- 1Istituto Nazionale di Geofisica e Vulcanologia, Catania, Italy
- 2Institut de Physique du Globe de Paris, Paris, France
The structure of an active volcano is highly dependent on the interplay between the geodynamic context, the tectonic assessment as well as the magmatic processes in the plumbing system. This complex scenario, widely explored at Etna during the last 40 years, is nevertheless incomplete for the recent historical activity. In 1763 two eruptions occurred along the west flank of the volcano. There, an eruption started on 6th February and formed the scoria cone of Mt. Nuovo and a roughly 4-km-long lava flow field. Another small scoria cone, known as Mt. Mezza Luna, is not dated in historical sources. It is located just 1 km eastward of Mt. Nuovo and produced a 700 m long flow field. We focused on the activity of Mts. Nuovo and Mezza Luna for several reasons. First, the old geological maps and volcanological catalogues indicate that Mt. Mezza Luna and Mt. Nuovo cones were formed during the same eruption, while historical sources described Mt. Nuovo’s activity as producing a single scoria cone and do not give information about the formation of Mt. Mezza Luna. Second, petrologic studies highlight that the products of Mt. Mezza Luna are similar to the sub-aphyric Etna basalts; they preserve a composition relatively close to Etna primitive magma which were also erupted in 1763, during La Montagnola flank eruption, which took place along the South Rift of the volcano. Third, the two scoria cones built up along the so-called West Rift of Etna, which represents one of the main magma-ascent zones of the volcano. We applied a multidisciplinary approach that could prove useful for other volcanoes whose past activity is still to be reconstructed. Critical reviews of historical records, new field surveys, petrochemical analyses and petrologic modelling of the Mts. Nuovo and Mezza Luna eruptions have been integrated with literature data. The results allowed improving the stratigraphic record of historical eruptions reported in the Mount Etna Geological map, modelling the sub-volcanic magmatic processes responsible for magma differentiation, and evidencing recurrent mechanisms of magma transfer at Etna. Indeed, the intrusion of a deep primitive magma along the South Rift is often associated with the activation of other rift zones that erupt residual magma stored in the shallow plumbing system.
1 Introduction
The structure of Etna is closely determined by the interplay of processes involving magma dynamics as well as the geodynamic and volcano-tectonic setting of the area, which together influence the distribution of magma storage zones, the geometry of ascending magma paths and finally the eruptive style of volcanic activity (Condomines et al., 1995; Corsaro and Pompilio, 2004; Tanguy et al., 1997; Branca and Ferrara, 2013; Barreca et al., 2018, 2020).
This complex scenario has been widely explored from the 1980s onwards, as the development of Etna’s instrumental monitoring networks has led to an exponential increase of data available to study and better understand the recent eruptions. Therefore, during the last 40 years, systematic studies including seismicity, gas geochemistry, and ground deformation from the monitoring networks, associated with geological, volcanological and petrologic investigations, have delved deeply into Etna’s volcanic system. However, studies concerning the evolution of the volcano system in historic times, i.e. since the Greek colonization of Sicily (734 BC), are scarce. To fill this gap, it is crucial to reconstruct the complete chronology of Etna’s eruptions in historical times. Therefore, after the first geological map of Etna at 1:50.000 scale by Sartorius Von Waltershausen (1845), the studies of the scientific community continued for more than two centuries, and finally produced a comprehensive assessment of Etna historical eruptions thanks to an interdisciplinary approach. This comprises stratigraphy, historiographical studies and the radiometric, paleomagnetic and archeomagnetic datings acquired for the recent geological map of Mt. Etna volcano (Branca et al., 2011a; Tanguy et al., 2012; Branca et al., 2015a, 2019; Branca and Abate, 2019; Magli et al., 2021). However, even if numerous accounts are available for the volcanic activity of Etna during the last 400 years (Branca and Del Carlo 2005; Tanguy et al., 2007), some eruptions are still not cited in the historical sources (Branca and Vigliotti, 2015). Furthermore, the investigations of eruptive activity in recent historical times are rare and unsystematic (Tanguy, 1981), in particular since the 17th century.
In this paper, we focus on the volcanic activity that occurred in 1763 at Etna, for several interesting reasons. Firstly, from 5 February to 15 March 1763, an eruption occurred on the western flank of the volcano, producing a scoria cone called Mt. Nuovo. It was a typical Etna flank eruption (Branca and Del Carlo, 2005), i.e. driven by the radial drainage of laterally propagating magma from the central conduits, through eruptive fissures which cut the volcano’s slopes (see Armienti et al., 2004 for a review) and largely following the main volcano-tectonic structures of the edifice (Azzaro et al., 2012). Another small scoria cone of unknown age, named Mt. Mezza Luna, formed about 1100 m upslope, east of Mt. Nuovo. Concerning the timeline of Mt. Nuovo and Mt. Mezza Luna, an apparent contradiction seems important to solve. Indeed, the geological maps (Sartorius Von Waltershausen., 1845; Romano et al., 1979, Bellotti et al., 2010; Branca et al., 2011a) and volcanological catalogues (Sartorius Von Waltershausen, 1880; Romano and Sturiale, 1982; Behncke et al., 2005; Branca and Del Carlo, 2005) attribute Mt. Mezza Luna to the Mt. Nuovo eruption. On the contrary, the contemporaneous historical sources (Recupero 1815; Ferrara 1818) described an eruption that only formed the scoria cone of Mt. Nuovo, without mentioning Mt. Mezza Luna, a fact already pointed out by Tanguy (1980, 1981).
Secondly, petrologic studies of Mt. Nuovo and Mt. Mezza Luna are important to investigate the pre-eruptive magmatic processes of Etna plumbing system during past centuries, to be compared with the current ones. We also included in our study the well-documented La Montagnola eruption (Sturiale, 1970; Miraglia, 2002, Corsaro et al., 2009), which took place from 18 June to 10 September 1763 along the upper volcano’s south flank. The distinctive character of this eruption is that it emitted products with petrochemical features typical of “eccentric eruptions” (Rittmann 1965; Tanguy 1974, 1980; Armienti et al., 1988), also known as “deep lateral” (Tanguy and Kieffer, 1977), “peripheral” (Acocella and Neri, 2003) and more recently as “Deep Dyke Fed (DDF)” (Corsaro et al., 2009). Regardless of the naming, these particular type of flank eruptions are crucial to study because they emit a nearly aphyric basaltic-trachybasaltic magma that rapidly rises from a deep portion of the volcano’s plumbing system (about 10–12 b.s.l.), without interacting with melts stored in the shallow region. They therefore preserve a composition relatively close to Etna primitive magma and may play a crucial role in exploring the scarcely known deep portion of the volcano’s plumbing system. The output of DDF magma is quite rare at Etna and, besides La Montagnola eruption, it occurred in historical times during the eruptions of 1974 (Bottari et al., 1975; Tanguy and Kieffer, 1977), 2001 (Clocchiatti et al., 2004; Métrich et al., 2004; Corsaro et al., 2007) and 2002–2003 (Andronico et al., 2005; Spilliaert et al., 2006). In this framework, the evidence that also Mt. Mezza Luna products show petrochemical features attributable to DDF magma (Tanguy, 1980; Armienti et al., 1988) sounds promising to explore in greater detail.
Finally, another stimulating aspect in studying the 1763 eruptions lies in their relationship with the volcano-tectonic features of the volcano that follow a few dominant directions which are known as deep-seated “rift zones”, and represent preferential paths for magma ascent (Kieffer, 1975; Bousquet and Lanzafame, 2004 and references therein, Azzaro et al., 2012) (Figure 1). The main rift zones of Etna volcano are formed by different fissures and cones clustering along three main trends, i.e. the North-East, West and South (Azzaro et al., 2012). The distribution of flank eruptions during the past 2500 years indicates that the S rift is the most active magma-intrusion zone of Etna also in the long-term, since 51% of the events occurred there (Branca and Abate, 2019). The W rift (Bellotti et al., 2010; Azzaro et al., 2012) is an eruptive fissure system representing the second most active zone of magma-intrusion of Etna volcano in the last 2500 years (Branca and Abate, 2019). In this complex scenario, it could be interesting to speculate about the possible causes triggering the shift of volcanic activity from the W (Mt. Nuovo and Mt. Mezza Luna) to the S (La Montagnola) rift zones, as well as to explore the role of the rift zones as preferential paths of deep magma ascent at Etna.
