- 1State Key Laboratory of Petroleum Resources and Prospecting, China University of Petroleum, Beijing, China
- 2College of Geosciences, China University of Petroleum, Beijing, China
- 3China University of Petroleum-Beijing at Karamay, Karamay, China
Strike-slip deformation belts are interesting structures in the crust and are of significance in petroleum exploration. The Shunbei 5 fault belt (SB5), a long strike-slip deformation belt in the Tarim Basin, played an important role in the formation of a recently discovered major oilfield known as the Shunbei oilfield. In this study, models of plan view and vertical profile were established to interpret SB5 with multi-cycled tectonic activities. To this end, its structural framework, tectonic evolution, and associated plate tectonics were investigated using 2D and 3D seismic data. SB5 was formed as a dextral simple shear belt at the end of the Middle Ordovician. In the plan view, R-shears and P-shears with local transpressional and transtensional structures were observed. Along the vertical profiles, various structural styles occurred at various depths and strata in response to various stratigraphy mechanisms. Although these structures show clear boundaries between them, they correspond to the same formation time, indicating that they underwent deformation simultaneously. The second activity of SB5 occurred at the end of the late Ordovician, during which it was a dextral transtensional strike-slip deformation belt consisting of left-stepping en echelon R-shears. The R-shears were transtensional during the progressive deformation. Subsequently, SB5 underwent several strike slips of weak strength. Notably, SB5 cut through a deep Middle Cambrian gypsum salt layer and connected the deep Lower Cambrian source rock with deep Lower and Middle Ordovician carbonates to form the oil and gas reservoirs. The established models are of reference value in the interpretation of other subsurface strike-slip deformation belts.
Introduction
Strike-slip faults are an important tectonic feature in nature. A strike-slip deformation belt commonly consists of various structures with different mechanics, such as normal faults, shear faults, and reverse faults or folds (Riedel, 1929; Chinnery, 1963; Wilcox et al., 1973; Sylvester, 1988). These structures can form petroleum traps in a basin. Moreover, strike-slip faults may cut deep below the surface to form petroleum migration pathways. Therefore, strike-slipping structures are of important significance in petroleum exploration (Lowell, 1985; Allen and Allen, 2013; Chen et al., 2018; El Ghamry et al., 2020).
In recent years, the China Petroleum and Chemical Corporation (Sinopec) discovered a major oilfield known as the Shunbei oilfield around the Shunbei 5 well in the middle west of the Tarim basin (Figure 1). The oil and gas reservoirs exist in Lower and Middle Ordovician carbonates, unevenly distributed along faults at depths exceeding 7,000 m (Cao et al., 2020). Its total resource is estimated at 17 × 108 t, of which oil and gas account for 12 × 108 t and 5,000 × 108 m3, respectively (Jiao, 2018). The reservoirs are fractures and caves and are characterized by ultra-depth, and ultra-high pressure and temperature.
The formation of the Shunbei oilfield is related to the Shunbei 5 strike-slip deformation belt (SB5). The strike-slip faults in this belt connected the deep Lower Cambrian source rock with the Ordovician carbonates. The complex structural framework of SB5 determined the uneven distribution of oil and gas along the fault (Cao et al., 2020). However, the interpretation of the structural framework of SB5 is debatable (Deng et al., 2018; Li et al., 2019; Liu, 2020; Wang et al., 2020). The active time of SB5 is difficult to determine and its formation mechanism is not clear. U-Pb isotopic dating of calcite in fractures in Ordovician Carbonate rocks indicated that the formation of SB5 began at the end of the Middle Ordovician (Wu et al., 2020). However, according to seismic data, the formation time of strike-slip faults in the Tabei and Tazhong uplifts was in the late Ordovician and the strike-slip faults in the Tazhong uplift formed earlier than those in the Tabei uplift (Wu et al., 2012; Zhen et al., 2015; Han et al., 2017; Deng et al., 2018; Wu et al., 2018; Huang, 2019).
In this study, models were constructed to determine the kinematic types and analyze the plane structural framework of strike-slip deformation belts from the perspective of structural geology theory. Indicators for identifying the formation time of strike-slip faults in the vertical profile and plan view were initially established. Based on these models and indicators, the structural framework and formation time of SB5 were interpreted. Integrating plate tectonics, the formation mechanism of SB5 was discussed and its significance in petroleum geology was addressed. The presented formation mechanisms of the strike-slip faults can provide guidance for the exploration of the Shunbei oilfield and contribute to better understanding of global strike-slip faults.
Geological Setting
The Tarim Basin is located in the Xinjiang Uygur Autonomous Region of China, with an area of 56 × 104 km2. It is situated on the Tarim plate and is surrounded by mountains such as the Tianshan mountains to the north, Kunlun mountains to the south, and Altyn-Tagh mountains to the southeast (Figure 1). SB5 is located in the central Tarim Basin, across the Tazhong Uplift, Awati Depression, and Tabei Uplift (Figure 2).
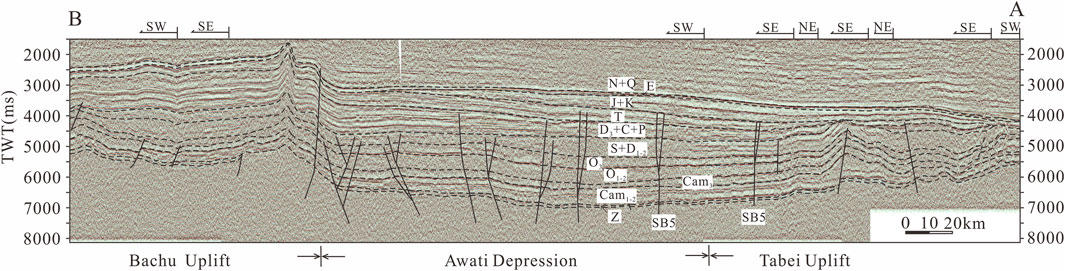
FIGURE 2. 2D Seismic profile showing some tectonic units of the Tarim Basin. (N + Q)-Neogene and Quaternary, E-Paleogene, (J + K)-Jurassic and Cretaceous, T-Triassic, (D3 + C + P)-Upper Devonian and Carboniferous and Permian, (S + D1-2)-Silurian and Lower and Upper Devonian, O3-Upper Ordovician, O1-2-Lower and Middle Ordovician, Cam3-Upper Cambrian, Cam1-2-Lower and Middle Cambrian, Z-Sinian
The sedimentary cover includes Nanhua, Sinian, Paleozoic, Mesozoic, and Cenozoic sediments (Figure 3). The late Proterozoic Nanhua system comprises continental rift sediments; the rift occurred during the breakup of the Rodinia supercontinent (Wu L. et al., 2016; Shi et al., 2016; Ren et al., 2018; Shi et al., 2018). During the Sinian, the Tarim Basin entered a thermal depression with the deposition of cold climate marine and ice ocean carbonates, clastic rocks, and moraine and volcanic clastic rocks (Deng et al., 2019).
