- 1Institute of Geography, Pedagogical University of Krakow, Cracow, Poland
- 2Institute of Geography, Slovak Academy of Sciences, Bratislava, Slovakia
Geodiversity is the natural diversity of features of geological structure, relief, and soil cover, including the relationships between these features, their properties, and their impact on other elements of the natural and cultural environment. It is described and analyzed using various types of quantitative, qualitative, or quantitative–qualitative methods. The concept of a geodiversity map presented in this article belongs to the third of these groups of methods. Despite the use of optimization methods in the form of a hexagon grid or the analytic hierarchy process calculator, it still remains partially subjective. The use of this method to calculate the geodiversity of an entire province (the Western Carpathians) gives a general view of the natural diversity of this area and allows regions to be selected for more detailed analyses or comparisons to be made between them. The geodiversity map is also a very good background on which to illustrate geotourist potential, which is expressed in terms of the number and distribution of geosites. However, in the case of the Western Carpathians, these two variables do not correlate with each other.
Introduction
The concept of geodiversity appeared in the literature in the 1990s and very quickly became popular among scientists around the world (e.g., Dixon, 1996; Kozłowski, 1997, 2004; Kostrzewski, 1998, 2011; Zwoliński, 2004; Gray, 2004, 2005, 2018a; Serrano and Ruiz-Flaño, 2007a; Gordon et al., 2012; Najwer and Zwoliński, 2014). It is defined in several contexts: geological, as the natural diversity of features of geological structure (rocks, minerals, and fossils), relief (forms and processes), and soil cover, including the relationships between these features, their properties, and their impact on other elements of the natural and cultural environment (Gray, 2004, 2013; Zwoliński, 2004); geological and anthropogenic, as the natural variation of the Earth’s surface, including geological and geomorphological forms which was formed by endo- and exogenous processes and human activity (Kozłowski et al., 2004a; 2004b); geographical, in which geodiversity is defined as the diversity of geocomplexes and geocomponents in a studied area, i.e., landscape diversity (Kostrzewski, 1998; Mizgajski, 2001); and anthropocentric, in which geodiversity is defined as the diversity of abiotic nature in terms of its ecological, economic, social, and esthetic significance to humans (Guthrie, 2005). It can also be used as an element of sustainable development (e.g., Mizgajski, 2001; Panizza, 2009; Gray, 2018a; Herrera-Franco et al., 2020) or holistically as the diversity of all abiotic elements of nature that are the basis of biodiversity. Biodiversity and geodiversity together create ecodiversity (e.g., Jedicke, 2001; Naveh, 2007; Braun et al., 2019; Halvorsen et al., 2020).
It is assumed that geodiversity is the variability of the components of the relief, lithosphere, hydrosphere, pedosphere, and atmosphere and is understood as a combination of landscape and man and products of his culture (Stanley, 2004). According to the Australian Heritage Commission (AHC, 2002), geodiversity is the natural diversity of geological, geomorphological, and deposition objects and their assemblages, ecosystems, and natural and anthropogenic processes. The most complex definition and significance of geodiversity in geoconservation were created by Gray (2013).
In the scientific literature, geodiversity is associated with geoheritage as a value-neutral concept describing the diversity of inanimate nature found on Earth, while geoheritage is a value-laden concept used to identify all geodiversity components selected for geoconservation (Brilha, 2018). Geoconservation includes the protection of geoheritage elements (Gordon, 2019). Moreover, effective conservation management can have positive social and ecological effects and bring prosperity to people living near protected areas (Abukari and Mwalyosi, 2020).
Any human activity that relates directly to the natural heritage should operate on the basis of the principles of geoethics, which includes research on the values that underlie appropriate human behavior and practices in relation to the Earth system (Peppoloni and Di Capua, 2015, 2015, 2016, 2017; Peppoloni et al., 2015; Antić et al., 2020). This definition clearly emphasizes the relationship of man with the environment and emphasizes the importance of conscious human activity in this relationship in accordance with the basic principles of ethics (Peppoloni and Di Capua, 2016; Antić et al., 2020). Therefore, the concept of geoheritage and geodiversity in relation to geotourism must be based on these principles.
More and more often, geodiversity is becoming the backbone of assessment of forms of abiotic nature, which are used as geohabitats in the creation of new tourist (geotourist) products. In the literature, it is usually a component of the assessment of geosites (Gray, 2018b).
According to the authors of this article, the assessment of geodiversity of a given area may be the first step to a more detailed analysis of selected parts of mountain ranges and their assessment for geotouristic purposes. A number of quantitative and qualitative methods of geodiversity assessment developed in the literature (e.g., Zwoliński et al., 2018, and cited literature) provide a number of possibilities for modification and improvement, or adaptation to specific goals.
Therefore, the aim of this article is to develop an improved method (based primarily on the one proposed by Zwoliński (2009)) for determining geotourist potential in a selected mountain range (the authors chose the Western Carpathians, the mountain range that they know best and one also very well described in the literature) constituting the first step in assessing this potential and selecting specific areas with a high index of geodiversity, which in the future will be subject to more detailed analyses of valorization and assessment in terms of their natural and educational value. The authors posed the following research questions: 1) Will the geodiversity analysis of such a large area as the Western Carpathians be a reliable indicator of its geodiversity and will it show areas with the greatest geotouristic potential in relation to, for example, the existing geosite database? 2) Can this method be used to assess mountain ranges more specifically?
As an example of the use of the developed geodiversity method in practice, an analysis of the geodiversity of the Western Carpathians was carried out. The mountain range is characterized by great geological, geomorphological, and hydrological diversity (see the Study Area section).
The authors share the opinion of many scientists (including Zwoliński et al., 2018) that geodiversity analysis using GIS analyses may play a significant role in forecasting a holistic and integrated ecosystem and geosystem services approach to support the sustainable management of natural systems, creating tourist (geotourist) products (e.g., Burlando et al., 2011; Velázquez et al., 2014; Vasiljević et al., 2018; Albani et al., 2020; Herrera-Franco et al., 2020) or conscious management of protected areas and geoheritage (e.g., Kirchner and Kubalíková, 2014; González-Amuchastegui and Serrano, 2018; Rypl et al., 2019; Carruana Herrera and Martínez Murillo, 2020; Štrba et al., 2020).
Literature Review
In recent years, geodiversity has become very popular as a subject of scientific publications, not only in the context of the definition of the term (e.g., Zwoliński, 2004; Gray, 2005, 2008, 2018a; Brilha, 2016; Coratza et al., 2018) but also, primarily, as an element of description, evaluation, analysis, or assessment of selected areas (e.g., Gray, 2019; Perotti et al., 2019; Albani et al., 2020; Fernández et al., 2020; Herrera-Franco et al., 2020).
Therefore, in this part of the article, the analysis of the literature on the methods used in geodiversity research will be presented in more detail.
Zwoliński et al. (2018) refer that the methods used so far in the description, analysis, and assessment of geodiversity can be divided according to the source of data (direct and indirect, as described by Pellitero et al., 2015) or to the procedure (qualitative, quantitative, and quantitative–qualitative).
Qualitative methods are based primarily on the knowledge and experience of a researcher who is also an expert, or on the knowledge of a group of experts (Zwoliński et al., 2018). These are descriptive methods in which an important element is the scale of the phenomenon, which can be general (e.g., Kale, 2015), or very detailed (e.g., Seijmonsbergen et al., 2014). Qualitative methods use graphic documentation, maps, figures, etc. (e.g., Zwoliński and Stachowiak, 2012; Najwer et al., 2014; Seijmonsbergen et al., 2014; Zwoliński et al., 2018; Jankowski et al., 2020). The expert assessment is supported by in-depth studies of the literature on the subject (Kozłowski et al., 2004a; Gray, 2008; Bradbury, 2014) or material goods and benefits resulting from the marketing of the described area (Sharples, 2002; Gray, 2004, 2005, 2013). The weakness of these methods is their low objectivity (Zwoliński et al., 2018) and their inability to be used in other areas on Earth.
Quantitative methods are based on simple algorithms, measurements, calculations, and GIS analyses, which can additionally be subjected to further calculations and statistical analyses (Zwoliński et al., 2018). The geodiversity assessment is based on analysis of indices (e.g., Serrano and Ruiz-Flaño, 2007a, 2007b; Jaskulska et al., 2013; Zwoliński, 2018; Zwoliński et al., 2018; Jankowski et al., 2020) or algebra map analysis (e.g., Kot and Leśniak, 2006; Zwoliński, 2009; Hjort and Luoto, 2012; Jačková and Romportl, 2008; Zwoliński and Stachowiak, 2012; Najwer et al., 2014; Kot, 2015), additionally using the scoring method (Zwoliński et al., 2018; Jankowski et al., 2020).
