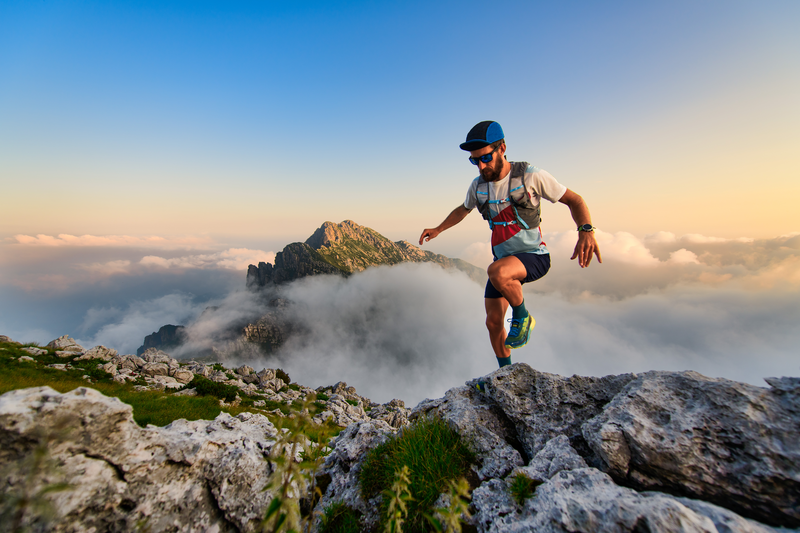
95% of researchers rate our articles as excellent or good
Learn more about the work of our research integrity team to safeguard the quality of each article we publish.
Find out more
ORIGINAL RESEARCH article
Front. Earth Sci. , 24 January 2022
Sec. Economic Geology
Volume 9 - 2021 | https://doi.org/10.3389/feart.2021.787697
This article is part of the Research Topic Reservoir Formation Conditions and Enrichment Mechanisms of Shale Oil and Gas View all 48 articles
The exploration and development of the shale in the Lower Silurian Longmaxi Formation in the Chongqing area has shown that gas production here is commercially viable. In contrast, the Longmaxi Formation shale in Hunan shows that gas production is not commercial. Why is there such a difference between the Chongqing and Hunan areas for the same formation. A mount of previous studies showed that gas is stored primarily in the organic matter (OM) pores in shale. This study has investigated the Longmaxi shale from two well locations in Chongqing and Hunan in order to understand whether differences in pore structure are the cause of the difference in commerciality in the two areas. The formation burial histories were established, analyzing samples for mineral composition, total organic carbon (TOC) content, thermal maturity (Ro), and OM pore structures. The results show that the Longmaxi shale in Chongqing and Hunan has similar TOC content and Ro. The average TOC content is above 3.0% for samples from both areas, and the thermal maturity was all between 2.4 and 2.9%. OM pores of the Longmaxi shale in Chongqing are abundant in number and relatively large sized, with mostly circular and oval shapes. In contrast, the number and size of the OM pores in the Longmaxi shale in Hunan are small, and the shape is irregular. OM pore structure of the Longmaxi shale in Chongqing is therefore favorable for preservation of gas. The poor pore structure of the Longmaxi shale in Hunan is not conducive to gas preservation. The OM pore structure difference of the Longmaxi shale in Chongqing and Hunan is determined by the formation burial history. The Longmaxi shale in the Chongqing area experienced rapid burial in the Early Jurassic and rapid uplift in the Late Cretaceous. The Longmaxi Formation in Hunan experienced rapid burial in the Early Triassic and rapid uplift in the Early Jurassic. The long evolution time (100 Ma) of the Longmaxi shale in Chongqing produced a large amount of gas supply and enough storage space (OM pores) for the gas occurrence, which makes the amount of gas be preserved in geological history. The short evolution time (50 Ma) of the Longmaxi shale in Hunan produced insufficient gas supply and not enough OM pores in reservoir, which leads to gas loss in geological history, resulting in low gas content. Therefore, to evaluate the potential of shale gas, the evolution time of organic matter must be considered. In other words, the time interval between rapid subsidence and uplift of formation during geological history must be considered.
To date, there has been successful exploration and development of the Lower Silurian Longmaxi shale in the Chongqing area in southern China. For example, the gas content of well JY1 reached 6.1 m3/t and the gas production reached 6.0 × 104 m3/d (Table 1; Figure 1, Guo et al., 2017; Guo et al., 2019; Zhao et al., 2016). In contrast, exploration and development of the Longmaxi shale in Hunan in southern China has not achieved commercial gas production (Liu et al., 2018; Xi et al., 2018; Zhai et al., 2018). For instance, in well SY1, the measured gas content is only 1.5 m3/t, with the gas production only 0.2–0.6 × 104 m3/d (Table 1; Figure 1, Wan et al., 2017; Wu et al., 2017). Why is there such a big difference in exploration and development results in the same formation between the Chongqing and Hunan areas.
TABLE 1. Reservoir parameters and gas content of the Lower Silurian Longmaxi shale in Chongqing and Hunan.
FIGURE 1. The study areas and the well locations of the Longmaxi shale in Chongqing and Hunan, South China.
Previous studies have shown that the space where gas can be effectively saved in shale is organic matter (OM) pores (Loucks et al., 2009; Loucks et al., 2012; Milliken, et al., 2013; Wang et al., 2016a). Hence, OM porosity is a prerequisite for gas accumulation, and the structural properties of OM pores also affect the abundance and flow of gas in shale (Wang et al., 2016b, Wang et al., 2018 P.; Wang Y. et al., 2018). For example, if a large number of OM pores are developed with large pore size and regular pore shape (mostly circular and oval) in shale, a large amount of gas will occur in reservoir (Jiao et al., 2014; Cao et al., 2015; Wang et al., 2019).
The development of OM pores is controlled by various geological factors, such as total organic carbon (TOC) content, mineral composition, kerogen type, and thermal maturity (Ji et al., 2017; Nie et al., 2019). In general, the higher the silica content, the more OM pores are preserved, as silica provides a mineral framework supporting the preservation of OM pores (Ji et al., 2019; Hu et al., 2019). Several studies have shown that kerogen type Ι is most beneficial to the development of OM pores (Wu et al., 2019; Hou et al., 2019). Too low and too high thermal maturity are also not conducive to the development or preservation of OM pores (Chen et al., 2014; Chen et al., 2017). In marine shale, thermal maturity of 1.5% < Ro < 3.0% is most conducive to the development of OM pores (Inan et al., 2018; Wang P. et al., 2018; Hou et al., 2019).