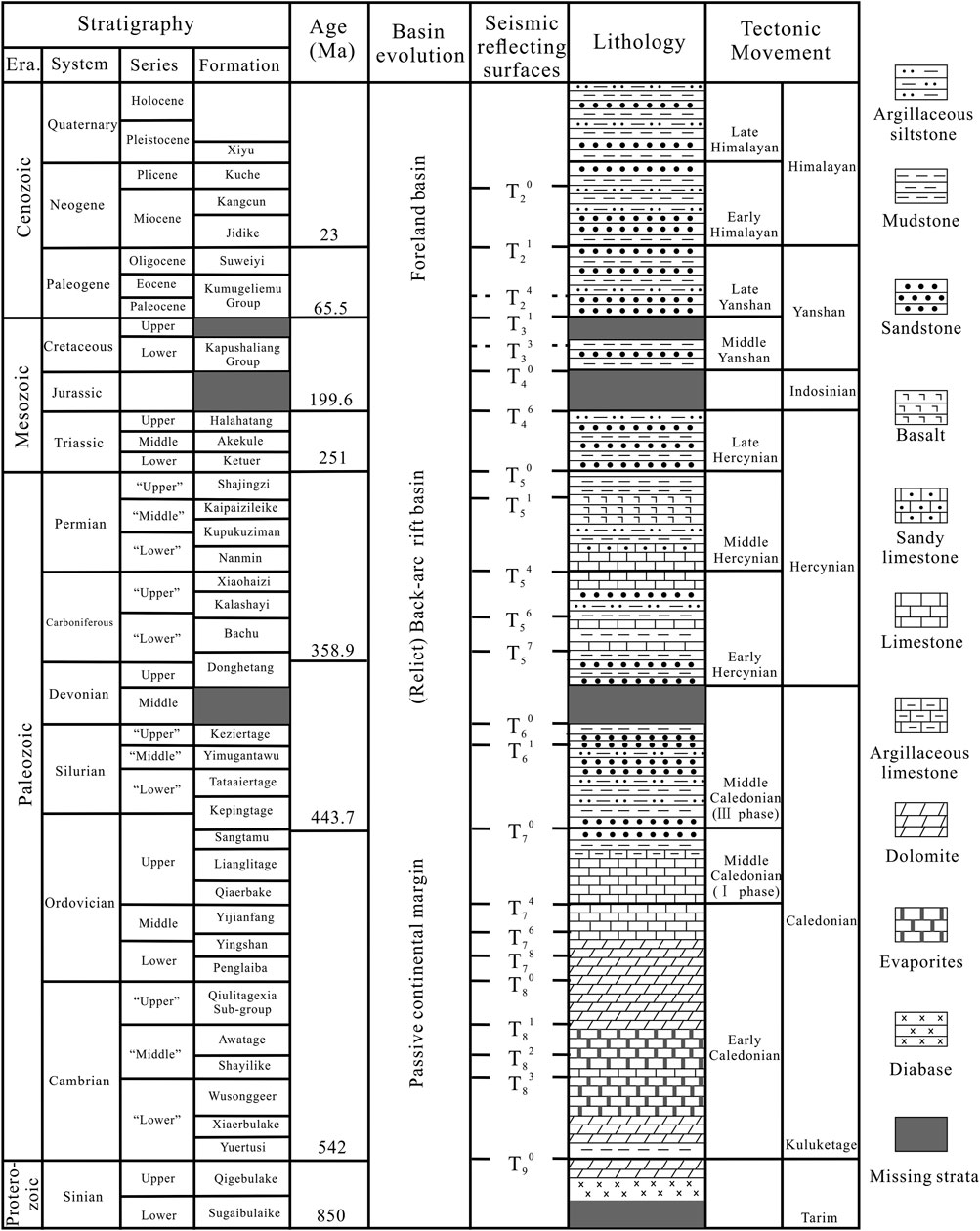
FIGURE 3. Generalized stratigraphy of the Shunbei 5 strike-slip deformation belt in Tarim Basin (Modified after Lu et al., 2015; Deng et al., 2019; Han et al., 2021).
During the Cambrian, the south and north margins were passive margins, and the inner basin was a successive basin with marine carbonates, mudstone, gypsum salt rocks, and siliceous mudstone. The marine carbonate sedimentation continued to the end of the Middle Ordovician when the first episode of the Caledonian movement occurred (Han et al., 2021). During the Upper Ordovician, marine clastic sediments with thick mudstone were deposited, developing suitable cap rocks (Lu et al., 2015). During the Silurian, marine clastic sediments were deposited. The late Caledonian movement at the end of the late Ordovician and the early Hercynian movement caused regional unconformity between the Silurian and Upper Devonian (Figure 3).
From the late Devonian to the Triassic, the tectonic regime of the Tarim Basin changed into margin subduction and collision with interactive marine and terrestrial deposits. The Upper Devonian and Carboniferous strata comprise marine clastic rocks. The Permian and Triassic strata comprise terrestrial clastic rocks and volcanic rocks (Figure 3). The Middle Hercynian movement occurred at the end of the Carboniferous, the late Hercynian movement at the end of the Permian, and the Indochina movement at the end of the Triassic.
From the Jurassic to the Oligocene, the Tarim Basin experienced inner plate tectonics after collision, with the deposition of terrestrial clastic rocks and gypsum salt rocks (Figure 3). The middle Yanshanian movement occurred at the end of the Jurassic, the late Yanshanian movement at the end of the Cretaceous, and the early Himalayan movement at the end of the Paleogene.
In the Neogene, the Tarim basin was a reactive foreland basin, with the deposition of terrestrial clastic rocks and gypsum salt rocks.
The Tarim Basin is rich in oil and gas resources, with estimated oil reserves of 59.94 × 108 t (Zhou et al., 2005), ordinary gas reserves of 117398.96 × 108 m3, and non-ordinary gas reserves of 3 × 1012 m3 (Zhou et al., 2005; Huang, 2019). Through extensive exploration works covering more than 30 years, the source rocks, structural styles, trap types, and reservoir model have been well established in three hydrocarbon-bearing systems, the Kuqa depression, platform basin area, and the southwest depression (Du et al., 2019).
Data and Methodology
The Shunbei-5 strike-slip deformation belt and its related structures were determined based on 2D and 3D seismic data provided by Sinopec between 2009 and 2016 (Figure 2). The geochronologic calibration of the seismic reflectors was performed by the Institute of Exploration and Development and Northwest Petroleum Bureau, Sinopec. The seismic data were interpreted in OpenWorks (Halliburton Landmark). Seismic coherence slices were used to identify the individual fault segments (Bahorich and Farmer, 1995). The coherence slices of surface T70 (stratigraphic boundary between the Kepingtage and Sangtamu Formations) and T74 (stratigraphic boundary between the Upper Ordovician and Middle Ordovician) were used to analyze the structural characteristics of faults and fault combinations.
A strike-slip deformation belt has various types of structures (Allen and Allen, 2013). Here, a composite strike-slip deformation belt is defined as a large-scale strike-slip deformation belt with multiphase activities, various mechanics, and directional structures both superposing in vertical sections and arraying in maps. Based on physical modeling of a wrench zone, en echelon tensional fractures (T-fracture) and shear fractures were identified (Riedel, 1929). Synthetic shears (R-shears) and antithetic shears (R'-shears) were defined as Riedel shears (Skempton, 1966; Hills, 2012). Other secondary structures in a wrench zone include P-shears, Y-shears, and convergent structures such as folds and reverse faults (Harding, 1974; Schreurs, 1994; Xu et al., 2017; Chen, 2020b; Chen, 2020a). Applying these methods and theories, the formation time and formation mechanism of SB5 were determined.
Structural Frame Work of the Shunbei 5 Strike-Slip Deformation Belt
The Shunbei 5 strike-slip formation belt (SB5) is located in the central part of the Tarim basin (Figure 1). In this study, emphasis was placed on the central part of SB5.