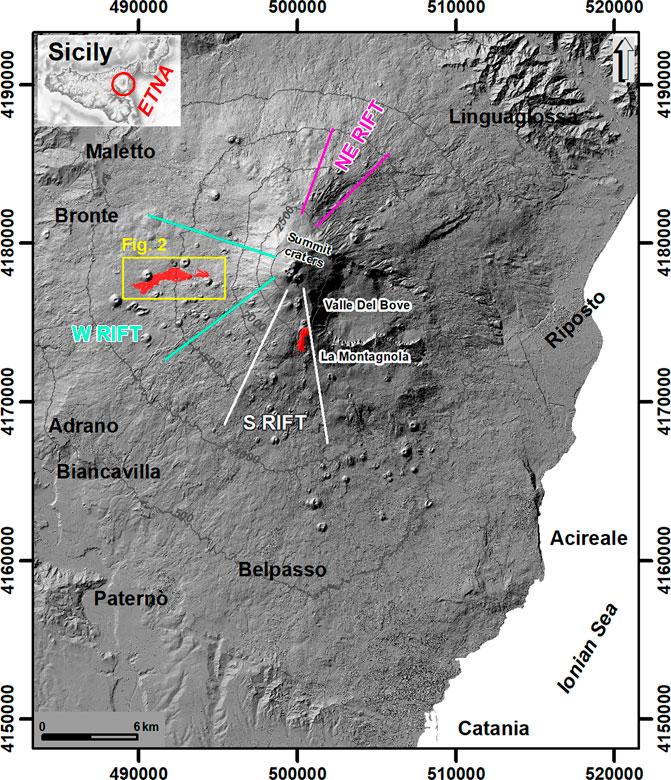
FIGURE 1. Shaded relief of Etna re-sampled to 10 m resolution (Gwinner et al., 2006); studied area is localized in the west flank of the volcano (yellow rectangle zoomed-in Figure 2); in the south flank, the lava flow of La Montagnola is shown in red. Colored lines delimit the S, W, and NE rifts. Inset represents the shaded relief of Sicily obtained from the TINITALY DEM re-sampled to 100 m resolution available at: http://tinitaly.pi.ingv.it (Tarquini et al., 2007), red circle indicates Etna position.
In this paper, we present the results of a multidisciplinary study integrating historical analyses, field surveys, petrochemical analyses and petrologic modelling. This approach has allowed us to propose a new reconstruction of: 1) the eruptive events that occurred in 1763 on the west and south flanks of Etna; 2) the temporal evolution of magmatic processes occurring within the volcano’s plumbing system; 3) the interaction between magma dynamics in the plumbing system and tectonic structures of the volcano.
2 Geological and Structural Setting of the Study Area
The studied area is located on the west flank of Etna where the oldest volcanics consist of thin tholeiitic lava flows lying on the sedimentary basement along the left bank of the Simeto River (Tanguy, 1978; Bellotti et al., 2010; Branca et al., 2011a). These lava flows, dated about 330 ka (De Beni et al., 2011), represent the earliest subaerial volcanic products of the Etna region (Basal Tholeiitic phase of Branca et al., 2008; Branca et al., 2011a; Branca et al., 2011b). Later, effusive activity produced small lava flows from scattered eruptive fissures that opened in the lower SW sector of Etna between about 178 and 136 ka (Timpe phase of Branca et al., 2008; Branca et al., 2011a; Branca et al., 2011b). The main volcanic cover of the west flank formed during the Stratovolcano phase (from 60 ka to the present). In particular, the lava flows of Ellittico volcano, about 40–30 ka old, reached the Simeto valley floor; later, during the activity of Mongibello volcano (from 15 ka to the present), several superposed compound lava flow fields and pyroclastic deposits formed the present geological setting of the volcano’s west flank (Bellotti et al., 2010). From a structural point of view, the west flank has a concentration of eruptive fissures and scoria cones developed down to about 1150 m of elevation, about 4.5 km wide, and aligned from 245° to 280° following WSW and W trends. This rift zone began to form during the early stage of Mongibello volcano activity, roughly starting from 15 ka (Bellotti et al., 2010; Azzaro et al., 2012). In greater detail concerning the last 2500 years, several historical eruptions occurred in this sector of the volcano, the most prominent of which built the Mt. Minardo and Mt. Ruvolo scoria cones during the Roman age, around 240 BC and 160 BC respectively (Branca et al., 2015a). These flank eruptions are typical for the intense explosive and effusive activity that produced large scoria cones and extended lava fields, which greatly modified the morphological setting of the volcano’s lower west flank. During the Medieval age, several flank eruptions occurred in this sector of the volcano approximately in 1060 AD, 1150 AD and 1200 AD, and formed the scoria cones named Mt. Gallo, Mt. Arso and Mt. Forno respectively (for details see Branca and Abate, 2019). Starting from the 17th century, many other significant flank eruptions took place in this area in 1610, 1651-54, 1763, 1832, 1843. Some of them caused damage to the cultivated areas and urban fabric, as well as threatened the towns of Adrano in 1610 and partially destroyed Bronte in 1651-54. The last lateral eruption along the W rift occurred in 1974, when an eruptive fissure formed two scoria cones at about 1650 m a.s.l., named Mts. De Fiore I and II.
3 Materials and Methods
The lava flow volume was calculated using the 2005 Digital Terrain Model (DTM, Gwinner et al., 2006) with the planimetric approach (area × average thickness, Stevens et al., 1999). Considering that lava flow thickness is topography influenced, we divided the lava field into four areas of homogenous thickness corresponding to four sectors of homogenous slope. Four cross-sections have been drawn along the lava field, whose average thickness has been evaluated by interpolating the basal surface of lava flow field (Figure 2).
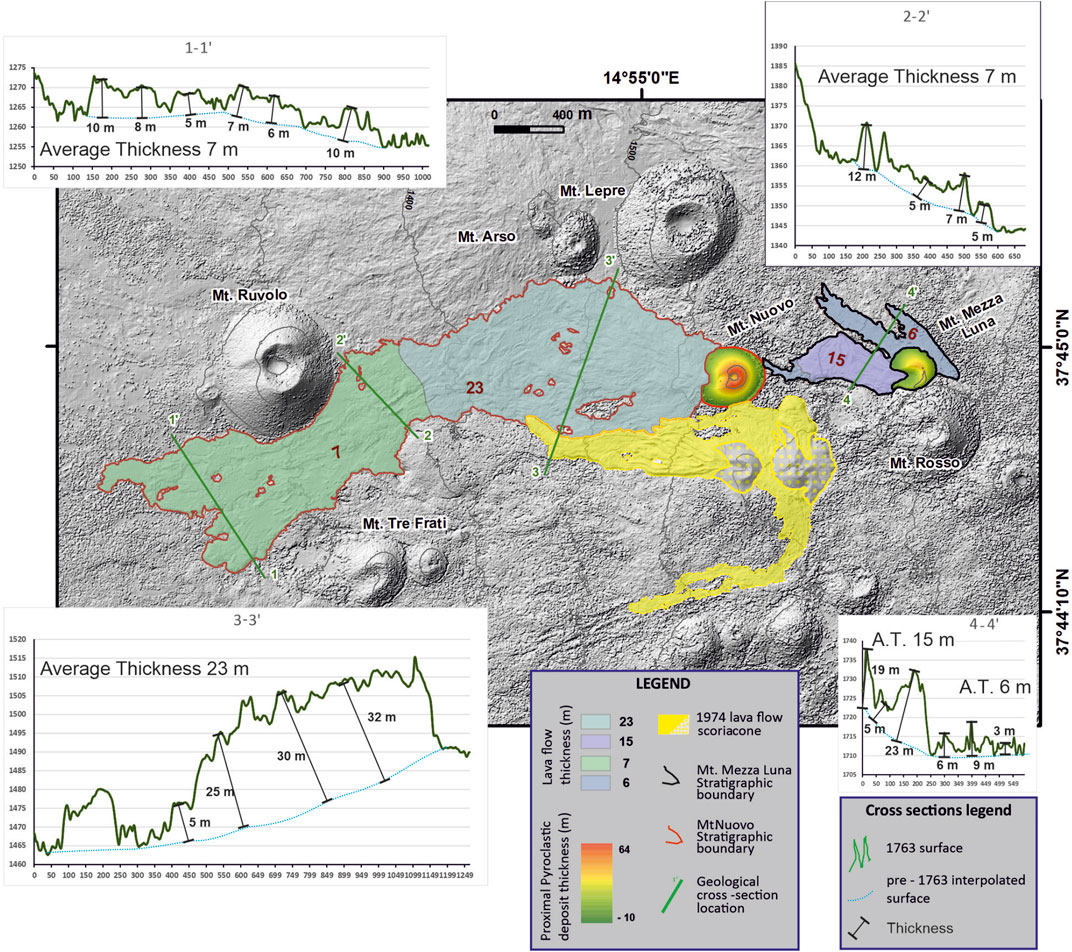
FIGURE 2. Geological map of Mt. Nuovo and Mt. Mezza Luna. Four sectors of homogenous thickness evaluated interpolating the basal surface are shown in different colours (see the text for details). The green line indicates the location of the geological cross sections. Mts. De Fiore (1974 eruption) lava flows field is plotted for comparison. A.T. = Average thickness. In Section 4– 4’ there are two different values of average thickness due to the major difference between the two lava flows branches in term of thickness.
The volume of Mt. Nuovo and Mt. Mezza Luna scoria cones has been calculated by subtracting the pre and post eruption surfaces (DTM). In particular, the pre-eruption surface has been drawn starting from a shapefile of points with a buffer of 5 m outside the scoria cones boundary. The ArcGIS Natural Neighbor tool allowed tracing a smooth surface without peaks, pits, or valleys that were not already present in the input dataset; we consider it as the best tool to use given the mild topography characterizing the area of interest. The interpolated pre-eruption surfaces have been subtracted from the post-eruption surfaces, obtaining the scoria cones thickness and subsequently, the volume has been calculated with the Cut and Fill ArcGIS tools (De Beni et al., 2019).
The paleomagnetic age of Mt. Mezza Luna cinder cone has been determined in a dozens of sun-oriented paleo-magnetic large samples weighing 0.5–1 kg. The magnetic remanence has been measured with a special rotative inductometer (Thellier, 1981) at the St. Maur laboratory (France), managed by the Institut de Physique du Globe de Paris. Details of the method and the precision and accuracy of measurements can be found in Tanguy (1980) and Tanguy et al. (2003, 2007 and references therein).
