- 1Plateau Atmosphere and Environment Key Laboratory of Sichuan Province, College of Atmospheric Sciences, Chengdu University of Information Technology, Chengdu, China
- 2Key Laboratory of Land Surface Process and Climate Change, Northwest Institute of Eco-Environment and Resources, Chinese Academy of Sciences, Lanzhou, China
- 3School of Atmospheric Sciences, Nanjing University, Nanjing, China
- 4National Center for Atmosphere Research, Boulder, CO, United States
- 5Institute of Mountain Hazards and Environment, CAS, Chengdu, China
Based on the field observation and WRF-CLM model, the effects of Gyaring and Ngoring lakes on the short-term climate over the Yellow River source area during May to September have been studied through two experiments with and without the lakes. A backward water vapor transfer model was also employed to investigate the contribution of water vapor evapotranspiration from the Gyaring and Ngoring lakes and various surface types to the local precipitation. The results show that without the Gyaring and Ngoring lakes, the sensible heat is increased by 120%, whereas the latent heat is decreased by 58.5%, and the height of atmospheric boundary layer increases from 500 to 1,500–2,000 m during daytime over the lake area. The sum of sensible and latent heat fluxes in the lake area simulated by the experiment with and without the lakes is 185.8 and 130.3 W m−2, respectively. The precipitation amount over the lake area is significantly increased without considering the lake effect, generally by more than 20–40 mm. About 63.8% of the total precipitation in Gyaring and Ngoring lakes is contributed by the external water vapor sources. The evapotranspiration from the grassland is the secondary water vapor source for the precipitation in the Yellow River source area, and 25.2% of the total precipitation is contributed by this source. Around 4.2% of the total precipitation in the lake area is contributed by the evaporation from the Gyaring and Ngoring lakes.
Introduction
The meteorological research on the Tibetan Plateau began as a result of studies on the dynamic effects of the large-scale topography of this region, initially studying how the large-scale topography of the Tibetan Plateau dynamically deflects the atmospheric air flows and causes them to rise to discovering its significant thermal effects (Manabe and Broccoli, 1990; Yanai et al., 1992; Kutzbach et al., 1993; Zhou et al., 2014). Against the background of research on the dynamic and thermal effects of the large-scale topography, medium- and small-scale circulations, energy, and water cycles over the complex surfaces of the Tibetan Plateau, the characteristics of circulations and the atmospheric boundary layer (ABL) associated with the complex topography are becoming important directions for future research. The basin of Gyaring and Ngoring lakes is a representative area with complex topography. As a component of the terrestrial hydrosphere, lakes are involved in local water and energy cycles, and large lakes can often intensify the intense local weather events (Rouse et al., 2008; Dutra et al., 2010). The important role of lakes in local weather and climate systems is attributed to their distinct hydrothermal properties from those of the surrounding land surfaces, i.e., relatively low albedo, high specific heat capacity, and small surface roughness. These differences directly result in the marked differences of surface thermal conditions and the regional water/energy cycles between lake–atmosphere and land–atmosphere interfaces, which would subsequently alter the temperature and moisture structure of the ABL (Schwartz and Karl, 1990), formation of convection and cumulus clouds (Miles and Verlinde, 2005), mesoscale circulations (Segal and Arritt, 1992), and thus the distribution of precipitation (Laird et al., 2009). Therefore, it is of great importance to broaden our knowledge about the lake–atmosphere interactions and their roles in the weather and climate at local to regional scales.
The Tibetan Plateau harbors more than 1,000 lakes with an area exceeding 1 km2, and the total lake area exceeds 50,000 km2, accounting for more than 50% of the total lake area in China (Ma et al., 2011). Despite the harsh climatic environment on the Tibetan Plateau, many efforts have been devoted to investigate the land–atmosphere and lake–atmosphere interactions by either limited field observations or regional lake–air coupled models. Based on the valuable 1-year in-situ weather records, You et al. (2007) showed the low-level atmospheric circulations over Lake Nam Co featured by notable land–lake breezes in summer. Based on the turbulent observations, Li et al. (2012) demonstrated that there exist remarkable differences in turbulent fluxes between the lake–air and land–air interfaces around Nam Co Basin, leading to the spatial heterogeneity in surface fluxes and atmospheric boundary stability. Lu et al. (2007) have carried out a set of numerical simulations with and without Qinghai Lake and pointed out that Qinghai Lake serves as a cold dry “island” and results in the notable outflow of low-level air over the lake to the surrounding area in daytime during summer, whereas at nighttime, Qinghai Lake is a warm wet “island,” and land winds blow from the northeast to the lake area. Based on the simulation of the Fifth-generation Penn State/NCAR Mesoscale model (MM5), Lu et al. (2008) revealed the cold lake effect of Lake Nam Co during summer that delays the timing of turbulent mixing and convective activities in the ABL. Li et al. (2009) used the Weather Research and Forecasting (WRF) model to simulate the snow distribution over the Nam Co Basin and showed that the lake surface water temperature plays an important role in the formation of precipitation over the downwind regions of Lake Nam Co.