Qualitative–quantitative methods: the combination of both types of methods, according to Zwoliński et al. (2018), is the best solution for the assessment of geodiversity. This makes it possible to extend the comprehensive, but simple, assessment of geodiversity with additional elements resulting from the analysis and preliminary assessment of its components, e.g., the valuation of individual types of rocks and their age using the expert method (e.g., Benito-Calvo et al., 2009; Argyrioua et al., 2016; Forte et al., 2018; Jankowski et al., 2020; and references there in), or expert assessment of esthetic, scientific, educational values, etc (e.g., Zwoliński, 2009, 2010; Zwoliński and Stachowiak, 2012; Najwer and Zwoliński, 2014; Najwer et al., 2016; Jankowski et al., 2020). The final product of the combination of these methods is a summary map of geodiversity, the so-called digital-summation technique of map algebra (e.g., Zwoliński, 2009, 2009, 2010; Benito-Calvo et al., 2009; Zwoliński and Stachowiak, 2012; Najwer et al., 2014; Argyrioua et al., 2016; Forte et al., 2018; Jankowski et al., 2020). Often, when summing up the individual components that make up the geodiversity map, an application based on the weighted average (the so-called AHP) is used, which is also based on expert analysis (e.g., Zwoliński and Stachowiak, 2012; Najwer et al., 2016).
Algebra operations with data present in map are an increasing procedure in assessing geodiversity (e.g., De Waele et al., 2005; Pereira et al., 2013; Najwer et al., 2014; Najwer and Zwoliński, 2014; de Paula Silva et al., 2015, 2021; Seijmonsbergen et al., 2018; Zwoliński, 2018; Jankowski et al., 2020; Micić Ponjiger et al., 2021; Pál and Albert, 2021; Dias et al., 2021) becoming a standard method used all over the world. The same approach is used in this work.
Study Area
The Western Carpathians represent the Alpine collisional belt that is conventionally subdivided into the External (EWC), Central (CWC), and Internal Western Carpathians (IWC), (Plašienka et al., 1997; Lačný et al., 2016), while some authors prefer the classical double division, Outer and Inner Carpathians ( e.g., Hók et al., 2014). The Western Carpathians lie mainly in Slovakia and adjacent parts of the Czech Republic, Poland, and Hungary and, from the geological point of view, also in Austria and Ukraine (Figure 1).
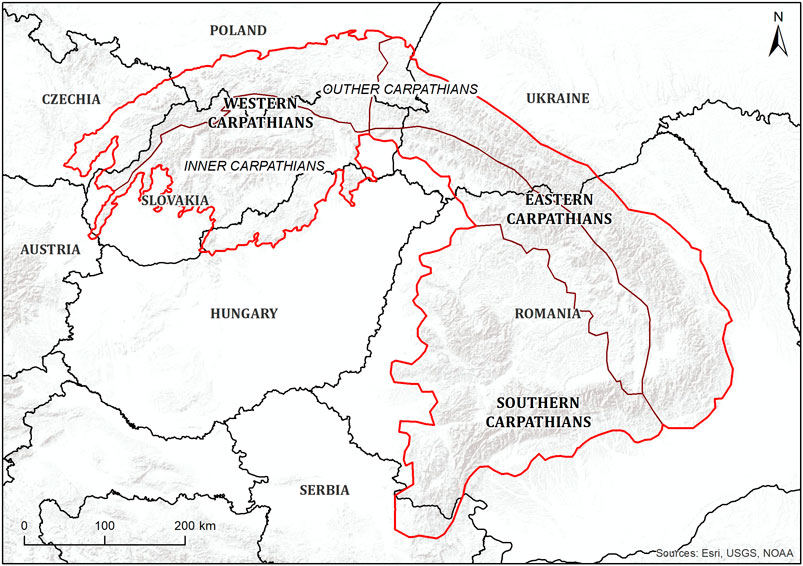
FIGURE 1. Location of the Western Carpathians. Source: own study based on Kondracki, 1978.
The EWC, or so-called Flysch Belt, characterized by alternating layers of sandstone and claystone, represent the outer accretionary wedge of the Carpathian orogen formed in the Eocene to Middle Miocene (Froitzheim et al., 2008; Schmid et al., 2008). The EWC are separated from the CWC by a narrow zone known as the Pieniny Klippen Belt that includes several units consisting mainly of limestone, sandstone, and marl layers of Jurassic and Cretaceous ages (Birkenmajer, 1986). The CWC are composed of three crustal-scale thrust sheets (the Tatric, Veporic, and Gemeric from bottom to top that are formed predominantly by Paleozoic metamorphic and plutonic rock layers) and three large-scale cover nappe systems (Fatric, Hronic, and Silicic, formed by Mesozoic carbonate rock layers), which were all individualized during the Cretaceous Palaeoalpine (ca 120–90 Ma) orogenic processes (Plašienka et al., 1997). The IWC nappe units (Meliatic-Turnaic-Silicic) are interpreted as being closely related to the Middle Triassic opening and Late Jurassic closure of the north-western branch of the Neotethys, along with the other units similar to the Southern Alps and Dinarides (Transdanubian and Bükk units), which occur to the north-west of the Mid-Hungarian Shear Zone (Lačný et al., 2016).
Inter-mountain basins of the CWC and the IWC are filled by Cenozoic sediments. Younger formations are represented also by Cenozoic Neovolcanism (from the Neogene to the Quaternary), mostly in the internal part of the Western Carpathians. Three main phases of the volcanic activity are distinguished (Hók et al., 2001): acid (rhyodacites and rhyolites), intermediate (andesites and dacites), and basic (basalts).
From the geomorphological point of view, the Western Carpathians represent a province within the Carpathian Mts. subsystem and are divided into two subprovinces, the Outer Western Carpathians and the Inner Western Carpathians (Mazúr and Lukniš, 1978; Balatka and Kalvoda, 2006; Kondracki, 2011; Solon et al., 2018).
They are an extensive, relatively flat, and elliptical elevated area (dome) bordered by the depressions of the Pannonian basin systems and Vienna basin, Carpathian Foredeep, and the Transcarpathian depression (Mazúr, 1965; Minár et al., 2011). The surface of this megaform is a mosaic of discrete mountains (mainly horst and dome structures) and basins (mainly graben and flexures) that represent young active morphostructures formed during the Late Miocene, Pliocene, and Quaternary evolution of recent relief (Urbánek and Lacika, 1998; Maglay et al., 1999; Minár et al., 2011).
The present surface of the Western Carpathians is diverse and its character depends mainly on altitude and the bedrock (Lukniš, 1972; Lauko, 2003; Lóczy et al., 2012). The Tatra Mts., the highest mountain range in the whole Carpathians (the Gerlachovský štít Peak 2,655 m a.s.l.), were modeled by glaciers in the Pleistocene. After the retreat of glaciers (ca 8,500 years ago; Lindner et al., 2003), we find here significant relict glacial landforms, e.g., cirques, U-shaped valleys, or moraines (Lukniš, 1973; Klimaszewski, 1988). To a lesser extent, we find the remains of glacial relief also in the Nízke Tatry Mts.
The surface of the Western Carpathians mountain ranges has been modeled to its present form mainly by periglacial, hillslope, and fluvial processes. Another important phenomenon is the karst relief (especially the plateaus of the Slovenský kras Karst Area and the Spišsko-gemerský kras Karst Area, as well as the karst on the Mesozoic formations within several mountain ranges (e.g., the Nízke Tatry Mts., the Tatra Mts., the Veľká Fatra Mts., and the Malá Fatra Mts.)), with abundant occurrence of karst landforms (caves, abysses, dolines, and gorges). The bottoms of inter-mountain basins are molded by erosive and accumulative fluvial forms of contemporary rivers.
Most of the territory of the Western Carpathians is drained into the Black Sea, within the Danube River drainage basin, (e.g., Miklánek, 2012) and its direct tributaries (the Morava River, the Váh River, the Nitra River, the Hron River, and the Ipeľ River) and the tributaries of the Tisza River (the Hornád River and the Slaná River). A smaller part of the area is drained into the Baltic Sea within the Vistula River drainage basin and its tributaries (the Dunajec River with the Poprad River, the San River, the Wisłok River, the Wisłoka River, the Skawa River, the Raba River, and the Soła River).
Rivers have mostly a rain–snow regime of runoff, with high-water periods in the spring and in summer (e.g., Šimo and Zaťko, 2002). On many rivers, floods pose a significant natural risk.