Major and trace elements of bulk rocks (Table 1) have been determined at Centre de Recherches Petrographiques et Geochimiques (SARM) in Nancy (France) using respectively Inductively Coupled Plasma Optical Emission Spectroscopy (ICP-OES) and Inductively Coupled Plasma Mass Spectrometry (ICP-MS) (Carignan et al., 2001). The analytical uncertainty (1σ) is <1% for SiO2 and Al2O3, <2% for Fe2O3, MgO, CaO, Na2O, K2O, <5% for MnO and TiO2, 5–10% for P2O5, and <5% for all trace elements except U (<8%).
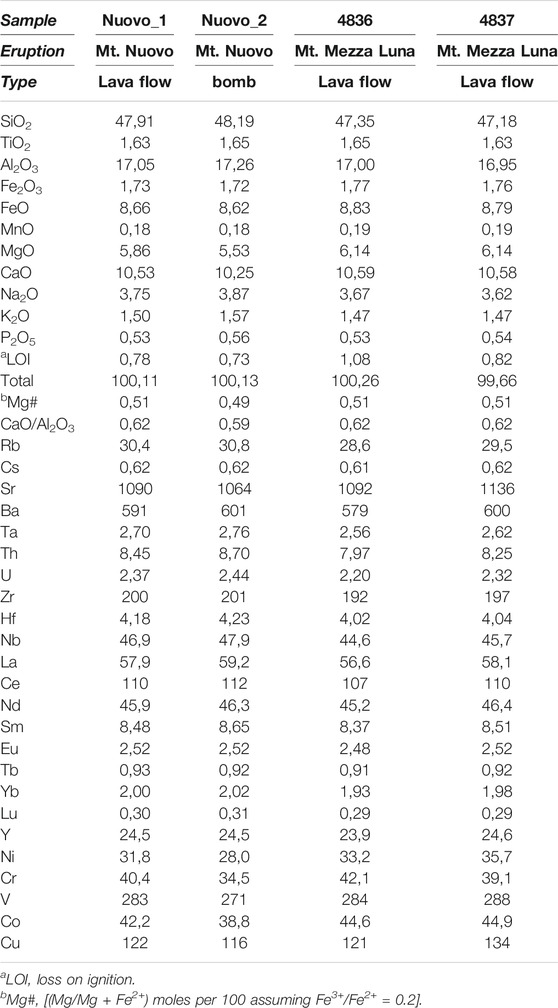
TABLE 1. Major and trace elements composition of the lavas/pyroclasts erupted during the Mt. Nuovo and Mt. Mezza Luna eruptions.
4 Results
4.1 The History of the 18th Century Eruptions
The canon Giuseppe Recupero (1720–1778) was a keen observer of Etna during the 18th century. However, he did not publish his numerous detailed observations, which were collected much later by his nephew Agatino. The final publication of two large volumes (Recupero, 1815) represents a benchmark surpassed only by the German geologist Wolfgang Sartorius Von Waltershausen, who compiled the first geological and topographic maps of Mount Etna and collected all the available documents on the history of eruptions (Sartorius Von Waltershausen, 1880; for details see Branca and Abate, 2019).
Following the destructive 1669 eruption and 1693 tectonic earthquakes (Tanguy, 1981; Branca and Del Carlo, 2005; Branca et al., 2015b), a “pre-scientific” era began in the 18th century with a detailed description of all the observed phenomena, mainly thanks to Recupero. After two further flank eruptions in 1689 and 1702, a rather unusually long period of summit activity lasted until 1755, with almost continuous explosions within the Central Crater and various lava overflows westward (1723, 1732) and eastward (1736, 1747). This activity lasted until 2 March 1755 when a copious lava emission fed a rapid lava flow which reached Mt. Lepre in the Valle del Bove, about 3 km east of the Central Crater, melting a large amount of snow and triggering a lahar which devastated the eastern flank of the volcano. This was probably the beginning of a flank fissure, which emitted several lava flows between 10 and 15 March 1755. The Central Crater, however, apparently continued its discontinuous explosive activity, namely in 1758 and 1759 when several lava flows overran its rims towards the southeast, east and northwest (see Tanguy, 1981, p. 617). All these lava flows, including those emitted by the 1755 flank eruption, are buried and inaccessible to sampling today.
In 1763 (5 February to 15 March), a new eruption occurred on the western flank of Etna, producing a lava flow and a scoria cone called Mt. Nuovo. Another recent smaller scoria cone, which produced a small scoria cone, is located about 1 km upslope; it is half-open and for this reason called “Mezza Luna” (half-moon) (Figure 3).
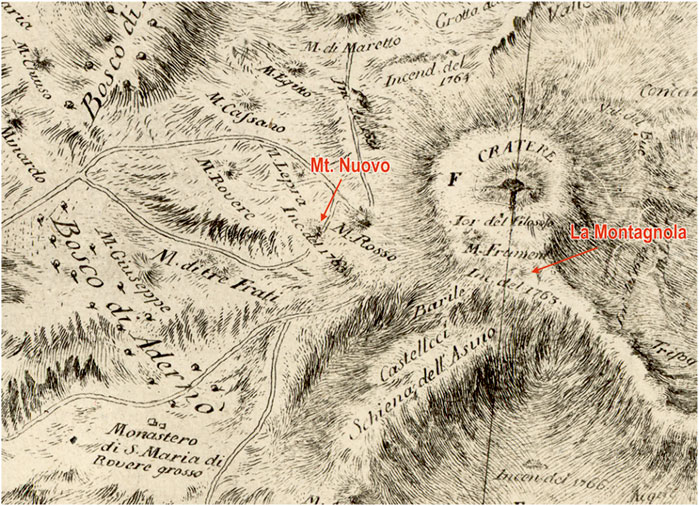
FIGURE 3. Detail of the oryctographic Map of Etna, from Recupero (1815). The 1763 eruption of Mt. Nuovo is located in the area of Mt. Rosso and Mt. Lepre in the west flank of the volcano; the 1763 eruption of La Montagnola is drawn in the upper south flank of the volcano.
The catalogue of historical eruptions compiled by Arnold von Lasaulx (in Sartorius Von Waltershusen, 1880), for the first time, ascribed Mt. Mezza Luna volcanic products to the Mt. Nuovo 1763 eruption. The author, by interpreting the chronicles of Giuseppe Recupero, suggested the presence of two scoria cones. In particular, the lower cone (Mt. Nuovo) located between Mt. Rosso and Mt. Lepre, and the upper cone (Mt. Mezza Luna) close to the northwest slope of Mt. Rosso. According to Lasaulx, the upper cone was formed first, at the onset of the eruption, on 5 February. Conversely, Giuseppe Recupero, who visited the eruptive fissure at first hand, on 27 and 28 February 1763, described an explosive vent around which just one conical scoria cone was growing and, a few steps eastward, he observed another explosive vent, whose base was joined to the main cone (Recupero, 1815, 2, p. 114-121). At the end of the eruption, Recupero clearly described one “new volcano” (Nuovo volcano) whose circular base had occluded and incorporated the eastern explosive vent that he had observed on 28 February.
During the summer of 1763 (18 June–10 September), Recupero (1815) observed and described another flank eruption that formed the large scoria cone named “La Montagnola”, at 2500 m a.s.l. on the south flank, and a 3.5 km-long lava flow. The eruption was characterised by intense and long-lasting explosive activity that produced abundant lapilli and ash fall mainly on the volcano’s southeast flank, causing severe damage to the cultivated areas. The daily observations of the eruption were highly detailed. In particular, Recupero measured for the first time at Etna the weight of tephra per unit area and reported 4 kg of ash per square metre, which had fallen over 7 days at Catania (Branca and Del Carlo, 2005). He also measured a maximum height of 1500–2000 m for the lava fountains, by recording a downfall time of pyroclasts until 21 s (Tanguy, 1981).
After the Montagnola eruption, Etna was characterised by frequent flank fissures in 1766, 1780, 1792, 1802, 1811, 1809, etc., namely a more or less persistent activity with frequent pulses and outpouring of large lava flows (Branca and Del Carlo, 2005; Tanguy et al., 2007). This kind of activity continued until the end of the 20th century when a further increase of the magma output led to sustained summit activity and more frequent flank eruptions (e.g. Condomines et al., 1995; Clocchiatti et al., 2004).
4.2 Mt. Nuovo and Mt. Mezza Luna: Volumes, Stratigraphic Relationship and Archeomagnetic Age
We carried out a new detailed geological survey of the study area (Figure 2), focused on investigating the stratigraphic relationship between Mt. Nuovo and Mt. Mezza Luna products and defining the volcanological parameters. Mt. Nuovo, located at 1600 m a.s.l., formed owing to a long-lasting Strombolian activity building up a scoria cone about 65 m high with a basal diameter of 330 m and a volume of 2.1 × 106 m3. At the west base of the cone is located the effusive vent that produced a 3.5 km-long compound lava field reaching an elevation of 1180 m a.s.l., with an area of 2.0 × 106 m3 and a volume reaching approximately 32 × 106 m3.
Mt. Mezza Luna is located at 1760 m a.s.l. about 1 km east of Mt. Nuovo. It is a scoria cone about 30 m high with a basal diameter of 230 m and a volume of 0.5 × 106 m3, which emitted a small single lava flow field, 800 m-long with an area 0.2 × 106 m3 and a volume reaching approximately 2.6 × 106 m3.