Gyaring and Ngoring are the two largest lakes in the Yellow River source region. Recently, Gyaring and Ngoring lakes receive more attention in exploring their impacts on regional energy/water cycles and the impacts on the regional weather and climate. Nowadays, the continuous observations for the surface energy budgets, i.e., radiation and turbulent flux, and the local weather conditions over Ngoring Lake have been well established (Li et al., 2015). The data provide essential references to calibrate the momentum/heat/moisture roughness length, surface water/ice albedo, and drag coefficients for high-altitude lakes and are indeed valuable for improving our understanding of the lake–atmosphere interactions over the Tibetan Plateau (Wen et al., 2015, 2016; Li et al., 2018). Li et al. (2016) adopted 2-year ice-free eddy covariance measurements over Ngoring Lake to investigate the surface roughness lengths and the associated influences on the turbulent heat fluxes, and their results demonstrated that Ngoring Lake is characterized by a persistent unstable atmospheric layer due to the lake–air temperature contrast. In addition, as extreme weather events occur frequently in the Tibetan Plateau, these high-altitude lakes feature intensive lake–air interactions and exert more significant feedback on the local meteorological elements. For example, based on multi-source field, reanalysis, and remote-sensed data, Li et al. (2018) pointed out that a cold, dry air incursion tends to decrease the over-lake potential temperature gradient and facilitate buoyancy flux, which further leads to an active convective boundary layer with the sharp increase in its thickness and heating of convection therein. However, most of previous numerical simulation studies on Gyaring and Ngoring lakes focused on the analysis of individual cases, only examined a relatively short period through numerical simulation, and did not thoroughly investigate the short-term climatic effect of the lakes. The contribution of water vapor evaporation from land surfaces, particularly lakes, to water vapor in the atmosphere over the Tibetan Plateau is not negligible. In addition, few studies have sufficiently investigated the contribution of water vapor evaporation from Gyaring and Ngoring lakes and water recycling in the surrounding soil and vegetated surfaces to precipitation in the lake area.
In this study, we employed the WRF model to perform numerical simulations to address the short-term climatic effect of Gyaring and Ngoring lakes on the surrounding area and a backward water vapor transfer model to investigate the contribution of water vapor evapotranspiration from various surface types to precipitation.
Study Area and Methodology
Study Area
Ngoring Lake (surface area of 610 km2) and Gyaring Lake (surface area of 526 km2) are located in the source region of the Yellow River (Li et al., 2017). The average altitude of the lake surfaces is 4,274 m above sea level (as shown in Figure 1). Ngoring Lake and Gyaring Lake are large freshwater lakes in the Tibetan Plateau. Around the lakes, the vegetation type is alpine meadow with a height of 5–10 cm. The vegetation coverage ranges from 0.55 to 0.75 (Li and Li, 2015), and the leaf area index is approximately 1.2–1.4 m2 m−2 in summer (Li et al., 2021). The albedo of the lakes and alpine meadow averaged over 12:00–14:00 local solar time (LST) in summer is 0.04 and 0.2, respectively. The grassland eddy flux tower site (97°33′16″E, 34°54′51″N), standing on a flat underlying surface, is located 1.5 km west to Ngoring Lake.
WRF Model and Numerical Experiment Design
Numerical models play an important role in weather simulation and forecasting (Wang and Yu, 2013; Wu et al., 2014; Zhou et al., 2014). The WRF model was used to simulate the meteorological conditions from 00:00 LST on May 4, 2013 to 24:00 LST on September 30, 2013 in the basin of Gyaring and Ngoring lakes. Table 1 summarizes the experimental parameters of the WRF model. A double one-way grid nesting technique was used. The results were output every hour for both the first and second domains of the WRF model. The model contained 30 layers of atmosphere in the vertical direction. The pressure at the top layer of atmosphere was set to 50 hPa. In addition to a control experiment, a sensitive experiment on the same area with the Gyaring and Ngoring lakes replaced by grassland surface was conducted to analyze the effects of the lakes on the air temperature, local wind field, near-surface energy budget, and precipitation in the lake area.
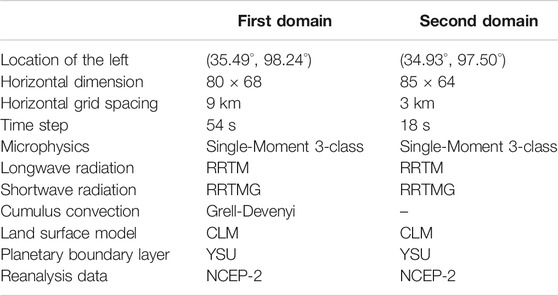
TABLE 1. Model configurations for the two nested domain and parameterization schemes used in the WRF model.