Different views on the OM pore evolution in shale have been proposed (Loucks and Reed, 2014; Wang et al., 2016a; Li et al., 2019). However, most experts believe that the process of petroleum generation in shale controls the evolution of OM pores, because the formation of OM pores coincides with petroleum generation from kerogen (Yang et al., 2017; Xu et al., 2019; Zhang et al., 2020). In turn, petroleum generation is controlled by burial history and thermal gradients (i.e., temperature and time), and hence, the evolution of OM pores is also a function of these parameters (He et al., 2018; He et al., 2020; Jin et al., 2018; Nie et al., 2020). As a result, the differences in burial history and/or thermal gradients in different locations can lead to differences in the evolution of OM pores in shales with similar TOC contents and kerogen types was proposed.
Many scholars have simulated the evolution of OM pores and note that OM pores develop different properties under different temperature and pressure conditions (Chen et al., 2014; Zhao et al., 2016; Hou et al., 2019). When simulating the evolution of OM pores in shale, most scholars tend to ignore the difference between geological time and laboratory time (Zhu et al., 2018; He et al., 2020; Nie et al., 2020). Geotectonic activities that result in the burial and uplift of a formation tend to last for millions of years, with experiments lasting up to months (Josh et al., 2019). The durations of maximum burial of the Longmaxi Formation in the Chongqing and Hunan areas are very different (Qin et al., 2018; Zhu et al., 2019; Li et al., 2020). This study speculated that the different burial histories of the Longmaxi shale in Chongqing and Hunan had different effects on the OM pore structure, leading to a large difference in gas content.
Therefore, this study has studied Longmaxi shale samples from the Chongqing and Hunan areas. Shale samples were analyzed for mineral composition, TOC content, thermal maturity, and OM pore structures, and the formation geological burial history was modeled in order to clarify the following aspects:
1) Reservoir characteristics; 2) OM pore structures; 3) OM pore evolution; 4) Effect of time on OM pore evolution of shale.
Chongqing is located in south China and falls within the upper Yangtze Platform depression (Chen et al., 2019; Fan et al., 2020; Wang et al., 2020), and its southeast and northeast are located at the margin of Sichuan Basin (Figure 2A). The study area is located in southeastern Chongqing. Its strata are mainly affected by the tectonic movement of the early Yanshan-late Himalaya orogeny and form a series of fold systems (Zhao et al., 2017; Nie et al., 2019; Nie et al., 2020; Xu et al., 2020). The study area has experienced only weak deformation, with less fault development and less compressive tectonic deformation than the area outside the basin (Wang et al., 2016b; Wang et al., 2018 P.; Ji et al., 2017, 2019).
Due to the large-scale transgression in the Early Silurian, the marine Longmaxi Formation was deposited in and around the Sichuan Basin as a result of sea level rise (Zhao et al., 2016; Jin et al., 2018). The lower part of the Longmaxi Formation is deep-water shelf deposition, and it contains abundant graphitic stones and local enrichment of banded pyrite (Hu et al., 2017; He et al., 2020; Xu et al., 2020). Upper part of the Longmaxi Formation is shallow shelf deposit, which contains a small amount of locally rich blocks and tuberous pyrite, reflecting that the water column shallowed upwards (Figure 3, Jin et al., 2018; He et al., 2020). The black organic matter (OM)-rich shale in this study area is located at the bottom of the Longmaxi Formation (Guo et al., 2017). The Longmaxi shale is widely distributed laterally and with a thickness between 40 and 210 m in the Chongqing area (Wang et al., 2016b; Wang et al., 2018 P.).
FIGURE 3. (A) Structural plan and cross-section through southeastern Chongqing (figure modified from Zhao et al., 2017). (B) Structural plan and section of northwestern Hunan (figure modified from Xi et al., 2018).
The study area is in Northwest Hunan, which is situated on the southeast margin of the Yangtze Platform, spanning two secondary tectonic units, bounded by the Baojing-Cili fault zone, southeast of which is the Jiangnan-Xuefeng nappe uplift zone, and to northwest is located the west Xiang-Exi fold zone (Figure 2B, Yang et al., 2016; Xi et al., 2019). The study area is located in the Sangzhi-Shimen syncline. which is a secondary tectonic unit in the southernmost part of the western Xiang-Exi block (Zhai et al., 2017).
From the Sinian to the early Paleozoic, a cratonic marine basin developed in the northwest Hunan area. The Late Ordovician-Early Silurian period was the tectonic transition stage of the middle and upper Yangtze Platform, and the study area was transformed from a passive continental margin basin to a foreland flexural basin (Xi et al., 2018; Zheng et al., 2019). The Longmaxi shale belongs to a relatively closed deep-water shelf sedimentary environment in the study area, and black carbonaceous and siliceous organic-rich shale with an average thickness of 52 m (Wan et al., 2017; Xi et al., 2019).
The Longmaxi shale samples were selected from the JY1 well in the Chongqing area and the SY1 well in the Hunan area (Table 2). Including 22 samples were selected from the JY1 well in Chongqing and 24 samples from the SY1 well in Hunan.
TABLE 2. The Longmaxi shale samples that are used for FIB-SEM analysis in Figures 7, 8 and their reservoir characteristics in Chongqing and Hunan.
The TOC content was determined by LECO CS-200 carbon sulfur analyzer. Before the test, the shale sample was crushed to 0.15 mm size, and carbonate content was removed with dilute hydrochloric acid. Then, acid was removed by repeated washing with fresh water.
The mineral composition was analyzed by Bruker D8 DISCOVER X-ray diffraction (XRD). Prior to analysis, samples were crushed to size less than 0.04 mm in diameter.