The Western Carpathians are not very rich in lakes. The only major area of their occurrence is the Tatra Mts., where we find more than 200 lakes of glacial origin (cirque as well as moraine). The largest is the Morskie Oko Lake in the Polish part of the Tatra Mts. (34.9 ha). Smaller landslide dammed lakes occur in some places.
The geological-tectonic development of the Western Carpathians governed suitable conditions for mineral and thermal water formations, especially thanks to the great extent of Mesozoic deposits, Tertiary marine, and freshwater deposits, as well as Alpine-type tectonics (Franko and Melioris, 2000).
Hydrogeological richness lies not only in the large number of springs (more than 1,000 in Slovakia alone), but mainly in their relatively even distribution throughout the territory (one spring of mineral or thermal water appears for each ca 40 km2; Franko and Melioris, 2000). The springs are intensively used (balneology, aquaparks and mineral water production). The occurrence of travertines (especially in the Podtatranská kotlina Basin and the Hornádská kotlina Basin) is connected with springs along tectonic lines.
Methods
The basis of methodology for creating a synthetic map of the geodiversity of the Western Carpathians was taken from the work by Zwoliński (2009) and modified by the authors. The main modifications consisted in 1) using hexagons as the main analytical fields; 2) the use of geomorphons as elements of the relief types; 3) specification in each of the four component maps of five classes corresponding to the best use of the geodiversity map for geotourist purposes.
When evaluating the created maps, it was necessary to choose spatial units that should be different from each other in terms of the analyzed layers. Basic geomorphological units such as individual mountain ranges, basins, lowlands, etc., are objects that people traditionally perceive in the landscape and naturally form the basis for such a division of territory. On the other hand, the issue of geomorphological division encounters several complications. The basic problem is the fact that the studied area is spread over the territory of four states, in each of which the basic geomorphological units were allocated by different authors, using different methods and at different times. It is also necessary to perceive the allocated units and their interpretation and systematization in accordance with the growth of knowledge about the geological and geomorphological development of the area. The largest part of the territory of the Western Carpathians lies in Slovakia, where the map of the regional geomorphological division (Mazúr and Lukniš, 1978) is still valid. The map was converted into a digital database (Urbánek et al., 2009) and the course of the borders was reinterpreted to a scale of 1:50,000, but it does not reflect the latest knowledge and there was no harmonization of borders extending to neighboring countries. Several authors have tried to create their own division for the territory of the Western Carpathians for the needs of their research, either based on traditional units (Minár et al., 2011) or created automatically on the basis of statistical processing of DMT using GIS tools (Bandura et al., 2019). For the needs of geodiversity analyses, we have chosen a map of mesoregions (Balon et al., 2015) mainly because it most coherently covers the entire studied area. As a result, however, the names of some mesoregions and their definition do not correspond to the official divisions valid in Slovakia, the Czech Republic, and Hungary (Figure 2).
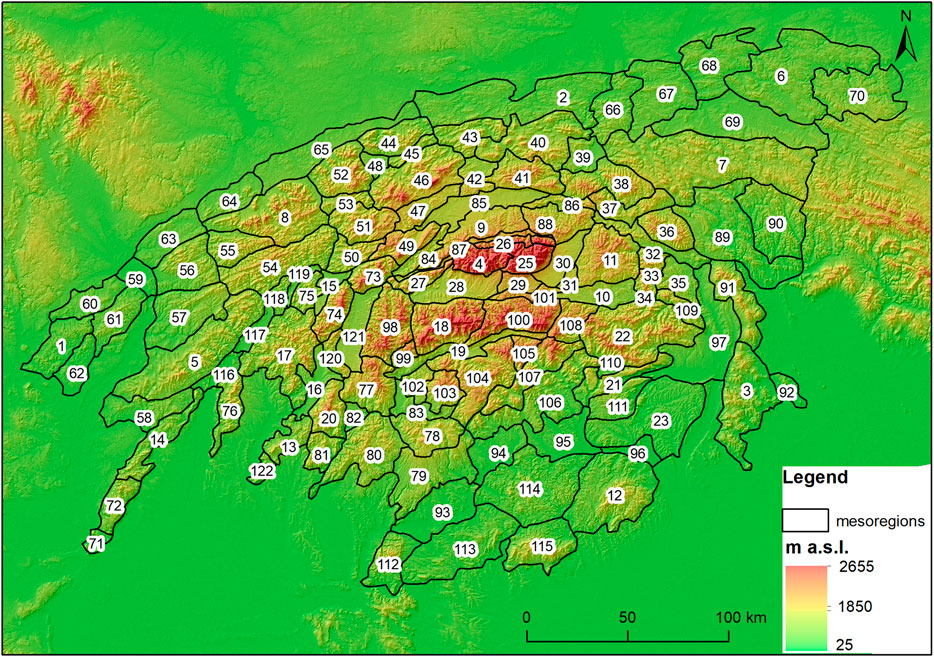
FIGURE 2. The mesoregions of Western Carpathians. Source: Balon et al., 2019. 1: Ždánický les Plateau, 2: Pogórze Wielickie Range, 3: Zempléni hegység Mts., 4: Western Tatra Mts., 5: Biele Karpaty Mts., 6: Pogórze Dynowskie Range, 7: Beskid Niski Mts., 8: Moravskosliezske Beskydy Mts., 9: Fore-Tatra Foothills, 10: Hornadska kotlina Basin, 11: Levočské vrchy Mts., 12: Bükk hegység Mts., 13: Veľký Tríbeč Mts., 14: Malé Karpaty Čachtické Mts., 15: Žilinská kotlina Basin, 16: Hornonitrianska kotlina Basin, 17: Strážovské vrchy Mts., 18: Nízke Tatry-Ďumbierske Tatry Mts., 19:Horehronské podolie Basin, 20: Vtáčnik Mts., 21: Zádielska dolina Valley, 22: Volovské vrchy Mts., 23: Bodvianska pahorkatina Range, 24: Belianske Tatra Mts., 25, High Tatra Mts., 26 Reglowe Tatra Mts., 27: Chočské vrchy Mts., 28: Liptovská kotlina Basin, 29: Štrbský prah Range, 30: Popradská kotlina Basin, 31: Wierzbow Range, 32: Spišsko-šarišské medzihorie Upland, 33: Bachureň Range, 34: Branisko Range, 35: Šarišske podhorie Range, 36: Čergov Mts., 37: Pogórze Popradzkie Range, 38: Beskid Sądecki Mts., 39: Kotlina Sądecka Basin, 40: Beskid Wyspowy Mts., 41 Gorce Mts., 42, Pogórze Orawsko-Jordanowskie Range, 43: Beskid Średni Mts., 44: Beskid Mały Mts., 45: Pasma Pewelsko-Krzeczowskie Range, 46: Beskid Żywiecko-Orawski Mts., 47: Działy Orawskie Range, 48: Kotlina Żywiecka Basin, 49: Oravská Magura Mts., 50: Kysucká vrchovina Range, 51: Beskid Żywiecko-Kysucki Mts., 52: Beskid Śląski Mts., 53: Międzygórze Jabłonkowsko-Koniakowskie Upland, 54: Javorníky Mts., 55: Vsetínské vrchy Mts., 56: Hostýnské vrchy Mts., 57: Vizovická vrchovina Mts., 58: Myjavska pahorkatina Range, 59: Hornomoravský úval Basin, 60: Litenčická pahorkatina Range, 61: Chřiby Mts., 62: Kyjovská pahorkatina Range, 63: Podbeskydská pahorkatina Range, 64: Jičínská pahorkatina Mts., 65: Pogórze Śląskie Range, 66: Pogórze Rożnowskie Range, 67: Pogórze Ciężkowickie Range, 68: Pogórze Strzyżowskie Range, 69: Doły Jasielsko-Sanockie Basin, 70: Pogórze Przemyskie Range, 71: Malé Karpaty Brezovské Mts., 72: Malé Karpaty Devínske Mts., 73: Krivánska Fatra Mts., 74: Lúčanská Fatra, 75: Súľovské skaly Mts., 76: Góry Iłowieckie Mts., 77: Kremnické vrchy Mts., 78: Javorie Range, 79: Krupinská planina Range, 80: Štiavnické vrchy Mts., 81: Pohronský Inovec Range, 82: Žiarská kotlina Basin, 83: Zvolenská kotlina Basin, 84: Oravská kotlina Basin, 85: Kotlina Orawsko-Nowotarska Basin, 86: Pieniny Mts., 87: Sub-Tatra Basin, 88: Magura Spiska Range, 89: Ondavská vrchovina Range, 90: Laborecká vrchovina Range, 91: Slanské vrchy Mts., 92: Pagóry Zemplińskie Range, 93: Ipeľská kotlina Basin, 94: Lučenská kotlina Basin, 95: Rimavská kotlina Basin, 96: Borsodi-medence Basin, 97: Košická kotlina Basin, 98: Veľká Fatra Mts., 99: Starohorské vrchy Mts., 100: Nízke Tatry-Kráľovohoľské Tatry Mts., 101: Kozie chrbty Mts., 102: Bystricka vrchovina Range, 103: Polána Range, 104: Veporské vrchy Mts., 105: Muránska planina Range, 106: Revúcka vrchovina Range, 107: Stolické vrchy Mts., 108: Slovenský raj Mts., 109: Černá hora Mts., 110: Rožňavská kotlina Basin, 111: Slovenský a Aggtelecký kras Range, 112: Börzsöny hegység Range, 113: Cerová vrchovina Range, 114: Cerov Mts., 115: Matra Mts., 116: Trenčianská kotlina Basin, 117: Ilavská kotlina Basin, 118: Podmanínska pahorkatina Hillyland, 119: Bytča Basin, 120: Žiar Mts., 121: Turčianska kotlina Basin, 122: Malý Tríbeč Mts.