Backward Water Vapor Tracing Method
In this study, we used a backward water vapor tracing method based on the Lagrangian trajectory method (Brubaker et al., 2001; Dirmeyer and Brubaker, 2007; Harding and Snyder, 2012). The hourly output data from the WRF model were analyzed. The specific analysis method is described as follows: first, the period from May 4, 2013 to September 30, 2013 was divided into 30 5-day window intervals. For each window, 1,000 air parcels were randomly released at the grid point where precipitation occurred (total precipitation for each of the 5 days) to comprehensively consider the uncertainties of backward tracing at various longitudes, latitudes, and heights. The probabilities of the spatial heights of the released 1,000 air parcels were determined for a specified longitude and latitude within this grid point by the vertical specific humidity profile (e.g., if 70% of the humidity is at the bottom layer of the model, then there is a 70% probability that an air parcel is released to the bottom layer), ensuring relatively more air parcels in high humidity layers. Then, for each released air parcel, its horizontal and vertical positions at 1 h before the current moment were calculated. The horizontal position was determined by the wind vector at the position of the air parcel at the current moment. The vertical position was determined by the previous moment when the potential temperature in the layer was the same as that at the initial position where the air parcel was released, i.e., each air parcel moved vertically along the constant potential temperature line. The contribution of evapotranspiration from each position where an air parcel passed to the corresponding grid point was calculated. The percentage of contribution was calculated by dividing the evapotranspiration at a position where an air parcel passed by the total precipitation in the vertical layer where the position was located. Each time there was a contribution from a position passed by an air parcel to the total precipitation at the current grid point in the current window, this contribution was deducted from the total precipitation. When the sum of local contributions equals the total precipitation at the current grid point in the current window, backward tracing is completed, and all the local sources for precipitation are considered to have been traced. Water vapor tracing was terminated when an air parcel reached the boundary of the modeled area or the tracing duration exceeded 7 days. Finally, the precipitation contributed by the evapotranspiration from each surface type was calculated, and this precipitation was referred to as surface-induced precipitation.
Results and Discussion
Validation of the Control Experiment Result
This section focuses mainly on the short-term climatic characteristics of the study area. Thus, the average simulated and observed values obtained at the grass site were compared. As shown in Figure 2, the model well captured the overall characteristics of the diurnal cycles of sensible and latent heat fluxes and 2 m air temperature with the R2 ranging from 0.89 to 0.99. However, the model overestimated the daytime sensible heat flux by 40 W m−2. In addition, the 2 m air temperatures between 00:00 LST and 09:00 LST during the simulation period were underestimated by the model.
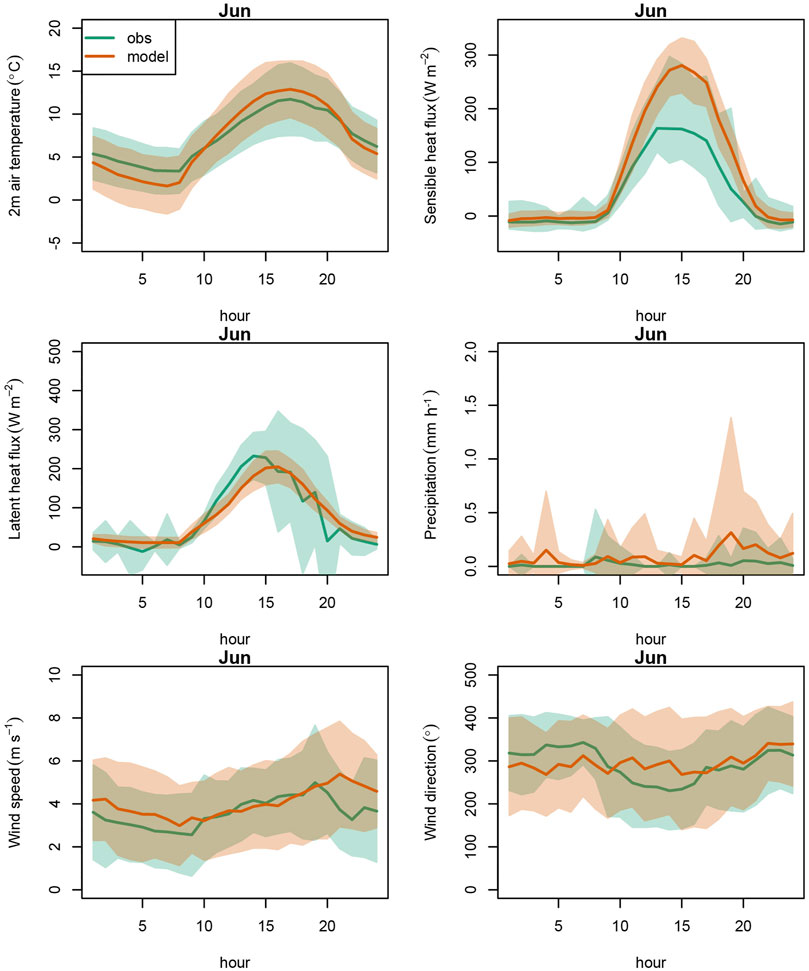
FIGURE 2. Comparison of the June 2013 averaged diurnal cycle between the WRF simulation and the site observation. The shaded areas are the standard error during the month.
The WRF model did not well capture the diurnal cycles of wind speed and precipitation and overestimated the wind speed and precipitation (Table 2) during June, July, and August with a mean overestimation of 0.58 m s−1 and 0.05 mm h−1, and such overestimation mainly occurred in nighttime (Figure 2). The observed wind direction showed clear lake–land breeze wind shift, while the WRF simulated wind shift was not as strong as the observation.

TABLE 2. Bias, root mean square error, and R2 between the WRF simulation and the site observation in June, July, and August 2013. The latent and sensible heat flux observations in July and August are missing, only June values are shown.