The Longmaxi shale lacks vitrinite due to its age (Zhao et al., 2016). Leica DM4500P polarizing microscope and a CRAIC microspectrophotometer were used to test the Rb. Before analysis, shale samples were polished at 20°C and 40% relative humidity. Then, the Rb was converted to equivalent vitrinite reflectance (Eq-Ro) based on the following linear regression: Eq-Ro = 0.618Rb+0.4 (Jacob et al., 1989).
HELIOS-NanoLab 650 instrument (made by FEI Corporation, United States) was used to survey OM pore structure characteristic. The secondary electron emission technology of FIB-SEM can brighten OM pores, highlighting the pore surface morphology (Wang P. et al., 2018). The preparation of FIB-SEM samples included argon ion polish and carbon spray.
TOC content average value of Chongqing samples is 3.0%, and the highest TOC content value of the samples reaches 5.2% (Figure 4). TOC content average value of Hunan samples is 3.1%, and the highest TOC content value reaches 4.5% (Figure 4).
The distribution range of Eq-Ro of the samples in Chongqing is 1.93–2.78%, with a mean value of 2.45% (Figure 5). Distribution range of Eq-Ro of the shale samples in Hunan is 2.14–2.84%, with an average value of 2.55% (Figure 5). The results show that the thermal maturity of the Longmaxi shale in these two wells from Chongqing and Hunan is very similar, and at both locations they are overmature or at best in the dry gas window (Wan et al., 2017; Nie et al., 2020).
The shale samples from Chongqing have an average clay mineral content of 39.4% and a range from 22.3 to 65.4% (Figure 6). The range in carbonate content ranges from 7.9–18.5% (Figure 6). The siliceous minerals are mainly quartz and feldspar, of which the quartz content ranges from 24.7 to 51.5% (Figure 6).
The shale samples from Hunan have an average clay mineral content of 38.5% with a range from 21.9 to 58.6% (Figure 6). The carbonate content varies from 6.7–23.4% (Figure 6). The siliceous minerals are mainly quartz and feldspar, of which the quartz content ranges from 25.8 to 61.3% (Figure 6).
The samples from Chongqing and Hunan are dominated by brittle minerals, in which the quartz content is relatively high, while the clay mineral contents are also high and there is a lesser amount of carbonate minerals (Figure 6). The mineral compositions of the shale samples from Chongqing and Hunan have similar compositions (Figure 6).
FIB-SEM reveals that the Chongqing samples have developed a big amount of OM pores with large diameters, mostly between 100 and 500 nm (Figure 7). The OM pores are mostly round and oval. By further magnifying the observation multiples, it was found that many OM pores with small diameter were nested in those with large size.
FIGURE 7. OM pore characteristics of the Longmaxi shale samples from Chongqing (YC4 well, 774 m and JY1 well, 2405 m, G-H, J, YC4 well, 756 m; I, L, JY1 well, 2405 m). A large number of OM pores with large size (100–500 nm) are seen in pyrobitumen. OM pores are mostly round and oval in shape. (A,B) were revised from Wang et al. (2016a). (E,F) were revised from Wang et al., 2020). OM pores with small size are nested in larger OM pores. (G,J) were revised from (Wang et al., 2020).
The OM pore sizes from FIB-SEM are concentrated in the range of 15–60 nm, and the shapes are irregular (Figure 8). Moreover, the amount of OM pores in Hunan samples is significantly smaller than that in Chongqing. It is uncommon to see smaller OM pores embedded in the larger OM pores with large size.
FIGURE 8. OM pore microscope characteristics of the Longmaxi shale samples in Hunan (SY1 well, 1,569.2 m). OM pores with small sizes (20–100 nm) are seen in the pyrobitumen.OM pores are irregular in shape.
OM pore structure of the Chongqing shale samples undoubtedly improves the gas adsorption capacity of reservoir. On the contrary, the OM pore structure of the Hunan samples is not so good as that in Chongqing, which ultimately leads to the difference of gas content in shale of the two regions. Therefore, it is of great significance to clarify the reasons for the difference in the OM pore structure of the Longmaxi shale in Chongqing and Hunan.
As mentioned above, the OM pore structure in marine shale is mainly controlled by kerogen type, TOC content, thermal maturity, mineral composition, and thermal evolution (Loucks et al., 2009; Loucks et al., 2012; Milliken et al., 2013). The results of a series of reservoir characteristics experiments from Chongqing samples and Hunan show that they have similar TOC content, thermal maturity, and mineral composition (Figures 5–7). Research results have also been suggested that the Longmaxi shale kerogen types are also Ⅰ type in the two regions (Wang et al., 2020a; 2020b).
Obviously, these similar reservoir geological factors are not the reason for the difference of OM pore structure in Chongqing and Hunan samples, so only the thermal evolution process of the shale needs detailed comparative study. To analyze the thermal evolution process, it is necessary to combine the burial history of formations and regional structural characteristics to conduct a comprehensive study.
OM pore evolution is synchronized with the thermal evolution process (Chen et al., 2014; Hou et al., 2019). In the course of geologic history, tectonic evolution determines the subsidence and uplift of formation (He et al., 2018; Zhu et al., 2019). The continuous burial of strata has an absolute control over the formation of hydrocarbon gas and OM pores in shale (Guo et al., 2017; Jin et al., 2018). At the thermal evolution threshold, the solid kerogen of shale begins to form liquid hydrocarbons, with a small amount of OM pores (Wang P. et al., 2018).
With the increase of stratum subsidence and the enhancement of thermal evolution, liquid hydrocarbons from solid kerogen that were trapped in the reservoir will evolve into pyrobitumen (Wang et al., 2020a; 2020b). Pyrolysis of the solid kerogen and pyrobitumen in shale continues and a large number of gaseous hydrocarbons were generated (Chen et al., 2014). When the depth of stratum reaches the maximum, the pyrobitumen reaches the peak of gas generation with the maximum rate of OM pore formation (Hou et al., 2019).
When the formation began to rise, the thermal evolution began to weaken, and the production of thermal evolution and OM pores decreased (Guo et al., 2017; He et al., 2020). When the formation continues to rise to a certain depth, the thermal evolution of organic matter in shale stops, and the formation of OM pores also stops accordingly (Wu et al., 2019).