The altitude data for this study were obtained from the NASADEM project (NASADEM, 2020). The terrain model was used in the form of a raster with a spatial resolution of 30 m in the ETRS89 LAEA coordinate system (EPSG code: 3,035). Most of the analyses were carried out on the basis of the numerical terrain model, but the CORINE Landcover map (CORINE Landcover map, 2018) and the digital geological map of the Carpathians (Lexa et al., 2000) were also used. The area of the Western Carpathians has been divided into hexagons of 200 m high (a vector grid was created using a command in QGIS 3), and the values from partial maps are assigned to each polygon or its raster representation (raster maps with a resolution of 30 m). The use of hexagons in the analysis had one main reason; the hexagons are filling all the areas by replication which provides less variations on distances between its center and its outer limit (Zhang, 2005).
In order to obtain the most objective terrain forms from the digital terrain model, the authors used geomorphons (Stepinski and Jasiewicz, 2011; Jasiewicz and Stepinski, 2013). This tool made it possible to automatically and continuously divide the whole area into areas that are quasi-homogeneous in terms of the geomorphological form of the relief. This is a basic prerequisite for quantification and evaluation, including in terms of geodiversity. On the other hand, a somewhat schematic picture is created. The disadvantage is that, in nature, different forms naturally have different dimensions and relief elements important in terms of geodiversity can be numerous, but occupy smaller areas (this applies to the summit category, where the tool evaluates as a summit only the immediate vicinity of the inflection point of the peak and the wider area already falls under the slope category) (Jasiewicz and Stepinski, 2013).
The following analytical maps were created (based on Zwoliński’s method, 2009): energy of relief map (relative heights), relief fragmentation map (forms of relief), geological diversity map (lithological map), and land cover diversity map (based on the CORINE Landcover map). There are four basic parameters for which it is possible to perform a surface analysis. In the literature, there are also other parameters, such as the presence of caves, lakes, watercourses, etc. (e.g., Zwoliński and Stachowiak, 2012), which also have an impact on the geodiversity index, but are in a way a component of the relief and therefore one of the main components mentioned above. The synthetic geodiversity map was created by adding four analytical maps with the adopted weights using the weighted sum tool in ArcGIS. The geodiversity map has been classified into five classes using the Jenks method. The particle maps were then subjected to the expert weighting method, AHP (analytic hierarchy process). This method of multicriteria analysis proposed by Prof. Thomas L. Saaty (1990) allows comparison of measurable data and non-measurable data based on expert knowledge. The individual analytical maps have weights assigned in the Analytical Priority Calculator (bpmsg.com/ahp/ahp-calc.php). It was decided to assign the following weights (in percent) to the maps: relief energy map, 56.8%, relief fragmentation map, 28.4%, lithological map, 10.5%, and durability of relief map, 4.3%
The weights represent final impact on the synthetic map of geodiversity (Figure 3).
The created analytical and synthetic maps were thus overlaid by the map of mesoregions. Using the zonal statistics tools within the Spatial Analyst Tools ArcMap extension (zonal statistics as table and zonal histogram), we obtained a set of statistical data for each mesoregion, describing the values of the monitored indicators within their boundaries.
Results
The synthetic geodiversity map consists of four component maps, which will be analyzed below.
Analysis of Energy of Relief Geodiversity
The energy of relief map represents relative heights within each hexagon, the lowest value in a given hexagon is subtracted from the value of the highest altitude above sea level. The ESRI ArcGIS zonal statistics tool with range parameter was used to achieve the results. Relative heights were then classified into the five classes (Figure 4).
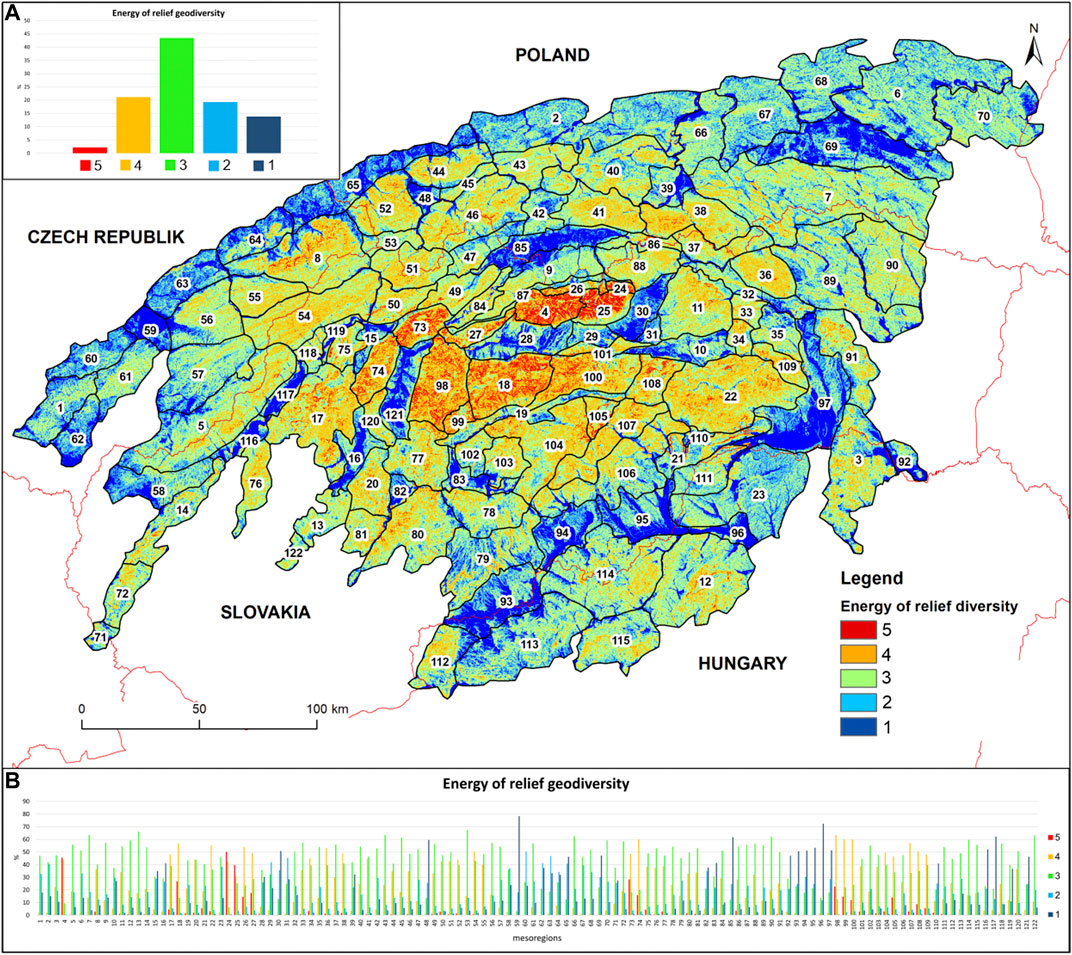
FIGURE 4. Energy of relief geodiversity map: 1: 0–10 m, 2: 10–20 m, 3: 20–50 m, 4: 50–100 m, 5: 100–10,000 m. For numbers of mesoregions, see Figure 2. Source: own research.
The higher the value, the greater the differences in altitudes within the hexagon. The most numerous group consists of hexagons with a value of 3 (43.5%), followed by categories 4 (21.2%) and 2 (19.3%). Hexagons of the highest category five have a relatively low proportion (2.2%). The average value of a hexagon for the whole examined area is 2.8. Only at 12 out of 122 mesoregions, the share of hexagons was the highest (the category exceeds 10%). Conversely, in the case of 22 mesoregions, such hexagons do not occur at all and in the case of about half (59 mesoregions), their share is in the range of 0–1% (Figure 4A).
