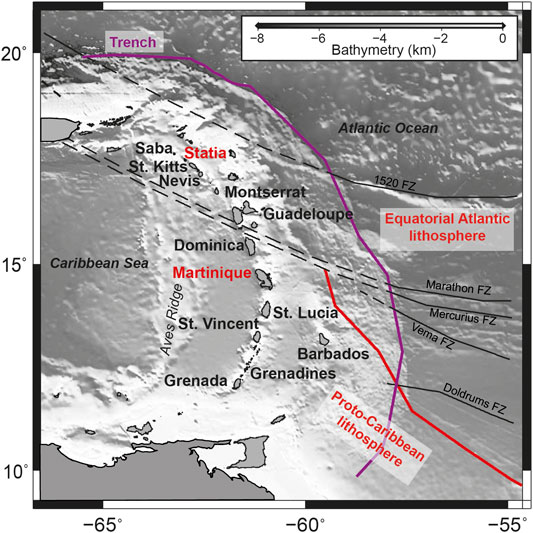
FIGURE 1. Bathymetric map of the Lesser Antilles. The locations of Statia (northern LAA) and Martinique (central LAA) sampled for this study are shown in red text. The boundary (red line) between the proto-Caribbean and equatorial Atlantic seafloor is shown (Map adapted from Cooper et al., 2020).
The plutonic xenolith samples from Statia and Martinique are described in detail in Cooper et al. (2019) and Cooper et al. (2016) respectively. The plutonic xenoliths erupted from both islands have common mineral assemblages, dominated by plagioclase and amphibole, but also contain variable proportions of olivine, clinopyroxene, orthopyroxene and oxides (Supplementary File S1). The abundance of amphibole in the plutonic xenoliths is in contrast to the lavas of both islands, where amphibole is rare and/or antecrystic. This ‘cryptic’ amphibole fractionation–referred to as the ‘amphibole sponge’—is a common process in volcanic arcs worldwide and imparts a control on the geochemical trends observed in lavas (Davidson et al., 2007; Smith 2014). The range of textures of the plutonic xenoliths from both islands is diverse, but to a first order, these samples can be divided into either cumulates, which represent a subtractive assemblage, or non-cumulate gabbros (NCGs), which have a plutonic origin and likely represent solidified portions of the final erupted magmas. These types are distinctive in texture, mineralogy and mineral chemistry and therefore likely represent different portions of vertically extensive mush-dominated plumbing systems (Melekhova et al., 2019).
The plutonic xenoliths provide evidence for open system behaviour within these vertically extensive mush zones. In Statia, plutonic xenolith hosted melt inclusions preserve a record of melt differentiation and range in composition from basalt to rhyolite along liquid lines of descent. This range in melt chemistry is greater than that of volcanic whole rocks, revealing that volumetrically large mush zones within the plumbing system beneath Statia contain a large diversity of melts which are filtered by the crust prior to eruption (Cooper et al., 2019). In Martinique, the plutonic xenoliths provide textural and geochemical evidence for mixed crystal cargos and are characterised by the presence of both early and late-stage crystallisation of amphibole (Supplementary File S1). This late-stage amphibole formed through the breakdown of clinopyroxene in the presence of a water-rich, plagioclase-undersaturated reactive melt or fluid which percolated through the crystal mush (Smith 2014; Cooper et al., 2016). Therefore, the chemistry of the cumulus phases within the plutonic xenoliths may be overprinted by later-stage (post-cumulus) open system magmatic processes. Taken together these two complimentary sample suites provide an excellent opportunity to examine the role of cumulate fractionation and magmatic processes occurring during storage and ascent through the crust in controlling the Fe isotopic composition of the resultant arc lavas.
Analytical Techniques
The petrology, mineral chemistry and whole rock major and trace elements of a large sample set of sample set of Martinique and Statia plutonic xenoliths were originally described by Cooper et al. (2016) and Cooper et al. (2019) respectively. Whole-rock isotope data from Martinique plutonic xenoliths were originally published in Brown et al. (2021) but are compared to the record from Statia here. To provide key context for stable Fe isotope analysis, we chose a representative subset of the plutonic xenolith samples described in these studies, covering a range of mineral assemblages and whole-rock geochemistry (Supplementary Data).
Radiogenic Sr, Nd and Pb Isotopes
The radiogenic isotope compositions of Sr, Nd, and Pb were measured in the Arthur Holmes isotope Geology Laboratory at the University of Durham (United Kingdom), see Brown et al. (2021) for full analytical techniques. Briefly, whole rock powders were digested in concentrated HF + HNO3 acids, before being processed on columns containing Eichrom Sr Spec resin. In this column chemistry, the REE fraction containing Nd and the bulk of the matrix was eluted in 3M HNO3. After the matrix was eluted, Sr was collected in MQ H2O and then Pb was eluted in 8M HCl. The matrix-REE aliquot from the first column was then further purified on Bio-rad Polyprep columns containing 2 ml of AG 50 W- X8 cation resin. All isotope analyses were measured on a Thermo Finnigan Neptune MC-ICPMS. Samples were introduced as a 3% HNO3 solution using an ESI PFA50 self-aspirating nebuliser coupled to a Glass Expansion ‘cinnabar’ micro-cyclonic spray chamber. This combination yielded typical sensitivities at equivalent concentrations of 1 μg/g for Sr-Nd-Pb of ∼60 V total Sr, 60–70 total Nd and ∼90 V ppm−1 total Pb with an uptake rate of ∼50 μl min−1. All analyses comprised one block of 50 cycles with an integration time of 4 s per cycle with a total analysis time ∼3.5 min. During mass spectrometry the isotope reference materials NIST NBS 987, NIST NBS 981 and an internal Nd isotope standard J&M were measured during the Sr, Pb and Nd analytical sessions respectively. The average 87Sr/86Sr for NBS 987 was 0.710272 ± 0.000016 (2SD, n = 11). The average of NBS 981 was 206Pb/204Pb = 16.94157 ± 0.00067, 207Pb/204Pb 15.49947 ± 0.00085, 208Pb/204Pb = 36.72158 ± 0.00263 (n = 10). The average 143Nd/144Nd ratio for J&M was 0.511113 ± 0.000010 (2SD n = 16). Repeat digestions and analyses of unleached aliquots of USGS BHVO-1 basalt reference material analysed over many analytical sessions in Durham yielded an average 87Sr/86Sr ratio of 0.703475 ± 0.000022 (2SD, n = 31) and a143Nd/144Nd ratio of 0.512983 ± 0.000010 2SD (20ppm 2RSD, n = 32). BHVO-1 measured alongside the samples in this study gave 87Sr/86Sr = 0.0703474 ± 0.000008 2se, 143Nd/144Nd = 0.512988 ± 0.000003 1se, 206Pb/204Pb = 18.69108 ± 0.00053 2se. These values are in excellent agreement with the preferred values given by the GeoREM database (Jochum et al., 2005).
Iron Stable Isotopes
The Fe isotope measurements were performed at the Arthur Holmes Isotope Geology Laboratory at the University of Durham, United Kingdom. Prior to column chemistry samples were fully dissolved using concentrated HF + HNO3 acids and evaporated to incipient dryness before being re-dissolved in concentrated HCl to further decompose any residues which may have formed by the addition of HF acid in the initial dissolution step. The pure and quantitative separation of Fe from matrix elements was achieved by following the established protocol of Dauphas et al. (2004). Prior to column chemistry the sample residues were brought into solution in 6M HCl, these sample aliquots were then passed on a column containing 1.2 ml of BioRad AG1-X8 (200–400 mesh) anion exchange resin. The matrix was eluted from the column by the addition of 8 ml of 6M HCl, before finally eluting the Fe in 9 ml of 0.4M HCl. Samples were analysed on a Thermo Scientific Neptune Plus MC-ICPMS following the protocols described by Weyer and Schwieters (2003) but are briefly described here. The instrument was run in wet plasma mode using a quartz SIS spray chamber. Medium resolution mode was used to separate analyte peaks from interferences occupying the same nominal mass space. Samples were measured 3–4 times each with data acquisition consisting of one block of 50 cycles of 4 s each. Instrumental mass bias was corrected using the standard sample bracketing technique, where IRMM-014 was used as both the bracketing and reporting standard. Final Fe isotope values are expressed in standard delta notation as the permille deviation of the 56Fe/54Fe ratio in the sample relative to the standard. During analyses, an internal pure Fe wire standard (DuFeW) was measured to monitor instrument performance. The values obtained for this standard were δ56Fe = 0.225 ± 0.024‰ (2SD) and δ57Fe = 0.376 ± 0.044 (2SD) (n = 28). The external reproducibility of the method was assessed by analysing the USGS reference material BIR-1. The values obtained for the BIR-1 reference material are δ56Fe = 0.06 ± 0.028‰ (2SD) and δ57Fe = 0.09 ± 0.021 (2SD) (n = 4), which is in excellent agreement with previous published values (Millet et al., 2012).