The thermal evolution of shale will affect gas accumulation in the following three aspects:
1) Start time of shale formation thermal evolution
Gas generation is always a process of dispersal (Wang P. et al., 2018; Jin et al., 2018). The later the thermal evolution begins, the more gas is left in the reservoir (He et al., 2018; Nie et al., 2020). In the thermal evolution process, the gas will always be a dispersion process after generation, and the longer the duration, the greater the amount of dispersion (Zhang et al., 2020). This requires that the thermal evolution start as late as possible (i.e., the later the first rapid subsidence of the formation is, the better). That is, the later the thermal evolution starts, the later the gas loss starts, and the more gas reserves there are.
2) End time of shale formation thermal evolution
Continuous gas supply will replenish the amount of lost gas and maintain a dynamic balance between gas generation and loss (Hu et al., 2019; Wang et al., 2020b). If the formation is lifted too early, the thermal evolution of organic matter in the shale will end earlier, which cannot guarantee that there will be a continuous supply of gas in the reservoir to slow the gas loss. Therefore, the later the thermal evolution ends (the later the last rapid rise of formation), the longer the evolution duration will be, and the more favorable it will be for the continuous evolution of OM to generate a mass of gas (Zhao et al., 2017). If the hydrocarbon evolution lasts for a long time and there is enough gas to supply in the shale reservoir, this can effectively slow the gas loss (Jin et al., 2018).
3) Time interval of structure and thermal evolution
Continuous and long time thermal evolution can make organic matter fully evolve and form excellent OM pore structure. After the last rapid uplift of the formation, the thermal evolution ends, and the supply of continuous gas hydrocarbon ends at the same time, and the loss of gas begins to increase (Zhao et al., 2017; He et al., 2018; Nie et al., 2019). At this time, if there is no large amount of OM pores which can store gas effectively, the gas generated in the previous evolution process will be lost in large quantities during the geological history (Zhao et al., 2017; Nie et al., 2020). Therefore, the later the thermal evolution ends (the later the last rapid rise of formation), the longer the evolution duration will be, and the more favorable it will be for the continuous evolution of organic matter to generate excellent OM pore structure (Figures 7, 8). OM pore structure formed during the thermal evolution of shale will not change (provided that the formation will not undergo a second large and rapid settlement after the first large and rapid settlement). If the hydrocarbon evolution lasts for a long time and there is a good OM pore structure to supply effective space for gas occurrence in the shale reservoir, this also can effectively slow the gas loss.
Taking the burial history of Well JY1 as an example, the maximum burial depth of the Longmaxi Formation shale in Chongqing is 6,400 m (Figure 9A). Thermal evolution degree of the Longmaxi Formation shale has reached the deep high-temperature dry gas generation (Jin et al., 2018). Taking the burial history of Well SY1 as an example (Figure 9B), the maximum burial depth of the Longmaxi shale in Hunan is 6,000 m (Wan et al., 2017), which is not much different from that in Chongqing, which also leads to similar thermal maturity of the shale in the two regions.
FIGURE 9. (A) Burial history of the Longmaxi shale in Chongqing based on JY1 well, revised from Jin et al. (2018). (B) Burial history of the Longmaxi shale in Hunan based on SY1 well, revised from Wan et al. (2017).
The burial history of Well JY1 in Chongqing and Well SY1 in Hunan was taken as examples for comparative analysis:
1) The Longmaxi Formation in Chongqing was rapidly buried in the early Jurassic period. The rapid uplift occurred in the late Cretaceous and evolution lasted for about 100 Ma (Figure 12).
After Silurian deposition, the Longmaxi Formation in Chongqing was in the stage of rapid subsidence. The rapid and large subsidence occurred in the late Jurassic in the Jurassic periods (Figure 9A). After the Cretaceous period, the formation began to rise rapidly, and the thermal evolution lasted for a long time, reaching 100 Ma, leading to the formation of a large number of hydrocarbon gases and excellent OM pore structure (Figure 9A, and Guo et al., 2017). The continuous evolution time and sufficient evolution process provide a large amount of gas supply and excellent storage space (OM Pores) for the gas occurrence, so that shale gas is preserved to the maximum extent in the geological history process (Figures 10A, 11A; Table 3).
2) The rapid burial time of the Longmaxi Formation in Hunan is early Triassic. The rapid uplift time of the formation was in the early Jurassic, and the evolution time was about 50 Ma (Figure 9B).
FIGURE 10. OM pore evolution of the Longmaxi shale in Chongqing and Hunan. (A) Longmaxi shale in Chongqing; (B) Longmaxi shale in Hunan.
FIGURE 11. Gas accumulation and OM pore structure model of the Longmaxi shale in Chongqing and Hunan. (A) Abundant large OM pores with amount of gas were found in the Longmaxi shale in Chongqing; (B) Few OM pores and little gas were found in the Longmaxi shale in Hunan.
TABLE 3. Tectonic subsidence and uplift time of the Longmaxi Formation in Chongqing and Hunan. Some data of the Longmaxi shale in Chongqing and Hunan from Jin et al. (2018), Wan et al. (2017), and Zhai et al. (2017).
The Longmaxi Formation settled rapidly in the early Triassic and uplift rapidly in the early Jurassic in Hunan (Figure 10B). Due to the early and rapid rise of formation, the thermal evolution ended prematurely, the evolution duration was short (50 Ma), and the evolution was not sufficient (Figure 10B). On the one hand, it leads to the lack of effective gas supply in the reservoir; on the other hand, due to the missing of good OM pore structure, it is unable to supply efficient storage space for the occurrence of gas (Figure 11B), which leads to a large amount of gas loss in the later geological history, thus leading to the low gas content in the current shale reservoir (Figure 11B; Table 3).
The burial and uplift of formation are controlled by regional tectonic activity. The marine shale in the Lower Paleozoic distributed in Chongqing and Hunan has experienced many periods of tectonic movement (Zhai et al., 2017; Liu et al., 2018). Different areas experience different time and stage of tectonic movement, which leads to different thermal evolution history of shale, thus leading to different thermal evolution and OM pore structure.
Taking the age of tectonic activity in Chongqing and Hunan as an example, the most recent significant and rapid uplift of formation in the geological history of the two regions occurred in the late Yanshan-early Himalayan period (Zhai et al., 2017; Li et al., 2020). At this time, Chongqing and Hunan suffered the most intense tectonic activity, which had the greatest influence on the tectonic evolution of this area.