Short-Term Local Climate Result Analysis
There is a substantial change of the latent heat flux in the original lake area after removal of the lakes (Figure 3A). The results from the experiment with the lakes show the latent heat peaks in September (196.0 W m−2). After removal of the lakes, the latent heat in September decreases to 41.0 W m−2, which is the lowest among all months; in addition, the latent heat decreases substantially in other months. The average latent heat of the lakes between May and September simulated by the experiment with the lakes is 155.7 W m−2. Without the lakes, the average latent heat decreases by 58.5% in 64.7 W m−2. From a perspective of the entire study area, there is no substantial difference in the simulated latent heat between the experiments with and without the lakes due to the limited lake area compared to the entire study region. However, the latent heat in the entire study area simulated by the experiment with the lakes is 65.1 W m−2, slightly higher than 61.2 W m−2 in the experiment without the lakes.
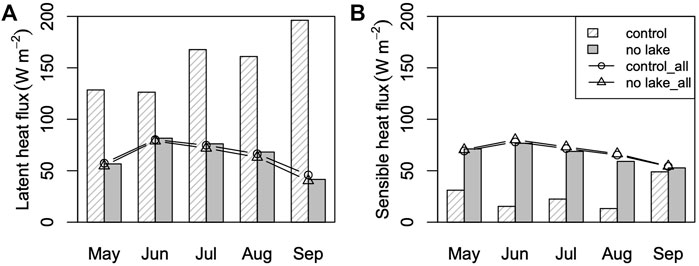
FIGURE 3. The comparison of monthly mean latent heat flux (A) and sensible heat flux (B) simulated by the experiments with and without lakes. The histogram shows the average sensible heat/latent heat flux in the lake area, and the solid line shows the average sensible heat and latent heat changes over the entire study area.
There is a change in the sensible heat flux over the lake area after removal of the lakes (Figure 3B). The results from the experiment with the lakes show that the sensible heat is the lowest (15.4 W m−2) in June among all months. After removal of the lakes, the sensible heat in June increases to 76.7 W m−2. In addition, the sensible heat increases substantially in other months. The average sensible heat in the lake area between May and September simulated by the experiment with the lakes is 30.1 W m−2. Without the lakes, the average sensible heat over the lake area between May and September increases by 118% in 65.7 W m−2. From a perspective of the entire study area, there is no substantial difference in the sensible heat simulated by the experiments with and without the lakes due to the limited lake area compared to the entire study region. However, the sensible heat in the entire study area simulated by the experiment with the lakes is 67.5 W m−2, slightly lower than that simulated by the experiment without the lakes (68.9 W m−2).
Overall, the lakes tend to decrease the sensible heat flux and increase the latent flux in the lake area. During the simulation period (May to September 2013), the average sum of the sensible and latent heat fluxes in the lake area simulated by the experiment with (without) the lake is 185.8 (130.4) W m−2, indicating that the presence of lakes leads to a considerable increase in the total amounts of heat and water vapor exchange at the land–air interface. From a perspective of the entire study area, the sum of the sensible and latent heat fluxes simulated by the experiments with (without) the lakes is 132.6 (130.1) W m−2, suggesting that there is also a slight decrease in the sum of the sensible and latent heat fluxes after removal of the lakes due to their limited area.
The height of the ABL over the area of Gyaring and Ngoring lakes and the surrounding land surfaces simulated in the control experiment is ∼500 and above 1,500 m, respectively (Figure 4A). The height of the ABL over the lakes increases to 2,000 m in the no lake experiment (Figure 4B). The presence of the lakes effectively reduces the height of the ABL over the lake area during daytime.
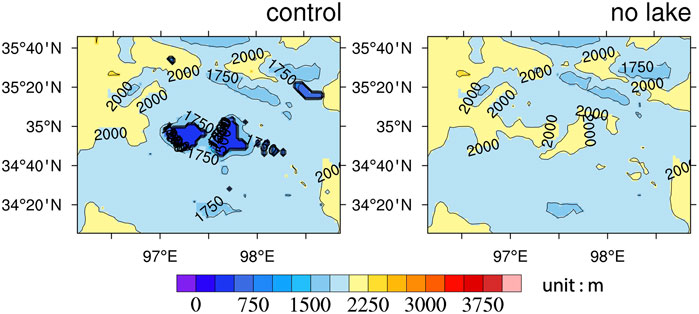
FIGURE 4. The average height of atmospheric boundary layer produced by the experiments with (control) and without lakes (no lake) at 14:00 LST during May to September 2013.
Figure 5 shows the distribution of the average wind field at 14:00 LST. Local dispersion wind field can be noted over Gyaring and Ngoring lakes; it flows from the center of the lakes to the surrounding land. The dispersion wind field is absent in the no lake experiment, implying that Gyaring and Ngoring lakes can affect the wind field characteristics near the surface.