Results
The major and trace element data and the radiogenic and Fe stable isotope compositions of the samples measured as part of this study are presented in the supplementary data table. Plutonic xenoliths from both Statia (41–58 wt% SiO2, 3–13 wt% MgO) and Martinique (40–57 wt% SiO2, 2–10 wt% MgO) cover a large range in major element compositions. In cumulate samples, this a reflection of their variable mineral assemblages, whereas in non-cumulate gabbros (NCGs) the compositional ranges reflect the chemistry of the melts from which the samples crystallised (Cooper et al., 2016; Cooper et al., 2019). In addition to textural differences [e.g., oscillatory zoned plagioclase in NCGs (Supplementary File S1)], cumulate and NCG samples can also be distinguished based on their trace element signatures. Multi-element normalised diagrams (Figure 2) of whole rock trace elements highlight the similarity of concentrations and shape of patterns from both islands, however Martinique samples display a greater range than those from Statia. This is particularly evident in terms of more incompatible elements in NCG’s and lava samples, and more compatible elements in cumulates.
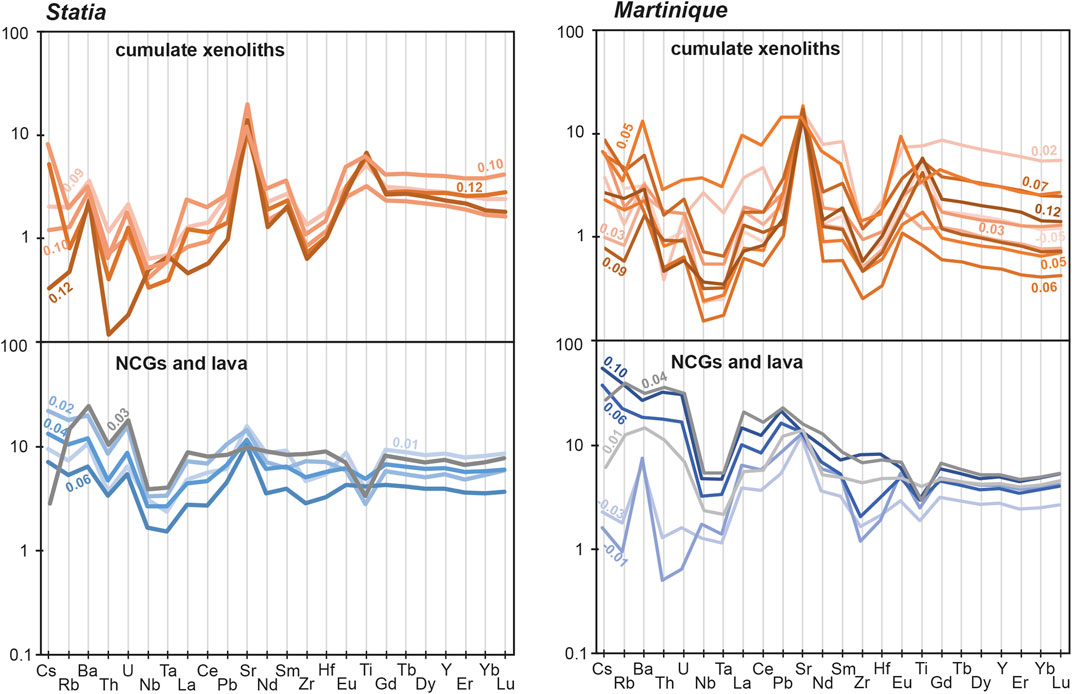
FIGURE 2. Multi-element normalised diagrams of Statia and Martinique whole-rock analyses divided into cumulates (orange lines) and NCG’s (blue lines) and Lavas (grey lines). Measured δ56Fe values of samples are labelled on each line.
Whole-rock isotope data from Martinique plutonic xenoliths was originally published in Brown et al. (2021) but are compared to the record from Statia here. Plutonic xenoliths from both islands display significant variations in 87Sr/86Sr, 206Pb/204Pb and 143Nd/144Nd isotope ratios (Figure 3). The range in radiogenic isotopes is significantly greater in samples from Martinique (87Sr/86Sr = 0.7039–0.7046, 206Pb/204Pb = 19.12–19.91, 143Nd/144Nd = 0.51274–0.51290) than Statia (87Sr/86Sr = 0.7036–0.7040, 206Pb/204Pb = 18.43–19.09, 143Nd/144Nd = 0.51295–0.51307) (Figure 3). These ranges do not overlap each other, with Martinique lavas and plutonic xenoliths having more radiogenic Sr and Pb, and less radiogenic Nd than those at Statia. The plutonic xenoliths from both islands have lower SiO2 wt%, but notably cover a similar range in Sr, Pb, Nd isotope ratios to the lavas. Both 87Sr/86Sr and 206Pb/204Pb increase with differentiation (increasing SiO2 wt%) in the lavas (particularly evident in Martinique). Within the plutonic xenolith samples, 87Sr/86Sr also increases with increasing SiO2 wt%, although there is a large range in 87Sr/86Sr between 40–50 wt% SiO2 at both islands. Trends in 206Pb/204Pb and 143Nd/144Nd with SiO2 of the plutonic xenoliths are not as clear.
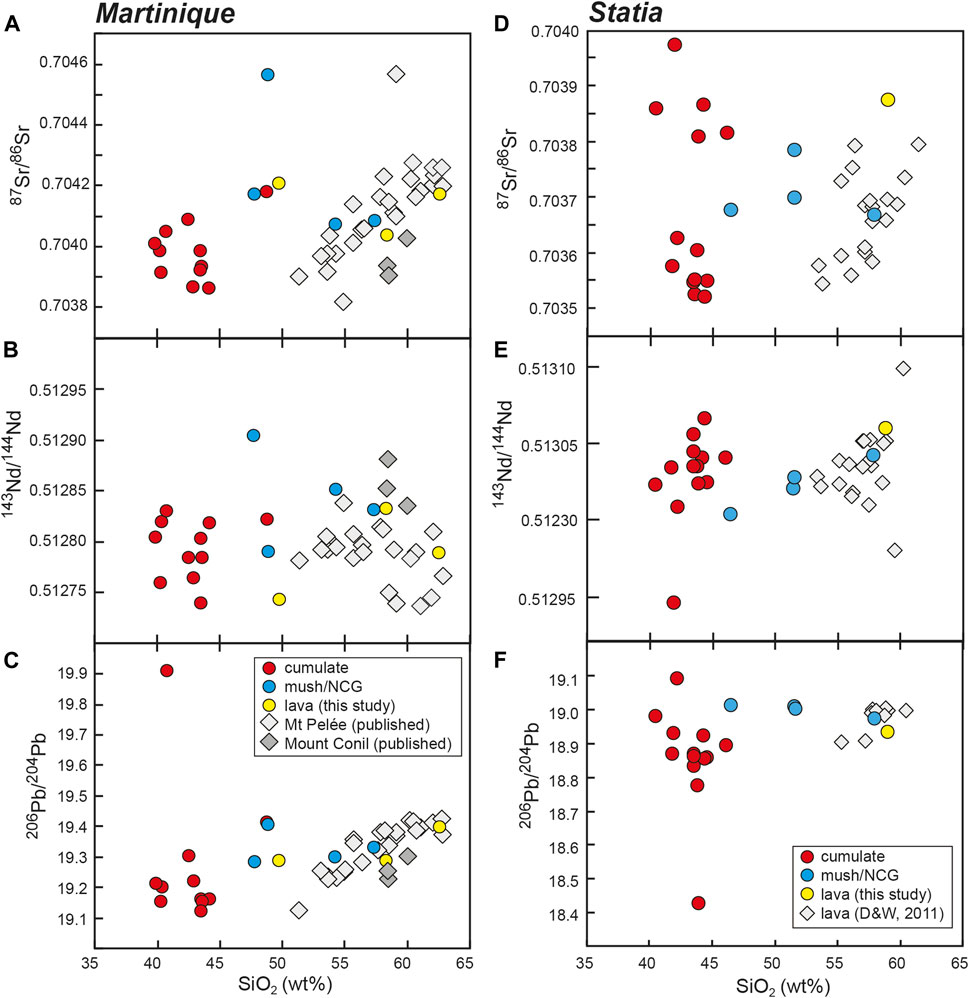
FIGURE 3. Radiogenic isotopes (87Sr/86Sr, 143Nd/144Nd and 206Pb/204Pb) versus SiO2 (wt%) of whole-rock samples (coloured circles) from Martinique (A–C) and Statia (D–F) analysed in this study (Martinique data originally published in Brown et al., 2021).