It can be seen that the last large uplift time of the formation in Chongqing area during the geological history was about 120 Ma (Figure 12, Zhai et al., 2017), which corresponds to the Cretaceous period and coincides with the uplift time of the Longmaxi Formation in this area according to the burial history of Well JY1 (Figure 9A). This also explains why the Longmaxi shale in Chongqing rose rapidly in the late Cretaceous period. The last significant uplift time of the formation in Hunan was about 165–200 Ma (Figure 12), which corresponds to the Jurassic period, and coincides with the uplift time of the Longmaxi Formation in this area according to the burial history of Well SY1 (Figure 9B). This also explains why the Longmaxi shale in Hunan rose rapidly in the early Jurassic.
FIGURE 12. Tectonic activity age in Chongqing and Hunan regions, revised from Zhai et al. (2017).
Therefore, the evaluation of shale gas exploration and development potential should not only consider the reservoir geological factors such as TOC content, thermal maturity, mineral composition, and kerogen type, but also consider the continuous thermal evolution time of shale in the process of geologic history. The continuous and sufficient thermal evolution time of shale is controlled by the earliest subsidence and the latest uplift time of formation. If the formation sedimentation time is early and the uplift time is late, then the thermal evolution of shale lasts long, and the organic matter has enough time to fully evolve, thus generating enough gas and OM pores, creating material and storage conditions for shale gas accumulation.
Focused ion beam-scanning electron microscope (FIB-SEM) was used to observe organic matter (OM) pores of the lower Silurian Longmaxi shale samples in Chongqing and Hunan with similar TOC contents, thermal maturities, mineral composition, and kerogen type. The following are conclusions:
1) OM pore structure of the Longmaxi shale in Chongqing and Hunan is obviously different. OM pores of the Longmaxi shale in Chongqing have large size with circles and ellipses under the microscope, which improves the gas adsorption and connectivity of shale. OM pores of the Hunan Longmaxi shale samples have small size with irregular shape under the microscope. OM pore structure difference is the main reason for shale gas content difference between the two regions.
2) The reason for the OM pore structure difference of the Chongqing and Hunan shale samples is the thermal evolution time. The Longmaxi Formation in the Chongqing area was rapidly buried in the early Jurassic period. The rapid uplift occurred in the late Cretaceous and thermal evolution lasted for about 100 Ma. The rapid burial time of the Longmaxi Formation in Hunan is early Triassic. The rapid uplift time is in the early Jurassic, and the thermal evolution time is about 50 Ma.
3) The thermal evolution of the Longmaxi shale in the Chongqing area lasted for a long time, and amount of gas and OM pores were formed. The long thermal evolution time provides a large number of gas source supply and effective storage space (OM pores) for the gas occurrence, which maximizes the preservation of shale gas in the geological history. The thermal evolution time of the Longmaxi shale in the Hunan area is short. There is no enough time to evolve so that the shale reservoir does not get enough continuous gas supply and OM pores provide effective space for gas occurrence in Hunan.
4) To evaluate shale gas exploration and development potential, the continuous thermal evolution time must be considered. Long-term continuous thermal evolution will provide not only continuous gas supply for shale reservoir but also good organic matter pore structure for gas occurrence. The continuous thermal evolution time is controlled by the earliest rapid subsidence and the latest rapid uplift time of formation. If the formation subsidence time is early and the uplift time is late, the thermal evolution of shale will last long. There will be enough time for thermal evolution to generate a large amount of gas and good OM pore structure, creating material and storage conditions for shale gas accumulation.
The raw data supporting the conclusion of this article will be made available by the authors, without undue reservation.
XL: supervision, resources, methodology, formal analysis, investigation, manuscript, and review and editing. PW: conceptualization, data curation, formal analysis, methodology, resources, software, supervision, investigation, writing original draft, and review and editing. SW, CJ and RZ : formal analysis, investigation, manuscript, and review and editing CZ: supervision, methodology, data curation, resources, writing, and review and editing. ZJ: resources and project administration.
This work is financially supported by the grants from the National Natural Science Foundation of China (No. 42002050), Open project of Key Laboratory of Structure and Petroleum Resources, Ministry of Education (No. TPR-2021-19), and the China Postdoctoral Science Foundation Funded Project (No. 2020M680815).
XL and SW were employed by the company China National Offshore Oil Corporation Research Institute, and CJ was employed by the company Kunlun Digital Technology Co., Ltd.
The remaining authors declare that the research was conducted in the absence of any commercial or financial relationships that could be construed as a potential conflict of interest.
All claims expressed in this article are solely those of the authors and do not necessarily represent those of their affiliated organizations, or those of the publisher, the editors and the reviewers. Any product that may be evaluated in this article, or claim that may be made by its manufacturer, is not guaranteed or endorsed by the publisher.