Usually, the wrench direction of a subsurface strike-slip deformation belt is determined by the arraying styles of faults (Figure 4; Ghosh and Chattopadhyay, 2008). Both right-stepping tensional faults and right-stepping R-shear faults indicate a sinistral strike-slip fault (Figures 4A,C). Both left-stepping tensional faults and left-stepping R-shear faults indicate a dextral strike-slip fault (Figures 4B,D). Regarding SB5, in the T70 reflector, the faults array in a right-stepping en echelon belt (Figures 5A,B, 6A,B. The echelon angles of the faults generally range from 14° to 35°, with some at 44° ± 5° (Figures 6A,B). Along the vertical profiles, the faults appear to be normal faults (T-fracture) (Figure 7, Figure 8). Considering Figures 4B,D, these faults were deduced to be R-shears, and they indicate a dextral transtensional strike-slip fault. In the T74 reflector, the faults array in complex patterns (Figures 5C,D, 6C,D). The echelon angles of the faults generally range from 16° to 32° (counterclockwise), and from 9° to 12° (clockwise), with some at 43° (Figures 6C,D), corresponding to R-shears, P-shears, and several spreading branches of R-shears, respectively (Figures 4B, 5). Nevertheless, these faults present the characteristics of dextral strike-slip faults on the coherence slices of surface T74, accompanied by alternating transtensional and compression zones.
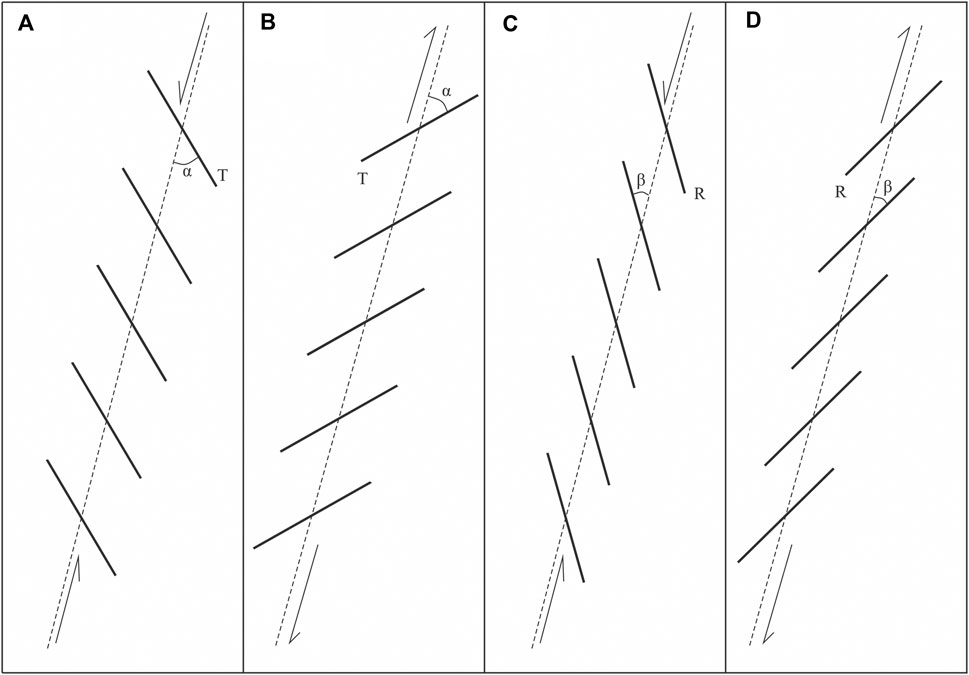
FIGURE 4. Wrench directions and fault arraying types. (A) Right-stepping tensional faults indicating a sinistral strike-slip fault system, (B) Left-stepping tensional faults indicating a dextral strike-slip fault, (C) Right-stepping R-shear faults indicating a sinistral strike-slip fault system, (D) Left-stepping R-shear faults indicating a dextral strike-slip fault system. α is the angle between the direction of principal displacement zone and the T fracture, and β is the angle between the direction of principal displacement zone and R-shears.
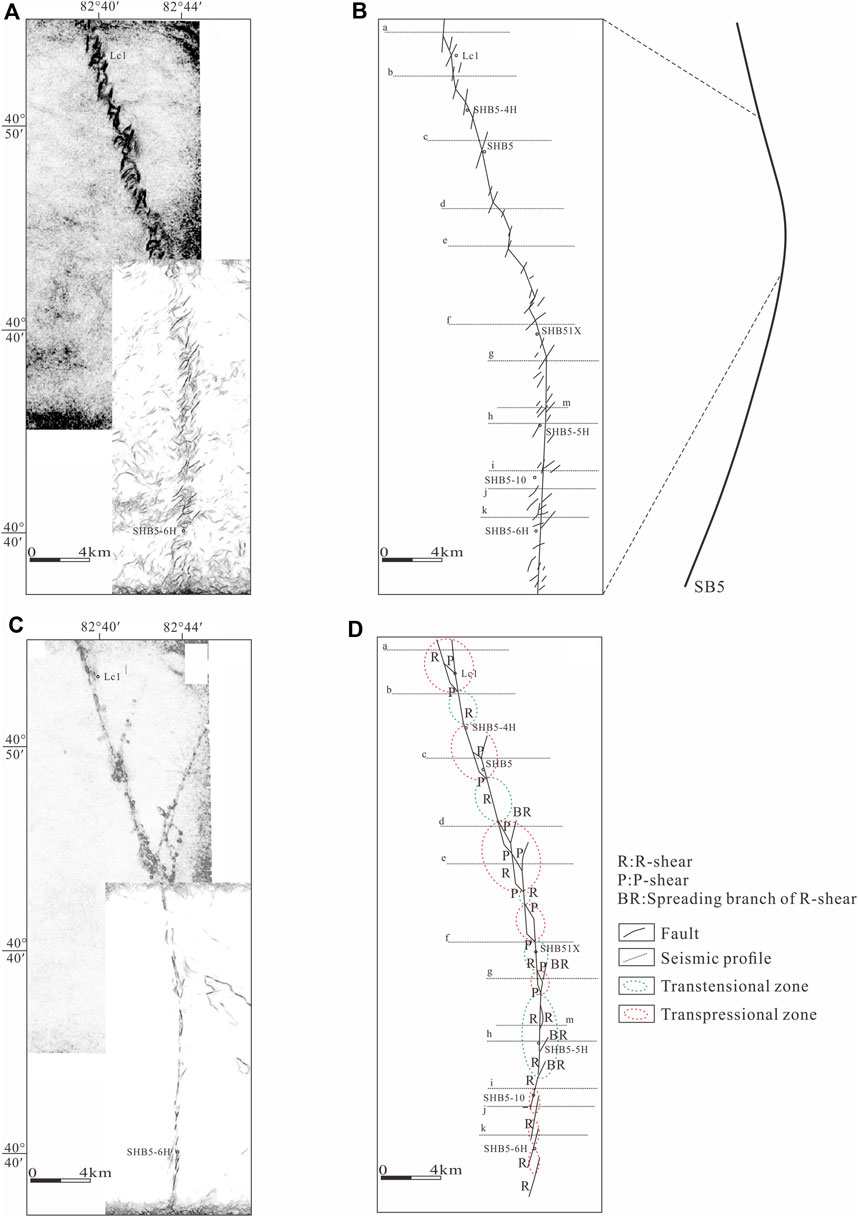
FIGURE 5. 3D coherence slice of the Shunbei 5 strike-slip deformation belt (SB5). (A) T70 reflector indicating the bottom of the Silurian, (B) Fault interpretation of a, (C) T74 reflector indicating the bottom of the Upper Ordovician, (D) Fault interpretation of c.