The Fe isotope composition of the measured plutonic xenoliths range from δ56Fe = +0.12 to -0.01‰ in Statia and from +0.12 to -0.05‰ in Martinique. At Statia, the heaviest values are found in cumulates, and lighter values are found in NCGs and lava samples. There is a trend of decreasing δ56Fe with indicators of differentiation (increasing SiO2, Na2O + K2O and Rb/Sr), consistent with the fractionating mineral assemblage (Figure 4). In addition, δ56Fe also correlates with trace element indicators of mineral fractionation, such as plagioclase (Eu/Eu*), amphibole (Dy/Yb) (Figure 5). The V/Sc ratio of all but one cumulate sample is higher than in NCGs and lavas, (Figure 5), but this likely reflects of the presence of oxides within these samples [cumulate with no oxides (Supplementary File S1) has lower V/Sc], rather than tracing variations in oxygen fugacity (e.g., Lee et al., 2005). The cumulate samples from Martinique extend to the same heavy δ56Fe (+0.12‰) as Statia, but cover a larger range in δ56Fe (+0.12 to -0.05‰) than NCGs (+0.10 to -0.03‰). The Fe isotopes of cumulate samples show no clear relationship with indicators of differentiation (e.g., Eu/Eu*, Dy/Yb), with a large range in δ56Fe (0.17‰) over a narrow range in SiO2 (40–44 wt% SiO2, with one exception at 49 wt% SiO2). In contrast to Statia, the Fe isotope values of Martinique NCGs increase (from δ56Fe -0.03 to +0.10‰) with increasing SiO2 (48–57 wt%). These samples also display a trend of increasing δ56Fe with decreasing Dy/Yb and increasing Rb/Sr, but show no clear relationship with other trace elements (Figure 5). The multi-element normalised diagrams highlight the contrasting relationship between δ56Fe and trace elements in NCGs from the two islands (Figure 2). Statia NCGs show progressively lighter δ56Fe values with increasing concentration of incompatible elements, whereas Martinique NCG’s generally show the inverse relationship. The Fe isotopes from both sample suites show no discernible correlations to radiogenic isotopes (87Sr/86Sr, 206Pb/204Pb and 143Nd/144Nd) (Figure 6).
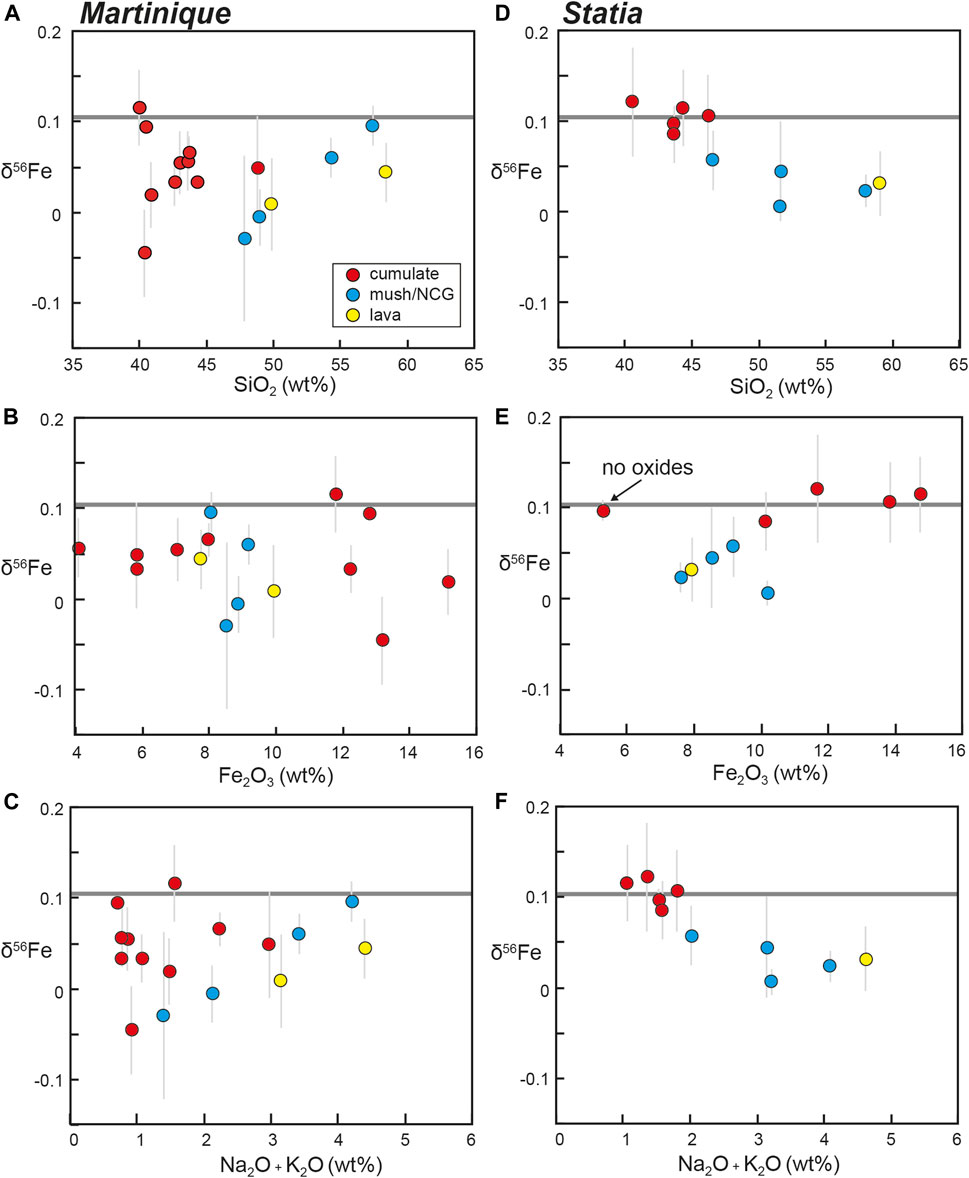
FIGURE 4. Measured δ56Fe values versus major elements of cumulates (red circles), NCG’s (blue circles) and lavas (yellow circles) from Martinique (A–C) and Statia (D–F).
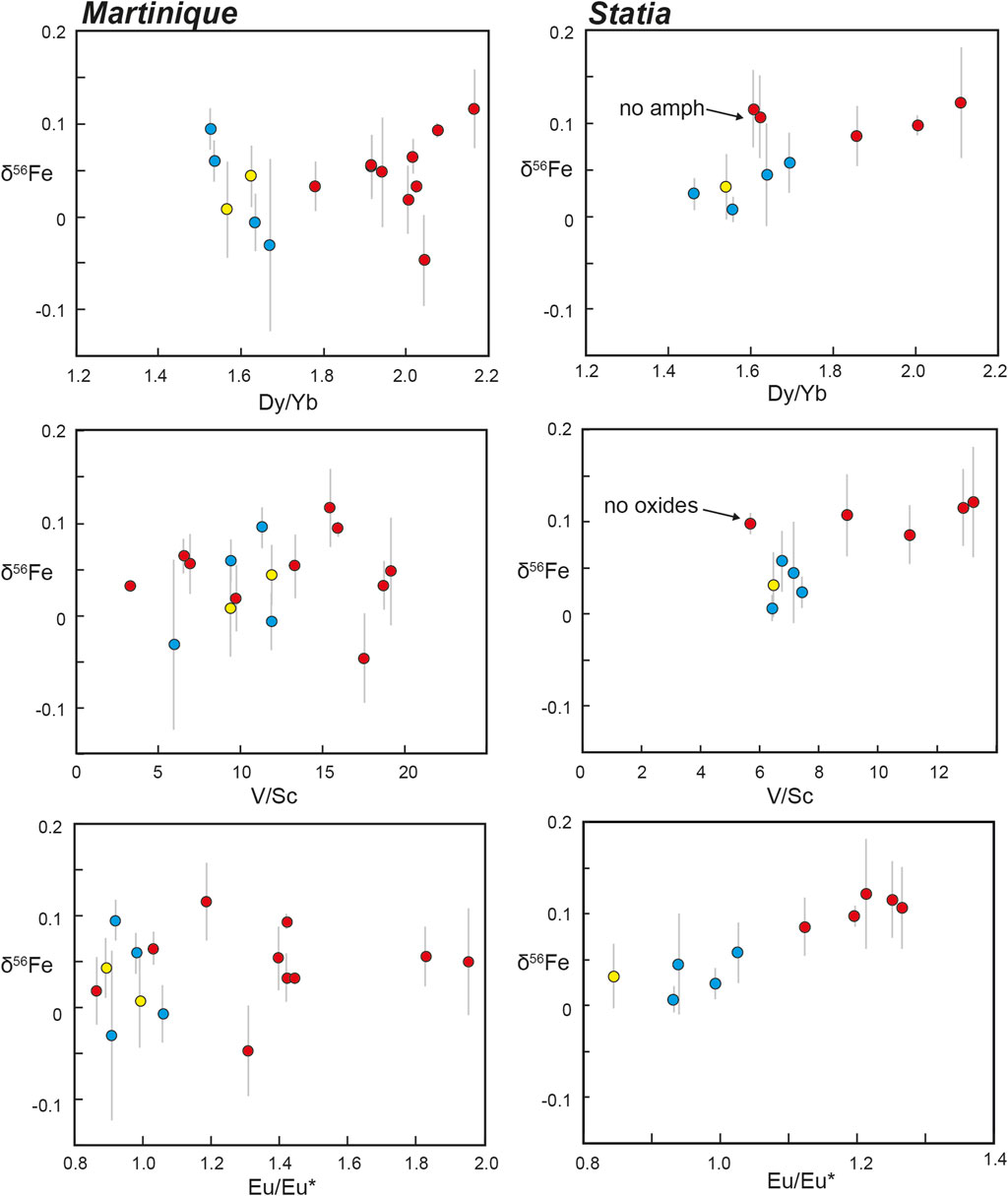
FIGURE 5. Measured δ56Fe values versus Dy/Yb, V/Sc and Eu/Eu* of cumulates (red circles), NCG’s (blue circles) and lavas (yellow circles) from Martinique (A–C) and Statia (D–F).
