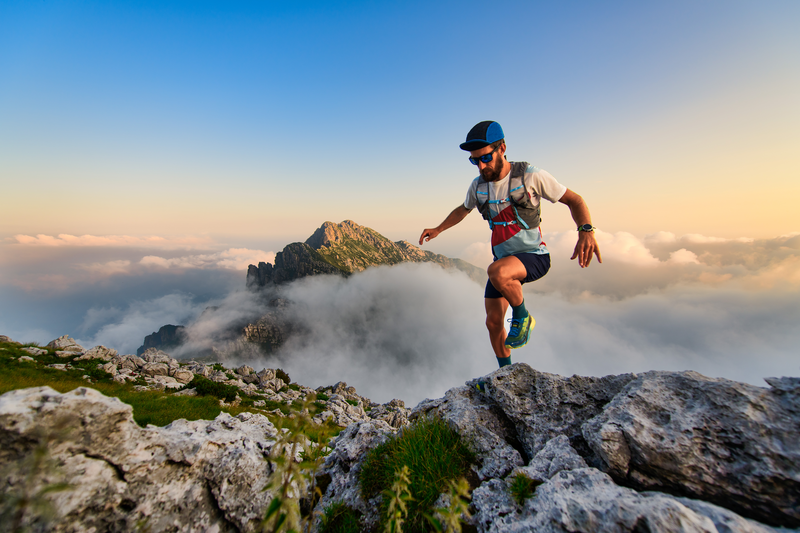
94% of researchers rate our articles as excellent or good
Learn more about the work of our research integrity team to safeguard the quality of each article we publish.
Find out more
ORIGINAL RESEARCH article
Front. Earth Sci. , 24 December 2021
Sec. Paleontology
Volume 9 - 2021 | https://doi.org/10.3389/feart.2021.789781
This article is part of the Research Topic Human-Animal Interactions in Prehistoric China View all 11 articles
The reconstruction of environmental and climatic changes in the Pleistocene is an essential contribution to our understanding of human evolutionary and behavioral adaptations. Well preserved fluvio-lacustrine sediments at Nihewan basin have yielded a rich record of Early Pleistocene Paleolithic sites and mammalian fossils which provide a unique opportunity for exploring hominin behavior and paleoecology in North China. Taxonomic studies of mammalian fossils have provided important clues to the general environmental setting and landscapes of Early Pleistocene humans in the fluvio-lacustrine basin of Nihewan, but little is known about their isotopic signatures. In this paper, mammal teeth species at the Madigou archaeological site (ca. 1.2 Ma) were selected for bulk and sequential enamel stable isotope (C, O) analysis. Results show a variety of ecological environments, including grassland and sparse forest landscapes, and distinct patterns across taxa. C3-C4 mixed vegetation predominated, but C4 vegetation was also relevant at times. Madigou early humans likely experienced cold/warm or dry/wet fluctuations in this northern China basin. We hypothesize that the environmental fluctuations and diversified landscapes may have driven flexibility in various aspects of early human technological behaviors, and allowed hominins to face the environmental challenges of northern latitudes after the initial expansion from Africa into East Asia at the onset of the Middle Pleistocene Climate Transition.
Understanding the impact of paleoenvironmental variability on hominin behavioral adaptations is a key area of research in human evolution (deMenocal, 1995; Ambrose, 2001; Behrensmeyer, 2006), and is of crucial relevance for understanding the initial dispersal of humans from Africa into Eurasia (Gabunia et al., 2000; Bar-Yosef and Belfer-Cohen, 2001; Van der Made, 2011) and hominin behavioral adaptations during the Middle Pleistocene Climate Transition (MPT) at ∼ 1.25–0.7 Ma, which is marked by a progressive increase in the amplitude of climate oscillations (Ruddiman et al., 1986; Mudelsee and Schulz, 1997; Clark et al., 2006; Wang et al., 2017). It has been hypothesized that the MPT triggered substantial hominin dispersals from Africa to Eurasia (Larick and Ciochon, 1996; Wu and Liu, 2001; Deng et al., 2007; Abbate and Sagri, 2012), and it may be linked to a more sustained settlement by Homo erectus in northern latitudes of East Asia. A more continuous occupation of northern latitudes would be aided by a diversity of adaptive behaviors, following patterns observed elsewhere (deMenocal, 2011; Grove, 2012; Potts, 2012, 2013; Potts and Faith, 2015), in which human biological evolution and lithic technological innovations were coupled with a high frequency of climatic fluctuation cycles.
The Nihewan Basin (Figure 1) in North China is well known for its abundance of archaeological sites through the Lower and Upper Pleistocene (Schick et al., 1991; Zhu et al., 2001, 2004; Deng et al., 2006, 2007; Ao et al., 2010, 2013; Zuo et al., 2011). Nihewan paleoenvironments have been reconstructed through the analysis of sedimentary features and mammalian enamel stable isotopes (Deng et al., 2001; An et al., 2005; Ding et al., 2005; Pei et al., 2009), pollen (Li et al., 1996; Wu et al., 2007; Pei et al., 2009), magnetic susceptibility (Deng et al., 2007; Pei et al., 2009, 2019), iron oxides (Pei et al., 2009), soluble salts (Li et al., 2010), and site formation processes (Jia et al., 2019). Recent archaeological studies (Pei et al., 2017, 2019; Yang et al., 2017, 2020, 2021) have discussed the links between climatic variability and human adaptations, suggesting that changes in lithic technological strategies occurred at the beginning of the MPT. Such changes would be evidenced by the flexibility in raw material procurement, diversification of flaking techniques, a refinement of retouching techniques, and an increase of tool types. However, the environmental context in which such new technological patterns emerged has not yet been properly ascertained.
FIGURE 1. (A–C) Location of the Nihewan Basin in China and the distribution of key Pleistocene sites in the Cenjiawan Platform. (D) Trenches at the MDG site complex, viewed from the southwest. Abbreviations: LU = lower unit. TBSU = thick brown sand unit. UU = upper unit (Pei et al., 2019).