In particular, field evidence shows that the frontal portion of the lava flow emitted from Mt. Mezza Luna covers Mt. Nuovo scoria cone, indicating that Mt. Mezza Luna followed Mt. Nuovo, as Tanguy (1981) previously described.
To further constrain the stratigraphic position of Mt. Mezza Luna, we undertook an archeomagnetic sampling of the lava flow, according to the procedure explained in Tanguy (1980) and Tanguy et al. (2003, 2007) and corrected for local disturbances of the Earth’s magnetic field, which are strong on the west flank of Etna (Tanguy and LeGoff, 2004). The result (Figure 4) clearly indicates that the age of Mt. Mezza Luna is close to 1760 AD (±40 years) and partly overlaps the measure of the 1763 La Montagnola eruption. Therefore, Mt. Mezza Luna certainly formed in the second half of the 18th century. Unfortunately, the analytical uncertainty of the measurements prevents establishing if it formed before or after La Montagnola eruption, even if a very close date seems reasonable.
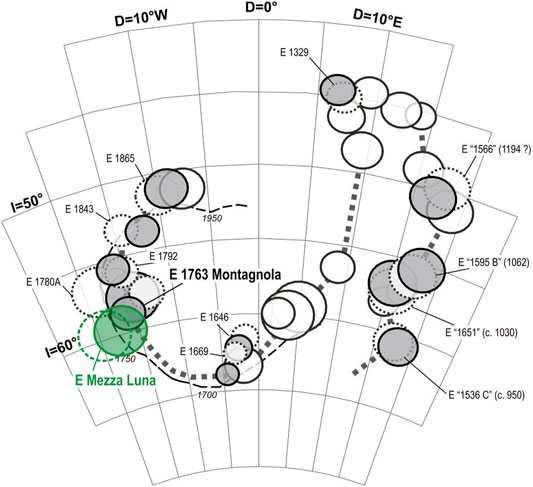
FIGURE 4. Position of Mt, Mezza Luna in the archeomagnetic curve of Etna historical lavas (modified from Le Goff and Tanguy, 2004). The Alpha 95 confidence circles of the paleomagnetic directions before (dashed circles) and after correction for local disturbances of the Earth magnetic field (shadowed circles) are reported for the various sites. Mt. Mezza Luna flow (shadowed green circle) partly overlaps the 1763 eruption of La Montagnola.
4.3 Petrochemistry
We focused on the petrochemical features of Mt. Nuovo and Mt. Mezza Luna products derived from this study, integrated with literature data of La Montagnola eruption (Corsaro et al., 2009).
Petrography and modal mineralogy of Mt. Nuovo and Mt. Mezza Luna are different. Indeed, Mt. Nuovo contains from 15 to 25% vol. phenocrysts of prevalent plagioclase, clinopyroxene and olivine; on the other hand, Mt. Mezza Luna is scarcely porphyritic, has 5–8% phenocrysts of olivine and clinopyroxene and rare plagioclase (1–2% vol), and is fairly similar to sub-aphyric products of 1763 La Montagnola (Tanguy, 1980; Armienti et al., 1988). The groundmass texture of all the samples varies from glassy to cryptocrystalline, depending on the cooling history.
Mts. Nuovo and Mezza Luna products are hawaiites, while La Montagnola lavas are more primitive basalts (Le Maitre, 2002). All of them belong to a Na-alkalic series just as the volcanic rocks erupted in historical time up to the 1970’s, when a clear shift toward a K-alkalic suite has occurred and persists until now (Tanguy, 1980; Condomines et al., 1995; Tanguy et al., 1997).
The products of Mts. Nuovo and Mezza Luna display a low variability of major elements (Figure 5A) and most trace elements (Figures 5B–D), sometimes at the limit of analytical uncertainty. In particular, Mt. Nuovo is slightly more evolved than Mt. Mezza Luna; a compositional gap separates the hawaiites of Mts. Nuovo and Mezza Luna from La Montagnola basalts, which are the most primitive analysed products of 1763.
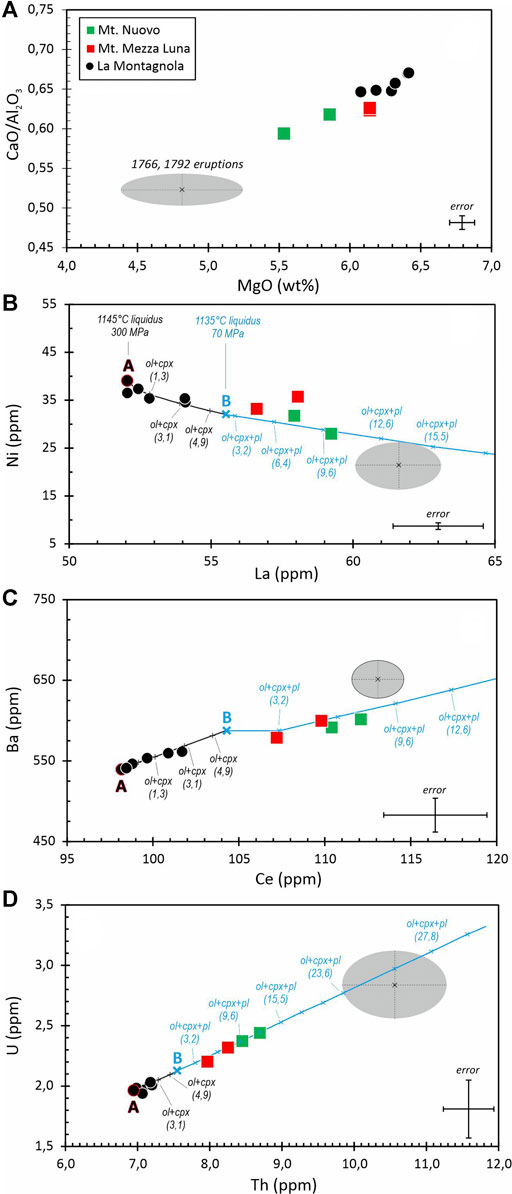
FIGURE 5. Variations of MgO versus CaO/Al2O3 (A), La versus Ni (B), Ce versus Ba (C) and Th versus U (D) in the volcanic products of Mt. Mezza Luna and Mt. Nuovo. Data of La Montagnola eruption are from Corsaro et al. (2009). The liquid evolution lines modelled starting from compositions “A” (at 300 MPa, black line) and “B” (at 75 MPa, blue line) are drawn (see the text for details). Ticks mark the cumulative volume percentage of the minerals that formed during the fractional crystallization process. The products of the 1766 (Viccaro and Cristofolini, 2008) and 1792 (Corsaro and Cristofolini 1996) eruptions are plotted for comparison; the grey area represents the variability of the analyses expresses as the average ± 1 sigma.
A first qualitative evaluation of the compositional patterns shows that the fractional crystallization of the most common minerals (plagioclase, clinopyroxene and olivine) present in the historic Etna lavas could be the main process of magma differentiation during 1763. Indeed, the decreasing patterns of CaO/Al2O3 and MgO from the most primitive sample of La Montagnola (MgO = 6.41%, CaO/Al2O3 = 0.67) to the most evolved of Mt. Nuovo (MgO = 5,53%, CaO/Al2O3 = 0.59) match well with the fractional crystallization of clinopyroxene, plagioclase and olivine. Also the more evolved compositions of 1766 (Viccaro and Cristofolini, 2008) and 1792 products (Corsaro and Cristofolini, 1996) plot along the same trend (Figures 5A–C), suggesting that fractional crystallization is the main process controlling magma evolution throughout the 18th century.
The liquid evolution line of a fractional crystallization process in isobaric conditions has been calculated with the alphaMelts code (Ghiorso et al., 1995; Smith and Asimov, 2005). Although several authors (Tanguy, 1980; Condomines et al., 1995; Tanguy et al., 1997) have hypothesized a large magma reservoir near 30 km depth, based on presence of orthopyroxene relics within the 2001–2002 alkaline lavas (Clocchiatti et al., 2004), we consider here shallower environments, as described in the following. The most primitive sample of La Montagnola eruption (i.e. LYMC8 in Corsaro et al., 2009; indicated as point A in Figures 5B–D) represents the liquid starting composition. Pressure is assumed = 300 MPa, i.e. the highest entrapment pressure of olivine hosted melt inclusions in La Montagnola scoria (see Table 3 and Figure 10 in Corsaro et al., 2009). Accordingly, LYMC8 composition has been recalculated assuming that H2O = 3.0%, in agreement with the water content measured in olivine hosted melt inclusions at 300 MPa (see Table 3 in Corsaro et al., 2009). The simulation is bracketed at temperatures from the liquidus to 1060° (at step 5°), at NNO buffer (see Table 3 in Corsaro et al., 2009). The partition coefficients used for trace element calculations are specific for Etna rocks (D'Orazio et al., 1998). The liquid evolution line resulting from the crystallization of about 1% vol. olivine and 3% vol. clinopyroxene reproduces fairly well both the trace elements distribution and the type/abundance of phenocrysts observed in La Montagnola lavas. However, the model does not allow the crystallization of plagioclase, and therefore does not work satisfactorily to reproduce Mt. Mezza Luna and Mt. Nuovo lavas where, on the contrary, this mineral is present. We have therefore hypothesized that the residual liquid of La Montagnola magma fractional crystallization rises at shallower depths, where open system degassing with lower water contents may enhance the plagioclase crystallization (Spilliaert et al., 2006). The environmental conditions of the new simulation, as follows: the starting composition is the average of the liquids at 1124°C and 1119°C (point B in Figures 5B–D), recalculated assuming that H2O = 1%, according to the water content of olivine hosted melt inclusions in La Montagnola lavas at P < 100 MPa (see Figure 10 in Corsaro et al., 2009). P is fixed at 70 MPa, in agreement with the depth of the shallower storage zone proposed for Etna by Corsaro and Pompilio (2004); temperature ranges from the liquidus to 1060° (at step of 5°), at QFM buffer, due to the more oxidized conditions of the shallow plumbing system.