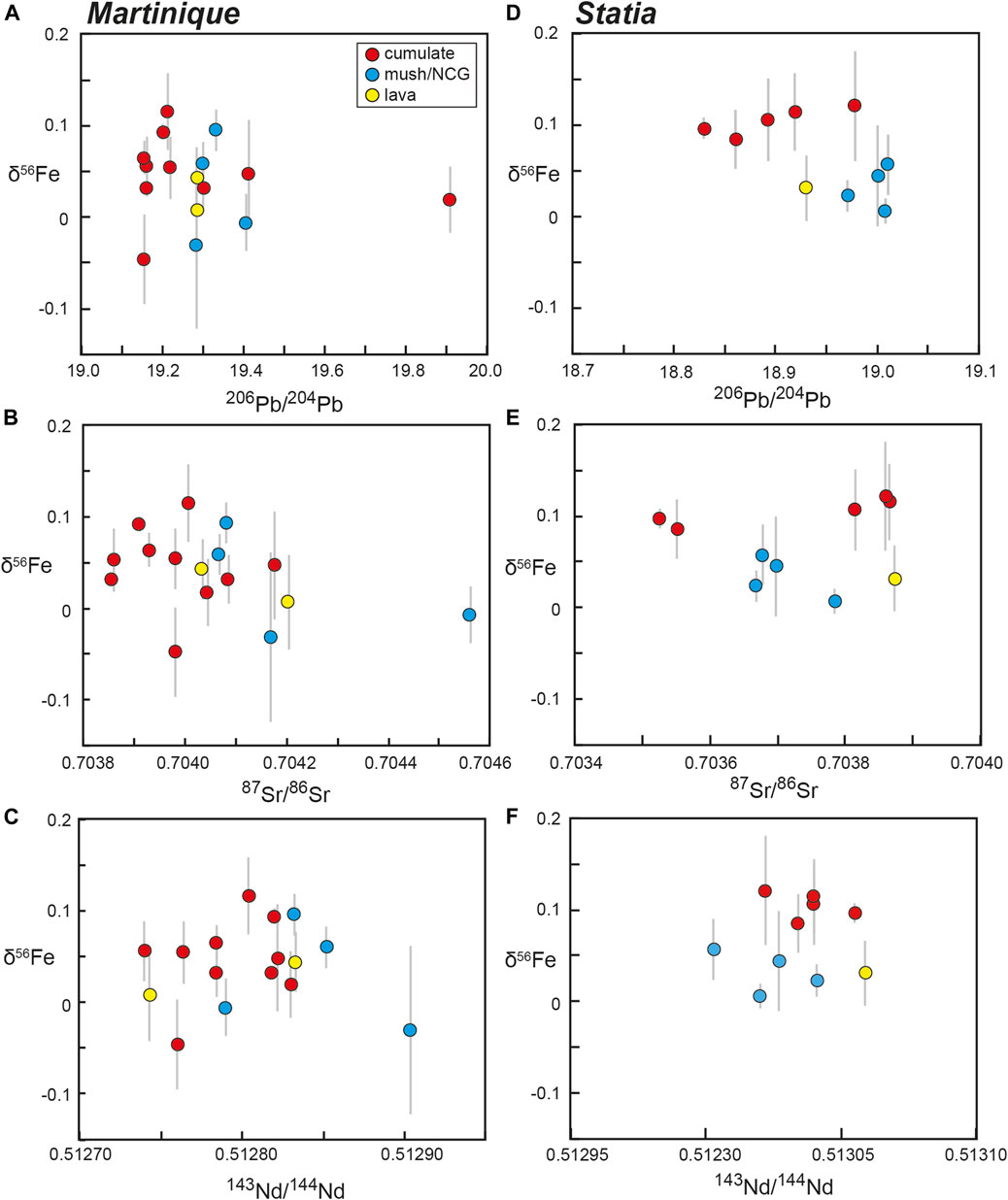
FIGURE 6. Radiogenic isotopes (87Sr/86Sr, 143Nd/144Nd and 206Pb/204Pb) versus δ56Fe values of cumulates (red circles), NCG’s (blue circles) and lavas (yellow circles) from Martinique (A–C) and Statia (D–F).
Discussion
Source Variation Versus Crustal Assimilation
Before considering the Fe isotope systematics of LAA plutonic xenoliths and lavas, it is important to investigate geochemical and radiogenic isotopic signatures, to assess the control of variations in source and subsequent crustal processes such as differentiation, mixing and assimilation. Davidson and Wilson (2011) compared the geochemical and Pb, Sr and Nd isotopic signatures of volcanic rocks from both Martinique (Mt Pelée) and Statia (the Quill). This study found that the compositions of extrapolated parental magmas at the two islands are distinct as differentiation trends do not converge on a reasonable common parent. These isotopic differences can be explained by variations in slab contributions along the arc (Carpentier et al., 2008; DuFrane et al., 2009), but cannot be the result of simple sediment-mantle mixing and subsequent melting of the source (Davidson and Wilson, 2011). Therefore, the addition of fluids as well as variations in the amount and/or type of sediment contaminating the mantle source towards the south of the LAA need to be considered. The inferred parental magmas at Martinique have a stronger sediment signature (higher Th/La, La/Yb, 87Sr/86Sr and 206Pb/204Pb) than in Statia, although these trends are not systematic along the whole length of the arc (Davidson and Wilson, 2011). Our data from the complementary plutonic xenoliths support this observation, with Martinique plutonic xenoliths having more radiogenic Sr and Pb, and less radiogenic Nd than those at Statia. Current plate reconstructions (Müller et al., 2019; Braszus et al., 2021) show that the northern LAA is underlain by ∼90 Ma Equatorial Atlantic lithosphere, whereas the subducted lithosphere beneath the southern LAA is ∼120 Ma formed at the proto-Caribbean mid-ocean ridge. Therefore, it is possible that inputs sourced from the slab in the north LAA versus the central-south LAA would have district geochemical signatures, even without addition of the subducted sediment component.
There is a long-standing debate as to whether geochemical variations in the LAA and other volcanic arcs are attributed to heterogeneity in the sub arc mantle source via addition of slab derived sediments (White and Dupré, 1986; Carpentier et al., 2008; Labanieh et al., 2010; Labanieh et al., 2012; Hu et al., 2021) versus subsequent crustal modification by assimilation processes (Davidson, 1986; Davidson, 1987; Van Soest et al., 2002; Davidson and Wilson 2011; Bezard et al., 2014; Bezard et al., 2015; Brown et al., 2021). Geochemical evidence for sediment and crustal assimilation during storage in LAA magmatic plumbing systems is provided by an excess in incompatible elements in lavas compared with fractional crystallisation models and by covariations between Sr and Pb isotopes and indices of differentiation (Roobol and Smith, 2004; Davidson and Wilson, 2011). Indeed, both 87Sr/86Sr and 206Pb/204Pb increase with differentiation (increasing SiO2 wt%) in the lavas (particularly evident in Martinique) which suggest that the isotopic compositions of the primitive or parental magmas have been changed by open-system differentiation processes during ascent and storage in the crust.
We do not have any age constraints on the erupted plutonic xenoliths and therefore cannot rule out that these samples may not be directly related to their volcanic counterparts. However, the plutonic xenoliths from both Statia and Martinique cover the same Sr, Pb and Nd isotopic range as their volcanic counterparts, providing strong evidence that the plutonic xenoliths are directly associated with the final erupted lavas. This relationship suggests that either crystallisation of cumulate assemblages occurred throughout the whole differentiation of the final erupted magmas, or that the melts were extracted from an already isotopically diverse mid-crustal mush zone. In addition, the plutonic xenoliths represent a range of depths (0–15 km) within the crust (Cooper et al., 2016; Cooper et al., 2019; Melekhova et al., 2019). Therefore, we are confident that the studied samples are a good representation of the mushy trans-crustal plumbing systems within which the final erupted magmas passed through and were stored prior to eruption.