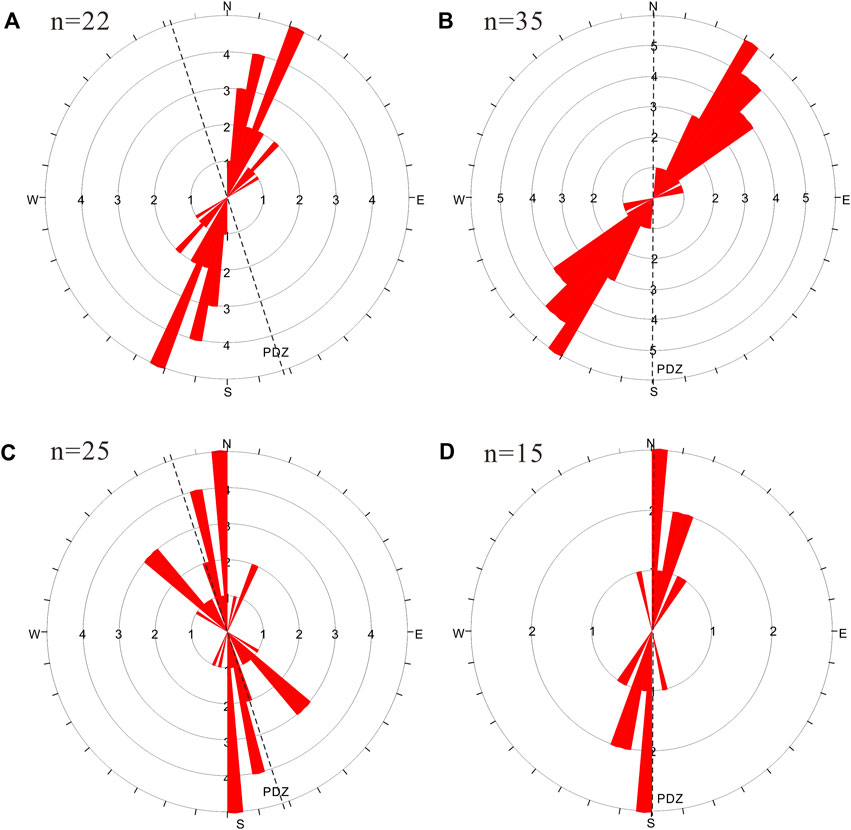
FIGURE 6. Strike-weighted rose diagrams for each fault set in the bottom of the Silurian (T70) and the bottom of the Upper Ordovician (T74). (A) The north segment of SB5 in T70; (B) The south segment of SB5 in T70; (C) The north segment of SB5 in T74; (D) The south segment of SB5 in T74. PDZ = principal displacement zone. The boundary between the northern and southern segments of SB5 is the turning pint of SB5 in Figure 5B.
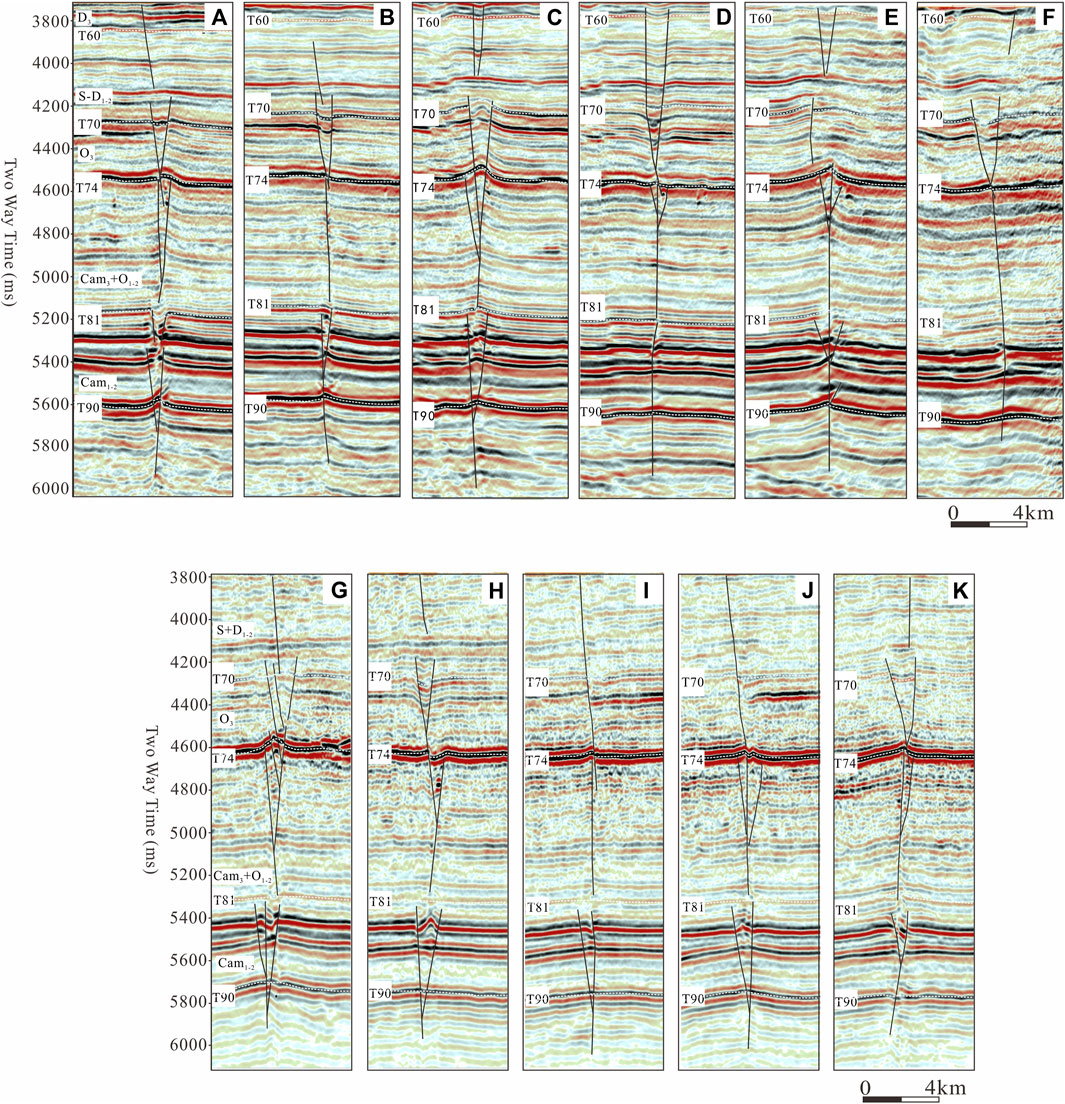
FIGURE 7. 3D seismic profiles of the Shunbei 5 strike-slip deformation belt (SB5) (see Figure 6 for locations).
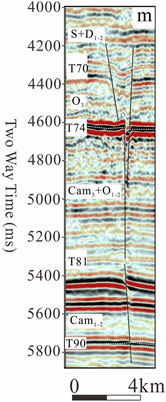
FIGURE 8. 3D seismic profile of a transtensional part of an R-shear (see Figure 6 for location).