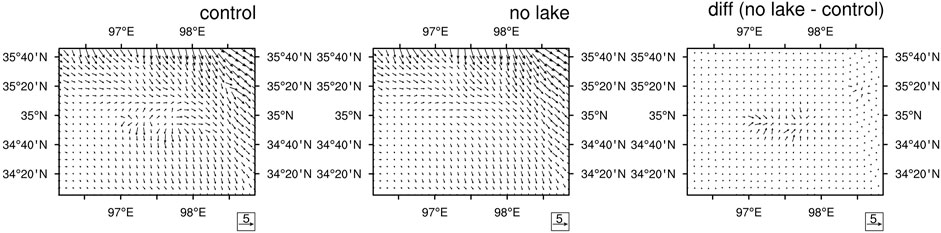
FIGURE 5. The surface wind field (unit: m s−1) simulated by the control experiment with lakes and the no lake experiment at 14:00 LST averaged during May to September 2013.
The precipitation over Gyaring and Ngoring lakes simulated by the control experiment is high in the south and low in the north (Figure 5). Compared to the control experiment, the precipitation over the central lake area simulated by the no lake experiment is increased by more than 20 mm over some locations (Figure 6). The increase reaches up to more than 25% of the precipitation in this area for the same period.
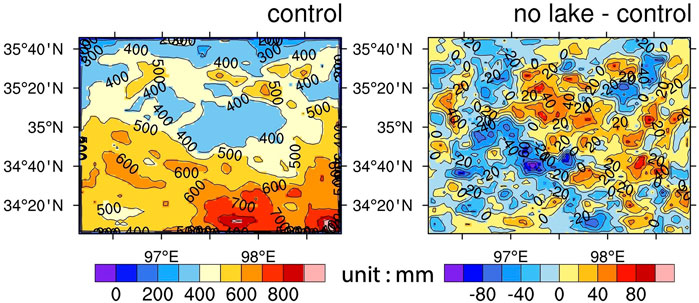
FIGURE 6. The cumulative precipitation simulated by the control experiment with lakes (left), and the difference in the simulated cumulative precipitation between the no lake experiment and control experiment (right) during May to September 2013.
Figure 7 shows prevailing westerly winds at 550 hPa over the Gyaring and Ngoring lakes with the air temperature decreasing from south to north. Compared to the control experiment, the no lake experiment produced slightly increased air temperature in most parts of the study area with a maximum increase of ∼0.15°C located in the south of Gyaring and Ngoring lakes.
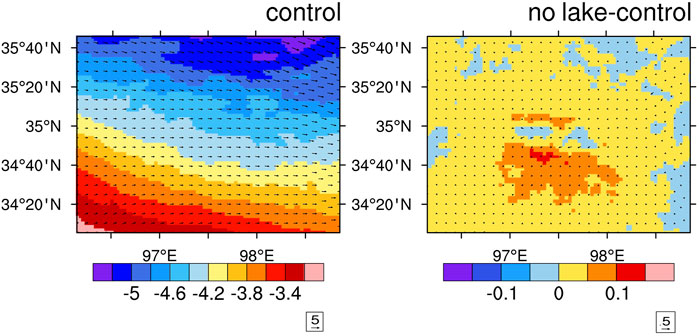
FIGURE 7. The average surface air temperature (unit: °C) and wind field (unit: m s−1) produced by the control experiment with lakes and the differences between no lake experiment and the control experiment during May to September 2013.
Contribution of the Water Vapor Evapotranspiration to the Local Precipitation
Figure 8 shows the distribution of surface types included in the WRF model in the second nested domain of the study area. Grasslands account for the highest proportion (76.3%) of all surface types, followed by shrublands (11.8%). Mixed shrubland/grassland composite zones and water bodies account for 7.9% and 3.5% of all surface types, respectively.
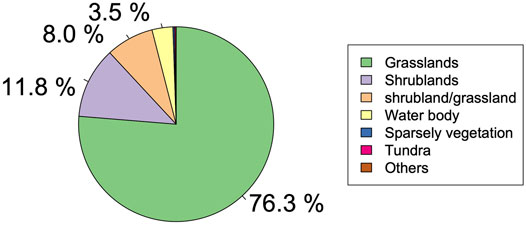
FIGURE 8. Percentage of each surface type to the total surface over the Yellow River source area in the second nested model domain.
The external water vapor sources (those outside the second nested domain) account for 63.8% of the water vapor sources of precipitation in the entire study area and are the primary water vapor sources for precipitation (Figure 9). The evapotranspiration from the grassland surfaces is the secondary water vapor source for precipitation, which accounts for 25.2% of the total water vapor sources. In addition, only 4.2% of precipitation in the Gyaring and Ngoring lakes is contributed by the evaporation from the lakes.
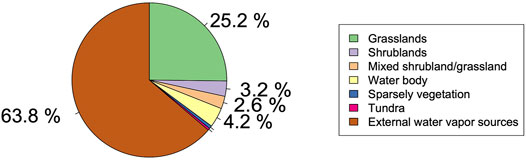
FIGURE 9. Percentage contribution of external water vapor sources and evapotranspiration from different underlying surfaces to precipitation in the Yellow River source area during May to September 2013.
Precipitation in the entire study area is mainly affected by the evapotranspiration from grassland surfaces (except for the external water vapor sources), which contributes to the precipitation in almost all parts of the second nested domain area (Figure 10), while the evaporation from the lakes is the primary water vapor source for the precipitation over the lakes. Precipitation induced by evaporation from the lakes also mainly occurs within a limited area that comprises the lakes and their surrounding area. Evapotranspiration from the shrubland surfaces, grassland/shrubland composite surfaces, sparsely vegetated surfaces, and tundra surfaces contributes relatively insignificantly to precipitation.