Trace element variations in cumulate samples (Figure 2) are largely a reflection of their mineralogy, plus any interstitial melt which may be present. Therefore, it is difficult to reconstruct differentiation processes using whole-rock trace element trends within these samples. NCG’s contain variable proportions of crystals and melt and likely reflect a combination of cumulus mineral growth and the solidification of melts in the mid to upper crust. In contrast, whole-rock trace element data from lava samples largely reflects the composition of melts. Therefore, we can use the differences in trace element concentrations between these different sample types to understand differentiation processes occurring throughout the crust beneath the LAA. For example, does fractionation of the cumulate mineral assemblage (primarily olivine, plagioclase, clinopyroxene, amphibole, magnetite) govern Fe isotope fractionation in the final erupted products in volcanic arcs?
Fe Isotope Systematics of LAA Crust
Radiogenic isotopes provide strong evidence for crustal and/or sediment assimilation. A recent study by Brown et al. (2021) using crystal scale Sr isotopes from Martinique plutonic xenoliths revealed that sediment assimilation largely occurs at shallow crustal levels, with Sr isotope compositions becoming larger and more radiogenic during shallow magmatic storage. However, Fe isotopes from both studied islands show no clear trends with radiogenic isotopes (Figure 6). This decoupling between radiogenic isotopes and Fe isotopes is also observed in the lavas from the Banda arc (Nebel et al., 2015). Here, it was found that subducting sediment does not influence the Fe isotope compositions of arc lavas. In addition, crustal contamination was also found to have no influence on the Fe isotope values of crystal phases in the alkaline igneous suite of the Ilímaussaq Complex, South Greenland (Schoenberg et al., 2009). We therefore suggest that crustal and/or sediment assimilation during magmatic differentiation is not the cause of the Fe isotope variations in our data. Nebel et al. (2015) corrected the composition of arc lavas for fractional crystallisation to suggest that primitive magmas of the Banda arc are isotopically light in Fe due to depletion of the sub-arc mantle source. However, this method may have missed the cryptic fractionation processes occurring deeper within the crust, which we investigate here using the plutonic xenolith samples.
The range in Fe isotopic composition of NCGs and lavas from Statia and Martinique is typical of volcanic arcs worldwide (Foden et al., 2018), with average δ56Fe values (0.04 ± 0.09‰) significantly lighter than estimates of MORB (Figure 4) (Teng et al., 2013). These light Fe isotopic compositions of oceanic arc basalts have previously been attributed to melt depletion of mantle wedge peridotites (Nebel et al., 2015; Foden et al., 2018). However, δ56Fe of cumulate samples presented here extend to MORB-like values. Iron isotope values from Lesser Antilles basaltic lavas from Foden et al. (2018) range from δ56Fe 0.09 to -0.002‰. The Statia cumulate Fe isotope values presented here, all have heavier values between δ56Fe 0.12 to 0.09‰, and in Martinique, cumulates also extend to heavier Fe isotope values (δ56Fe = 0.12‰). These data suggest that the sub-arc melts which enter the crust beneath the LAA may have heavier Fe isotope values, prior to their subsequent journey through the crust. These resolvable, yet complimentary differences between arc cumulates and lavas, demonstrate that an isotopically light source is not a requirement to produce the light Fe isotopic compositions of volcanic arc lavas. Because the plutonic xenoliths represent portions of vertically extensive plumbing systems, there is a significant reservoir of heavy Fe stored in crystal mush zones that has been previously overlooked. This result has significant implications in modelling element cycling at subduction zones, suggesting that magmatic processes occurring in the crust should be investigated prior to interpreting isotopic variations in primitive arc lavas. Any magmas that reach the surface in subduction zones are likely modified to some extent on their ascent through the crust. Therefore, using arc lavas in isolation to investigate the heterogeneity of the mantle source is problematic.
Fe Isotopes of the Plumbing System Beneath Statia
In samples from Statia, there is a clear difference between the δ56Fe values of cumulates, and those of NCGs and lavas. There is also a progressive shift to lighter δ56Fe values with indicators of differentiation (e.g., increasing SiO2, Na2O + K2O, Rb/Sr). These data suggest that primitive melts entering the crust may have heavier (MORB-like) δ56Fe values, and cumulate crystallisation within magmatic plumbing systems progressively drives the melt to lighter δ56Fe values. The plutonic xenoliths from the LAA represent direct samples of the amphibole sponge (Cooper et al., 2016; Cooper et al., 2019). The importance of ‘cryptic’ amphibole fractionation in controlling the geochemical composition of lavas in the LAA was demonstrated by trends of decreasing Dy/Yb with increasing SiO2, due to amphibole removal, preferentially partitioning middle REE over heavy REE (Davidson et al., 2007). The samples analysed in this study display progressively lighter δ56Fe values with decreasing Dy/Yb (Figure 5), suggesting that amphibole crystallisation within the mid-crustal mush zone drives the lighter δ56Fe values of the associated melts. The NCGs, displaying progressively lighter δ56Fe values, represent a variety of crystal rich portions of the upper crustal reservoirs (Cooper et al., 2019). These samples have therefore crystallised from melts that have already undergone prior amphibole fractionation. This geochemical evidence is supported by the petrology of the plutonic xenoliths, with amphibole rich samples (Supplementary File S1) displaying heavy δ56Fe values (and high Dy/Yb). In addition, two cumulates with amphibole-free assemblages fall outside of the main geochemical trend (Figure 5). Amphibole fractionation and removal of heavy δ56Fe also occurs beneath the Moho (Turner et al., 2018), but our samples confirm that this process is also prevalent at crustal depths. In addition to amphibole, it is likely that the other phases present in the plutonic xenoliths (e.g., olivine, plagioclase, clinopyroxene, magnetite) also play a role in generating the Fe isotope trends observed in our data. In LAA plutonic xenoliths, textural evidence reveals that magnetite always crystallises after prior crystallisation of olivine and clinopyroxene. Therefore, the effect of magnetite fractionation, which sequesters heavy Fe, is limited by the co-crystallisation of olivine and clinopyroxene, which preferentially incorporate light Fe (Sossi et al., 2012; Foden et al., 2018).
Li et al. (2020) suggested that garnet may control Fe isotope fractionation within deep crustal hot zones. However, geochemical trends in LAA lavas (e.g. Dy/Yb) suggest that no garnet fractionation occurs within the lower crust. Consequently, it is feasible that amphibole crystallisation could exert a first order control on the Fe isotope systematics of the arc crust. Ye et al. (2020) derived equilibrium fractionation factors for a range of different equilibrium mineral pairs and demonstrated that minerals in which Fe has lower coordination numbers and higher oxidation states have shorter Fe–O bonds and preferentially incorporate isotopically heavy Fe (Schauble, 2004; Young et al., 2015). This is relevant to amphibole within volcanic arcs, and because Fe can be accommodated in tetrahedral and small octahedral sites in amphibole (Leake et al., 1997) with variable oxidation states (Della Ventura et al., 2018), it serves as a potential candidate to sequester isotopically heavy Fe, thus driving the evolved liquid towards progressively lighter Fe isotopic compositions. It should be noted that amphibole-melt Fe isotope fractionation factors are known to be strongly influenced by the redox state of the system (Schoenberg et al., 2009). Consequently, without detailed understanding of the amphibole Fe3+/∑Fe ratio in these samples it is not possible to quantitatively derive the fractionation factor for amphibole to reconcile our results, and this remains a target for future study.
By comparing Statia cumulate and NCG assemblages to Lesser Antilles experimental run products, we can reconstruct the conditions which may promote Fe isotope fractionation oceanic arc crust. Melt inclusions from Statia cumulates suggest the plumbing system is water rich with H2O up to 9 wt% (Cooper et al., 2019). Volatile saturation pressures (Cooper et al., 2019) coupled to experimental comparisons (Martel et al., 1999; Pichavant et al., 2002; Melekhova et al., 2017) in equilibrium to the plutonic xenolith assemblages reported here, suggest these samples crystallised in the mid-crust (4 kbar and ≤ 15 km depth). The melt compositions from Martinique basaltic andesite (Pichavant et al., 2002) and andesite experiments (Martel et al., 1999) closely match the melt inclusion chemistry from Statia hornblende gabbro cumulates and gabbronorite NCG’s (Cooper et al., 2019). These experiments were run at NNO-NNO+1 and have melt compositions from ∼50 to 70 wt% SiO2. We therefore suggest that Fe isotope fractionation can occur in the mid crust, under volatile-rich and moderately oxidising redox conditions in the compositional window between basaltic andesites and dacites.