Although strike-slip deformation belts are complex, those with a small slip distance would have a simple structure. In general, the fault mechanics of strike-slip deformation belts are determined by the mechanical properties of the rocks (Chen, 2020b). These faults can form various structural assemblages along the horizontal plane. Figure 9 shows a dextral simple shear with a small slip distance as an example. Both physical modeling and numerical simulations such as PFC calculation indicate that R-shears commonly occur earlier, followed by P-shears and Y-shears (Cho et al., 2008). Y-shears occur parallel to the main displacement zone, such as P1 and P11 in Figure 9. P-shears occur in the oversteps of R-shears, being the boundaries of convergent bends such as P3. Central parts of R-shears appear to be transtensional, such as P2. Direct slip will occur at the transitional zone between R-shears and P-shears, such as P7. In SB5, transpressional structures formed at the oversteps (Figure 5D). Transpressional structures are characterized by reverse faults or folds in seismic profiles, as shown in Figures 7A,C,E,G,I–K. Transtensional structures formed in the central part of the R-shears (Figure 6). Transtensional structures are characterized by normal faults or grabens in seismic profiles, as shown in Figures 7B,F,H. Parallel normal faults were observed in the T74 reflector along an R-shear (Figures 6D, 9). The fault arraying patterns were identical to those shown in Figure 4. A total of eight transpressional zones and five transtensional zones were identified in SB5, alternating with each other.
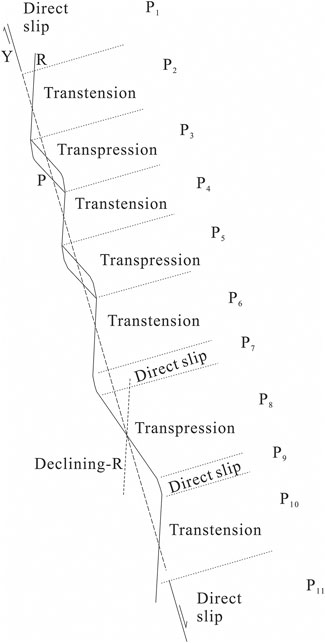
FIGURE 9. Structures in a dextral strike-slip fault deformation belt with one-time activity and a small strike-slip distance.
Time and Mechanism of the Shunbei 5 Strike-Slip Deformation Belt
Due to the differences in the thicknesses and mechanical properties of rocks, various structural assemblages may superpose on vertical sections to form composite superposition relationships. Consequently, the formation time of wrench formation becomes difficult to determine. Although structural assemblages on vertical sections are very complex, the wrench time can be determined from the strata cut by the strike-slip faults. As strata mechanics and rock thicknesses vary, the same tectonic movement may lead to different structural styles in various strata. In other words, various strata may exhibit different structures along the vertical profile. As shown in Figure 10A, these structures have clear boundaries between them, but these were correspond to the same formation time. A1 is a superposition of a compressive structure (e.g., a fold) overlain by extensive structures (e.g., normal faults), A2 a superposition of normal faults overlain by a compressive fold, A3 a superposition of normal faults overlain by other normal faults, and A4 a superposition of a compressive fold overlain by other compressive fold and reverse faults. If various structures with different mechanical properties occur in a layer boundary, they correspond to two tectonic movements (Figure 10B). B1 is a superposition of first reverse faults interspersed by secondary normal faults, B2 a superposition of first normal faults interspersed by secondary reverse faults, B3 a superposition of first normal faults interspersed by other secondary normal faults, and B4 a superposition of first reverse faults interspersed by other secondary reverse faults.
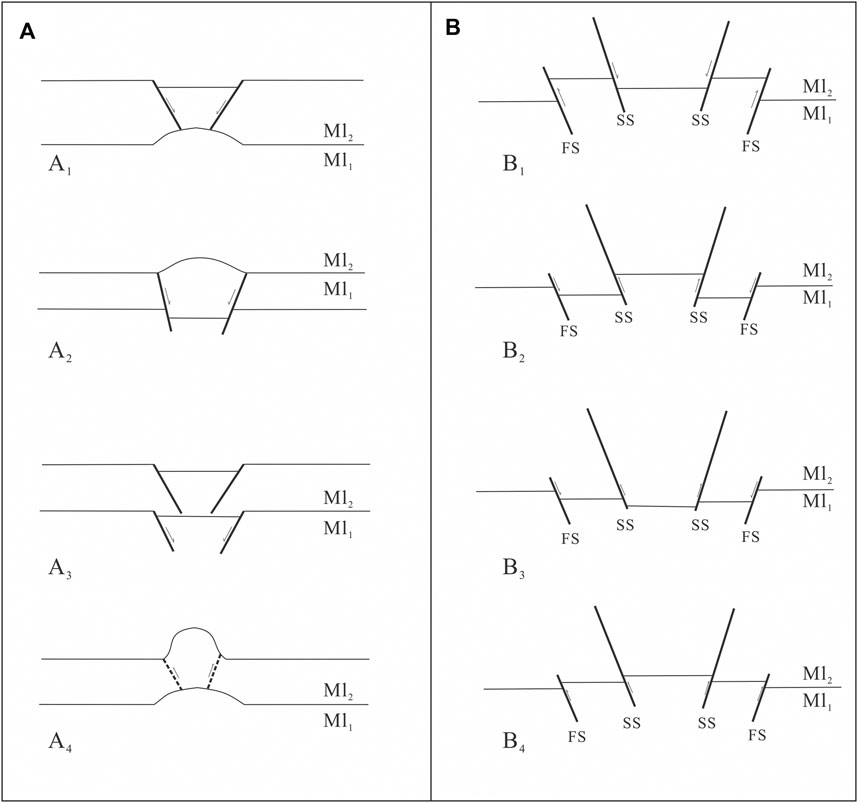
FIGURE 10. Possible vertical superposition of wrench related structures in various strata. (A) Structures formed in one movement, (B) Structures formed in two movements; Ml1-Mechanical layer 1, Ml2-Mechanical layer 2, FS-First time structure, SS-Second time structure
The formation time should be determined based on the strata cut by wrench related faults, unconformities, and arraying patterns of the wrench related structures. In Figures 7, 8, each profile exhibits different structural styles from deep to shallow areas. Through the superposition of structures from the T90 and T74 reflectors, the following patterns could be identified: transpressional–transtensional–transpressional (Figure 7A), transpressional–transtensional (Figure 7B), transpressional–transpressional (Figure 7C), transtensional–transtensional (Figure 7D), transpressional–transtensional–transpressional (Figure 7E), transtensional (Figure 7F), transtensional–transpressional (Figure 7G), transpressional–transtensional (Figure 7H), transtensional–transpressional (Figure 7I), transpressional– transpressional (Figure 7J), and transtensional–transpressional (Figure 7K). Regarding Figure 8, the superposition reveals a transtensional shear. These structures were delimited by layer beddings, and referring to Figure 10A, they were deduced to be formed at the end of the Middle Ordovician. This time is identical to the age of the first periodic calcite vein in fractures (Wu et al., 2020; Wang et al., 2021). Except for the transpressional structures in Figures 7C,E, the structures in the T70 reflector were mostly transtensional zones, appearing as en echelon patterns on the plan view (Figure 5B) and normal faults in vertical profiles (Figures 7, 8). The normal faults cut into the T74 reflector, and referring to Figure 10B, their formation time was found to be different, which is the end of the Ordovician. This time is identical with the age of another penetrative calcite vein in fractures (Yang et al., 2020). As mentioned above, SB5 underwent several times of activities after the two times of activities (Figures 2, 7, 8).