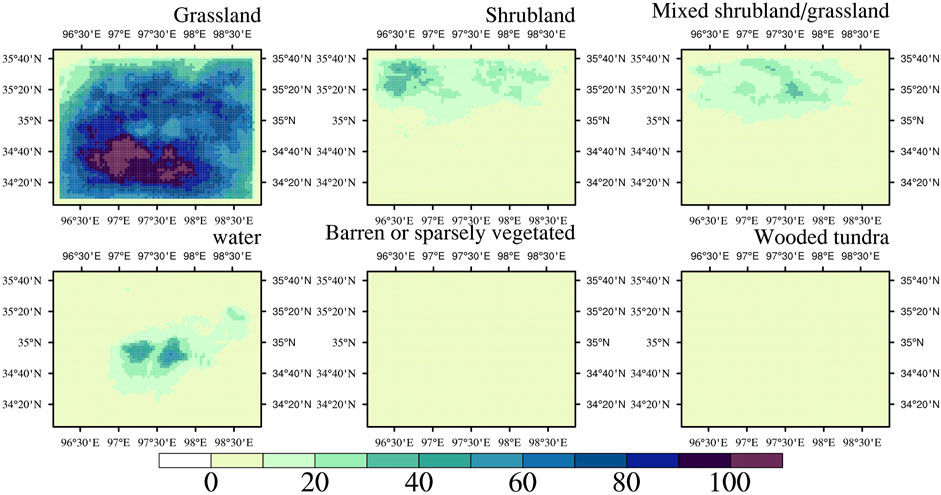
FIGURE 10. Contribution of the evapotranspiration from different underlying surfaces in the Yellow River source area to the precipitation during May to September 2013 (unit: mm).
Conclusion
In this study, two numerical experiments (a control experiment with Gyaring and Ngoring lakes and a sensitive experiment without the lakes) were performed using a coupled WRF-community land model to simulate the sensible and latent heat fluxes, local precipitation, and the height of the ABL over the study area in a period of 5 months (May to September). The effects of Gyaring and Ngoring lakes on the short-term climate in the lake area were investigated. In addition, a backward water vapor transfer model was employed to quantitatively analyze the contribution of evapotranspiration from various surface types to precipitation in the Gyaring and Ngoring lake areas. The main conclusions derived from this study are:
(1) After replacement of the lakes with grasslands, the sensible heat increases by 118%, and the latent heat decreases by 58.5% for the experimental period (May to September 2013). After removal of the lakes, the sensible heat in the original lake area increases, whereas the latent heat decreases. The sum of the sensible and latent heat fluxes in the lake area simulated by the experiment with (without) the lakes is 185.8 (130.4) W m−2. This indicates that there is a considerable decrease in the total amounts of heat and water vapor exchange at the land–air interface due to the absence of the lakes. From a perspective of the entire study area, the sum of the sensible and latent heat fluxes simulated by the experiment with (without) the lakes is 132.6 (130.1) W m−2, suggesting that there is also a slight decrease in the sum of the sensible and latent heat fluxes due to the absence of the lakes.
(2) Precipitation in the Gyaring and Ngoring lakes simulated by the experiment with the lakes is high in the south and low in the north. After removal of the lakes, the total precipitation in the original central lake area during May to September increases significantly, generally by more than 20 mm with more than 80 mm increase in some locations. The increase in temperature and the emergence of a divergent wind field at 550 hPa due to the absence of lakes are both factors conducive to the increase in precipitation.
(3) The control experiment results show that the height of the ABL over Gyaring and Ngoring lakes and the surrounding area is ∼500 and above 1,500 m, respectively. Without the lakes, the height of the ABL simulation over the original lake area increases to above 1,500–2,000 m. The presence of Gyaring and Ngoring lakes tends to reduce the height of the ABL over the lakes during daytime.
(4) Of the total precipitation in Gyaring and Ngoring lakes, 63.8% is contributed by the external water vapor sources. Evapotranspiration from grassland surfaces is the secondary water vapor source for precipitation in the study area, and 25.2% of the total precipitation is contributed by this source. Of the total precipitation in the lake area, 4.2% is contributed by the evaporation from the Gyaring and Ngoring lakes.
While deriving these conclusions, we also notice that further work is required to make improvements (e.g., coupling the WRF model with surface parameters retrieved from satellite remote sensing data) because there is a noticeable discrepancy between the surface data in the WRF model and the actual data. In addition, the results of contribution of evapotranspiration from various surface types in the basin of Gyaring and Ngoring lakes require further validation.
Data Availability Statement
The raw data supporting the conclusion of this article will be made available by the authors, without undue reservation.
Author Contributions
XY: writing—original draft, conceptualization, methodology, and software. JW: methodology, software, formal analysis, and writing—review and editing. AH: writing—review and editing, formal analysis, and visualization. YL: methodology and software. XM: software and writing—review and editing. YZ: writing—review and editing. YW: visualization and data curation. LM: resources.