Fe Isotopes of the Plumbing System Beneath Martinique
While the plutonic xenoliths from Martinique also have heavy Fe isotope values (δ56Fe up to 0.12‰), they display a larger range in δ56Fe values than comparable samples from Statia, indicating that the mush zone is more isotopically diverse. Martinique cumulates extend to lighter δ56Fe values (-0.05‰) than Statia cumulates. These lighter Fe isotope values may be a consequence of a higher proportion of olivine within the cumulate mineral assemblages (Supplementary Figure S1), which preferentially incorporates light Fe (Sossi et al., 2012; Foden et al., 2018). A greater diversity in trace elements and radiogenic isotope compositions is also observed in Martinique. Compared with the island of Statia, Martinique has a long volcanic history (0–25 Ma) with greater volcanic production rates (Wadge, 1984). Therefore, due to the volume of melts that have passed through the system, it is likely that the sub-volcanic plumbing system is both volumetrically larger and more chemically diverse than the plumbing system feeding the Quill beneath Statia. Consequently, the plumbing system beneath Martinique is considered somewhat more complex than that below Statia. Martinique cumulate samples provide evidence for open system behaviour within the mid-crustal mush zones (Cooper et al., 2016). In particular, textural and geochemical signatures of late-stage interstitial amphibole (Supplementary File S1) reveal that they crystallised after hydrous percolating melts react with clinopyroxene (Cooper et al., 2016). These percolating melts, which pass through the crystal mush framework, react with the cumulus assemblage, and may therefore overprint the original δ56Fe signature. In contrast to the plutonic xenoliths, amphibole is rarely present within Martinique lavas, suggesting that the depth of shallow pre-eruptive storage of melts is outside the amphibole stability field. This is one process that may explain the trends between δ56Fe values and Dy/Yb ratio. In this scenario, δ56Fe values decrease with decreasing Dy/Yb in cumulates, due to the progressive fractionation of amphibole, but increase in NCGs due to amphibole breakdown at shallow crustal levels, creating an inflection in δ56Fe values versus Dy/Yb within the resultant lithologies (Figure 5). Therefore, in systems where trans-crustal plumbing systems are large and complex, with open system behaviour, then fractionation patterns may represent complex dissolution and reprecipitation processes occurring as magmas ascend through the crust.
It is possible that the Fe isotope fractionation in oceanic arc crust proposed here is unique to the conditions in the LAA. Schoenberg et al. (2009) suggested that each magmatic system should be investigated independently due to the complexity of Fe isotope systematics. This is highlighted in the contrasting Fe isotope variations in the magmatic plumbing systems of Martinique and Statia. Nonetheless, MORB-like Fe isotope values are found in cumulates that crystallised early in the differentiation of primitive melts at both volcanic centres. Foden et al. (2018) found a relationship between the Fe isotope values of mafic to intermediate global arc lavas with the slab thermal parameter [ϕ = slab age (yr) x vertical descent velocity (km/yr)] of each arc. This parameter is used as a proxy for the thermal structure of the downgoing slab (Syracuse and Abers, 2006; van Keken et al., 2011). The proportion of water in the downgoing slab that is transported to a given depth is also a function of this parameter (van Keken et al., 2011). The Lesser Antilles has a ϕ at the lower end of the global arc range, and the data presented in Foden et al. (2018) suggests the sub-arc mantle may have heavier Fe isotope values as a result. Therefore, we suggest that plutonic xenoliths from an oceanic arc with a high ϕ should be targeted to test if mantle derived melts entering the crust can also have MORB-like Fe isotope values in these contrasting subduction systems.
Conclusion
In this study we present the first Fe isotope data from volcanic arc plutonic xenoliths to reveal a previously hidden magmatic control on the Fe isotope systematic of arc lavas occurring in the crust which would not be accessible from studying the volcanic products alone. Iron isotopes are decoupled from radiogenic isotopes and are therefore not controlled by sediment assimilation in the crust, which is a common process in the LAA. We suggest that cumulate fractionation, and dominantly amphibole removal, in mid crustal plumbing systems drives arc lavas to progressively lighter Fe isotope compositions. Therefore, an isotopically light source is not a prerequisite to produce the light Fe isotopic compositions of volcanic arc lavas A similar conclusion was drawn in a continental arc system with respect to Cu sequestration in cumulate hosted sulphides, as an explanation for the decoupling of Cu contents of bulk continental crust and arc lavas (Chen et al., 2020). In light of these conclusions and the decoupling of isotopic systems–geochemical, radiogenic and stable isotopes need to be considered independently and reevaluated using the plutonic record alongside lavas to understand element cycling in subduction zones.
Geochemical and Fe isotope variations suggest that amphibole, in particular, is the major control in driving the Fe isotope composition of lavas in the Lesser Antilles. This result adds to the growing evidence that the ‘amphibole sponge’ has a large control on final erupted products within oceanic arcs. The ubiquitous presence of amphibole in the plumbing systems of the Lesser Antilles is likely a consequence of high water contents along the length of the arc (Cooper et al., 2020), which increases the stability of amphibole within the crust. Therefore, more studies on contrasting oceanic arcs (e.g., dryer, thicker crust, high ϕ) are needed in order to confirm if this process can account for the light Fe isotope values of arc lavas globally.
This whole-rock study on plutonic and volcanic samples provides a good starting point for future work. In order to better constrain the drivers of stable isotope fractionation in volcanic and magmatic systems, further crystal-scale isotope measurements and experimental studies are needed to understand isotopic fractionation between individual minerals and melt.
Data Availability Statement
The original contributions presented in the study are included in the article/Supplementary Material, further inquiries can be directed to the corresponding author.
Author Contributions
GC and EI both conceived the idea of the study carried out the analyses and equally contributed to writing the manuscript.
Funding
GC was funded by NERC grants (Nos. NE/K004328/1 and NE/K010824/1) during the early stages of this study and is currently funded by a NERC standard grant (NE/T000317/1) at the University of Cardiff. EI is currently supported as a research fellow on the ERC advanced grant (SHRED: 833632) awarded to Catherine Chauvel (IPGP) and previously as a PhD student at Durham was supported by a departmental studentship originally awarded to Helen Williams (Cambridge).
Conflict of Interest
The authors declare that the research was conducted in the absence of any commercial or financial relationships that could be construed as a potential conflict of interest.
Publisher’s Note
All claims expressed in this article are solely those of the authors and do not necessarily represent those of their affiliated organizations, or those of the publisher, the editors and the reviewers. Any product that may be evaluated in this article, or claim that may be made by its manufacturer, is not guaranteed or endorsed by the publisher.
Acknowledgments
Geoff Nowell is thanked for performing the radiogenic isotope measurements and maintaining the instruments at Durham Geochemistry Centre. Elena Melekhova and Jon Blundy are thanked for the sampling of Martinique plutonic xenoliths and continued discussions on the workings of volcanic arcs. Members and project partners of the NERC VoiLA consortium and PIGBAG are thanked for fruitful discussions throughout the course of this study. Catherine Chauvel is thanked for her input during an early iteration of this manuscript. Bill Leeman is thanked for comments and editorial handling, and Tomoaki Morishita and Marco Brenna are thanked for very useful reviews which greatly improved the manuscript.