The formation of the SB5 strike-slip fault is related to plate tectonics around the Tarim Plate (basin). The activity at the end of the Middle Ordovician was caused by the near N-S compression, which is related to the closing of the Proto-Tethys Ocean (Wu et al., 2020), during which the SB5 strike-slip fault was a simple shear belt. The activity at the end of the late Ordovician is related to the southward subduction of the Northern Kunlun Ocean, the northwestward subduction of the Altyn Ocean and the southward subduction of the Northeast Tianshan-Junggar Ocean (Wu G. H. et al., 2016; Wu et al., 2020), during which the SB5 strike-slip fault was a transtensional zone. From the Silurian to Middle Devonian, the east Kunlun-Qaidam Plate and middle Kunlun terrain attached to the Tarim Plate, causing the closing of the Altyn Ocean and the Northern Kunlun Ocean, respectively. At the end of the Permian, the north Tianshan-Junggar Ocean and the south Tianshan Ocean closed, making the Tarim Plate become a part of the Eurasian Plate. At the same time, the southern part of the Tarim Plate changed from a passive margin into an active margin with volcanic arcs. Collisions occurred between the Tianshuihai terrain and the Tarim Plate, and between the Qiangtang terrain and the Eurasian Plate in the early Triassic and at the end of the Triassic, respectively (Hendrix et al., 1992; Graham et al., 1993; Pan et al., 1997). In the Jurassic, the Tethys Ocean Plate was subducted beneath the Qiangtang Plate. With the Lasa collision (140–125 Ma), the Lasa Plate attached to the Qiangtang Plate. In the late Cretaceous, the Kexisitan Plate collided the Lasa Plate at the southwest part (Hendrix et al., 1992; Graham et al., 1993), causing local erosion. The Himalayan collision occurred in the middle-late Cenozoic (45 Ma), attaching the Indian Plate to the Eurasian plate and closing the Tethys Ocean. This collision caused an extensive N-S compression.
Control of the Shunbei 5 Strike-Slip Deformation Belt on the Formation of the Oil Reservoir
The SB5 reservoir is a typical reservoir with a total oil production of 2.86 × 104 t (Figure 11).
Although there are four sets of source rocks in the Tarim basin, source analysis proved that the oil and gas in the Shunbei oilfield are contained in the source rock at the bottom of the Cambrian strata (Song et al., 2016; Gao et al., 2018; Ma et al., 2018; Gu et al., 2020; Chen et al., 2021). Analyses of the physical and geochemical properties and fluid inclusions in rocks indicate that the time of the first hydrocarbon charging in the Shunbei oilfield was in the late Silurian, following which several charging events might have occurred (Wang et al., 2019; Cao et al., 2020; Wang et al., 2020). The time of the first hydrocarbon charging agrees with the hydrocarbon expulsion peak in the Tarim Basin (Wang et al., 2020).
The reservoir lies in the Middle Ordovician stratum overlain by Upper Ordovician marl and mudstone, which serve as the cap rocks (Figure 11). The source rock is the base of the Lower Cambrian. The Middle Cambrian includes gypsum and salt rock formations, which are suitable cap rocks (Chen et al., 2021). SB5 played an important role both in the hydrocarbon migration from the Cambrian source rock to the reservoir and in the formation of the Ordovician reservoirs. Firstly, a strike-slip fault can cut deep to connect deep source rocks with shallow reservoirs. Normal faults and thrust faults may cut along a detachment, similar to salt layers, but they cannot penetrate deep source rocks. A typical strike-slip fault alternates between transtensional and transpressional areas; the former provide suitable migration paths and the latter form suitable traps. Transtensional areas have been found to offer higher oil output per unit pressure drop than transpressional areas (Liu, 2020). Secondly, wrenching improves the porosity and permeability of rocks. In the Shunbei oilfield, the accumulation spaces are fractures and caves; the former is related to the wrenching and the latter is related to carbonate rock corrosion along the strike-slip faults (Jiao, 2018; Qi, 2019; Lv et al., 2021).
Conclusion
The Shunbei 5 strike-slip deformation belt (SB5) of the Shunbei area of the central Tarim Basin is an important intracratonic strike-slip fault system with high potential for oil and gas exploration. Based on detailed structure characterization and geometric analysis, the tectonic architecture and structural evolution of SB5 were determined and proposed, respectively. Moreover, the control of the strike-slip deformation belt on the formation of oil and gas reservoirs is discussed. The main findings can be summarized as follows:
1) The Shunbei 5 strike-slip deformation belt (SB5) was formed at the end of the Middle Ordovician with the closing of the proto-Tethys Ocean, during which it was a dextral parallel strike-slip fault belt comprising R-shears and P-shears. In the plan view, transpressional and transtensional structures are alternately arranged. Along the vertical profile, the lithological strata exhibit different structural styles, attributable to the varying mechanisms.
At the end of the late Ordovician, SB5 was affected by the southward subduction of the Northern Kunlun Ocean, the northwestward subduction of the Altyn Ocean, and the southward subduction of the Northeast Tianshan-Junggar Ocean, during which it was a transtensional dextral strike-slip fault belt. The structures were left-stepping R-shears with transextension in the progressive deformation.
2) SB5 cut through the Middle Cambrian gypsum salt layer to connect the Lower Cambrian source rock with the Ordovician reservoirs, leading to the formation of the oil and gas reservoirs of the Shunbei oilfield.
3) A simple shear strike-slip deformation belt with a small displacement may consist mainly of R-shears. The oversteps may include transpressional structures with P-shears. Outside the oversteps, the main structures may be transtensional. Moreover, the transition of the R-shears and P-shears may exhibit a parallel pattern. Even with one tectonic movement, various rocks may exhibit various structures with different mechanical properties according to differences in their mechanical properties. This finding may facilitate a deeper understanding of other strike-slip deformation belts around the world.
Data Availability Statement
The original contributions presented in the study are included in the article/Supplementary Material, further inquiries can be directed to the corresponding authors.
Author Contributions
HY and SC presented the idea and design of the research. HY and SC wrote the article and led the data analysis and interpreted the results with YN, HZ, SX, XW and GF. All authors contributed to the original article revision and approved it for publication.
Funding
This study was jointly funded by the “National Key Research and Development Plan-ultra-deep Layer, Mesoproterozoic and Neoproterozoic Cap Sealing Property and Oil-gas Preservation Mechanism” (no.2017YFC0603105), the “Mechanism of Deep Hydrocarbon Migration and Enrichment in Key Areas of Sichuan Basin” (no.XDA14010306), and the “Development in Large-scale Oil-gas Field and Coalbed Methane Project”-“Reservoir Formation Conditions and Controlling Factors in Deep-ultra-deep Cambrian in Tarim Basin” (no. 2017ZX05005-002-005).
Conflict of Interest
The authors declare that the research was conducted in the absence of any commercial or financial relationships that could be construed as a potential conflict of interest.
Publisher’s Note
All claims expressed in this article are solely those of the authors and do not necessarily represent those of their affiliated organizations, or those of the publisher, the editors, and the reviewers. Any product that may be evaluated in this article, or claim that may be made by its manufacturer, is not guaranteed or endorsed by the publisher.
References
Allen, P. A., and Allen, J. R. (2013). Basin Analysis: Principles and Application to Petroleum Play Assessment. New Jersey: John Wiley & Sons.
Bahorich, M., and Farmer, S. (1995). 3-D Seismic Discontinuity for Faults and Stratigraphic Features: The Coherence Cube. Leading edge 14 (10), 1053–1058. doi:10.1190/1.1437077
Cao, Z., Lu, Q., Gu, Y., Wu, X., You, D., and Zhu, X. (2020). Characteristics of Ordovician Reservoirs in Shunbei 1 and 5 Fault Zones, Tarim Basin. Oil Gas Geol. 41 (5), 975–984. doi:10.11743/ogg20200508
Chen, S., Xu, S., Cai, Y., and Ma, X. (2018). Wrench Related Faults and Their Control on the Tectonics and Eocene Sedimentation in the L13-L15 Sub-sag Area, Pearl River Mouth basin, China. Mar. Geophys. Res. 39 (3), 363–381. doi:10.1007/s11001-017-9328-1
Chen, S., Wang, Z., Yang, W., Wang, Y., and Yuan, H. (2021). Geological Background of Early Cambrian in Tarim Basin and its Control on the Development of Source Rocks. Sci. Technol. Eng. 21 (1), 124–129.