Stable isotope (C, O) analysis of tooth enamel provides direct evidence of the ecology and habitat of fossil mammals (Quade et al., 1992; Cerling et al., 1997; Cerling and Harris, 1999; Van der Merwe, 2013; Rivals et al., 2018; Uno et al., 2018), but is yet to be applied systematically to the Nihewan Pleistocene sequence. Here we contribute to this effort by presenting the first analysis of isotope values to mammalian teeth from the Madigou site (MDG). Our study includes bulk sampling from the whole teeth enamel and sequential sampling of several specimens, which were used to reconstruct paleolandscapes and seasonal variability in Early Pleistocene Nihewan, and to contextualize both with dynamics observed in the use of stone tools by early humans at the site.
According to different pathways of photosynthesis, terrestrial plants are generally divided into three categories, C3 (Calvin), C4 (hatch slack) and CAM (Crassulacean acid metabolic acid), which cause the differences of carbon isotopic fractionation during the processes of carbon fixation. δ13C values of C3 plants, including trees, shrubs and cold-tolerant herbs (Deng et al., 2001), range from −34‰ to −22‰, while those of C4 plants, typical of drier and warmer environments (Raven et al., 1999), range from −17‰ to −9‰ (O'Leary, 1988; Farquhar et al., 1989; Cerling et al., 1997). Other factors such as rainfall, altitude, light intensity, atmospheric carbon dioxide concentration and the canopy effect also affect the δ13C values of plants (Farquhar et al., 1989). δ13C values of C3 plants become more negative with the increase of the rainfall, altitude and latitude (Kohn, 2010). Isotopic fractionation takes place from diets to teeth enamel when plants are eaten by herbivores, and when herbivores are consumed by carnivores. Compared to those in plants, δ13C values of teeth enamel from large herbivores and carnivores increase by ∼14‰ and ∼9‰ respectively (Cerling and Harris, 1999; Tejada-Lara et al., 2018). Following earlier work (Cerling et al., 1997; Wang et al., 2008; Biasatti et al., 2010; Uno et al., 2018), the δ13C values in tooth enamel lower than -8‰ are attributed in this study to animals that only eat C3 food, from -8‰ to −2‰ to those with a C3-C4 mixed diet, and higher than −2‰ to those consuming mainly C4 foods.
The oxygen isotope composition in mammalian teeth is mainly determined by that of body water, which derives directly from drinking water (Pederzani and Britton, 2019). Due to evaporation, δ18O values in plant leaves are higher than those in meteoric water. This results in leaf-eating herbivores having higher δ18O values than those drinking meteoric water (Pederzani and Britton, 2019), thus enabling to distinguish browsers from grazers. Additionally, δ18O values vary with the altitude, temperature and latitude, which helps to track animal movement across different ecozones (Pederzani and Britton, 2019).
Two sampling strategies are usually applied to the isotopic analysis of fossil teeth enamel. Bulk sampling of the whole enamel is used to reconstruct the average diet and ecological setting during the period of tooth formation (Feranec and MacFadden, 2000). Sequential sampling of the enamel along the direction of enamel growth may reveal the spatiotemporal dietary and environmental changes throughout the development of the tooth (Balasse, 2002).
The Nihewan Basin (which includes the Yangyuan Basin and Yuxian Basin in Hebei Province, and the Datong Basin in Shanxi Province), is an intermontane basin between the Inner Mongolian Plateau and North China (Deng et al., 2019; Pei et al., 2019) (Figure 1A). It is well known for its extensive late Cenozoic fluvio-lacustrine sequence (the Nihewan Beds), reliably-constrained geochronology, and abundant archaeological sites (Schick et al., 1991; Zhu et al., 2001, 2004; Deng et al., 2006, 2007; Ao et al., 2010, 2013; Zuo et al., 2011). (Figures 1A–C). The Nihewan Beds contain fluvio-lacustrine deposits from the Late Pliocene to the late Middle/Upper Pleistocene (Zhao et al., 2010; Nian et al., 2013; Deng et al., 2019). These deposits include the Pliocene-Pleistocene boundary (Liu et al., 2012) and the Nihewan faunas (sensu lato) (Teilhard de Chardin and Piveteau, 1930; Zhou et al., 1991; Qiu and Qiu, 1995), and are constrained at the bottom by the Pliocene red clay and overlain by the Late Pleistocene Malan loess (Deng et al., 2019) at the top of the sequence. Current geochronological and archaeological research show that early hominins may have continuously occupied the Nihewan Basin from 1.66 Ma (Zhu et al., 2004) to the Late Pleistocene (Schick et al., 1991; Zhu et al., 2001, 2004; Pei et al., 2019).
Madigou (40°13′07–16″N, 114°39′58″–40′18″E) is located in the northwest margin of the Cenjiawan platform (eastern part of the Nihewan Basin). Paleomagnetism indicates that the MDG stratigraphy comprises the early Brunhes normal chron and the late Matuyama reverse chron, including the Jaramillo normal subchron (Figure 2). The MDG archaeological layers are positioned within the pre-Jaramillo Matuyama chron, with an estimated age of ca. 1.2 Ma, i.e., chronologically within the onset of the MPT. Stratigraphic correlations of seven trenches excavated at MDG indicate that the MDG chronostratigraphic sequence begins with MDG-E2, followed by MDG-E3, MDG-E5 and MDG-E7, and contains the most recent units at MDG-E6 (Figure 1D) (Pei et al., 2019). A total of 1,517 lithic artifacts and over 900 fossil remains, including Equus, Coelodonta antiquitatis, Cervidae, Bovidae, and others, were unearthed from the lower part of the sequence in each trench, especially in MDG-E2 and MDG-E3 (Pei et al., 2019). Predominance of ungulates in the fossil assemblage suggested open grasslands and a sparse steppe (Pei et al., 2019).
FIGURE 2. Lithostratigraphy and magnetic stratigraphy of MDG-E2, MDG-E3 and MDG-E6, and positions of the samples studied (excluding one sample from MDG-E7) (Hilgen et al., 2012; Pei et al., 2019).