Supplementary Material
The Supplementary Material for this article can be found online at: https://www.frontiersin.org/articles/10.3389/feart.2021.795858/full#supplementary-material
References
Aeolus Lee, C.-T., Leeman, W. P., Canil, D., and Li, Z.-X. A. (2005). Similar V/Sc Systematics in MORB and Arc Basalts: Implications for the Oxygen Fugacities of Their Mantle Source Regions. J. Petrol. 46 (11), 2313–2336. doi:10.1093/petrology/egi056
Annen, C., Blundy, J. D., and Sparks, R. S. J. (2006). The Genesis of Intermediate and Silicic Magmas in Deep Crustal Hot Zones. J. Petrol. 47 (3), 505–539. doi:10.1093/petrology/egi084
Bezard, R., Davidson, J. P., Turner, S., Macpherson, C. G., Lindsay, J. M., and Boyce, A. J. (2014). Assimilation of Sediments Embedded in the Oceanic Arc Crust: Myth or Reality? Earth Planet. Sci. Lett. 395, 51–60. doi:10.1016/j.epsl.2014.03.038
Bezard, R., Turner, S., Davidson, J. P., Macpherson, C. G., and Lindsay, J. M. (2015). Seeing through the Effects of Crustal Assimilation to Assess the Source Composition beneath the Southern Lesser Antilles Arc. J. Petrol. 56 (4), 815–844. doi:10.1093/petrology/egv018
Braszus, B., Goes, S., Allen, R., Rietbrock, A., Collier, J., Harmon Henstock, N. T., et al. (2021). Subduction History of the Caribbean from Upper-Mantle Seismic Imaging and Plate Reconstruction. Nat. Commun. 12 (1), 4211–4214. doi:10.1038/s41467-021-24413-0
Brown, J. R., Cooper, G. F., Nowell, G. M., Macpherson, C. G., Neill, I., and Prytulak, J. (2021). Isotopic Compositions of Plagioclase from Plutonic Xenoliths Reveal Crustal Assimilation below Martinique, Lesser Antilles Arc. Front. Earth Sci. 9, 682583. doi:10.3389/feart.2021.682583
Carpentier, M., Chauvel, C., and Mattielli, N. (2008). Pb–Nd Isotopic Constraints on Sedimentary Input into the Lesser Antilles Arc System. Earth Planet. Sci. Lett. 272 (1-2), 199–211. doi:10.1016/j.epsl.2008.04.036
Chen, K., Tang, M., Lee, C.-T. A., Wang, Z., Zou, Z., Hu, Z., et al. (2020). Sulfide-bearing Cumulates in Deep continental Arcs: The Missing Copper Reservoir. Earth Planet. Sci. Lett. 531, 115971. doi:10.1016/j.epsl.2019.115971
Chen, Y., Niu, Y., Duan, M., Gong, H., and Guo, P. (2021). Fractional Crystallization Causes the Iron Isotope Contrast between Mid-ocean ridge Basalts and Abyssal Peridotites. Commun. Earth Environ. 2 (1), 1–9. doi:10.1038/s43247-021-00135-5
Cooper, G. F., Blundy, J. D., Macpherson, C. G., Humphreys, M. C. S., and Davidson, J. P. (2019). Evidence from Plutonic Xenoliths for Magma Differentiation, Mixing and Storage in a Volatile-Rich crystal Mush beneath St. Eustatius, Lesser Antilles. Contrib. Mineral. Petrol. 174 (5), 39. doi:10.1007/s00410-019-1576-4
Cooper, G. F., Davidson, J. P., and Blundy, J. D. (2016). Plutonic Xenoliths from Martinique, Lesser Antilles: Evidence for Open System Processes and Reactive Melt Flow in Island Arc Crust. Contrib. Mineral. Petrol. 171 (10), 87. doi:10.1007/s00410-016-1299-8
Cooper, G. F., Macpherson, C. G., Blundy, J. D., Maunder, B., Allen, R. W., Goes, S., et al. (2020). Variable Water Input Controls Evolution of the Lesser Antilles Volcanic Arc. Nature 582, 525–529. doi:10.1038/s41586-020-2407-5
Dauphas, N., Janney, P. E., Mendybaev, R. A., Wadhwa, M., Richter, F. M., Davis, A. M., et al. (2004). Chromatographic Separation and Multicollection-ICPMS Analysis of Iron. Investigating Mass-dependent and -Independent Isotope Effects. Anal. Chem. 76 (19), 5855–5863. doi:10.1021/ac0497095
Dauphas, N., John, S. G., and Rouxel, O. (2017). Iron Isotope Systematics. Rev. Mineral. Geochem. 82, 415–510. doi:10.2138/rmg.2017.82.11
Davidson, J. P., and Wilson, M. (2011). Differentiation and Source Processes at Mt Pelée and the Quill; Active Volcanoes in the Lesser Antilles Arc. J. Petrol. 52 (7–8), 1493–1531. doi:10.1093/petrology/egq095
Davidson, J. P. (1987). Crustal Contamination versus Subduction Zone Enrichment: Examples from the Lesser Antilles and Implications for Mantle Source Compositions of Island Arc Volcanic Rocks. Geochim. Cosmochim. Acta 51, 2185–2198. doi:10.1016/0016-7037(87)90268-7
Davidson, J. P. (1986). Isotopic and Trace Element Constraints on the Petrogenesis of Subduction-Related Lavas from Martinique, Lesser Antilles. J. Geophys. Res. 91 (B6), 5943. doi:10.1029/jb091ib06p05943
Davidson, J., Turner, S., Handley, H., Macpherson, C., and Dosseto, A. (2007). Amphibole "sponge" in Arc Crust? Geol 35 (9), 787–790. doi:10.1130/g23637a.1
Debret, B., Bouilhol, P., Pons, M. L., and Williams, H. (2018). Carbonate Transfer during the Onset of Slab Devolatilization: New Insights from Fe and Zn Stable Isotopes. J. Petrol. 59, 1145–1166. doi:10.1093/petrology/egy057
Debret, B., Millet, M.-A., Pons, M.-L., Bouilhol, P., Inglis, E., and Williams, H. (2016). Isotopic Evidence for Iron Mobility during Subduction. Geology 44, 215–218. doi:10.1130/g37565.1
Debret, B., Reekie, C. D. J., Mattielli, N., Beunon, H., Ménez, B., Savov, I., et al. (2020). Redox Transfer at Subduction Zones: Insights from Fe Isotopes in the Mariana Forearc. Geochem. Persp. Let. 12, 46–51. doi:10.7185/geochemlet.2003
Della Ventura, G., Mihailova, B., Susta, U., Cestelli Guidi, M., Marcelli, A., Schlüter, J., et al. (2018). The Dynamics of Fe Oxidation in Riebeckite: A Model for Amphiboles. Am. Mineral. 103 (7), 1103–1111. doi:10.2138/am-2018-6382
DuFrane, S. A., Turner, S., Dosseto, A., and Van Soest, M. (2009). Reappraisal of Fluid and Sediment Contributions to Lesser Antilles Magmas. Chem. Geol. 265 (3-4), 272–278. doi:10.1016/j.chemgeo.2009.03.030
El Korh, A., Luais, B., Deloule, E., and Cividini, D. (2017). Iron isotope fractionation in subduction-related high-pressure metabasites (Ile de Groix, France). Contrib. Mineral. Petrol. 172, 1–19. doi:10.1007/s00410-017-1357-x
Foden, J., Sossi, P. A., and Nebel, O. (2018). Controls on the Iron Isotopic Composition of Global Arc Magmas. Earth Planet. Sci. Lett. 494, 190–201. doi:10.1016/j.epsl.2018.04.039
Germa, A., Quidelleur, X., Labanieh, S., Chauvel, C., and Lahitte, P. (2011). The Volcanic Evolution of Martinique Island: Insights from K–Ar Dating into the Lesser Antilles Arc Migration since the Oligocene. J. Volcanol. Geothermal Res. 208 (3-4), 122–135. doi:10.1016/j.jvolgeores.2011.09.007
Gerrits, A. R., Inglis, E. C., Dragovic, B., Starr, P. G., Baxter, E. F., and Burton, K. W. (2019). Release of Oxidizing Fluids in Subduction Zones Recorded by Iron Isotope Zonation in Garnet. Nat. Geosci. 12, 1029–1033. doi:10.1038/s41561-019-0471-y
Hu, Y., Teng, F.-Z., and Chauvel, C. (2021). Potassium Isotopic Evidence for Sedimentary Input to the Mantle Source of Lesser Antilles Lavas. Geochim. Cosmochim. Acta 295, 98–111. doi:10.1016/j.gca.2020.12.013
Inglis, E. C., Debret, B., Burton, K. W., Millet, M.-A., Pons, M.-L., Dale, C. W., et al. (2017). The Behavior of Iron and Zinc Stable Isotopes Accompanying the Subduction of Mafic Oceanic Crust: A Case Study from Western Alpine Ophiolites. Geochem. Geophys. Geosyst. 18, 2562–2579. doi:10.1002/2016gc006735
Jochum, K. P., Nohl, U., Herwig, K., Lammel, E., Stoll, B., and Hofmann, A. W. (2005). GeoReM: A New Geochemical Database for Reference Materials and Isotopic Standards. Geostand Geoanalyt. Res. 29, 333–338. doi:10.1111/j.1751-908x.2005.tb00904.x
Johnson, C. M., Beard, B. L., and Albarede, F. (2004). “Overview and General Concepts,” in Geochemistry of Non- Traditional Stable Isotopes: Reviews in Mineralogy and Geochemistry. Editors C. M. Johnson, B. L. Beard, and F. Albarede 325, 1–24
Kelley, K. A., and Cottrell, E. (2009). Water and the Oxidation State of Subduction Zone Magmas. Science 325, 605–607. doi:10.1126/science.1174156