The spatial distribution of the values of this indicator outlines the structure of the dome megaform of the Western Carpathians. The highest values of relief energy prevail in the cente (the Tatra Mts., the Nízke Tatry Mts., the Veľká Fatra Mts., the Malá Fatra Mts., partly the Slovenské rudohorie Mts., and the Rudawy Wschodnie Mts.), and towards the periphery, it has a declining trend, e.g., we record slightly above-average values in the mesoregions of medium-high mountains, especially in the area of the Outer Western Carpathians and the Slovenské stredohorie Mts. (the Rudawy Zachodnie Mts.). Relief energy values around the average then occur in the lower mountains lying on the periphery (e.g., the Malé Karpaty Mts., the Biele Karpaty Mts., the Mátra Mts., and the Slanské vrchy Mts.) and the foothills. The lowest values belong to the mesoregions of basins (some of which also lie in the central part between the highest mountains) and lower hilly lands in the northern, southern, and western foothills of the Western Carpathians (Figure 4).
Summing up, analyzing geodiversity in the Western Carpathians on the basis of a map of energy of relief, it can be clearly stated that in the majority of the area, we are dealing with a medium and slightly higher and slightly lower degrees of relief energy variation (classes 3, 4, and 2). The occurrence of the highest class of geodiversity (class 5) is associated with the highest elevated mountain areas and generally the values of the energy of the relief correlate with altitude.
Analysis of Relief Fragmentation Geodiversity
The basis for this partial analysis was the map of geomorphons (Stepinski and Jasiewicz, 2011; Jasiewicz and Stepinski, 2013). The area was divided into 10 basic morphological categories, which were assigned five values of geodiversity (Figure 5).
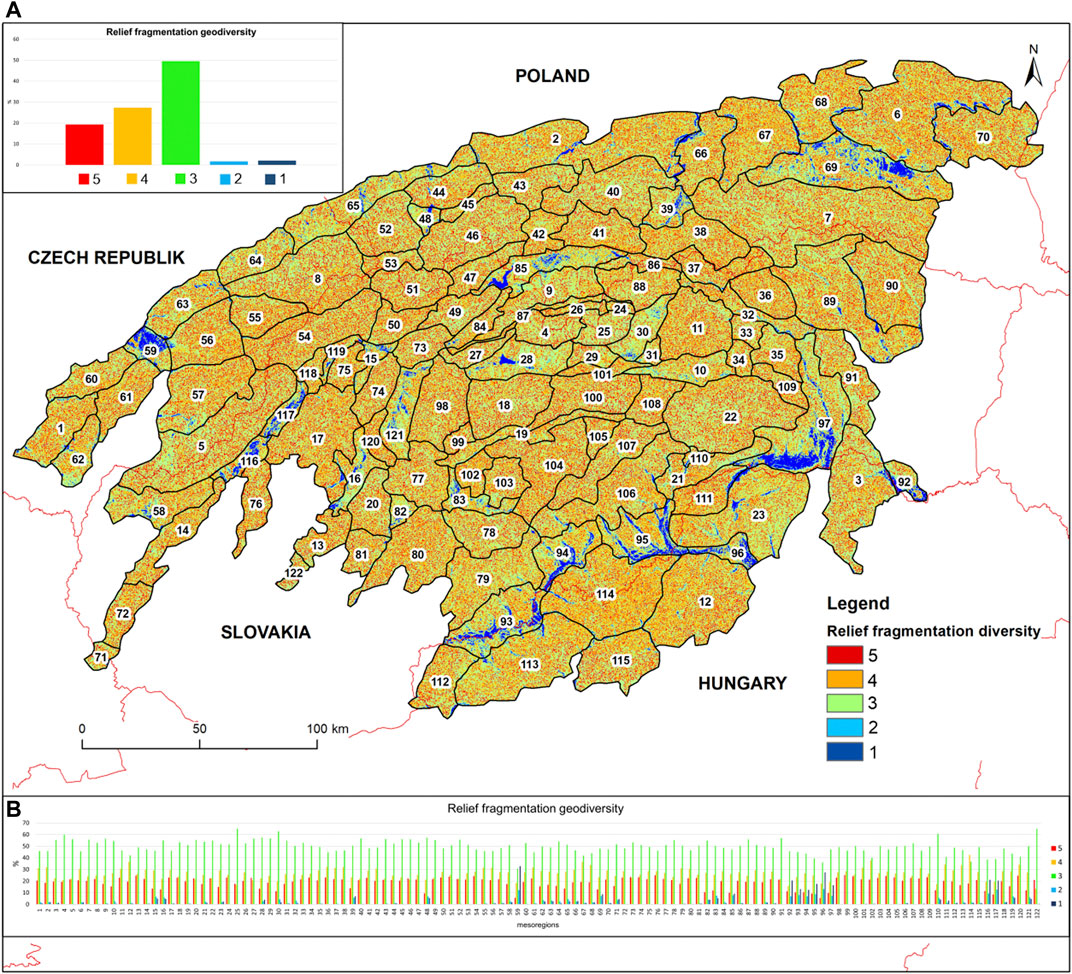
FIGURE 5. Relief geodiversity map. 5: summit/spur; 4: ridge/valley/shoulder; 3: slope/hollow; 2: footslope; 1: flat/depression. For numbers of mesoregions, see Figure 2. Source: own research.
Within the entire studied area of the Western Carpathians, category three dominates (49.5%) followed by categories 4 (27.3%) and 5 (19.3%). The total average value of hexagons in the entire Western Carpathians is 3.6. Within the individual mesoregions, the average values of the geodiversity of the relief range from 2.52 (the valley of Central Morava River) to 3.83 (the Žiar Mts.). The differences between mesoregions are small, with about 71% of them reaching a median value of three and with the remaining approximately 29% having a value of 4. Slightly above-average numbers of hexagons with a higher assigned value of geodiversity of relief fragmentation occur in the lower mountains (Figure 5A).
Categories of lower values are usually under-represented. Above-average representation of hexagons with lower values (1 and 2) is achieved by several basin mesoregions. There is a strong correlation between the number of hexagons with values of 1 and 2 (r = 0.91).
The representation of hexagons with a value of five ranges from about 5 to 25%, with the majority falling into the category of spurs. The increased proportion of hexagons with a value of five is found mainly in the lower mountains (the Starohorské vrchy Mts., the Považský Inovec Mts., the Veľký Tríbeč Mts., the Žiar Mts., the Veporské vrchy Mts., and the Javorníky Mts.), while in the higher mountains, the larger areas cover more continuous slopes undisturbed by other forms of relief. However, the differences between mesoregions are again insignificant (the average share of category five within mesoregions is 19%, median 20%) (Figure 5). The lowest proportion of pixels with the highest value is reached by the basin mesoregions.
Summing up, analyzing relief geodiversity in the Western Carpathians on the basis of a map of geomorphons, it can be clearly stated that in the majority of the area, there is a medium degree of relief variation (class 3). The occurrence of the higher geodiversity classes (4 and 5) is also relatively important. The lowest classes of geodiversity occur in low-lying basin mesoregions.
Analysis of Geological Geodiversity
The lithological geodiversity map was created on the basis of the 1:500,000 geological map of the Western Carpathians (Lexa et al., 2000). Individual lithological sections were assigned to one of five classes depending on their age, mineral composition, and resistance (Figure 6).