The new simulation shows that Mt. Mezza Luna and Mt. Nuovo mainly plot along the liquid evolution line produced by the crystallization of about 1% vol. olivine, 4–5% vol. clinopyroxene and 5–6% vol. plagioclase, from 1119°C to 1114°C, in agreement with the more evolved composition and mineral assemblage of Mt. Mezza Luna and Mt. Nuovo products. It is noteworthy that also the magma emitted during the following eruptions of 1766 and 1792 (Figure 5) seems linked to the same fractional crystallization process and reaches much more evolved compositions than Mt. Mezza Luna and Mt. Nuovo.
5 Discussion
The integration of multidisciplinary data concerning the 1763 eruptions at Etna has enabled shedding light on: 1) the evident mismatch existing between volcanological catalogues and historical sources, 2) the stratigraphic position of Mt. Mezza Luna and the chronology of the 1763 eruptions at Etna; 3) the geometry and magma dynamics of the plumbing system, as well as 4) the possible relationship between rift zones and volcanic activity.
5.1 The Timeline of the Mt. Nuovo, Mt. Mezza Luna and La Montagnola Eruptions
The volcanological catalogues attribute Mt. Mezza Luna to the Mt. Nuovo eruption. Instead, the historical sources of contemporaries’ report that the eruption only produced the scoria cone of Mt. Nuovo, without mentioning the presence of Mt. Mezza Luna and the related lava flow (Recupero, 1815; Ferrara, 1818). In particular, Recupero, who visited the ongoing eruption of Mt. Nuovo on 27 and 28 February 1763, did not record the presence of another active scoria cone, i.e. Mt. Mezza Luna, that he would have noticed since it was just 1 km far from Mt. Nuovo and presumably was rumbling due to the explosive activity. Recupero’s chronicles are generally very accurate, exactly as when he reported the amount of ash fallen in 7 days on the city of Catania during La Montagnola eruption (Branca and Del Carlo, 2005). Therefore, we are persuaded that Recupero does not mention Mt. Mezza Luna simply because it did not exist at the time of the Mt. Nuovo eruption. We think that Mt. Mezza Luna formed later and was not described by the contemporaries. There are other examples of flank eruptions that in the 19th century had not been documented, such as the so-called Dagala dell’Orso lava flow (Branca et al., 2011a; Tanguy et al., 2012) on the upper northwest slope of the volcano, and the 1918 eruption on the upper northwest slope, recognized later by De Fiore (1920). This circumstance might appear strange today because terrestrial and spatial networks allow a real-time monitoring of Etna activity, but it was plausible in the past centuries, when an eruption was exclusively testified by visual observations. Therefore, it is not surprising that eruptions in scarcely populated areas and/or during bad weather conditions had not been recorded, especially if the eruptive activity was short-lived, as presumably Mt. Mezza Luna eruption was. Indeed, the low lava volume erupted (about 2.6 × 106 m3) is comparable with the volumes erupted during past eruptions that typically lasted just a few days (Table 2).

TABLE 2. Low-volume and short-lived historic eruptions extracted from the catalogues of Etna’s eruptions (Behncke et al., 2005; Branca and Del Carlo, 2005).
Overall, we conclude that the discrepancy between the historical sources and the following volcanological catalogues is due to Lasaulx, who attributed Mt. Mezza Luna to the eruption of Mt. Nuovo (in Sartorius, 1880). This interpretation conditioned the subsequent catalogues of historical eruptions of Etna published during the following centuries (Romano and Struriale 1982; Behncke et al., 2005; Branca and Del Carlo, 2005) and the associate geological maps.
The geological survey we carried out has further reinforced the conclusions from the analysis of the historical sources mentioned above. Indeed, the lava flow of Mt. Mezza Luna overlaps the base of the Mt. Nuovo scoria cone, proving that Mt. Mezza Luna activity followed the Mt. Nuovo eruption. To try to define the relationship between Mt. Mezza Luna and La Montagnola, we considered the archeomagnetic curve of Etna historical lavas (Tanguy and LeGoff, 2004). In Figure 4, Mt. Mezza Luna is dated 1760 (±40 years) but, due to the wide overlap of the confidence circles, it is not possible to determine if Mt. Mezza Luna precedes or not La Montagnola eruption. Instead, it can be reasonably assessed that Mt. Mezza Luna formed in the second half of the 18th century, probably very close in time to La Montagnola eruption.
In summary, historical sources and geological surveys indicate that the eruptive event of Mt. Mezza Luna is subsequent to Mt. Nuovo activity (5 February–15 March) and, taking into account archeomagnetic data, probably occurred in the second half of the 18th century. Unfortunately, we cannot establish the relationship between Mt. Mezza Luna and La Montagnola eruptions, but speculations on this unsolved aspect might be provided by petrologic and volcano-tectonic investigations, as discussed in the following sections.
5.2 Magma Dynamics During the 18th Century Eruptions
The petrologic modelling has highlighted that the compositional variability of Mts. Nuovo and Mt. Mezza Luna products matches the polibaric fractional crystallization of the parental La Montagnola magma. Specifically, we propose that a first step of magma differentiation takes place at about 300 MPa, (∼12 km b.s.l., calculated with the density of sedimentary rocks of Etna’s basement reported in Corsaro and Pompilio, 2004), i.e. at the same storage depth proposed for the DDF magma erupted during the 1974, 2001 and 2002-03 DDF flank eruptions (Corsaro et al., 2009 and references therein). In this environment, the most primitive la Montagnola magma evolves slightly for the scarce crystallization (about 4% vol.) of olivine and clinopyroxene. The second step takes place at a shallower level, estimated at about 70 MPa, (∼3 km b.s.l., according to Corsaro and Pompilio, 2004), where the residual liquid continues to cool and crystallize, forming also plagioclase, up to about 12% vol. of total minerals. This shallower environment is suitable to produce the compositional variability and the minerals observed in the lavas of Mt. Mezza Luna and of the slightly more evolved and plagioclase richer Mt. Nuovo.
Therefore, according to thermodynamic modelling, we hypothesize the following scenario that links pre-eruptive magmatic processes with the occurrence of the 1763 eruptions of Mts. Nuovo, Mezza Luna and La Montagnola. The volcanic activity of 1763 started with the eruption of Mt. Nuovo (5 February–15 March 15) that tapped the top of a shallow magma reservoir where the most evolved and plagioclase-rich magma of the study period was stored (Figure 6A). We hypothesize that the depressurization induced by the drainage of the erupted material probably enhanced the mobilization and ascent of a less crystallized and evolved magma from the bottom of the shallow plumbing system, which fed the Mt. Mezza Luna eruption (Figure 6B). Such a scenario entails the presence of a compositional zoned shallow magma reservoir that progressively drained and fed, first the activity of Mt. Nuovo, and later Mt. Mezza Luna. The temporal eruptive sequence inferred from petrologic modelling underpins the results of the field survey showing that Mt. Mezza Luna lavas overlap, and therefore follows, the scoria cone of Mt. Nuovo. It is an open question whether Mt. Nuovo and Mezza Luna formed during different eruptions or rather belong to distinct phases of the same eruption. This last circumstance is documented for the 1974 flank eruption that, interestingly, took place along the same W rift of Mts. Nuovo and Mezza Luna; it produced two scoria cones (Mts. De Fiore I and II) and lava fields in the course of two phases separated by an interval of 23 days. Taking into account some differences between the 1974 and Mts. Nuovo-Mezza Luna eruptions, we believe that Mt. Nuovo and Mt. Mezza Luna represent distinct eruptions. Indeed, the petrography and the composition of the 1974 magma remained fairly homogeneous during the two phases of activity (Corsaro et al., 2009). Also Lormand et al. (2020) have recently confirmed that an identical source magma from a deep region of the plumbing system (10 ± km b.s.l.) was emitted during the phases I and II of the 1974 eruption. On the contrary, Mts. Nuovo and Mezza Luna magmas show differences in the bulk rock composition and the abundance and proportion of the minerals that adhere more closely to the hypothesis that they formed during two separated eruptions, even if quite near in time, because the petrochemical differences are slight. Volcanological data also seems to sustain the hypothesis of distinct eruptions. Indeed, the calculated lava volume of Mt. Nuovo (32 × 106 m3) is at least one order of magnitude higher than Mt. Mezza Luna (2.6 × 106 m3). On the contrary, the lava volumes emitted during the two phases of the 1974 eruption are rather similar, i.e. 2.4 × 106 m3 for DFI and 2.1 × 106 m3 for DFII (Corsaro et al., 2009). In our opinion, this different behaviour is further evidence that Mt. Nuovo and Mt. Mezza Luna represent different eruptions rather than distinct phases of the same eruption. In the future, more detailed investigations, such as the textural studies of Lormand et al. (2020) who have investigated the degassing and cooling histories of the 1974 ascending magma, could contribute to uphold or discard our conclusion.