Labanieh, S., Chauvel, C., Germa, A., Quidelleur, X., and Lewin, E. (2010). Isotopic Hyperbolas Constrain Sources and Processes under the Lesser Antilles Arc. Earth Planet. Sci. Lett. 298 (1–2), 35–46. doi:10.1016/j.epsl.2010.07.018
Labanieh, S., Chauvel, C., Germa, A., and Quidelleur, X. (2012). Martinique: A clear Case for Sediment Melting and Slab Dehydration as a Function of Distance to the Trench. J. Petrol. 53 (12), 2441–2464. doi:10.1093/petrology/egs055
Leake, B. E., Woolley, A. R., Arps, C. E., Birch, W. D., Gilbert, M. C., Grice, J. D., et al. (1997). Nomenclature of Amphiboles; Report of the Subcommittee on Amphiboles of the International Mineralogical Association, Commission on New Minerals and Mineral Names. Can. Mineral. 35 (1), 219–246. doi:10.1127/ejm/9/3/0623
Li, Q.-W., Zhao, J.-H., Wang, Q., Zhang, Z.-F., An, Y.-J., and He, Y.-T. (2020). Iron Isotope Fractionation in Hydrous Basaltic Magmas in Deep Crustal Hot Zones. Geochim. Cosmochim. Acta 279, 29–44. doi:10.1016/j.gca.2020.03.032
Macdonald, R., Hawkesworth, C. J., and Heath, E. (2000). The Lesser Antilles Volcanic Chain: a Study in Arc Magmatism. Earth Sci. Rev. 49, 1–76. doi:10.1016/s0012-8252(99)00069-0
Martel, C., Pichavant, M., Holtz, F., Scaillet, B., Bourdier, J.-L., and Traineau, H. (1999). Effects offO2and H2O on Andesite Phase Relations between 2 and 4 Kbar. J. Geophys. Res. 104, 29453–29470. doi:10.1029/1999jb900191
Melekhova, E., Blundy, J., Martin, R., Arculus, R., and Pichavant, M. (2017). Petrological and Experimental Evidence for Differentiation of Water-Rich Magmas beneath St. Kitts, Lesser Antilles. Contrib. Mineral. Petrol. 172, 98. doi:10.1007/s00410-017-1416-3
Melekhova, E., Schlaphorst, D., Blundy, J., Kendall, J.-M., Connolly, C., McCarthy, A., et al. (2019). Lateral Variation in Crustal Structure along the Lesser Antilles Arc from Petrology of Crustal Xenoliths and Seismic Receiver Functions. Earth Planet. Sci. Lett. 516, 12–24. doi:10.1016/j.epsl.2019.03.030
Millet, M.-A., Baker, J. A., and Payne, C. E. (2012). Ultra-precise Stable Fe Isotope Measurements by High Resolution Multiple-Collector Inductively Coupled Plasma Mass Spectrometry with a 57Fe-58Fe Double Spike. Chem. Geol. 304-305, 18–25. doi:10.1016/j.chemgeo.2012.01.021
Müller, R. D., Zahirovic, S., Williams, S. E., Cannon, J., Seton, M., Bower, D. J., et al. (2019). A Global Plate Model Including Lithospheric Deformation along Major Rifts and Orogens since the Triassic. Tectonics 38, 1884–1907. doi:10.1029/2018TC005462
Nebel, O., Sossi, P. A., Bénard, A., Wille, M., Vroon, P. Z., and Arculus, R. J. (2015). Redox-variability and Controls in Subduction Zones from an Iron-Isotope Perspective. Earth Planet. Sci. Lett. 432, 142–151. doi:10.1016/j.epsl.2015.09.036
Nebel, O., Sossi, P. A., Foden, J., Bénard, A., Brandl, P. A., Stammeier, J. A., et al. (2018). Iron Isotope Variability in Ocean Floor Lavas and Mantle Sources in the Lau Back-Arc basin. Geochim. Cosmochim. Acta 241, 150–163. doi:10.1016/j.gca.2018.08.046
Pichavant, M., Martel, C., Bourdier, J-L., and Scaillet, B. (2002). Physical Conditions, Structure, and Dynamics of a Zoned Magma Chamber: Mount Pelée (Martinique, Lesser Antilles Arc). J. Geophys. Res. 107 (B5), 2093. doi:10.1029/2001jb000315
Roobol, M. J., and Smith, A. L. (2004). Volcanology of Saba and St. Eustatius, Northern Lesser Antilles. Netherlands: Koninklijke nederlandse Akademie van wetenschappen.
Schauble, E. A. (2004). Applying Stable Isotope Fractionation Theory to New Systems. Rev. Mineral. Geochem. 55 (1), 65–111. doi:10.2138/gsrmg.55.1.65
Schoenberg, R., Marks, M. A., Schuessler, J. A., von Blanckenburg, F., and Markl, G. (2009). Fe Isotope Systematics of Coexisting Amphibole and Pyroxene in the Alkaline Igneous Rock Suite of the Ilímaussaq Complex, South Greenland. Chem. Geol. 258 (1-2), 65–77. doi:10.1016/j.chemgeo.2008.06.023
Smith, D. J. (2014). Clinopyroxene Precursors to Amphibole Sponge in Arc Crust. Nat. Commun. 5 (1), 4329–4336. doi:10.1038/ncomms5329
Solano, J. M. S., Jackson, M. D., Sparks, R. S. J., Blundy, J. D., and Annen, C. (2012). Melt Segregation in Deep Crustal Hot Zones: a Mechanism for Chemical Differentiation, Crustal Assimilation and the Formation of Evolved Magmas. J. Petrol. 53 (10), 1999–2026. doi:10.1093/petrology/egs041
Sossi, P. A., Foden, J. D., and Halverson, G. P. (2012). Redox-controlled Iron Isotope Fractionation during Magmatic Differentiation: an Example from the Red Hill Intrusion, S. Tasmania. Contrib. Mineral. Petrol. 164 (5), 757–772. doi:10.1007/s00410-012-0769-x
Syracuse, E. M., and Abers, G. A. (2006). Global Compilation of Variations in Slab Depth beneath Arc Volcanoes and Implications. Geochem. Geophys. Geosys. 7 (5), Q05017. doi:10.1029/2005gc001045
Teng, F.-Z., Dauphas, N., Huang, S., and Marty, B. (2013). Iron Isotopic Systematics of Oceanic Basalts. Geochim. Cosmochim. Acta 107, 12–26. doi:10.1016/j.gca.2012.12.027
Turner, S., Williams, H., Piazolo, S., Blichert-Toft, J., Gerdes, M., Adam, J., et al. (2018). Sub-arc Xenolith Fe-Li-Pb Isotopes and Textures Tell Tales of Their Journey through the Mantle Wedge and Crust. Geology 46, 947–950. doi:10.1130/g45359.1
van Keken, P. E., Hacker, B. R., Syracuse, E. M., and Abers, G. A. (2011). Subduction Factory: 4. Depth-dependent Flux of H2O from Subducting Slabs Worldwide. J. Geophys. Res. Solid Earth 116 (B1). doi:10.1029/2010jb007922
Van Soest, M. C., Hilton, D. R., Macpherson, C. G., and Mattey, D. P. (2002). Resolving Sediment Subduction and Crustal Contamination in the Lesser Antilles Island Arc: A Combined He-O-Sr Isotope Approach. J. Petrol. 43 (1), 143–170. doi:10.1093/petrology/43.1.143
Wadge, G. (1984). Comparison of Volcanic Production Rates and Subduction Rates in the Lesser Antilles and Central America. Geol. 12, 555–558. doi:10.1130/0091-7613(1984)12<555:covpra>2.0.co;2
Weyer, S., and Schwieters, J. B. (2003). High Precision Fe Isotope Measurements with High Mass Resolution MC-ICPMS. Int. J. Mass Spectrom. 226 (3), 355–368. doi:10.1016/s1387-3806(03)00078-2
White, W. M., and Dupré, B. (1986). Sediment Subduction and Magma Genesis in the Lesser Antilles: Isotopic and Trace Element Constraints. J. Geophys. Res. 91 (B6), 5927–5941. doi:10.1029/jb091ib06p05927
Ye, H., Wu, C., Brzozowski, M. J., Yang, T., Zha, X., Zhao, S., et al. (2020). Calibrating Equilibrium Fe Isotope Fractionation Factors between Magnetite, Garnet, Amphibole, and Biotite. Geochim. Cosmochim. Acta 271, 78–95. doi:10.1016/j.gca.2019.12.014
Keywords: plutonic xenoliths, Fe isotopes, Lesser Antilles arc, Martinique, Statia, subduction zone, oceanic arc, crust
Citation: Cooper GF and Inglis EC (2022) A Crustal Control on the Fe Isotope Systematics of Volcanic Arcs Revealed in Plutonic Xenoliths From the Lesser Antilles. Front. Earth Sci. 9:795858. doi: 10.3389/feart.2021.795858
Received: 15 October 2021; Accepted: 09 December 2021;
Published: 05 January 2022.
Edited by:
William Prescott Leeman, Rice University, United StatesReviewed by:
Marco Brenna, University of Otago, New ZealandTomoaki Morishita, Kanazawa University, Japan
Copyright © 2022 Cooper and Inglis. This is an open-access article distributed under the terms of the Creative Commons Attribution License (CC BY). The use, distribution or reproduction in other forums is permitted, provided the original author(s) and the copyright owner(s) are credited and that the original publication in this journal is cited, in accordance with accepted academic practice. No use, distribution or reproduction is permitted which does not comply with these terms.
*Correspondence: G. F. Cooper, CooperG3@cardiff.ac.uk