Cao, T., Song, Z., Wang, S., Cao, X., Li, Y., and Xia, J. (2015). Characterizing the Pore Structure in the Silurian and Permian Shales of the Sichuan Basin, China. Mar. Pet. Geology 61, 140–150. doi:10.1016/j.marpetgeo.2014.12.007
Chen, Q., Kang, Y., You, L., Yang, P., Zhang, X., and Cheng, Q. (2017). Change in Composition and Pore Structure of Longmaxi Black Shale during Oxidative Dissolution. Int. J. Coal Geology 172, 95–111. doi:10.1016/j.coal.2017.01.011
Chen, S., Zhu, Y., Qin, Y., Wang, H., Liu, H., and Fang, J. (2014). Reservoir Evaluation of the Lower Silurian Longmaxi Formation Shale Gas in the Southern Sichuan Basin of China. Mar. Pet. Geology 57, 619–630. doi:10.1016/j.marpetgeo.2014.07.008
Chen, Z., Li, W., Wang, L., Lei, Y., Yang, G., Zhang, B., et al. (2019). Structural Geology and Favorable Exploration prospect Belts in Northwestern Sichuan Basin, SW China. Pet. Exploration Development 46 (2), 413–425. doi:10.1016/s1876-3804(19)60022-4
Fan, C., Li, H., Qin, Q., He, S., and Zhong, C. (2020). Geological Conditions and Exploration Potential of Shale Gas Reservoir in Wufeng and Longmaxi Formation of southeastern Sichuan Basin, China. J. Pet. Sci. Eng. 191, 107138. doi:10.1016/j.petrol.2020.107138
Guo, X., Hu, D., Li, Y., Wei, Z., Wei, X., and Liu, Z. (2017). Geological Factors Controlling Shale Gas Enrichment and High Production in Fuling Shale Gas Field. Pet. Exploration Development 44 (4), 513–523. doi:10.1016/s1876-3804(17)30060-5
Guo, X., Qin, Z., Yang, R., Dong, T., He, S., Hao, F., et al. (2019). Comparison of Pore Systems of clay-rich and Silica-Rich Gas Shales in the Lower Silurian Longmaxi Formation from the Jiaoshiba Area in the Eastern Sichuan Basin, China. Mar. Pet. Geology 101, 265–280. doi:10.1016/j.marpetgeo.2018.11.038
He, Z., Li, S., Nie, H., Yuan, Y., and Wang, H. (2018). The Shale Gas “sweet Window”: “the Cracked and Unbroken” State of Shale and its Depth Range. Mar. Petrol. Geol. 101, 334–342. doi:10.1016/j.marpetgeo.2018.11.033
He, Z., Nie, H., Li, S., Luo, J., Wang, H., and Zhang, G. (2020). Differential Enrichment of Shale Gas in Upper Ordovician and Lower Silurian Controlled by the Plate Tectonics of the Middle-Upper Yangtze, south China. Mar. Pet. Geology 118, 104357. doi:10.1016/j.marpetgeo.2020.104357
Hou, Y., Zhang, K., Wang, F., He, S., Dong, T., Wang, C., et al. (2019). Structural Evolution of Organic Matter and Implications for Graphitization in Over-mature marine Shales, south China. Mar. Pet. Geology 109, 304–316. doi:10.1016/j.marpetgeo.2019.06.009
Hu, H., Hao, F., Lin, J., Lu, Y., Ma, Y., and Li, Q. (2017). Organic Matter-Hosted Pore System in the Wufeng-Longmaxi (O 3 W-S 1 1) Shale, Jiaoshiba Area, Eastern Sichuan Basin, China. Int. J. Coal Geology 173, 40–50. doi:10.1016/j.coal.2017.02.004
Hu, X., Chen, L., Qi, L., Lei, Z., and Luo, Y. (2019). Marine Shale Reservoir Evaluation in the Sichuan Basin-A Case Study of the Lower Silurian Longmaxi marine Shale of the B201 Well in the Baoluan Area, Southeast Sichuan Basin, China. J. Pet. Sci. Eng. 182, 106339. doi:10.1016/j.petrol.2019.106339
Inan, S., Badairy, H. A., Inan, T., and Zahrani, A. A. (2018). Formation and Occurrence of Organic Matter-Hosted Porosity in Shales. Int. J. Coal Geo. 199, 39–51. doi:10.1016/j.coal.2018.09.021
Ji, L., Su, L., Wu, Y., and He, C. (2017a). Pore Evolution in Hydrocarbon-Generation Simulation of Organic Matter-Rich Muddy Shale. Pet. Res. 2 (2), 146–155. doi:10.1016/j.ptlrs.2017.07.002
Ji, W., Hao, F., Schulz, H.-M., Song, Y., and Tian, J. (2019). The Architecture of Organic Matter and its Pores in Highly Mature Gas Shales of the Lower Silurian Longmaxi Formation in the Upper Yangtze Platform, south China. Bulletin 103 (12), 2909–2942. doi:10.1306/04101917386
Ji, W., Song, Y., Rui, Z., Meng, M., and Huang, H. (2017b). Pore Characterization of Isolated Organic Matter from High Matured Gas Shale Reservoir. Int. J. Coal Geology 174, 31–40. doi:10.1016/j.coal.2017.03.005
Jiao, K., Yao, S., Liu, C., Gao, Y., Wu, H., Li, M., et al. (2014). The Characterization and Quantitative Analysis of Nanopores in Unconventional Gas Reservoirs Utilizing FESEM-FIB and Image Processing: An Example from the Lower Silurian Longmaxi Shale, Upper Yangtze Region, China. Int. J. Coal Geology 128-129, 1–11. doi:10.1016/j.coal.2014.03.004
Jin, Z., Nie, H., Liu, Q., Zhao, J., and Jiang, T. (2018). Source and Seal Coupling Mechanism for Shale Gas Enrichment in Upper Ordovician Wufeng Formation - Lower Silurian Longmaxi Formation in Sichuan Basin and its Periphery. Mar. Pet. Geology 97, 78–93. doi:10.1016/j.marpetgeo.2018.06.009
Josh, M., Delle Piane, C., Esteban, L., Bourdet, J., Mayo, S., Pejcic, B., et al. (2019). Advanced Laboratory Techniques Characterising Solids, Fluids and Pores in Shales. J. Pet. Sci. Eng. 180, 932–949. doi:10.1016/j.petrol.2019.06.002
Li, F., Wang, M., Liu, S., and Hao, Y. (2019). Pore Characteristics and Influencing Factors of Different Types of Shales. Mar. Pet. Geology 102, 391–401. doi:10.1016/j.marpetgeo.2018.11.034
Li, S., Li, Y., He, Z., Chen, K., Zhou, Y., and Yan, D. (2020). Differential Deformation on Two Sides of Qiyueshan Fault along the Eastern Margin of Sichuan Basin, China, and its Influence on Shale Gas Preservation. Mar. Pet. Geology 121, 104602. doi:10.1016/j.marpetgeo.2020.104602
Liu, A., Ou, W., Huang, H., Wei, K., Li, H., and Chen, X. (2018). Significance of Paleo-Fluid in the Ordovician-Silurian Detachment Zone to the Preservation of Shale Gas in Western Hunan-Hubei Area. Nat. Gas Industry B 5 (6), 565–574. doi:10.1016/j.ngib.2018.11.004
Loucks, R. G., Reed, R. M., Ruppel, S. C., and Hammes, U. (2012). Spectrum of Pore Types and Networks in Mudrocks and a Descriptive Classification for Matrix-Related Mudrock Pores. Bulletin 96 (6), 1071–1098. doi:10.1306/08171111061
Loucks, R. G., Reed, R. M., Ruppel, S. C., and Jarvie, D. M. (2009). Morphology, Genesis, and Distribution of Nanometer-Scale Pores in Siliceous Mudstones of the Mississippian Barnett Shale. J. Sediment. Res. 79, 848–861. doi:10.2110/jsr.2009.092
Loucks, R. G., and Reed, R. M. (2014). Scanning-electron-microscope Petrographic Evidence for Distinguishing Organic-Matter Pores Associated with Depositional Organic Matter versus Migrated Organic Matter in Mudrock. Gulf. Coast. Assoc. Geo. Soc. J. 3, 51–60.