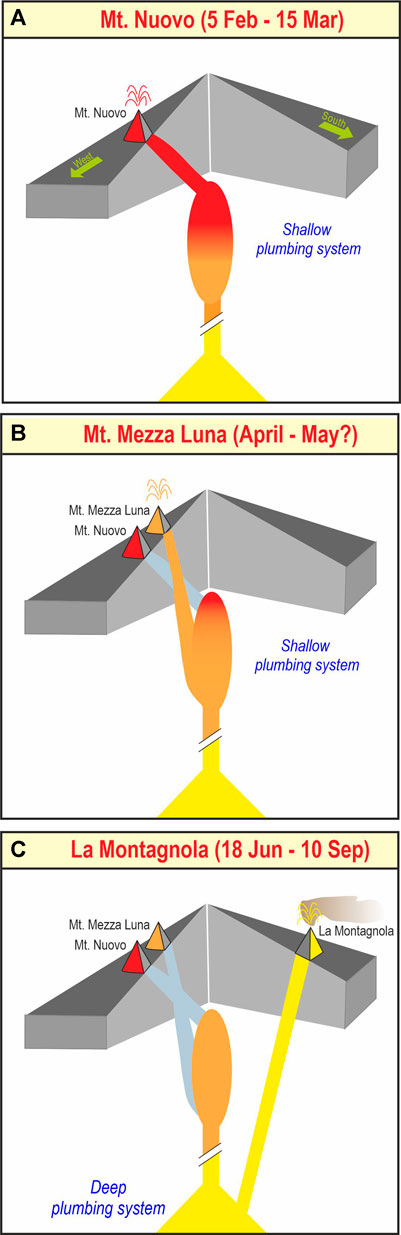
FIGURE 6. Cartoon illustrating magma dynamics of Etna plumbing system driving the eruptions of Mt. Nuovo (A), Mt. Mezza Luna (B) and La Montagnola (C) in 1763. See text for details.
Finally, the volcanic activity of the 18th century ended with La Montagnola eruption fed by a “Deep Dyke Fed” magma that ascended from the deep portion of the volcano’s plumbing system, without any interaction with magmatic melts stored in the shallow region (Figure 6C). This magma, which is the most primitive of the 18th century, is the parent of Mts. Nuovo and Mezza Luna melts via a polybaric fractional crystallization. It is worth noting that La Montagnola eruption represents a petrologic benchmark because, for the first time in historic times, it saw the emission of a magma directly ascending from the deep portion of the volcano’s plumbing system, which no longer occurred until the eruption of 1974.
5.3 Relationship of Magma Dynamics With the Rift Zones
The volcanic activity of the 18th century provides subject for thought on the possible relationship between pre-eruptive magma dynamics and the volcano tectonics, focusing on the role of the main rift zones.
It is interesting to note that the first two eruptions (Mt. Nuovo and Mezza Luna) of 1763 took place, close in time and space, along the W rift, while the last one (La Montagnola) shifted to the S rift and produced the most primitive and deep magma of the period. Branca and Del Carlo (2005) showed that during an eruption, the activation of eruptive fissures along multiple rift zones is possible in historical times. This circumstance occurred in 1832, when the first magma intrusion affected the southern base of the summit cone and later a new dyke intruded the W Rift. Also in 1971, a first phase of magma intrusion at the S base of the summit cone, was followed by a second phase generated by an ENE trending dyke, in 1985, when the eruptive fissures of the March-July activity involved the S rift, while in December they shifted to the east flank. Also two bi-radial eruptions are documented in historic times, so called because the magma was contemporaneously emitted by distinct rifts. They occurred in 1879 (S and NE rifts) and 2002-03 (S and NE rifts). A crucial question arising from the studied eruptions and the examples above is: what mechanisms/processes control the shift of an eruptive fissure system? This complex matter has been extensively studied in recent decades (Acocella, 2021; Bonaccorso et al., 2017; Galetto et al., 2021, and references therein) showing that the mechanisms controlling the propagation and geometry of a dyke are fundamental for magma ascent, transport, emplacement in the crust and final eruption. The geometry and the final extent of an ascending dyke are therefore governed by a number of factors such as changes in magma injection rate, structural barrier, topographic load and tectonic stress perturbation (Acocella, 2021; Bonaccorso et al., 2017; Galetto et al., 2021, and references therein). For old historical eruptions that do not have the data of the present-day monitoring networks, we may only hypothesize that one of the above circumstances led to the solidification and stop of the dyke feeding the Mt. Nuovo eruption, after the drainage of the magma stored at the top of the shallow reservoir (Figure 6A). The subsequent eruption of Mt. Mezza Luna was fed by a new dyke that found the easiest path to ascend along the weakened rocks of the W rift, very close to Mt. Nuovo (Figure 6B). Finally, a significant shift of the eruptive fissure system to the S rift, coupled with the emission of a DDF magma, occurred during La Montagnola eruption (Figure 6C).
At Etna, DDF magmas are rare (Armienti et al., 1988; Correale et al., 2014; Corsaro and Métrich, 2016) and in historical times they have been emitted only four times, three of which along eruptive fissures located right on the S rift zone (e.g. La Montagnola in 1763, 2001 and 2002-03 flank eruption). Furthermore, Branca and Del Carlo (2005) have proposed that that Class B eruptions, typical for intense long-lasting explosive activity and abundant tephra fallouts, have taken place along eruptive fissures located mainly on the southeastern flank in the last three centuries (1669, 1763 La Montagnola, 1886, 1892, 2001, 2002-03). The distribution of flank eruptions during the past 2500 years unquestionably indicates that the S rift is the most active magma-intrusion zone of Etna also in the long-term, since 51% of the events occurred there (Branca and Abate, 2009). Finally, also the regional compressive N-S stress field, as well as the confining pressure disequilibrium caused by the presence of the Valle del Bove depression in the east flank of the volcano might have facilitated the intrusion of a N-S dyke below the S rift zone. In conclusion, the study of the 1763 eruptions of Etna confirms that the S rift represents the preferential pathway of deep and volatile-rich magma ascent of the volcano.
The link between eruptive activity and the main structural lineaments of the volcano appears less binding for the W Rift. Apart from the 1974 eruption, we have no further evidence of the eruption of DDF magma along the W Rift; in older Greek–Roman epochs (734 BC–476 AD) several flank eruptions of class B occurred on the lower west flank of the volcano. Among them, the so-called Mt. Ruvolo and Mt. Minardo eruptions were characterized by intense explosive and effusive activity that produced large scoria cones and extensive lava fields that greatly modified the morphology of these sectors of the volcano (Branca et al., 2015a).
Finally, the NE Rift from which, to our knowledge, DDF magmas have never been erupted, probably largely intersects a portion of the volcano plumbing system that is too shallow to draw on the deep magma.
6 Conclusive Remarks
A multidisciplinary approach, including the critical re-reading of historical chronicles, new field work with sampling, the petrologic study of the volcanic products, as well as the integration of literature data, has proved an effective tool to investigate the past eruptions of an active volcano as Etna, and in particular the three eruptions occurring in 1763 (Mt. Nuovo, Mt. Mezza Luna and La Montagnola) for which several outstanding issues still remained.
We have demonstrated that discrepancies may exist between the historical chronicles and the subsequent catalogues of the eruptions. In this regard, Mt. Mezza Luna is not attributable to the eruption of Mt. Nuovo as sustained by Lasaulx (Sartorius Von Waltershausen, 1880), who was to erroneously influence the catalogues and geological map published in the following centuries (Romano and Sturiale, 1982; Behncke et al., 2005; Branca and Del Carlo, 2005). Indeed, Mt. Mezza Luna did not exist at the time of the Mt. Nuovo eruption, as the historical sources of the contemporaries affirm. On the contrary, Mt. Mezza Luna is a distinct eruption that follows the activity of Mt. Nuovo, and probably occurred in the second half of the 18th century. Mt. Mezza Luna eruption is not described by the contemporaries because it was short-lived and took place in a scarcely populated area of the volcano.
Petrologic modelling proved a useful tool to infer magma dynamics within the volcano’s plumbing system in 1763, allowing to constrain the temporal sequence of the 1763 eruptions. It started with Mt. Nuovo, followed with Mezza Luna and finished with the La Montagnola eruptions, during which a DDF magma ascending from a deep portion of the volcano’s plumbing system was erupted for the first time in historical times at Etna.
The reasons why in 1763 the eruptive activity shifts from the W rift (Mts. Nuovo and Mezza Luna eruptions) to the S Rift (La Montagnola eruption) remains the object of speculation. Indeed, mechanisms that control the propagation of a dyke are highly complex and difficult to constrain, especially for the old historical eruptions that were not monitored with the present-day instrumental networks. However, it can be confirmed that also in the 18th century, the S rift was a preferential pathway of magma ascent from the deep zone of the volcano’s plumbing system, as it has occurred during the flank eruptions of 2001 and 2002-03. In particular, Etna seems to show recurrent mechanisms of magma transfer activating multiple rifts. Indeed, the intrusion of a deep primitive magma along the S Rift is often associated with the activation of other rift zones (W Rift in 1763, NE Rift in 2001 and 2002-03), which produce the eruption of residual magma stored in the shallow zone of the volcano’s plumbing system.