Chert dominates among lithic raw materials, followed by siliceous dolomite (Pei et al., 2019). MDG knappers showed a preference for specific rock types, such as siliceous dolomite cobble for bipolar knapping, brecciated chert blocks for freehand hard hammer flaking, and high-quality chert for retouching tools (Pei et al., 2019).
The MDG fossils were spatially associated with stone artifacts, and preliminary zooarchaeological results suggest human action over part of the fossil assemblage (Pei et al., 2019). Thus, the ecological and environmental data retrieved from the isotopic analysis of fossil enamel presented herein also informs on the landscapes occupied by early humans at Nihewan.
Seventy-seven fossil teeth from archaeological layers at MDG were selected for isotopic analysis: 67 from trench MDG-E2, 8 from trench MDG-E3, and one from each MDG-E6, and MDG-E7 (Figure 2). Bulk sampling was made from 71 teeth of Cervidae (Muntiacus sp.), Moschidae, Bovidae, Rhinocerotidae (Coelodonta antiquitatis), Equidae (Equus wangi sp. Nov. and Equus qingyangensis sp. Nov.), Canidae (Canis chihliensis?) and others (Table 1; Supplementary Table S1).
Six additional teeth were serially sampled: 2 of Coelodonta antiquitatis, 3 of Equus wangi sp. Nov., and 1 of Equus qingyangensis sp. Nov. (see details in Supplementary Tables S2–7). No first molars were included, to prevent the breastfeeding effect on isotopic data.
Bioapatite pretreatment was undertaken at the Institute of Vertebrate Paleontology and Paleoanthropology, Chinese Academy of Sciences (IVPP), and followed the protocols described in Lee-Thorp et al. (1989), Bocherens et al. (1994), Koch et al. (1997), and Wright and Schwarcz (1999). Before sampling, any contaminations on the enamel surface were removed with a dental drill. For the 71 teeth selected for bulk sampling, 15–30 mg enamel powder were extracted evenly from different parts of the enamel and grinded to below 200 meshes with agate mortar. Sequential samples of six additional teeth were collected from crown to neck along the enamel growth axis. The average sampling interval was 5 mm, and 15–20 mg of each sample was collected.
To remove the organic matter, about 1.5 ml of 2.5% sodium hypochlorite was added into the 2.0 ml tubes for each sample. After full reaction, samples were centrifuged and washed to neutrality with distilled water. Subsequently, 1.5 ml of 1 M acetic acid was added for 20 h to each sample to remove the secondary carbonate. Samples were subsequently cleaned with distilled water, freeze-dried and ground into powder again.
Isotopic measurements were undertaken in an Isotope Ratio Mass spectrometer (MAT-253) combined with a Gas bench system in the Laboratory for Stable Isotope Geochemistry, Institute of Geology and Geophysics, Chinese Academy of Sciences. The isotopic results were expressed as δ13C and δ18O, relative to the VPDB. The isotopic standards used for isotopic calibration were NBS 18, NBS 19 and GBW04405 (δ13CVPDB = 0.57 ± 0.03‰, δ18O VPDB = −8.49 ± 0.14‰; Certified reference material approved by the State Bureau of Technical Supervision, the People’s Republic of China). The precisions of δ13C and δ18O values are better than 0.15‰ and 0.20‰ respectively. Isotopic data are listed in Supplementary Tables S1–7.
Figure 3 shows large isotopic variations among specimens that suggest different niches. The δ13C values range from −13.0‰ to −2.1‰ and average −7.3 ± 2.7‰ (n = 77), while the δ18O values range from −12.5‰ to −1.1‰ and average −8.8 ± 1.9‰ (n = 77).
The δ13C value of Moschidae (n = 1) is −13.0‰, indicating a closed C3 environment. This sample shows the highest δ18O value (−1.1‰).
Cervidae (n = 6) includes Muntiacus sp. and other unidentifiable Cervidae taxa. Their δ13C values range from−12.9‰ to −10.0‰, with a mean of −12.0 ± 1.1‰. δ18O values range between −11.4‰ and −4.5‰, averaging −6.9 ± 2.7‰ (n = 6). However, given the abnormally low δ18O values of MDG13 (−8.3‰), MDG55 (−7.4‰) and MDG57 (−11.4‰), which might be due to the fact that the individuals come from other regions, the isotope data from those teeth are excluded from the following statistical analysis and discussion. The mean values of δ13C and δ18O in remaining Cervidae (n = 3) are −12.6 ± 0.5‰ and −4.7 ± 0.1‰ respectively, which indicates that MDG Cervidae fed in a pure C3 environment.
Bovidae (n = 5) show δ13C values from −6.6‰ to −2.1‰, averaging −4.2 ± 1.9‰ and δ18O values from −11.5‰ to −7.4‰ (average of −9.4 ± 1.9‰). This suggests that they consumed mixed C3-C4 plants. It is notable that one specimen (MDG61) has the highest δ13C value (−2.1‰) among the entire assemblage, indicating a nearly neat C4 environment.
The δ13C values of Coelodonta antiquitatis (n = 29) differ from those of carnivores and artiodactyls, ranging from −11.7‰ to -5.5‰ (mean = −7.9 ± 1.4‰). This indicates that the habitat of Coelodonta antiquitatis ranged between closed forest and open grassland landscapes. MDG Coelodonta teeth yield the lowest average δ18O value [−10.0 ± 1.2‰, (n = 29)] in the entire assemblage, which could be related to consumption of meteoric water.
The δ13C values of Equus (n = 23) range from −8.7‰ to −3.4‰ (average of −6.0 ± 1.8‰) and their δ18O values range from −9.7‰ to -5.3‰ (mean = −8.3 ± 1.0‰). This indicates their preference for more open environments compared to Coelodonta antiquitatis.
The δ13C value of a sole specimen of Canidae is −12.2‰. Its δ18O value is −5.3‰, higher than those from Coelodonta, Equus, and Bovidae.