Chen, S. (2020a). On the Occurrences of Fractures in Wrench Zones. Wjm 10 (03), 27–38. doi:10.4236/wjm.2020.103003
Chen, S. (2020b). On the Orientation of Fractures with Transpressional and Transtensional Wrenches in Pre-existing Faults. Wjm 10 (11), 199–209. doi:10.4236/wjm.2020.1011014
Chinnery, M. A. (1963). The Stress Changes that Accompany Strike-Slip Faulting. Bull. Seismol. Soc. Am. 53 (5), 921–932. doi:10.1785/bssa0530050921
Cho, N., Martin, C. D., and Sego, D. C. (2008). Development of a Shear Zone in Brittle Rock Subjected to Direct Shear. Int. J. Rock Mech. Min. Sci. 45 (8), 1335–1346. doi:10.1016/j.ijrmms.2008.01.019
Deng, S., Li, H., Zhang, Z., Wu, X., and Zhang, J. (2018). Characteristics of Differential Activities in Major Strike-Slip Fault Zones and Their Control on Hydrocarbon Enrichment in Shunbei Area and its Surroundings, Tarim Basin. Oil Gas Geol. 39 (5), 878–888. doi:10.11743/ogg20180503
Deng, S., Li, H., Zhang, Z., Zhang, J., and Yang, X. (2019). Structural Characterization of Intracratonic Strike-Slip Faults in the central Tarim Basin. Bulletin 103 (1), 109–137. doi:10.1306/06071817354
Du, J., Tian, J., Guoxin, L., Haijun, Y., Yijie, Z., Yong, L., et al. (2019). Strategic Breakthrough and prospect of Qiulitag Structural belt in Kuqa Depression. China Pet. Explor. 24 (1), 16. doi:10.3969/j.issn.1672-7703.2019.01.003
El Ghamry, M. N., El Amawy, M., and Hagag, W. (2020). The Role of Late Cretaceous Wrench Tectonics in Hydrocarbon Endowment in El-Gindi Basin, Northern Western Desert, Egypt. Mar. Pet. Geol. 112, 104093. doi:10.1016/j.marpetgeo.2019.104093
Gao, X., Wu, X., Hong, C., and Wen, S. (2018). Geochemical Characteristics of Ordovician Crude Oil in the No. 1 Fault Zone of Shunbei Oilfield. Pet. Geol. Eng. 36, 37–40.
Ghosh, N., and Chattopadhyay, A. (2008). The Initiation and Linkage of Surface Fractures above a Buried Strike-Slip Fault: An Experimental Approach. J. Earth Syst. Sci. 117 (1), 23–32. doi:10.1007/s12040-008-0009-y
Graham, S. A., Hendrix, M. S., Wang, L. B., and Carroll, A. R. (1993). Collisional Successor Basins of Western China: Impact of Tectonic Inheritance on Sand Composition. Geol. Soc. Am. Bull. 105 (3), 323–344. doi:10.1130/0016-7606(1993)105<0323:csbowc>2.3.co;2
Gu, R., Yun, L., Zhu, X., and Zhu, M. (2020). Oil and Gas Sources in Shunbei Oilfield, Tarim Basin. Pet. Geol. Exp. 42 (2), 248–262. doi:10.11781/ssydz202002248
Han, X., Deng, S., Tang, L., and Cao, Z. (2017). Geometry, Kinematics and Displacement Characteristics of Strike-Slip Faults in the Northern Slope of Tazhong Uplift in Tarim Basin: A Study Based on 3D Seismic Data. Mar. Pet. Geol. 88, 410–427. doi:10.1016/j.marpetgeo.2017.08.033
Han, J., Kang, A., Neng, Y., Huang, C., Li, Q., Chen, P., et al. (2021). Vertical Layered Structure of Shunbei No. 5 Strike-Slip Fault Zone and its Significance on Hydrocarbon Accumulation. Xinjiang Pet. Geol. 42 (2), 152. doi:10.7657/XJPG20210204
Harding, T. (1974). Petroleum Traps Associated with Wrench Faults. AAPG Bull. 58 (7), 1290–1304. doi:10.1306/83d91669-16c7-11d7-8645000102c1865d
Hendrix, M. S., Graham, S. A., Carroll, A. R., Sobel, E. R., McKNIGHT, C. L., Schulein, B. J., et al. (1992). Sedimentary Record and Climatic Implications of Recurrent Deformation in the Tian Shan: Evidence from Mesozoic Strata of the north Tarim, South Junggar, and Turpan Basins, Northwest China. Geol. Soc. Am. Bull. 104 (1), 53–79. doi:10.1130/0016-7606(1992)104<0053:sracio>2.3.co;2
Hills, E. S. (2012). Elements of Structural Geology. Wilmersdorf: Springer Science & Business Media.
Huang, C. (2019). Multi-stage Activity Characteristics of Small-Scale Strike-Slip Faults in Superimposed basin and its Identification Method: a Case Study of Shunbei Area. Pet. Geol. Exp. 41 (3), 379–389. doi:10.11781/sysydz201903379
Jiao, F. (2018). Significance and prospect of Ultra-deep Carbonate Fault-Karst Reservoirs in Shunbei Area, Tarim Basin. Oil Gas Geol. 39 (2), 207–216. doi:10.11743/ogg20180201
Li, Y., Qi, L., Zhang, S., Yun, L., Cao, Z., Han, J., et al. (2019). Characteristics and Development Mode of the Middle and Lower Ordovician Fault-Karst Reservoir in Shunbei Area, Tarim basin. Acta Petrolei Sin. 40, 1470–1484. doi:10.7623/syxb201912006
Liu, B. (2020). Analysis of the Main Controlling Factors of Oil and Gas Differential Accumulation in the Shunbei Area, Tarim Basin-taking Shunbei No. 1 and No. 5 Strike-Slip Fault Zones as Examples. China Pet. Explor. 25 (3), 83. doi:10.3969/j.issn.1672-7703.2020.03.008
Lu, X., Hu, W., Wang, Y., Li, X., Li, T., Lyu, Y., et al. (2015). Characteristics and Development Practice of Fault-Karst Carbonate Reservoirs in Tahe Area, Tarim Basin. Oil Gas Geology. 36 (3), 347–355. doi:10.11743/ogg20150301
Lv, H., Han, J., Zhang, J., Liu, Y., and Li, Y. (2021). Development Characteristics and Formation Mechanism of Ultra-deep Carbonate Fault-Dissolution Body in Shunbei Area, Tarim Basin. Pet. Geol. Exp. 43 (1), 14–22. doi:10.l781/sysydz202101014
Ma, A., Zhu, C., Gu, Y., and Li, H. (2018). Concentrations Analysis of Lower Thiadiamondoids of Cambrian Oil from Well Zhongshen 1C of Tazhong Uplift, Tarim Basin, NW China. Nat. Gas Geosci. 29 (7), 1009–1019. doi:10.11764/j.issn.1672-1926.2018.06.007
Pan, G., Zl, C., and Xz, L. (1997). Geological–Tectonic Evolution in the Eastern Tethys. Beijing: Geological Publishing House, 218. (in Chinese with English abstract).