Funding
This study is supported by the National Natural Science Foundation of China under Grant Numbers 41975130 and 41975081, the Strategic Priority Research Program of Chinese Academy of Sciences (No. XDA20050102), CAS “Light of West China” Program (E12903010, Y929641001), the Sichuan Provincial Science and Technology Planning Project (2021YJ0025), the Jiangsu University “Blue Project” Outstanding Young Teachers Training Project, the Fundamental Research Funds for the Central Universities, and the Jiangsu Collaborative Innovation Center for Climate Change.
Conflict of Interest
The authors declare that the research was conducted in the absence of any commercial or financial relationships that could be construed as a potential conflict of interest.
Publisher’s Note
All claims expressed in this article are solely those of the authors and do not necessarily represent those of their affiliated organizations, or those of the publisher, the editors, and the reviewers. Any product that may be evaluated in this article, or claim that may be made by its manufacturer, is not guaranteed or endorsed by the publisher.
References
Brubaker, K. L., Dirmeyer, P. A., Sudradjat, A., Levy, B. S., and Bernal, F. (2001). A 36-yr Climatological Description of the Evaporative Sources of Warm-Season Precipitation in the Mississippi River Basin. J. Hydrometeor 2 (6), 537–557. doi:10.1175/1525-7541(2001)002<0537:aycdot>2.0.co;2
Dirmeyer, P. A., and Brubaker, K. L. (2007). Characterization of the Global Hydrologic Cycle from a Back-Trajectory Analysis of Atmospheric Water Vapor. J. Hydrometeorology 8 (1), 20–37. doi:10.1175/jhm557.1
Dutra, E., Stepanenko, V., Balsamo, G., Viterbo, P., Miranda, P., Mironov, D., et al. (2010). An Offline Study of the Impact of Lakes on the Performance of the ECMWF Surface Scheme. Boreal Environ. Res. 15 (2), 100–112.
Harding, K. J., and Snyder, P. K. (2012). Modeling the Atmospheric Response to Irrigation in the Great Plains. Part II: The Precipitation of Irrigated Water and Changes in Precipitation Recycling. J. Hydrometeorology 13 (6), 1687–1703. doi:10.1175/jhm-d-11-099.1
Kutzbach, J. E., Prell, W. L., and Ruddiman, W. F. (1993). Sensitivity of Eurasian Climate to Surface Uplift of the Tibetan Plateau. J. Geology. 101 (2), 177–190. doi:10.1086/648215
Laird, N. F., Desrochers, J., and Payer, M. (2009). Climatology of Lake-Effect Precipitation Events over Lake Champlain. J. Appl. Meteorology Climatology 48 (2), 232–250. doi:10.1175/2008jamc1923.1
Li, M., Ma, Y., Hu, Z., Ishikawa, H., and Oku, Y. (2009). Snow Distribution over the Namco Lake Area of the Tibetan Plateau. Hydrol. Earth Syst. Sci. 13, 2023–2030. doi:10.5194/hess-13-2023-2009
Li, M., Yang, Y., Ma, Y., Sun, F., Chen, X., Wang, B., et al. (2012). Analyses on Turbulence Data Control and Distribution of Surface Energy Flux in Namco Area of Tibetan Plateau. Plateau Meteorology 31 (4), 875–884. (in Chinese). doi:10.1007/s11783-011-0280-z
Li, P., and Li, M. (2015). Study on Vegetation Fraction Based on Kriging Interpolation Method-A Case Study of Zhaling Lake, Eling Lake. J. Anhui Agric. Sci. 43 (8), 321–324. (in Chinese). doi:10.13989/j.cnki.0517-6611.2015.08.127
Li, Z., Lyu, S., Ao, Y., Wen, L., Zhao, L., and Wang, S. (2015). Long-term Energy Flux and Radiation Balance Observations over Lake Ngoring, Tibetan Plateau. Atmos. Res. 155, 13–25. doi:10.1016/j.atmosres.2014.11.019
Li, Z., Lyu, S., Chen, S., Ao, Y., Zhao, L., Chen, H., et al. (2021). Observed Characteristics of the Water and Heat Transfer of the Soil-Snow-Atmosphere System through the Snowpack in the Eastern Tibetan Plateau. Atmos. Res. 248, 105195. doi:10.1016/j.atmosres.2020.105195
Li, Z., Lyu, S., Wen, L., Zhao, L., Ao, Y., and Wang, S. (2017). Effect of a Cold, Dry Air Incursion on Atmospheric Boundary Layer Processes over a High-Altitude Lake in the Tibetan Plateau. Atmos. Res. 185, 32–43. doi:10.1016/j.atmosres.2016.10.024
Li, Z., Lyu, S., Wen, L., Zhao, L., Meng, X., and Ao, Y. (2018). Effect of Roughness Lengths on Surface Energy and the Planetary Boundary Layer Height over High-Altitude Ngoring Lake. Theor. Appl. Climatology 133 (3), 1191–1205. doi:10.1007/s00704-017-2258-7
Li, Z., Lyu, S., Zhao, L., Wen, L., Ao, Y., and Wang, S. (2016). Turbulent Transfer Coefficient and Roughness Length in a High-Altitude lake, Tibetan Plateau. Theor. Appl. Climatology 124 (3), 723–735. doi:10.1007/s00704-015-1440-z
Lu, Y., Ma, Y., Li, M., and Sun, F. (2008). Study on Characteristic of Atmospheric Boundary Layer over Lake Namco Region, Tibetan Plateau. Plateau Meteorology 27 (4), 1205–1210. (in Chinese).