The δ13C profiles from sequential samples of Coelodonta antiquitatis, Equus wangi sp. Nov., and Equus qingyangensis sp. Nov., suggest considerable variations of the diet throughout the life history of these specimens. Pure C3 or nearly pure C4 vegetation predominated occasionally, but C3-C4 mixed vegetation dominated. Variations observed in δ13C profiles could indicate an oscillation between dry and wet seasons, while variability in δ18O profiles may indicate periodic or seasonal fluctuations in precipitation (Figure 4; Table 2).
FIGURE 4. Sequential isotopic profiles from MDG teeth (A). MDG10: Coelodonta antiquitatis, Frag; (B). MDG11: Coelodonta antiquitatis, M3; (C). MDG16: Equus wangi sp. Nov., m3; (D). MDG19: Equus wangi sp. Nov., P4; (E). MDG22: Equus wangi sp. Nov., P4; (F). MDG23: Equus qingyangensis sp. Nov., p4).
Considering the fractionation of carbon isotope from diet to enamel bioapatite (with an enrichment of 14‰ in large herbivorous and of 9‰ in carnivores) (Tieszen et al., 1983; Cerling and Harris, 1999; Tejada-Lara et al., 2018), the niches of the MDG fauna can be reconstructed on the basis of isotopic data from bulk samples (Figure 5). We conclude that the fauna accumulated at the MDG site occupied a relatively broad niche, from open grassland to closed forest.
FIGURE 5. Niche reconstruction of the MDG fossil assemblage, based on δ13C diets and δ18Oenamel values.
In terms of δ13Cdiets values, Moschidae and Cervidae have the highest negative δ13Cdiets values, indicative of a closed forest. On the other end, Equus and Bovidae have the most positive δ13Cdiets values, typical of open environments. The large standard deviations in Bovidae (δ13Cdiets: 1.9‰) and Equus (δ13Cdiets: 1.8‰) suggest that they had a more flexible dietary breadth. Conversely, the smaller standard deviations in Coelodonta antiquitatis (δ13Cdiets: 1.4‰) may indicate a more specialized diet.
Regarding δ18Oenamel values, Figure 4 shows that Moschidae (−1.1‰) and Cervidae (−4.7 ± 0.1‰) have more positive average δ18Oenamel values than Equus (−8.3 ± 1.0‰), Bovidae (−9.4 ± 1.9‰) and Coelodonta antiquitatis (−10.0 ± 1.2‰). This indicates a preference in Moschidae and Cervidae for more 18O-enriched foods (such as leaves). Overall, the standard deviation in Coelodonta antiquitatis (δ13Cdiets: 1.4‰, δ18Oenamel: 1.2‰) suggests more limited foraging flexibility, habitat and narrower ecological adaptability than Equus (δ13Cdiets: 1.8‰, δ18Oenamel: 1.0‰) and Bovidae (δ13Cdiets: 1.9‰, δ18Oenamel: 1.9‰).
As shown in Figure 6, the isotopic profiles from Coelodonta antiquitatis and Equus indicate seasonal changes. MDG23 (Equus qingyangensis sp. Nov.) (δ13Cdiets: 1.7‰) and MDG16 (Equus wangi sp. Nov.) (δ13Cdiets: 1.4‰) have the largest variation in the δ13Cdiets standard deviation, which suggests their adaptability to varied landscapes in nearly pure C4, mixed C3-C4 and nearly pure C3 vegetation. In contrast, the low standard deviation in MDG11 (Coelodonta antiquitatis) (δ13Cdiets: 0.3‰) indicates a relatively fixed niche and narrow ecological adaptability for this individual. On the other hand, standard deviations of MDG16 (δ18Oenamel: 1.5‰), MDG19 (Equus wangi sp. Nov.) (δ18Oenamel: 1.1‰), MDG10 (Coelodonta antiquitatis) (δ18Oenamel: 0.9‰) and MDG22 (Equus wangi sp. Nov.) (δ18Oenamel: 0.9‰) are large, which reflects a seasonal variation of regional temperature and precipitation.
FIGURE 6. Reconstructed δ13Cdiets and δ18Oenamel values from sequential samples of MDG teeth. MDG10 and MDG11: Coelodonta antiquitatis. MDG16, 19 and 22: Equus wangi sp. Nov. MDG23: Equus qingyangensis sp. Nov.
It has been proposed that human occupation of the Nihewan Basin during the Early Pleistocene was discontinuous and that the area would only be populated during interstadial periods and in the warm seasons (Dennell, 2003, 2013). While systematic testing is still needed through multiple proxies and across the archaeological sequence (de la Torre et al., 2020), our contribution on the isotopic analysis of the MDG faunal assemblages does not seem to support such hypothesis. Variability of patterns in δ18O and δ13C values (Figure 4) strongly suggests input of mammal carcasses to the site during various seasons. In addition, considering other archaeological evidence in the Nihewan Basin, it has been suggested that Early Pleistocene humans in North China could have adopted flexible technological strategies as a response to environmental fluctuations (Pei et al., 2019).
In the case of MDG, early humans preferentially used preferentially siliceous dolomite cobbles in the bipolar technique, breccia chert blocks for freehand hard-hammer percussion, and selected high-quality chert for retouching tools (Pei et al., 2017, 2019). This suggests a structured procurement of raw materials based on the technological requirements of each knapping activity.
Mammal fossils unearthed in archaeological sites play an important role in assessing the impact of environmental instability in human behavioral adaptations. This paper analyzed stable isotope ratios of fossil tooth enamel at the recently discovered Early Pleistocene site of MDG, in the Nihewan Basin. Isotopic data from bulk teeth enamel shows that the MDG fauna occupied a wide niche, including pure C3, C3-C4 mixed, and nearly pure C4 environments. The δ13C and δ18O profiles of tooth sections indicate substantial regional dry/cold and warm/wet fluctuations and seasonal variations.
Most likely, changing environments had an impact on human behavioral adaptations archaeologically detectable through stone tool technological variability. Previous studies (Pei et al., 2019) have discussed the technological plasticity of MDG hominins in raw material procurement strategies, knapping techniques, tool preferences and lithic reduction sequences, all of which might potentially be linked to environmental fluctuations such as those reported in this study. Further studies should explore other paleoenvironmental proxies and their application to other archaeological assemblages at the Nihewan Basin, in order to test how early humans coped with the instability characteristic of the MPT, and to portrait more accurately dynamics of hominin occupation in north China during this period.