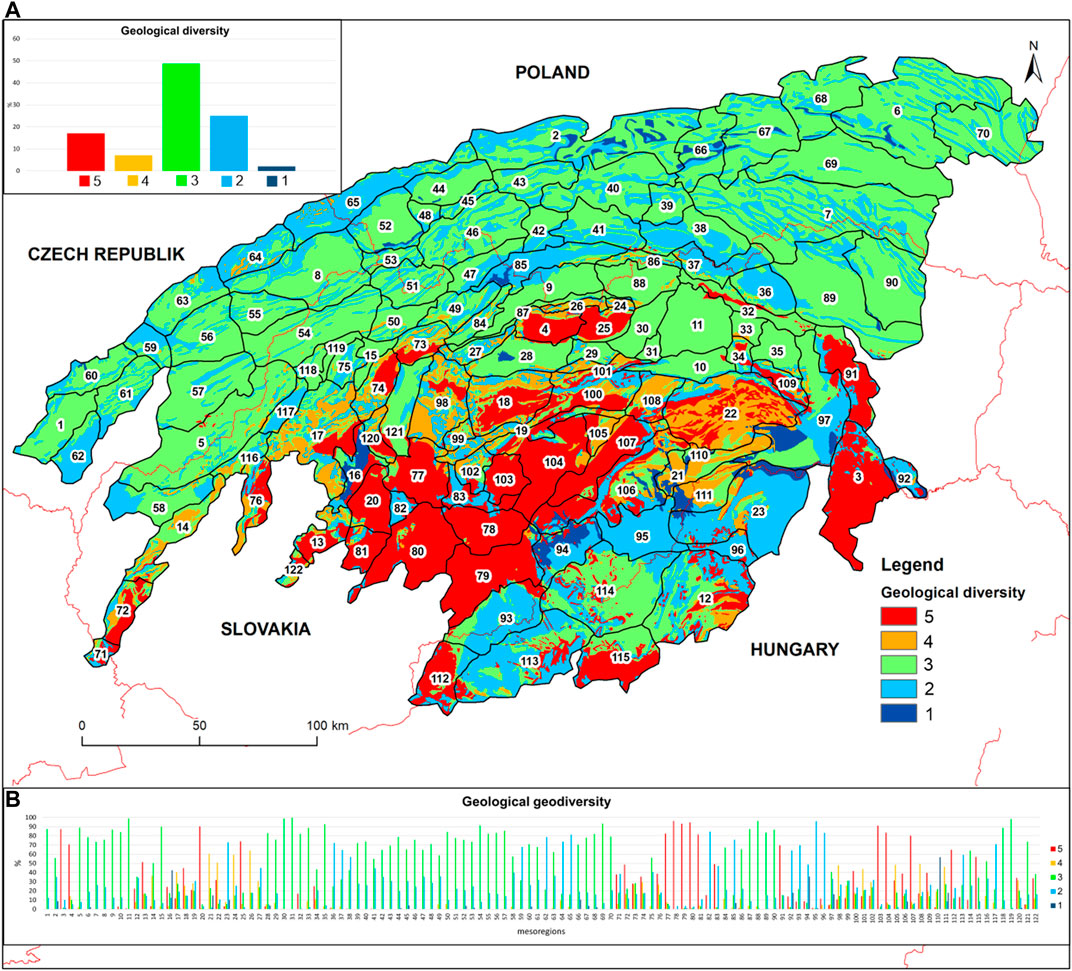
FIGURE 6. Geological geodiversity map. 1: Quaternary rocks; 2: clay shales, crushers, and claystones; 3: sandstones and flysch formations; 4: limestones and dolomites; 5: crystalline, volcanic, and metamorphic rocks. For numbers of mesoregions, see Figure 2. Source: own research.
The total average value of hexagons in the entire research area is 3.12. Within the individual mesoregions, the average values of geological geodiversity are from 1.97 (the Lučenecká kotlina Basin) to 4.89 (the Javorie Mts.–the Jaworze Mts.). The differences between mesoregions are moderate, with about 55% of them reaching a median value of three; with another approximately 30% being more or less equally split between the values of 2 and 4 and with the remaining approximately 15% having a value of 5. Class 1 is under-represented (Figure 6A).
The largest zone of the analyzed area is covered by flysch formations (both outer and inner Carpathian flysch) classified into geodiversity class 3. They constitute less than 49% of the whole area and are predominant in the mesoregions of the outer Beskids and in the Fore-Tatra Foothills, the Sub-Tatra Basin, and the Levočské vrchy Mts. In 58 out of 122 analyzed physico-geographical mesoregions (Balon et al., 2015), they cover over 50% of the area, and in nine of them (the Žilinská kotlina Basin, the Javorníky (Jaworniki) Mts., the Šarišská vrchovina Upland, the Doły Jasielsko-Sanockie Basin, the Spišská Magura Mts., the Bytčianska kotlina Basin, the Popradská kotlina Basin, the Levočské vrchy Mts., and the Wierzbow Range) the Carpathian flysch occupied more than 90% of the mesoregion’s area. The smallest area (less than 1%) of flysch formations is found in four mesoregions: the Žiarska kotlina Basin, the Javorie Mts., the Borsod Basin, and the Rimavská kotlina Basin (Figure 6).
The second largest lithological complex is of clayey rocks, classified into geodiversity class 2. They constitute 25% of the analyzed research area. The largest area is covered by the following mesoregions: the Borsod Basin (83%), the Žiarska kotlina Basin (86%), and the Rimavská kotlina Basin (96%). In 16 mesoregions, they cover more than 50% of the area. The smallest percentage share (less than 1%) is in the Rožňavská kotlina Basin, the Levočské vrchy Mts., the Popradská kotlina Basin, the Wierzbow Range, the Spišsko-šarišské medzihorie Upland, and the Spišská Magura Mts. (Figure 6).
Then, 17% of the analyzed area is covered by crystalline formations belonging to geodiversity class 5. They build the massifs of the Tatra Mountains and the Nízke Tatry Mts., but the largest area (over 90%) is in the mesoregions, the Vtáčnik Mts., the Poľana Mts., the Krupinská planina Upland, the Štiavnicke vrchy Mts., and the Javorie Mts. In 16 mesoregions, they cover over 50% of the area. However, in 52 mesoregions, this class does not exist at all. These mainly constitute the area of the Outer Flysch Carpathians (Figure 6).
Seven percent of the analyzed area is covered by carbonate formations classified as class 4 and occurring mainly in the Tatry Reglowe Mts. (64%), the Slovenský kras (Silicko-Zadielski) Karst Region (60%), the Belianske (Bielskie) Tatry Mts. (59%), and the Volovské vrchy Mts. (51%). In 35 regions, this class is not visible at all.
The smallest acreage (2%) is occupied by Quaternary deposits, but they also cover the bottoms of valleys flowing from the Tatra Mountains or the Nízke Tatry Mts., which is not included in this map scale. In 84 mesoregions analyzed, they were not taken into account at all, while the largest area is in the Lučenecká kotlina Basin (57%), the Hornonitrianska kotlina Basin (42%), and the Rožňavská kotlina Basin (36%) (Figure 6).
Summing up, analyzing lithological geodiversity in the Western Carpathians on the basis of a 1:500,000 geological map, it can be clearly stated that the majority of the area exhibits a medium or low degree of geological variation (classes 3 and 2), but in high mountain areas (the Tatra Mts. and the Nízke Tatry Mts.), volcanic ones (the Vtáčnik Mts., the Poľana Mts., the Krupinská planina Upland, the Štiavnicke vrchy Mts., and the Javorie Mts.), and karst ones (the Muránska planina Mts. and the Slovenský kras Karst Region), there are also areas classified as the highest geodiversity classes (4 and 5). The lowest class of geodiversity occurs within the low-lying Carpathian valleys, mainly in the Hornonitrianska kotlina Basin, the Lučenecká kotlina Basin, and the Rožňavská kotlina Basin.
Analysis of Land Cover Geodiversity
Land cover geodiversity map was created on the basis of strict criteria proposed by Zwoliński (2009). Individual criteria of the land cover types were built on the assumption that the largest value 5 would be assigned to objects without soil cover and anthropogenic objects. The lowest criterion of the diversity of this feature was assigned to the areas where the geology and forms of relief are covered by industrial and residential areas, heaps, sports grounds, or airport area. However, the authors used two exceptions in the case of quarries and peat bogs, assigning them the highest value due to the fact that both types of cover are very important from the point of view of geotourism and the creation of geosites.
Analysis of the Landcover map (CORINE Landcover map, 2018) reveals a clear advantage (over 52%) of the share of criterion 3 (forests) in land cover. This is not surprising, as the Carpathian mountain range is characterized by the presence of vegetation, and the average altitudes above sea level of individual mountain ranges correspond to lower and upper montane forests (Figure 7A).