Lu, Y., Yang, X., and Ma, Y. (2007). Numerical Simulation of Summer Circulation and Atmospheric Boundary Layer Characteristics over Qinghai Lake. Plateau Meteorology 26 (4), 686–692.
Ma, R., Yang, G., Duan, H., Jiang, J., Wang, S., Feng, X., et al. (2011). China's Lakes at Present: Number, Area and Spatial Distribution. Sci. China Earth Sci. 54 (2), 283–289. doi:10.1007/s11430-010-4052-6
Manabe, S., and Broccoli, A. J. (1990). Mountains and Arid Climates of Middle Latitudes. Science 247, 192–195. doi:10.1126/science.247.4939.192
Miles, N. L., and Verlinde, J. (2005). Observations of Transient Linear Organization and Nonlinear Scale Interactions in Lake-Effect Clouds. Part I: Transient Linear Organization. Monthly Weather Rev. 133, 677–691. doi:10.1175/mwr-2879.1
Rouse, W. R., Blanken, P. D., Bussières, N., Walker, A. E., Oswald, C. J., Schertzer, W. M., et al. (2008). An Investigation of the Thermal and Energy Balance Regimes of Great Slave and Great Bear Lakes. J. Hydrometeorology 9 (6), 1318–1333. doi:10.1175/2008jhm977.1
Schwartz, M. D., and Karl, T. R. (1990). Spring Phenology: Nature's Experiment to Detect the Effect of "Green-Up" on Surface Maximum Temperatures. Mon. Wea. Rev. 118, 883–890. doi:10.1175/1520-0493(1990)118<0883:spnetd>2.0.co;2
Segal, M., and Arritt, R. W. (1992). Nonclassical Mesoscale Circulations Caused by Surface Sensible Heat-Flux Gradients. Bull. Amer. Meteorol. Soc. 73 (10), 1593–1604. doi:10.1175/1520-0477(1992)073<1593:nmccbs>2.0.co;2
Wang, S., and Yu, E. (2013). Simulation and Projection of Changes in Rainy Season Precipitation over China Using the WRF Model. Acta Meteorol. Sin 27 (4), 577–584. doi:10.1007/s13351-013-0406-2
Wen, L., Lv, S., Li, Z., Zhao, L., and Nagabhatla, N. (20152015). Impacts of the Two Biggest Lakes on Local Temperature and Precipitation in the Yellow River Source Region of the Tibetan Plateau. Adv. Meteorology 2015, 1–10. doi:10.1155/2015/248031
Wen, L., Lyu, S., Kirillin, G., Li, Z., and Zhao, L. (2016). Air-lake Boundary Layer and Performance of a Simple lake Parameterization Scheme over the Tibetan highlands. Tellus A: Dynamic Meteorology and Oceanography 68 (1), 31091. doi:10.3402/tellusa.v68.31091
Wu, T., Song, L., Li, W., Wang, Z., Zhang, H., Xin, X., et al. (2014). An Overview of BCC Climate System Model Development and Application for Climate Change Studies. Acta Meteorol. Sin 28 (1), 34–56. doi:10.1007/s13351-014-3041-7
Yanai, M., Li, C., and Song, Z. (1992). Seasonal Heating of the Tibetan Plateau and its Effects on the Evolution of the Asian Summer Monsoon. J. Meteorol. Soc. Jpn. 70 (1B), 319–351. doi:10.2151/jmsj1965.70.1b_319
You, Q., Kang, S., Li, C., Li, M., and Liu, J. (2007). Variation Features of Meteorological Elements at Namco Station, Tibetan Plateau. Meteorol. Monthly 33 (3), 54–60. (in Chinese). doi:10.16089/j.cnki.1008-2786.2007.04.007
Keywords: WRF model, numerical simulation, backward water vapor transfer model, vapor sources, lake climate effect
Citation: Yang X, Wen J, Huang A, Lu Y, Meng X, Zhao Y, Wang Y and Meng L (2022) Short-Term Climatic Effect of Gyaring and Ngoring Lakes in the Yellow River Source Area, China. Front. Earth Sci. 9:770757. doi: 10.3389/feart.2021.770757
Received: 04 September 2021; Accepted: 21 December 2021;
Published: 26 January 2022.
Edited by:
Steffen Mischke, University of Iceland, IcelandReviewed by:
Xuezhen Zhang, Institute of Geographic Sciences and Natural Resources Research (CAS), ChinaWei Sun, Sun Yat-Sen University, China
Copyright © 2022 Yang, Wen, Huang, Lu, Meng, Zhao, Wang and Meng. This is an open-access article distributed under the terms of the Creative Commons Attribution License (CC BY). The use, distribution or reproduction in other forums is permitted, provided the original author(s) and the copyright owner(s) are credited and that the original publication in this journal is cited, in accordance with accepted academic practice. No use, distribution or reproduction is permitted which does not comply with these terms.
*Correspondence: Anning Huang, YW5odWFuZ0BuanUuZWR1LmNu