The original contributions presented in the study are included in the article/Supplementary Material, further inquiries can be directed to the corresponding author.
ZX: Investigation, analysis, writing, and original draft preparation. SP: Investigation, designed the research and writing. YH: Academic support and writing. IdlT: Writing. DM: Investigation and analysis. All authors have contributed to the article and approved the submitted version.
This research was supported by the Strategic Priority Research Program of Chinese Academy of Sciences (Gran No. XDB26000000), the National Natural Science Foundation of China (41872029, 41372032), and an ERC-Advanced Grant (Horizon 2020; BICAEHFID grant agreement No. 832980).
The authors declare that the research was conducted in the absence of any commercial or financial relationships that could be construed as a potential conflict of interest.
The reviewer CD declared a past co-authorship with the authors SP, IT, DM to the handling Editor.
All claims expressed in this article are solely those of the authors and do not necessarily represent those of their affiliated organizations, or those of the publisher, the editors and the reviewers. Any product that may be evaluated in this article, or claim that may be made by its manufacturer, is not guaranteed or endorsed by the publisher.
We would like thank professors Wei Dong, Haowen Tong and Dr. Boyang Sun from the Institute of Vertebrate Paleontology and Paleoanthropology (IVPP), Chinese Academy of Sciences, and Dr. Xiaomin Wang from Institute of Archaeology, Chinese Academy of Social Sciences, for their help in the taxonomic identification. We are also thankful to Dr. Jiao Ma from IVPP for her help in stable isotope analysis. Further thanks are due to Dr. Hao Li from IVPP for discussions and instructive comments.
The Supplementary Material for this article can be found online at: https://www.frontiersin.org/articles/10.3389/feart.2021.789781/full#supplementary-material
Abbate, E., and Sagri, M. (2012). Early to Middle Pleistocene Homo Dispersals from Africa to Eurasia: Geological, Climatic and Environmental Constraints. Quat. Int. 267, 3–19. doi:10.1016/j.quaint.2011.02.043
Ambrose, S. H. (2001). Paleolithic Technology and Human Evolution. Science 291, 1748–1753. doi:10.1126/science.1059487
An, Z. S., Huang, Y. S., Liu, W. G., Guo, Z. T., Clemens, S., Li, L., et al. (2005). Multiple Expansions of C4 Plant Biomass in East Asia since 7 Ma Coupled with Strengthened Monsoon Circulation. Geology 33, 705–708. doi:10.1130/G21423.1
Ao, H., Deng, C., Dekkers, M. J., Sun, Y., Liu, Q., and Zhu, R. (2010). Pleistocene Environmental Evolution in the Nihewan Basin and Implication for Early Human Colonization of North China. Quat. Int. 223-224, 472–478. doi:10.1016/j.quaint.2010.02.002
Ao, H., An, Z., Dekkers, M. J., Li, Y., Xiao, G., Zhao, H., et al. (2013). Pleistocene Magnetochronology of the Fauna and Paleolithic Sites in the Nihewan Basin: Significance for Environmental and Hominin Evolution in North China. Quat. Geochronol. 18, 78–92. doi:10.1016/j.quageo.2013.06.004
Balasse, M. (2002). Reconstructing Dietary and Environmental History from Enamel Isotopic Analysis: Time Resolution of Intra-Tooth Sequential Sampling. Int. J. Osteoarchaeol. 12, 155–165. doi:10.1002/oa.601
Bar-Yosef, O., and Belfer-Cohen, A. (2001). From Africa to Eurasia - Early Dispersals. Quat. Int. 75 (1), 19–28. doi:10.1016/S1040-6182(00)00074-4
Behrensmeyer, A. K. (2006). Climate Change and Human Evolution. Science 311, 476–478. doi:10.1126/science.1116051
Biasatti, D., Wang, Y., and Deng, T. (2010). Strengthening of the East Asian Summer Monsoon Revealed by a Shift in Seasonal Patterns in Diet and Climate after 2-3Ma in Northwest China. Palaeogeogr. Palaeoclimatol. Palaeoecol. 297, 12–25. doi:10.1016/j.palaeo.2010.07.005
Bocherens, H., Fizet, M., and Mariotti, A. (1994). Diet, Physiology and Ecology of Fossil Mammals as Inferred from Stable Carbon and Nitrogen Isotope Biogeochemistry: Implications for Pleistocene Bears. Palaeogeogr. Palaeoclimatol. Palaeoecol. 107, 213–225. doi:10.1016/S0031-0182(00)00110-310.1016/0031-0182(94)90095-7
Cerling, T. E., and Harris, J. M. (1999). Carbon Isotope Fractionation between Diet and Bioapatite in Ungulate Mammals and Implications for Ecological and Paleoecological Studies. Oecologia 120, 347–363. doi:10.1007/s004420050868
Cerling, T. E., Harris, J. M., and MacFadden, B. J. (1997). “Carbon Isotopes, Diets of North American Equids, and the Evolution of North American C4 Grasslands,” in Stable Isotopes and the Integration of Biological, Ecological, and Geochemical Processes. Editors H. Griffiths, D. Robinson, and P. van Gardingen (Oxford: Bios Scientific Publishers), 363–379.