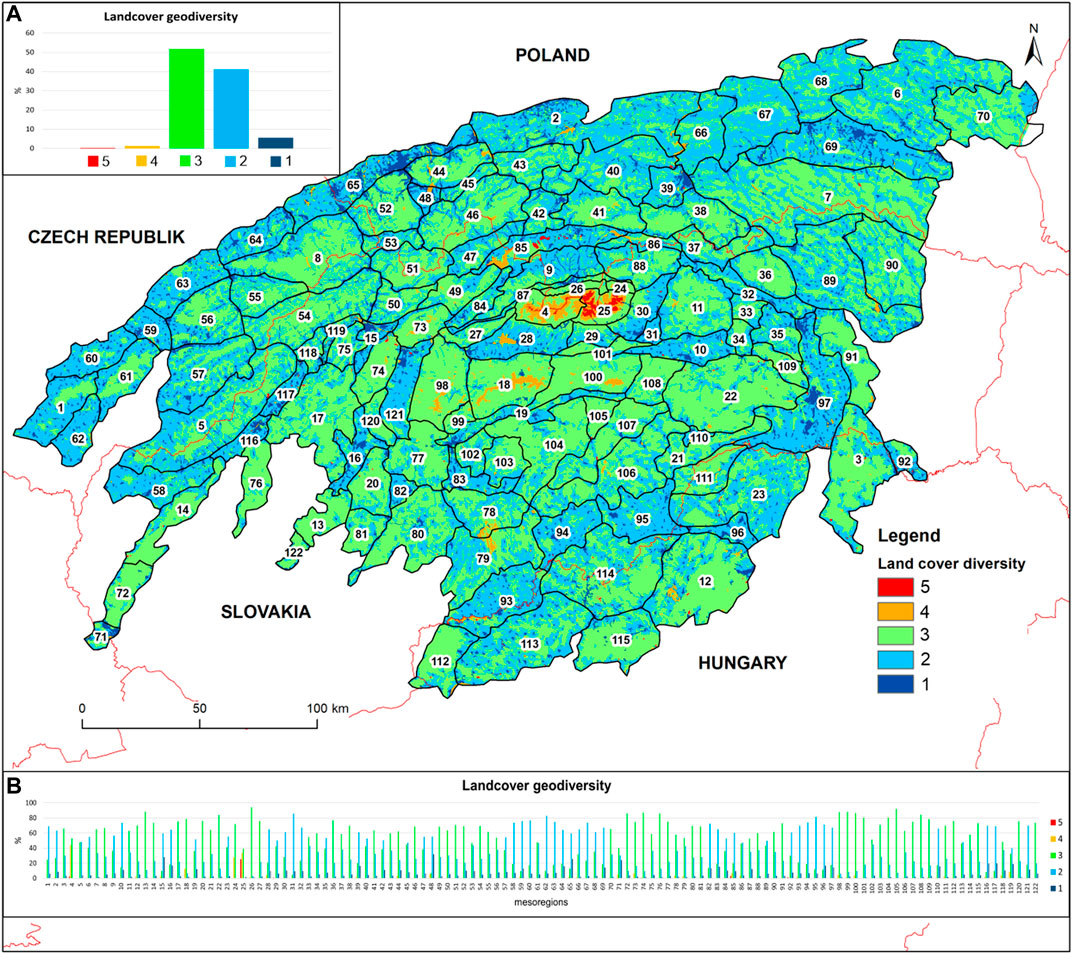
FIGURE 7. Landcover geodiversity map. 5: rocks, peat bogs, and quarries; 4: pastures and meadows; 3: forests, shrubs, and arable lands; 2: buildings; 1: industrial areas. For numbers of mesoregions, see Figure 2. Source: own research.
The total average value of hexagon in the entire research area is 2.49. Within the individual mesoregions, the average values of the CLC geodiversity are from 1.86 (the Kotlina Żywiecka Basin) to 3.81 (the Vysoké Tatry Mts.–the High Tatra Mts.). The differences between mesoregions are moderate, with about 45% of them reaching a median value of two and with the remaining about 54% value of 3. Other categories are under-represented. There is a strong correlation between the number of hexagons with values of 1 and 2 (r = 0.71).
In 69 out of 122 surveyed physical and geographical mesoregions (Balon et al., 2015), it is forests (classified in category 3) that constitute the largest single type of land cover, ranging from 46.71% in the Międzygórze Jabłonkowsko-Koniakowskie Range to 94.17% in the Tatry Reglowe Mountains. The Vrbovská pahorkatina Foothills (part of the Popadská kotlina Basin) are characterized by the lowest percentage of forests among the particular mesoregions, covering 4.7% (Figure 7).
The second largest type of land cover (classified in category 2) is arable land, meadows and pastures, orchards, and urban green areas, which together account for less than 41% of the total area coverage. In 51 out of 122 surveyed physical and geographical mesoregions, it was this criterion that constituted the largest percentage share of area occupied, from 85.78% in the Vrbovská pahorkatina Foothills to 47.14% in the Pasma Pewelsko-Krzeczowskie Range. The lowest presence of arable fields of meadows and pastures in the occupied area is found in the Belianske Tatry Mts. (0.03%), but in the High Tatra Mts., this class does not occur at all.
Of the total analyzed area, 5.6% is occupied by industrial areas, housing estates, heaps, sports grounds, and airports, together classified as geodiversity class 1, and they do not constitute the highest percentage of the area in any of the mesoregions and account for very little of the Belianske Tatry Mts. mesoregion. The mesoregion with the highest percentage of urbanized areas is the Kotlina Żywiecka Basin (31.87%) (Figure 7).
Of the study area, 1.3% is occupied by rocky grasslands, heaths, swamps, streams and reservoirs, and lakes, together classified as class 4. In 24 mesoregions, no such land cover was recorded in general, while the highest percentage of this type of land cover occurs in the Western Tatra Mts. (44.21%) (Figure 7).
Rocks, peat bogs, and quarries constitute the highest class 5 of geodiversity of land cover and they occupy the smallest percentage share in the entire Western Carpathians (less than 0.3%). In 47 mesoregions analyzed, this type of land cover does not occur at all, and in 71 mesoregions, this type of land cover occupies less than 1% of the area. The highest percentage is found in the High Tatra Mts. (over 25%), where there is a range of crags, as well as the Orawa-Nowy Targ Basin (3% of the area covered by peat bogs) (Figure 7).
Summing up, analyzing the geodiversity of land cover in the Western Carpathians on the basis of the CLC, it can be clearly stated that in most areas, there is a medium and low degree of land cover differentiation (classes 3 and 2), but in high mountain areas (the Tatra Mountains), there are also areas of the highest geodiversity (classes 4 and 5). The lowest class of geodiversity occurs within large cities, especially in the Kotlina Żywiecka Basin region.
Synthetic Geodiversity Map
The synthetic geodiversity map was created from the combination of the four component maps described above, for which the appropriate hierarchy of values was assigned using the AHP calculator (see the Methods section). The authors independently used the AHP calculator to determine which of the four components that make up the synthetic geodiversity map is the most important and which is the least important. The analysis shows that the most important element determining the geodiversity of the Western Carpathians is energy of relief geodiversity and it was given a weight of 56.8%, then relief geodiversity 28.4%, geological geodiversity 10.5%, and landcover geodiversity 4.3%. Summing up the individual elements, taking into account the above weights, made it possible to create a synthetic map of geodiversity. The values one to five themselves, where one is the areas with the lowest geodiversity and five are the areas with the highest geodiversity, were determined using the Jenks classification, where one means areas where the total score was in the range 1 (very low geodiversity): 1–2.066,666,667; 2 (low geodiversity): 2.066,666,668–2.77,254,902; 3 (medium geodiversity): 2.772,549,021–3.352,941,176; 4 (high geodiversity): 3.352,941,177–3.870,588,235; and 5 (very high geodiversity): 3.870,588,236–5.
In total geodiversity of the Western Carpathians, category three is the largest single class (39.55%). The total average value of hexagons in the entire Western Carpathians is 2.92 (Figure 8A).