Today, when most of the scientific community and financial resources are addressed towards the study of the present-day Etna eruptive activity, by integrating the multidisciplinary data of the monitoring networks; we hope that attention will also be given to the investigation of the past eruptive activity, which provides a fundamental contribution to also understanding the current phenomena. From this perspective, our multidisciplinary approach might be fruitful not only for Etna, but also for other volcanoes worldwide, whose past activity is still to be reconstructed.
Data Availability Statement
The original contributions presented in the study are included in the article/supplementary material, further inquiries can be directed to the corresponding author.
Author Contributions
RAC designed the article, sampled, interpreted petrochemical analyses, performed petrologic modelling, wrote and edited the article, discussed the results, and acquired funding. SB designed the article, interpreted historical sources, carried out field survey, wrote the article, and discussed the results. EDB carried out field survey, processed and interpreted volcanological data, wrote the article, and discussed the results. JCT interpreted historical sources, interpreted archeomagnetic dating, wrote the article, and discussed the results.
Funding
This study has been funded by the EC FP7 Mediterranean Supersite Volcanoes project (ECGA 308665) of the European Commission.
Conflict of Interest
The authors declare that the research was conducted in the absence of any commercial or financial relationships that could be construed as a potential conflict of interest.
Publisher’s Note
All claims expressed in this article are solely those of the authors and do not necessarily represent those of their affiliated organizations, or those of the publisher, the editors and the reviewers. Any product that may be evaluated in this article, or claim that may be made by its manufacturer, is not guaranteed or endorsed by the publisher.
Acknowledgments
Our thanks to S. Conway for revising the English language of the manuscript. Lucia Messina is thanked for the rock powder preparations.
References
Acocella, V., and Neri, M. (2003). What Makes Flank Eruptions? the 2001 Etna Eruption and its Possible Triggering Mechanisms. Bull. Volcanology 65, 517–529. doi:10.1007/s00445-003-0280-3
Acocella, V. (2021). Volcano-Tectonic Processes. Switzerland: Springer, 1–552. doi:10.1007/978-3-030-65968-4
Andronico, D., Branca, S., Calvari, S., Burton, M., Caltabiano, T., Corsaro, R. A., et al. (2005). A Multi-Disciplinary Study of the 2002?03 Etna Eruption: Insights into a Complex Plumbing System. Bull. Volcanol. 67 (4), 314–330. doi:10.1007/s00445-004-0372-8
Armienti, P., Innocenti, F., Petrini, R., Pompilio, M., and Villari, L. (1988). Sub-aphyric Alkali basalt from Mt.Etna: Inferences on the Depth and Composition of the Source Magma. Rendiconti della Società Italiana di Mineralogia e Petrologia 43, 877–891. Available at https://rruff.info/rdsmi/index.html.
Armienti, P., Tonarini, S., D’Orazio, M., and Innocenti, F. (2004). Genesis and Evolution of Mt. Etna Alkaline Lavas: Petrological and Sr-Nd-B Isotope Constraints. Per. Mineral. 73, 29–52.
Azzaro, Raffaele, R., Branca, S., Gwinner, K., and Coltelli, M. (2012). The Volcano-Tectonic Map of Etna Volcano, 1:100.000 Scale: an Integrated Approach Based on a Morphotectonic Analysis from High-Resolution DEM Constrained by Geologic, Active Faulting and Seismotectonic Data. Ital. J. Geosci. 131 (1), 153–170. doi:10.3301/IJG.2011.29
Barreca, G., Branca, S., Corsaro, R. A., Scarfì, L., Cannavò, F., Aloisi, M., et al. (2020). Slab Detachment, Mantle Flow, and Crustal Collision in Eastern Sicily (Southern Italy): Implications on Mount Etna Volcanism. Tectonics 39. doi:10.1029/2020TC006188
Barreca, G., Branca, S., and Monaco, C. (2018). Three-Dimensional Modeling of Mount Etna Volcano: Volume Assessment, Trend of Eruption Rates, and Geodynamic Significance. Tectonics 37 (3), 842–857. doi:10.1002/2017TC004851
Behncke, B., Neri, M., and Nagay, A. (2005). “Lava Flow hazard at Mount Etna (Italy): New Data from a GIS-Based Study,” in Kinematics and Dynamics of Lava Flows. Editors M. Manga, and G. Ventura (Geol. Soc. Am. Spec. Pap), 396, 187–205. Available at https://pubs.geoscienceworld.org/gsa/pages/special-papers. doi:10.1130/0-8137-2396-5.189
Bellotti, F., Branca, S., and Groppelli, G. (2010). Geological Map of Mount Etna West Rift (Italy). J. Maps 6, 96–122. doi:10.4113/jom.2010.1115
Bonaccorso, A., Aoki, Y., and Rivalta, E. (2017). Dike Propagation Energy Balance from Deformation Modeling and Seismic Release. Geophys. Res. Lett. 44, 5486–5494. doi:10.1002/2017GL074008
Bottari, A., Lo Giudice, E., Patane`, G., Romano, R., and Sturiale, C. (1975). L’eruzione etnea del Gennaio-Marzo 1974. Riv. Miner. Siciliana. 154–156, 175–199.
Bousquet, J. C., and Lanzafame, G. (2004). “The Tectonics and Geodynamics of Mt. Etna: Synthesis and Interpretation of Geological and Geophysical Data,” in Etna Volcano Laboratory" Calvari, Bonaccorso, Coltelli, Del Negro, Falsaperla. AGU (Geophysical Monograph Series), 143, 29–47. doi:10.1029/143gm03
Branca, S., and Abate, T. (2019). Current Knowledge of Etna's Flank Eruptions (Italy) Occurring over the Past 2500 years. From the Iconographies of the XVII century to Modern Geological Cartography. J. Volcanology Geothermal Res. 385, 159–178. doi:10.1016/j.jvolgeores.2017.11.004
Branca, S., Azzaro, R., De Beni, E., Chester, D., and Duncan, A. (2015b). Impacts of the 1669 Eruption and the 1693 Earthquakes on the Etna Region (Eastern Sicily, Italy): An Example of Recovery and Response of a Small Area to Extreme Events. J. Volcanology Geothermal Res. 303, 25–40. doi:10.1016/j.jvolgeores.2015.07.020
Branca, S., and Carlo, P. D. (2005). Types of Eruptions of Etna Volcano AD 1670-2003: Implications for Short-Term Eruptive Behaviour. Bull. Volcanol. 67, 732–742. doi:10.1007/s00445-005-0412-z
Branca, S., Coltelli, M., De Beni, E., and Wijbrans, J. (2008). Geological Evolution of Mount Etna Volcano (Italy) from Earliest Products until the First central Volcanism (Between 500 and 100 Ka Ago) Inferred from Geochronological and Stratigraphic Data. Int. J. Earth Sci. (Geol Rundsch) 97, 135–152. doi:10.1007/s00531-006-0152-0
Branca, S., Condomines, M., and Tanguy, J.-C. (2015a). Flank Eruptions of Mt Etna during the Greek-Roman and Early Medieval Periods: New Data from 226 Ra- 230 Th Dating and Archaeomagnetism. J. Volcanology Geothermal Res. 304, 265–271. doi:10.1016/j.jvolgeores.2015.09.002
Branca, S., D'Ajello Caracciolo, F., Malaguti, A. B., and Speranza, F. (2019). Constraining Age and Volume of Lava Flow Invasions of the Alcantara valley, Etna Volcano (Italy). New Insights from Paleomagnetic Dating and 3D Magnetic Modeling. J. Volcanology Geothermal Res. 374, 13–25. doi:10.1016/j.jvolgeores.2019.02.009
Branca, S., and Ferrara, V. (2013). The Morphostructural Setting of Mount Etna Sedimentary Basement (Italy): Implications for the Geometry and Volume of the Volcano and its Flank Instability. Tectonophysics 586, 46–64. doi:10.1016/j.tecto.2012.11.011
Branca, S., and Vigliotti, L. (2015). Finding of an Historical Document Describing an Eruption in the NW Flank of Etna in July 1643 AD: Timing, Location and Volcanic Products. Bull. Volcanol. 77, 95. doi:10.1007/s00445-015-0979-y
Branca, Stefano, S., Coltelli, M., Groppelli, G., and Lentini, F. (2011a). Geological Map of Etna Volcano, 1:50,000 Scale. Ital. J. Geosci. 130 (3), 265–291. doi:10.3301/IJG.2011.15
Branca, Stefano, S., Coltelli, Mauro, M., and Groppelli, Gianluca, G. (2011b). Geological Evolution of a Complex Basaltic Stratovolcano: Mount Etna, Italy. Italy. Ital. J. Geosci. 130 (3), 306–317. doi:10.3301/IJG.2011.13
Carignan, J., Hild, P., Mevelle, G., Morel, J., and Yeghicheyan, D. (2001). Routine Analyses of Trace Elements in Geological Samples Using Flow Injection and Low Pressure On-Line Liquid Chromatography Coupled to ICP-MS: A Study of Geochemical Reference Materials BR, DR-N, UB-N, AN-G and GH. Geostand. Newslett. 25, 187–198. doi:10.1111/j.1751-908x.2001.tb00595.x
Clocchiatti, R., Condomines, M., Guénot, N., and Tanguy, J.-C. (2004). Magma Changes at Mount Etna: the 2001 and 2002-2003 Eruptions. Earth Planet. Sci. Lett. 226, 397–414. doi:10.1016/j.epsl.2004.07.039
Condomines, M., Tanguy, J.-C., and Michaud, V. r. (1995). Magma Dynamics at Mt Etna: Constraints from U-Th-Ra-Pb Radioactive Disequilibria and Sr Isotopes in Historical Lavas. Earth Planet. Sci. Lett. 132, 25–41. doi:10.1016/0012-821x(95)00052-e
Correale, A., Paonita, A., Martelli, M., Rizzo, A., Rotolo, S. G., Corsaro, R. A., et al. (20142014). Erratum to "A Two-Component Mantle Source Feeding Mt. Etna Magmatism: Insights from the Geochemistry of Primitive Magmas" [Lithos 184-187 (2014) 243-258]. LITHOS 196-197, 243–258. p. 384, ISSN: 0024-4937. doi:10.1016/j.lithos.2014.03.012
Corsaro, R. A., and Cristofolini, R. (1996). Origin and Differentiation of Recent Basaltic Magmas from Mount Etna. Mineralogy Pet. 57, 1–21. doi:10.1007/BF01161619
Corsaro, R. A., and Métrich, N. (2016). Chemical Heterogeneity of Mt. Etna Magmas in the Last 15 Ka. Inferences on Their Mantle Sources. Lithos 252-253, 123–134. doi:10.1016/j.lithos.2016.02.006
Cristina Proietti, C., Emanuela De Beni, E., Mauro Coltelli, M., and Stefano Branca, S. (2011). The Flank Eruption History of Etna (1610-2006) as a Constraint on Lava Flow hazard. Ann. Geoph. 54, 5. doi:10.4401/ag-5333
Galetto, F., Bonaccorso, A., and Acocella, V. (2021). Relating Dike Geometry and , Njection Rate in Analogue Flux-Driven Experiments. Front. Earth Sci. 9, 665865. doi:10.3389/feart.2021.665865
Gwinner, K., Coltelli, M., Flohrer, J., Jaumann, R., Matz, K. D., Marsella, M., et al. (20062006). The HRSC-AX Mt. Etna Project: High-Resolution Orthoimages and 1 M DEM at Regional scaleISPRS Com. I Symposium. Paris, France, 3–6.