Clark, P. U., Archer, D., Pollard, D., Blum, J. D., Rial, J. A., Brovkin, V., et al. (2006). The Middle Pleistocene Transition: Characteristics, Mechanisms, and Implications for Long-Term Changes in Atmospheric pCO2. Quat. Sci. Rev. 25, 3150–3184. doi:10.1016/j.quascirev.2006.07.008
de la Torre, I., Benito-Calvo, A., Bibi, F., Njau, J., Pei, S., Rivals, F., et al. (2020). “Perspectives on the Biogeographic and Cultural Adaptations of Early Humans during the First Intercontinental Dispersals,” in The Matter of Prehistory: Papers in Honour of Antonio Gilman Guillén. Editors P. Díaz del Río, K. T. Lillios, and I. Sastre (Madrid: Bibliotheca Praehistorica Hispana), XXXVI, 73–84.
deMenocal, P. B. (1995). Plio-Pleistocene African Climate. Science 270, 53–59. doi:10.1126/science.270.5233.53
deMenocal, P. B. (2011). Climate and Human Evolution. Science 331, 540–542. doi:10.1126/science.1190683
Deng, T., Dong, J. S., and Wang, Y. (2001). Quaternary Terrestrial Ecosystem Evolution of North China Recorded by Fossil Stable Carbon Isotope (In Chinese). Chin. Sci. Bull. 46, 1213–1215. doi:10.1360/csb2001-46-14-1213
Deng, C., Shaw, J., Liu, Q., Pan, Y., and Zhu, R. (2006). Mineral Magnetic Variation of the Jingbian Loess/Paleosol Sequence in the Northern Loess Plateau of China: Implications for Quaternary Development of Asian Aridification and Cooling. Earth Planet. Sci. Lett. 241, 248–259. doi:10.1016/j.epsl.2005.10.020
Deng, C., Xie, F., Liu, C., Ao, H., Pan, Y., and Zhu, R. (2007). Magnetochronology of the Feiliang Paleolithic Site in the Nihewan Basin and Implications for Early Human Adaptability to High Northern Latitudes in East Asia. Geophys. Res. Lett. 34, 1–6. doi:10.1029/2007GL030335
Deng, C., Hao, Q., Guo, Z., and Zhu, R. (2019). Quaternary Integrative Stratigraphy and Timescale of China. Sci. China Earth Sci. 62, 324–348. doi:10.1007/s11430-017-9195-4
Dennell, R. (2003). Dispersal and Colonisation, Long and Short Chronologies: How Continuous Is the Early Pleistocene Record for Hominids outside East Africa? J. Hum. Evol. 45, 421–440. doi:10.1016/j.jhevol.2003.09.006
Dennell, R. W. (2013). The Nihewan Basin of North China in the Early Pleistocene: Continuous and Flourishing, or Discontinuous, Infrequent and Ephemeral Occupation? Quat. Int. 295, 223–236. doi:10.1016/j.quaint.2012.02.012
Ding, Z. L., Derbyshire, E., Yang, S. L., Sun, J. M., and Liu, T. S. (2005). Stepwise Expansion of Desert Environment across Northern China in the Past 3.5 Ma and Implications for Monsoon Evolution. Earth Planet. Sci. Lett. 237, 45–55. doi:10.1016/j.epsl.2005.06.036
Farquhar, G. D., Ehleringer, J. R., and Hubick, K. T. (1989). Carbon Isotope Discrimination and Photosynthesis. Annu. Rev. Plant Physiol. Plant Mol. Biol. 40, 503–537. doi:10.1146/annurev.pp.40.060189.002443
Feranec, R. S., and MacFadden, B. J. (2000). Evolution of the Grazing Niche in Pleistocene Mammals from Florida: Evidence from Stable Isotopes. Palaeogeogr. Palaeoclimatol. Palaeoecol. 162, 155–169. doi:10.1016/S0031-0182(00)00110-3
Gabunia, L., Vekua, A., Lordkipanidze, D., Swisher, C. C., Ferring, R., Justus, A., et al. (2000). Earliest Pleistocene Hominid Cranial Remains from Dmanisi, Republic of Georgia: Taxonomy, Geological Setting, and Age. Science 288 (5468), 1019–1025. doi:10.1126/science.288.5468.1019
Grove, M. (2012). Amplitudes of Orbitally Induced Climatic Cycles and Patterns of Hominin Speciation. J. Archaeological Sci. 39, 3085–3094. doi:10.1016/j.jas.2012.04.023
Hilgen, F. J., Lourens, L. J., van Dam, J. A., Beu, A. G., Boyes, A. F., Cooper, R. A., et al. (2012). “The Neogene Period,” in The Geologic Time Scale 2012. Editors F. Gradstein, J. G. Ogg, M. D. Schmitz, and G. M. Ogg (Oxford: Elsevier B. V.), 923–978. doi:10.1016/B978-0-444-59425-9.00029-9
Jia, Z., Pei, S., Benito‐Calvo, A., Ma, D., Sanchez‐Romero, L., and Wei, Q. (2019). Site Formation Processes at Donggutuo: A Major Early Pleistocene Site in the Nihewan Basin, North China. J. Quat. Sci 34, 621–632. doi:10.1002/jqs.3151
Koch, P. L., Tuross, N., and Fogel, M. L. (1997). The Effects of Sample Treatment and Diagenesis on the Isotopic Integrity of Carbonate in Biogenic Hydroxylapatite. J. Archaeological Sci. 24, 417–429. doi:10.1006/jasc.1996.0126
Kohn, M. J. (2010). Carbon Isotope Compositions of Terrestrial C3 Plants as Indicators of (Paleo)ecology and (Paleo)climate. Proc. Natl. Acad. Sci. 107, 19691–19695. doi:10.1073/pnas.1004933107
Larick, R. R., and Ciochon, R. L. (1996). The African Emergence and Early Asian Dispersals of the Genus Homo. Am. Scientist 84, 538–551.
Lee-Thorp, J. A., Sealy, J. C., and van der Merwe, N. J. (1989). Stable Carbon Isotope Ratio Differences between Bone Collagen and Bone Apatite, and Their Relationship to Diet. J. archaeological Sci. 16, 585–599. doi:10.1016/0305-4403(89)90024-1
Li, Y. C., Xu, Q. H., and Yang, X. L. (1996). Sporopollen Analysis of Feiliang Site in Yangyuan County, Hebei Province (In Chinese). Geogr. Territorial Res. 3, 55–59.