Qi, L. (2019). Characteristics and Inspiration of Ultra-deep Fault-Karst Reservoir in the Shunbei Area of the Tarim Basin. China Pet. Exploration 25 (1), 102–111.
Ren, R., Guan, S., Wu, L., and Zhu, G. (2018). Evolution of the Neoproterozoic Rift Basins and its Implication for Oil and Gas Exploration in the Tarim Basin. Pet. Res. 3 (1), 66–76. doi:10.1016/j.ptlrs.2018.03.003
Riedel, W. (1929). Zur Mechanik geologischer Brucherscheinungen ein Beitrag zum Problem der Fiederspatten. Zentbl. Miner. Geol. Palaont. Abt., 354–368.
Schreurs, G. (1994). Experiments on Strike-Slip Faulting and Block Rotation. Geol 22 (6), 567–570. doi:10.1130/0091-7613(1994)022<0567:eossfa>2.3.co;2
Shi, K. B., Liu, B., Tian, J. C., and Pan, W. Q. (2016). Sedimentarycharac teristicsandlithofaciespaleogeographyofSinianinTarimBasin. Acta Petrolei Sin. 37 (11), 1343–1360. doi:10.7623/syxb201611003
Shi, K., Liu, B., Jiang, W., Luo, Q., and Gao, X. (2018). Nanhua-Sinian Tectono-Sedimentary Framework of Tarim Basin, NW China. Oil Gas Geology. 39 (5), 862–877. doi:10.11743/ogg20180502
Skempton, A. (1966). “"Some Observations on Tectonic Shear Zones,” in 1st ISRM Congress: International Society for Rock Mechanics and Rock Engineering. New Jersey: Springer International Publishing.
Song, D., Wang, T., and Li, M. (2016). Geochemistry and Possible Origin of the Hydrocarbons from Wells Zhongshen1 and Zhongshen1C, Tazhong Uplift. Sci. China Earth Sci. 59 (4), 840–850. doi:10.1007/s11430-015-5226-z
Sylvester, A. G. (1988). Strike-slip Faults. Geol. Soc. Am. Bull. 100 (11), 1666–1703. doi:10.1130/0016-7606(1988)100<1666:ssf>2.3.co;2
Wang, Y., Chen, H., Guo, H., Zhu, Z., Wang, Q., Yu, P., et al. (2019). Hydrocarbon Charging History of the Ultra-deep Reservoir in Shun 1 Strike-Slip Fault Zone, Tarim Basin. Oil Gas Geol. 40 (5), 972–989.
Wang, B., Zhao, Y., He, S., Guo, X., Cao, Z., Deng, S., et al. (2020). Hydrocarbon Accumulation Stages and Their Controlling Factors in the Northern Ordovician Shunbei 5 Fault Zone, Tarim Basin. Oil Gas Geol. 41 (5), 965–974. doi:10.11743/ogg20200507
Wang, B., Yang, Y., Cao, Z., He, S., Zhao, Y., Guo, X., et al. (2021). U-pb Dating of Calcite Veins Developed in the Middle-Lower Ordovician Reservoirs in Tahe Oilfield and its Petroleum Geologic Significance in Tahe Oilfield. Earth Sci. doi:10.3799/dakx.2020.352
Wilcox, R. E., Harding, T. T., and Seely, D. R. (1973). Basic Wrench Tectonics. AAPG Bull. 57 (1), 74–96. doi:10.1306/819a424a-16c5-11d7-8645000102c1865d
Wu, G., Yang, H., Qu, T., Li, H., Luo, C., and Li, B. (2012). The Fault System Characteristics and its Controlling Roles on marine Carbonate Hydrocarbon in the Central Uplift, Tarim basin. acta Petrol. Sin. 28 (3), 793–805.
Wu, G. H., Pang, X. Q., and Li, Q. M. (2016a). The Structural Characteristics of Carbonate Recks and Their Effects on Hydrocarbon Exploration in Craton Basin: A Case Study of the Tarim Basin. Beijing: Science Press.
Wu, L., Guan, S., Ren, R., Wang, X., Yang, H., Jin, J., et al. (2016b). The Characteristics of Precambrian Sedimentary basin and the Distribution of Deep Source Rock: A Case Study of Tarim Basin in Neoproterozoic and Source Rocks in Early Cambrian, Western China. Pet. Exploration Dev. 43 (6), 988–999. doi:10.1016/s1876-3804(16)30116-1
Wu, G., Yuan, Y., Huang, S., Vandyk, T. M., Xiao, Y., Cai, Q., et al. (2018). The Dihedral Angle and Intersection Processes of a Conjugate Strike-Slip Fault System in the Tarim Basin, NW China. Acta Geol. Sin. - English Ed. 92 (1), 74–88. doi:10.1111/1755-6724.13495
Wu, G., Ma, B., Han, J., Guan, B., Chen, X., Yang, P., et al. (2020). Origin and Growth Mechanisms of Strike-Slip Faults in the central Tarim Cratonic basin, NW China. Petroleum Exploration & Development 48 (3), 595–607. doi:10.1016/S1876-3804(21)60048-4
Xu, S., Peng, H., Angel, F., Nieto-Samaniego, C., SP, , and Wu, X. (2017). The Similarity between Riedel Shear Patterns and Strike-Slip Basin Patterns. Geol. Rev. 63, 287–300.
Yang, Y., Wang, B., Cao, Z., Huang, C., Zhao, Y., Guo, X., et al. (2020). Genesis and Formation Time of Calcite Veins of the Middle-Lower Ordovician Reservoirs in the Northern Shuntuoguole Low-Uplift, Tarim Basin. Earth Sci. 46 (6), 2246–2257. doi:10.3799/dqkx.2020.200
Zhen, S., Tang, L., Li, Z., Li, M., Cao, Z., and Yang, S. (2015). The Characteristics, Formation and Petroleum Geology Significance of the Strike-Slip Fault System in Shunnan Area, Northern Slope of Tazhong Uplift. Nat. Gas Geosci. 26 (12), 2315–2324. doi:10.11764/j.issn.1672-1926.2015.12.2315
Keywords: strike-slip deformation belt, structural style, interpretation model, oil and gas reservoir, Tarim Basin
Citation: Yuan H, Chen S, Neng Y, Zhao H, Xu S, Wang X and Feng G (2021) Composite Strike-Slip Deformation Belts and Their Control on Oil and Gas Reservoirs: A Case Study of the Northern Part of the Shunbei 5 Strike-Slip Deformation Belt in Tarim Basin, Northwestern China. Front. Earth Sci. 9:755050. doi: 10.3389/feart.2021.755050
Received: 07 August 2021; Accepted: 17 September 2021;
Published: 30 September 2021.
Edited by:
Wenhui Song, China University of Petroleum (Huadong), ChinaReviewed by:
Jia Lu, China University of Geosciences, ChinaMianmo Meng, China University of Petroleum (Huadong), China
Copyright © 2021 Yuan, Chen, Neng, Zhao, Xu, Wang and Feng. This is an open-access article distributed under the terms of the Creative Commons Attribution License (CC BY). The use, distribution or reproduction in other forums is permitted, provided the original author(s) and the copyright owner(s) are credited and that the original publication in this journal is cited, in accordance with accepted academic practice. No use, distribution or reproduction is permitted which does not comply with these terms.
*Correspondence: Haowei Yuan, yuanatcugb@163.com; Shuping Chen, csp21c@163.com