Kieffer, G. (1975). Sur l’existence d’une rift-zone à l’Etna, 280 D. Paris: C.R. Acad. Sci., 263–266.
Lormand, C., Harris, A. J. L., Chevrel, M. O., Calvari, S., Gurioli, L., Favalli, M., et al. (2020). The 1974 West Flank Eruption of Mount Etna: A Data-Driven Model for a Low Elevation Effusive Event. Front. Earth Sci. 8, 590411. doi:10.3389/feart.2020.590411
Magli, A., Branca, S., Speranza, F., Risica, G., Siravo, G., and Giordano, G. (2021). Paleomagnetic Dating of Prehistoric Lava Flows from the Urban District of Catania (Etna Volcano, Italy). GSA Bull. doi:10.1130/B36026.1
Métrich, N., Allard, P., Spilliaert, N., Andronico, D., and Burton, M. (2004). 2001 Flank Eruption of the Alkali- and Volatile-Rich Primitive basalt Responsible for Mount Etna's Evolution in the Last Three Decades. Earth Planet. Sci. LettersSci. Lett. 228, 1–17. doi:10.1016/j.epsl.2004.09.036
Miraglia, L. (2002). Evidence for Heterogeneous Magmas in the Feeding System of the 1763 “La Montagnola” Eruption at Mount Etna. Plinius 27, 108–112.
Recupero, G. (1815). Storia naturale e generale dell’Etna. 2 voll. Catania: Regia Università degli Studi.
Romano, R., Lentini, F., and Sturiale, C. (1979). Carta geologica del Monte Etna,scala 1:50.000. LAC Firenze: Progetto Finalizzato Geodinamica, Istituto Internazionale di Vulcanologia-C.N.R. (Catania).
Romano, R., and Sturiale, C. (1982). The Historical Eruptions of Mt. Etna. Roma, Italy: Memorie Soc.Geol. It., 75–97.
R. W. Le Maitre (Editor) (2002). “Igneous Rocks. A Classification and Glossary of Terms,” Recommendations of the IUGS Subcomission on the Systematics of Igneous Rocks. 2nd edition (Cambridge University Press), 236.
Smith, P. M., and Asimow, P. D. (2005). Adiabat_1ph: A New Public Front-End to the MELTS, pMELTS, and pHMELTS Models. Geochem. Geophys. Geosyst. 6, 1–8. doi:10.1029/2004GC000816
Spilliaert, N., Allard, P., Métrich, N., and Sobolev, A. V. (2006). Melt Inclusion Record of the Conditions of Ascent, Degassing, and Extrusion of Volatile-Rich Alkali basalt during the Powerful 2002 Flank Eruption of Mount Etna (Italy). J. Geophys. Res. 111, B04203. doi:10.1029/2005JB003934
Sturiale, C. (1970). La singolare eruzione dell'Etna del 1763 "La Montagnola. Rend. Soc. It. Mineral. Petrol. 26, 314–351.
Tanguy, J.-C., Condomines, M., and Kieffer, G. (1997). Evolution of the Mount Etna Magma: Constraints on the Present Feeding System and Eruptive Mechanism. J. Volcanology Geothermal Res. 75, 221–250. doi:10.1016/s0377-0273(96)00065-0
Tanguy, J.-C., and Goff, M. L. (2004). Distortion of the Geomagnetic Field in Volcanic Terrains: an Experimental Study of the Mount Etna Stratovolcano. Phys. Earth Planet. Interiors 141, 59–70. doi:10.1016/j.pepi.2003.10.003
Tanguy, J.-C., Le Goff, M., Principe, C., Arrighi, S., Chillemi, V., Paiotti, A., et al. (2003). Archeomagnetic Dating of Mediterranean Volcanics of the Last 2100 years: Validity and Limits. Earth Planet. Sci. Lett. 211, 111–124. doi:10.1016/s0012-821x(03)00186-9
Tanguy, J. C., Condomines, M., LeGoff, M., Chillemi, V., La Delfa, S., and Patanè, G. (2007). Mount Etna Eruptions of the Last 2,750 Years: Revised Chronology and Location through Archeomagnetic and 226Ra-230Th Dating. Bull. Volcanol. 83, 70–55. doi:10.1007/s00445-007-0121-x
Tanguy, J. C., and Kieffer, G. (1977). The 1974 Eruption of Mount Etna. Bull. Volcanol 40, 239–252. doi:10.1007/bf02597566
Tanguy, J. C. (1981). Les Éruptions Historiques de L'Etna: Chronologie et Localisation. Bull. Volcanol. 44, 585–640. doi:10.1007/bf02600588
Tanguy, J. C. (1980). L’Etna: étude pétrologique et paléomagnétique, implications volcanologiques. Paris, France: Thesis Univ. Paris 6-Jussieu, 618.
Tanguy, J. C. (1974). Sur la lave de la récente eruption de l’Etna (30-1 au 29-3-1974). Paris, France: C. R. Acad. Sc., t279.
Tanguy, J. C. (1978). Tholeiitic basalt Magmatism of Mount Etna and its Relations with the Alkaline Series. Contr. Mineral. Petrol. 66, 51–67. doi:10.1007/bf00376085
Tanguy, Jean-Claude, J. C., Condomines, M., Branca, S., La Delfa, S., and Coltelli, M. (2012). New Archeomagnetic and 226Ra-230Th Dating of Recent Lavas for the Geological Map of Etna Volcano. Ital. J. Geosci. 131 (2), 241–257. doi:10.3301/IJG.2012.01
Tarquini, S., Isola, I., Favalli, M., Mazzarini, F., Bisson, M., Pareschi, M. T., et al. (2007). TINITALY/01: a New Triangular Irregular Network of Italy. Ann. Geophys. 50, 407–425.
Thellier, E. (1981). Sur la direction du champ magnetique terrestre, en France, durant les deux derniers millenaires. Phys. Earth Planet. Interiors 24, 89–132. doi:10.1016/0031-9201(81)90136-9
Keywords: Etna volcano, historical activity, historical geological maps, stratigraphic sequence, deep magma composition, volcano rift zones
Citation: Corsaro RA, Branca S, De Beni E and Tanguy J-C (2021) Tales From Three 18th Century Eruptions to Understand Past and Present Behaviour of Etna. Front. Earth Sci. 9:774361. doi: 10.3389/feart.2021.774361
Received: 11 September 2021; Accepted: 30 November 2021;
Published: 22 December 2021.
Edited by:
Roberto Isaia, Istituto Nazionale di Geofisica e Vulcanologia (INGV), ItalyReviewed by:
Nobuo Geshi, Geological Survey of Japan (AIST), JapanMarie-Noelle Guilbaud, National Autonomous University of Mexico, Mexico
Copyright © 2021 Corsaro, Branca, De Beni and Tanguy. This is an open-access article distributed under the terms of the Creative Commons Attribution License (CC BY). The use, distribution or reproduction in other forums is permitted, provided the original author(s) and the copyright owner(s) are credited and that the original publication in this journal is cited, in accordance with accepted academic practice. No use, distribution or reproduction is permitted which does not comply with these terms.
*Correspondence: Rosa Anna Corsaro, cm9zYW5uYS5jb3JzYXJvQGluZ3YuaXQ=