Li, X. L., Pei, S. W., Ma, N., Liu, D. C., and Peng, F. (2010). Soluble Salt Sediments and its Environmental Significance at the Donggutuo Site, Nihewan Basin (In Chinese). J. Palaeogeogr. 3, 307–314. doi:10.7605/gdlxb.2010.03.006
Liu, P., Deng, C., Li, S., Cai, S., Cheng, H., Baoyin, Y., et al. (2012). Magnetostratigraphic Dating of the Xiashagou Fauna and Implication for Sequencing the Mammalian Faunas in the Nihewan Basin, North China. Palaeogeogr. Palaeoclimatol. Palaeoecol. 315-316, 75–85. doi:10.1016/j.palaeo.2011.11.011
Mudelsee, M., and Schulz, M. (1997). The Mid-pleistocene Climate Transition: Onset of 100 Ka Cycle Lags Ice Volume Build-Up by 280 Ka. Earth Planet. Sci. Lett. 151, 117–123. doi:10.1016/s0012-821x(97)00114-3
Nian, X. M., Zhou, L. P., and Yuan, B. Y. (2013). Optically Stimulated Luminescence Dating of Terrestrial Sediments in the Nihewan Basin and its Implication for the Evolution of Ancient Nihewan lake (In Chinese). Quat. Sci. 33, 403–414. doi:10.3969/j.issn.1001-7410.2013.03.01
O'Leary, M. H. (1988). Carbon Isotopes in Photosynthesis. Bioscience 38, 328–336. doi:10.2307/1310735
Pederzani, S., and Britton, K. (2019). Oxygen Isotopes in Bioarchaeology: Principles and Applications, Challenges and Opportunities. Earth-Science Rev. 188, 77–107. doi:10.1016/j.earscirev.2018.11.005
Pei, S., Li, X., Liu, D., Ma, N., and Peng, F. (2009). Preliminary Study on the Living Environment of Hominids at the Donggutuo Site, Nihewan Basin. Chin. Sci. Bull. 54, 3896–3904. doi:10.1007/s11434-009-0646-9
Pei, S., Xie, F., Deng, C., Jia, Z., Wang, X., Guan, Y., et al. (2017). Early Pleistocene Archaeological Occurrences at the Feiliang Site, and the Archaeology of Human Origins in the Nihewan Basin, North China. Plos One 12, e0187251. doi:10.1371/journal.pone.0187251
Pei, S., Deng, C., de la Torre, I., Jia, Z., Ma, D., Li, X., et al. (2019). Magnetostratigraphic and Archaeological Records at the Early Pleistocene Site Complex of Madigou (Nihewan Basin): Implications for Human Adaptations in North China. Palaeogeogr. Palaeoclimatol. Palaeoecol. 530, 176–189. doi:10.1016/j.palaeo.2019.05.014
Potts, R., and Faith, J. T. (2015). Alternating High and Low Climate Variability: The Context of Natural Selection and Speciation in Plio-Pleistocene Hominin Evolution. J. Hum. Evol. 87, 5–20. doi:10.1016/j.jhevol.2015.06.014
Potts, R. (2012). Evolution and Environmental Change in Early Human Prehistory. Annu. Rev. Anthropol. 41, 151–167. doi:10.1146/annurev-anthro-092611-145754
Potts, R. (2013). Hominin Evolution in Settings of Strong Environmental Variability. Quat. Sci. Rev. 73, 1–13. doi:10.1016/j.quascirev.2013.04.003
Qiu, Z., and Qiu, Z. (1995). Chronological Sequence and Subdivision of Chinese Neogene Mammalian Faunas. Palaeogeogr. Palaeoclimatol. Palaeoecol. 116, 41–70. doi:10.1016/0031-0182(94)00095-p
Quade, J., Cerlinga, T. E., Barry, J. C., Morgan, M. E., Pilbeam, D. R., Chivas, A. R., et al. (1992). A 16-Ma Record of Paleodiet Using Carbon and Oxygen Isotopes in Fossil Teeth from Pakistan. Chem. Geology. Isotope Geosci. section 94, 183–192. doi:10.1016/0168-9622(92)90011-X
Raven, P. H., Event, R. F., and Eichhorn, S. E. (1999). Biology of Plants. sixth ed. New York: W.H. Freeman and Company Worth Publishers, 944.
Rivals, F., Uno, K. T., Bibi, F., Pante, M. C., Njau, J., and de la Torre, I. (2018). Dietary Traits of the Ungulates from the HWK EE Site at Olduvai Gorge (Tanzania): Diachronic Changes and Seasonality. J. Hum. Evol. 120, 203–214. doi:10.1016/j.jhevol.2017.08.011
Ruddiman, W. F., Raymo, M., and Mcintyre, A. (1986). Matuyama 41,000-year Cycles: North Atlantic Ocean and Northern Hemisphere Ice Sheets. Earth Planet. Sci. Lett. 80, 117–129. doi:10.1016/0012-821x(86)90024-5
Schick, K., Toth, N., Qi, W., Clark, J. D., and Etler, D. (1991). Archaeological Perspectives in the Nihewan Basin, China. J. Hum. Evol. 21, 13–26. doi:10.1016/0047-2484(91)90033-R
Teilhard de Chardin, P., and Piveteau, J. (1930). Les mammifères fossiles de Nihowan (Chine). Ann. de Paléontologie. 19, 1–134.