Milliken, K. L., Rudnicki, M., Awwiller, D. N., and Zhang, T. (2013). Organic Matter-Hosted Pore System, Marcellus Formation (Devonian), Pennsylvania. Bulletin 97 (2), 177–200. doi:10.1306/07231212048
Nie, H., Li, D., Liu, G., Lu, Z., Hu, W., Wang, R., et al. (2020). An Overview of the Geology and Production of the Fuling Shale Gas Field, Sichuan basin, china. Energ. Geosci. 1, 147–164. doi:10.1016/j.engeos.2020.06.005
Nie, H., Sun, C., Liu, G., Du, W., and He, Z. (2019). Dissolution Pore Types of the Wufeng Formation and the Longmaxi Formation in the Sichuan basin, south china: Implications for Shale Gas Enrichment. Mar. Pet. Geology 101, 243–251. doi:10.1016/j.marpetgeo.2018.11.042
Qin, M., Guo, J., He, H., Huang, Y., Jiao, P., Liu, C., et al. (2018). Geological Conditions and Gas-Bearing Characteristics of Shale Gas in Complex Structure Area Out of Sichuan basin: a Case of Wufeng—Longmaxi Formation in Northwestern Hunan, China. J. Cent. South. Univ. (Sci Tech). 49 (8), 1979–1990. (Chinese in English abstract). doi:10.11817/j.issn.1672-7207.2018.08.019
Wan, Y., Tang, S., and Pan, Z. (2017). Evaluation of the Shale Gas Potential of the Lower Silurian Longmaxi Formation in Northwest Hunan Province, China. Mar. Pet. Geology 79, 159–175. doi:10.1016/j.marpetgeo.2016.11.010
Wang, P., Jiang, Z., Chen, L., Yin, L., Li, Z., ZhangWang, C. G., et al. (2016b). Pore Structure Characterization for the Longmaxi and Niutitang Shales in the Upper Yangtze Platform, South China: Evidence from Focused Ion Beam-He Ion Microscopy, Nano-Computerized Tomography and Gas Adsorption Analysis. Mar. Pet. Geology 77, 1323–1337. doi:10.1016/j.marpetgeo.2016.09.001
Wang, P., Jiang, Z., Han, B., Lv, P., Jin, C., Zhang, K., et al. (2018a). Reservoir Characteristics and Controlling Factor of Shale Gas in Lower Cambrian Niutitang Formation, South China. Pet. Res. 3 (3), 210–220. doi:10.1016/j.ptlrs.2018.05.003
Wang, P., Jiang, Z., Ji, W., Zhang, C., Yuan, Y., Chen, L., et al. (2016a). Heterogeneity of Intergranular, Intraparticle and Organic Pores in Longmaxi Shale in Sichuan Basin, South China: Evidence from SEM Digital Images and Fractal and Multifractal Geometries. Mar. Pet. Geology 72, 122–138. doi:10.1016/j.marpetgeo.2016.01.020
Wang, P., Yao, S., Jin, C., Li, X., Zhang, K., Liu, G., et al. (2020a). Key Reservoir Parameter for Effective Exploration and Development of High-Over Matured marine Shales: A Case Study from the Cambrian Niutitang Formation and the Silurian Longmaxi Formation, south China. Mar. Petrol. Geol. 121, 104629. doi:10.1016/j.marpetgeo.2020.104619
Wang, P., Zhang, C., Li, X., Zhang, K., Yuan, Y., Zang, X., et al. (2020b). Organic Matter Pores Structure and Evolution in Shales Based on the He Ion Microscopy (HIM): A Case Study from the Triassic Yanchang, Lower Silurian Longmaxi and Lower Cambrian Niutitang Shales in China. J. Nat. Gas Sci. Eng. 84, 103682. doi:10.1016/j.jngse.2020.103682
Wang, Q., Wang, T., Liu, W., Zhang, J., Feng, Q., Lu, H., et al. (2019). Relationships Among Composition, Porosity and Permeability of Longmaxi Shale Reservoir in the Weiyuan Block, Sichuan Basin, China. Mar. Pet. Geology 102, 33–47. doi:10.1016/j.marpetgeo.2018.12.026
Wang, Y., Wang, L., Wang, J., Jiang, Z., Jin, C., and Wang, Y. (2018b). Characterization of Organic Matter Pores in Typical marine and Terrestrial Shales, China. J. Nat. Gas Sci. Eng. 49, 56–65. doi:10.1016/j.jngse.2017.11.002
Wu, J., Zhang, S., Cao, H., Sun, P., and Luo, X. (2017). Fracability Evaluation of Shale Gas Reservoir-A Case Study in the Lower Cambrian Niutitang Formation, Northwestern Hunan, China. J. Petro. Sci. Eng. 164, 675–684. doi:10.1016/j.petrol.2017.11.055
Wu, S., Yang, Z., Zhai, X., Cui, J., Bai, L., Pan, S., et al. (2019). An Experimental Study of Organic Matter, Minerals and Porosity Evolution in Shales within High-Temperature and High-Pressure Constraints. Mar. Pet. Geology 102, 377–390. doi:10.1016/j.marpetgeo.2018.12.014
Xi, Z., Tang, S., and Wang, J. (2018). The Reservoir Characterization and Shale Gas Potential of the Niutitang Formation: Case Study of the SY Well in Northwest Hunan Province, South China. J. Pet. Sci. Eng. 171, 687–703. doi:10.1016/j.petrol.2018.08.002
Xi, Z., Tang, S., Zhang, S., Yi, Y., Dang, F., and Ye, Y. (2019). Characterization of Quartz in the Wufeng Formation in Northwest Hunan Province, south China and its Implications for Reservoir Quality. J. Pet. Sci. Eng. 179, 979–996. doi:10.1016/j.petrol.2019.04.051
Xu, H., Zhou, W., Zhang, R., Liu, S., and Zhou, Q. (2019). Characterizations of Pore, mineral and Petrographic Properties of marine Shale Using Multiple Techniques and Their Implications on Gas Storage Capability for Sichuan Longmaxi Gas Shale Field in China. Fuel 241, 360–371. doi:10.1016/j.fuel.2018.12.035
Xu, S., Gou, Q., Hao, F., Zhang, B., Shu, Z., and Zhang, Y. (2020). Multiscale Faults and Fractures Characterization and Their Effects on Shale Gas Accumulation in the Jiaoshiba Area, Sichuan Basin, China. J. Pet. Sci. Eng. 189, 107026. doi:10.1016/j.petrol.2020.107026
Yang, R., Hao, F., He, S., He, C., Guo, X., Yi, J., et al. (2017). Experimental Investigations on the Geometry and Connectivity of Pore Space in Organic-Rich Wufeng and Longmaxi Shales. Mar. Pet. Geology 84, 225–242. doi:10.1016/j.marpetgeo.2017.03.033
Yang, X., Fan, T., and Wu, Y. (2016). Lithofacies and Cyclicity of the Lower Cambrian Niutitang Shale in the Mayang basin of Western Hunan, south china. J. Nat. Gas Sci. Eng. 28, 74–86. doi:10.1016/j.jngse.2015.11.007
Zhai, G.-y., Wang, Y., Wang, Y.-f., Zhou, Z., Yu, S.-f., Chen, X.-l., et al. (2018). Exploration and Research Progress of Shale Gas in China. China. Geo. 1, 257–272. doi:10.31035/cg2018024
Zhai, G., Wang, Y., Bao, S., Guo, T., Zhou, Z., Chen, X., et al. (2017). Major Factors Controlling the Accumulation and High Productivity of Marine Shale Gas and Prospect Forecast in Southern China. Earth Sci. 42 (7), 1057–1068. (in Chinese with English abstract). doi:10.3799/dqkx.2017.085
Zhang, K., Jia, C., Song, Y., Jiang, S., Jiang, Z., Wen, M., et al. (2020). Analysis of Lower Cambrian Shale Gas Composition, Source and Accumulation Pattern in Different Tectonic Backgrounds: A Case Study of Weiyuan Block in the Upper Yangtze Region and Xiuwu Basin in the Lower Yangtze Region. Fuel 263, 1–15. doi:10.1016/j.fuel.2019.115978
Zhao, J., Jin, Z., Jin, Z., Hu, Q., Hu, Z., Du, W., et al. (2017). Mineral Types and Organic Matters of the Ordovician-Silurian Wufeng and Longmaxi Shale in the Sichuan Basin, China: Implications for Pore Systems, Diagenetic Pathways, and Reservoir Quality in fine-grained Sedimentary Rocks. Mar. Pet. Geology 86, 655–674. doi:10.1016/j.marpetgeo.2017.06.031
Zhao, W., Li, J., Yang, T., Wang, S., and Huang, J. (2016). Geological Difference and its Significance of marine Shale Gases in South China. Pet. Exploration Development 43 (4), 547–559. doi:10.1016/s1876-3804(16)30065-9
Zheng, N., Li, T., and Cheng, M. (2019). Middle-Upper Ordovician Radiolarians in Hunan and Jiangxi Provinces, South China: Implications for the Sedimentary Environment and Nature of the Nanhua basin. J. Asian Earth Sci. 179, 261–275. doi:10.1016/j.jseaes.2019.05.001
Zhou, S., Yan, G., Xue, H., Guo, W., and Li, X. (2016). 2D and 3D Nanopore Characterization of Gas Shale in Longmaxi Formation Based on FIB-SEM. Mar. Pet. Geology 73, 174–180. doi:10.1016/j.marpetgeo.2016.02.033
Zhu, H., Ju, Y., Huang, C., Han, K., Qi, Y., Shi, M., et al. (2019). Pore Structure Variations across Structural Deformation of Silurian Longmaxi Shale: An Example from the Chuandong Thrust-fold Belt. Fuel 241, 914–932. doi:10.1016/j.fuel.2018.12.108
Keywords: marine shale, OM pores, thermal evolution, evolution time, tectonic subsidence, tectonic uplift
Citation: Li X, Wang P, Wang S, Jin C, Zhang C, Zhao R and Jiang Z (2022) Influence of the Duration of Tectonic Evolution on Organic Matter Pore Structure and Gas Enrichment in Marine Shale: A Case Study of the Lower Silurian Longmaxi Shale in Southern China. Front. Earth Sci. 9:787697. doi: 10.3389/feart.2021.787697
Received: 01 October 2021; Accepted: 30 November 2021;
Published: 24 January 2022.
Edited by:
Kun Zhang, Southwest Petroleum University, ChinaReviewed by:
Bo Liu, Northeast Petroleum University, ChinaCopyright © 2022 Li, Wang, Wang, Jin, Zhang, Zhao and Jiang. This is an open-access article distributed under the terms of the Creative Commons Attribution License (CC BY). The use, distribution or reproduction in other forums is permitted, provided the original author(s) and the copyright owner(s) are credited and that the original publication in this journal is cited, in accordance with accepted academic practice. No use, distribution or reproduction is permitted which does not comply with these terms.
*Correspondence: Pengfei Wang, d3BmdXBjNzI1QG91dGxvb2suY29t; Chen Zhang, emhhbmdjaGVuY3VwQDE2My5jb20=
Disclaimer: All claims expressed in this article are solely those of the authors and do not necessarily represent those of their affiliated organizations, or those of the publisher, the editors and the reviewers. Any product that may be evaluated in this article or claim that may be made by its manufacturer is not guaranteed or endorsed by the publisher.
Research integrity at Frontiers
Learn more about the work of our research integrity team to safeguard the quality of each article we publish.