Tejada-Lara, J. V., MacFadden, B. J., Bermudez, L., Rojas, G., Salas-Gismondi, R., and Flynn, J. J. (2018). Body Mass Predicts Isotope Enrichment in Herbivorous Mammals. Proc. Biol. Sci. 285 (1881), 1–10. doi:10.1098/rspb.2018.1020
Tieszen, L. L., Boutton, T. W., Tesdahl, K. G., and Slade, N. A. (1983). Fractionation and Turnover of Stable Carbon Isotopes in Animal Tissues: Implications for ?13C Analysis of Diet. Oecologia 57, 32–37. doi:10.1007/BF00379558
Uno, K. T., Rivals, F., Bibi, F., Pante, M., Njau, J., and de la Torre, I. (2018). Large Mammal Diets and Paleoecology across the Oldowan-Acheulean Transition at Olduvai Gorge, Tanzania from Stable Isotope and Tooth Wear Analyses. J. Hum. Evol. 120, 76–91. doi:10.1016/j.jhevol.2018.01.002
Van der Made, J. (2011). Biogeography and Climatic Change as a Context to Human Dispersal Out of Africa and within Eurasia. Quat. Sci. Rev. 30 (11-12), 1353–1367. doi:10.1016/j.quascirev.2010.02.028
Van der Merwe, N. J. (2013). Isotopic Ecology of Fossil Fauna from Olduvai Gorge at Ca 1.8 Ma, Compared with Modern Fauna. South Afr. J. Sci. 109, 1–14. doi:10.1590/sajs.2013/20130105
Wang, Y., Kromhout, E., Zhang, C., Xu, Y., Parker, W., Deng, T., et al. (2008). Stable Isotopic Variations in Modern Herbivore Tooth Enamel, Plants and Water on the Tibetan Plateau: Implications for Paleoclimate and Paleoelevation Reconstructions. Palaeogeogr. Palaeoclimatol. Palaeoecol. 260, 359–374. doi:10.1016/J.PALAEO.2007.11.012
Wang, T., Sun, Y., and Liu, X. (2017). Mid-Pleistocene Climate Transition: Characteristic, Mechanism and Perspective. Chin. Sci. Bull. 62, 3861–3872. doi:10.1360/N972017-00427
Wright, L. E., and Schwarcz, H. P. (1999). Correspondence Between Stable Carbon, Oxygen and Nitrogen Isotopes in Human Tooth Enamel and Dentine: Infant Diets at Kaminaljuyú. J. Archaeological Sci. 26, 1159–1170. doi:10.1006/jasc.1998.0351
Wu, W. X., and Liu, T. S. (2001). Climate Shifts during Pleistocene and its Implications for Early Human Dispersal (In Chinese). Mar. Geology. Quat. Geology. 4, 103–109. doi:10.16562/j.cnki.0256-1492.2001.04.019
Wu, F., Fang, X., Ma, Y., Herrmann, M., Mosbrugger, V., An, Z., et al. (2007). Plio-Quaternary Stepwise Drying of Asia: Evidence from a 3-Ma Pollen Record from the Chinese Loess Plateau. Earth Planet. Sci. Lett. 257, 160–169. doi:10.1016/j.epsl.2007.02.029
Yang, S. X., Petraglia, M. D., Hou, Y. M., Yue, J. P., Deng, C. L., and Zhu, R. X. (2017). Correction: The Lithic Assemblages of Donggutuo, Nihewan basin: Knapping Skills of Early Pleistocene Hominins in North China. PLOS ONE 12, e0189565. doi:10.1371/journal.pone.0189565
Yang, S. X., Deng, C. L., Zhu, R. X., and Petraglia, M. D. (2020). The Paleolithic in the Nihewan Basin, China: Evolutionary History of an Early to Late Pleistocene Record in Eastern Asia. Evol. Anthropol. 29, 125–142. doi:10.1002/evan.21813
Yang, S. X., Wang, F. G., Xie, F., Yue, J. P., Deng, C. L., Zhu, R. X., et al. (2021). Technological Innovations at the Onset of the Mid-Pleistocene Climate Transition in High-Latitude East Asia. Natl. Sci. Rev. 8, nwaa053. doi:10.1093/nsr/nwaa053
Zhao, H., Lu, Y., Wang, C., Chen, J., Liu, J., Mao, H., et al. (2010). ReOSL Dating of Aeolian and Fluvial Sediments from Nihewan Basin, Northern China and its Environmental Application. Quat. Geochronol. 5, 159–163. doi:10.1016/j.quageo.2009.03.008
Zhou, T. R., Li, H. Z., Liu, Q. S., Li, R. Q., and Sun, X. P. (1991). Cenozoic Paleogeography of the Nihewan Basin (In Chinese). Beijing: Science Press, 162.
Zhu, R. X., Hoffman, K. A., Potts, R., Deng, C. L., Pan, Y. X., Guo, B., et al. (2001). Earliest Presence of Humans in Northeast Asia. Nature 413, 413–417. doi:10.1038/35096551
Zhu, R. X., Potts, R., Xie, F., Hoffman, K. A., Deng, C. L., Shi, C. D., et al. (2004). New Evidence on the Earliest Human Presence at High Northern Latitudes in Northeast Asia. Nature 431, 559–562. doi:10.1038/nature02829
Keywords: stable isotopes, paleoenvironmental variability, Middle Pleistocene Climate Transition (MPT), human adaptations, Madigou site, Nihewan basin, North China
Citation: Xu Z, Pei S, Hu Y, de la Torre I and Ma D (2021) Stable Isotope Analysis of Mammalian Enamel From the Early Pleistocene Site of Madigou, Nihewan Basin: Implications for Reconstructing Hominin Paleoenvironmental Adaptations in North China. Front. Earth Sci. 9:789781. doi: 10.3389/feart.2021.789781
Received: 05 October 2021; Accepted: 08 November 2021;
Published: 24 December 2021.
Edited by:
Dongju Zhang, Lanzhou University, ChinaReviewed by:
Minmin Ma, Lanzhou University, ChinaCopyright © 2021 Xu, Pei, Hu, de la Torre and Ma. This is an open-access article distributed under the terms of the Creative Commons Attribution License (CC BY). The use, distribution or reproduction in other forums is permitted, provided the original author(s) and the copyright owner(s) are credited and that the original publication in this journal is cited, in accordance with accepted academic practice. No use, distribution or reproduction is permitted which does not comply with these terms.
*Correspondence: Shuwen Pei, peishuwen@ivpp.ac.cn
Disclaimer: All claims expressed in this article are solely those of the authors and do not necessarily represent those of their affiliated organizations, or those of the publisher, the editors and the reviewers. Any product that may be evaluated in this article or claim that may be made by its manufacturer is not guaranteed or endorsed by the publisher.
Research integrity at Frontiers
Learn more about the work of our research integrity team to safeguard the quality of each article we publish.