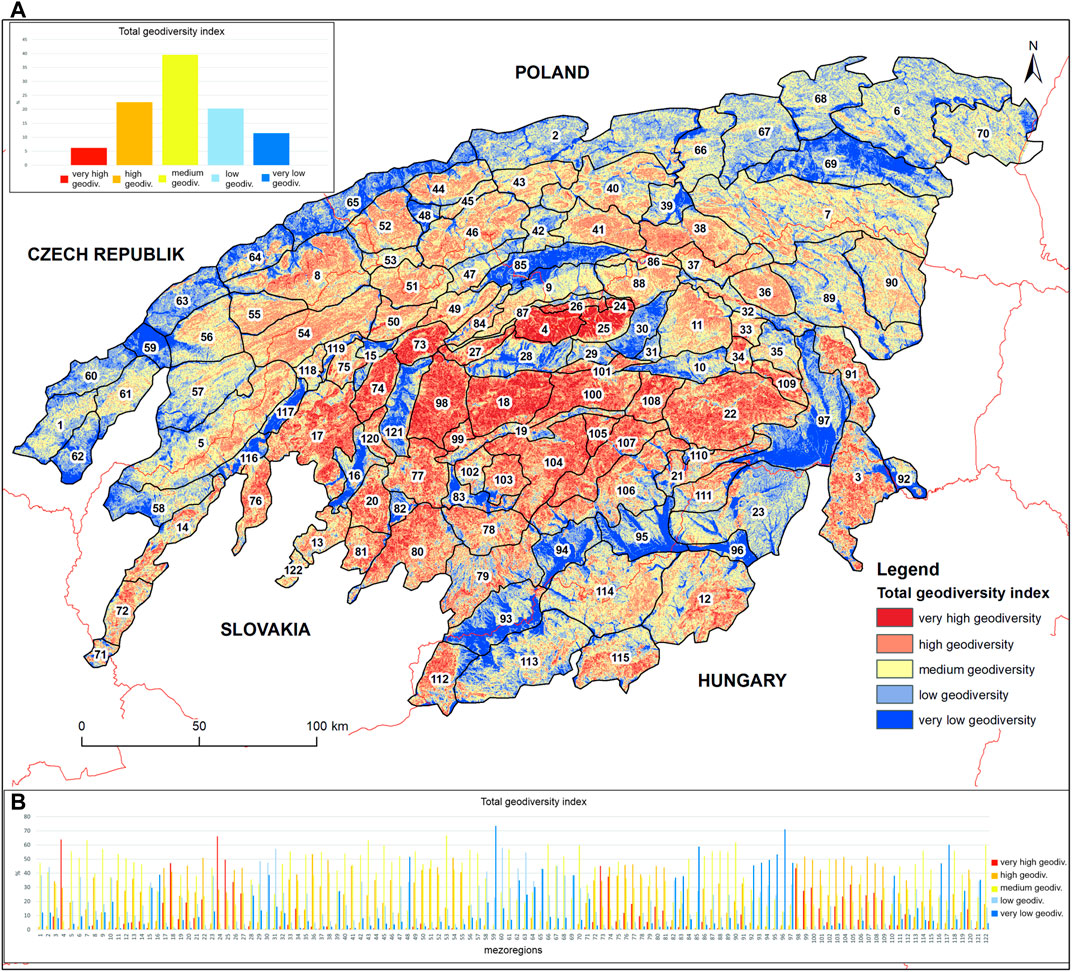
FIGURE 8. Synthetic geodiversity map. For numbers of mesoregions, see Figure 2. Source: own research.
The averages for mesoregions range from 1.30 (the Hornomoravský úval Basin) to 4.59 (the Belianske Tatry Mts.). The differences between mesoregions are moderate, with about 47% of them reaching a median value of three and with another approximately 23% also having a value of 4. Other categories are under-represented. The correlation between similar classes is moderate (r = 0.6 for classes 4 and 5) and strong (r = 0.62 for classes 1 and 2; r = 0.69 for classes 2 and 3).
Category three prevails in 52 out of 122 analyzed mesoregions and the values range from 40% (in the Beskid Mały Range) to over 66% (in the Międzygórze Jabłonkowsko-Koniakowskie Upland). The lowest values are found in the Hornomoravský úval Basin (3.6%) (Figure 8).
Categories four and two prevail in 22.5 and 20.3%, respectively, of the area of the Western Carpathians. Category four prevails in 26 analyzed mesoregions, reaching values from 42% in the Tatry Reglowe Mts. to 55.6% in the Čergov Mountains. The lowest percentage of this category is found in the Hornomoravský úval Basin (0.2%) (Figure 8).
Category two prevails in 11 mesoregions and the maximum values range from 38.6% (the Doły Jasielsko-Sanockie Basin) to 58.2% (the Litenčická pahorkatina Foothills). The lowest percentage is in the Western Tatra Mts. (0.4%) (Figure 8).
The lowest degree of geodiversity is found in 11.4% of the analyzed area of the Western Carpathians. The highest percentages of this category occur in 12 mesoregions, with values ranging from 39% in the Hornonitrianska kotlina Basin to 73.5% in the Hornomoravský úval Basin. The lowest percentage of this category, too, is found in the Western Tatras (0.02%).
The highest geodiversity value 5 covers only 6.2% of the area of the entire Western Carpathians and prevails in the high mountain areas (the Tatra Mts. and the Nízke Tatry Mts.), where values exceed 45%, and in the Belianske Tatry Mts., values reach 66%. In four mesoregions, the Kyjovská pahorkatina Foothills, the Litenčická pahorkatina Foothills, the Hornomoravský úval Basin, and the Kotlina Żywiecka Basin, this category does not occur at all, and in another 54 mesoregions, it covers an area of less than 1% (Figure 8).
The above analysis is undoubtedly most heavily influenced by diversity of relief, values of relative heights, and geological structure. The results of these analyses confirm the assumption of the authors of this article that values of geodiversity will be highest in areas with high-mountain features and lowest in wide valleys and inter-mountain basins.
Geodiversity vs Geotourism and Geosites
One of the measures of the geotourist potential of the research area may be the number of geosites occurring and described in this area. The map below shows the location of geosites that are included in the published databases of the Polish Geological Institute (PIG-PIB, 2014), the Slovak Geological Institute (Liščák, 2012), and the Czech Geological Survey (Interesting geosites of the Czech Republic, 2018), as well as a publication edited by Bartuś et al. (2012) and the work of Chrobak and Bąk (2019) (Figure 9). The above-mentioned databases list 1,342 geosites located in the Western Carpathians. The actual number of geosites is probably greater and includes geological sites described in Hungary, but unfortunately the Hungarian Geological Institute does not maintain a database.
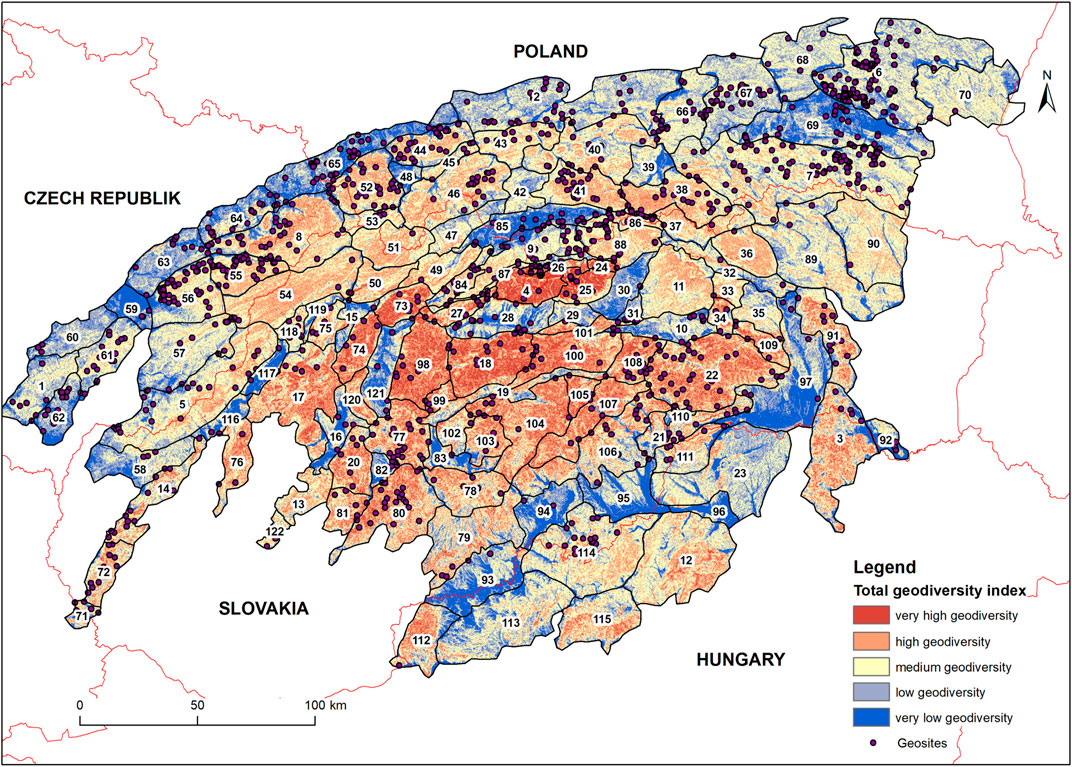
FIGURE 9. Geodiversity of the Western Carpathians vs location of geosites in the area. For numbers of mesoregions, see Figure 2. Source: own research.
The average of geosites per mesoregion is 11, but there are 13 mesoregions where not a single geosite is located; their number varies between one and nine in 63 mesoregions and in 44 between 10 and 40. The Beskid Niski Mts. and Pogórze Dynowskie Foothills are worth mentioning, with 89 and 108 geosites, respectively.
By correlating the number of geosites in individual mesoregions with the average geodiversity class in the mesoregion, it was found that there was no correlation (r = ˗0.00961). This is also visible on the map, where, although there are many geosites located within high and very high geodiversity areas (classes 4 and 5), a large percentage of geosites (especially in the northern part of the Western Carpathians and within the intra-mountain basins) is located in areas characterized by low and very low geodiversity. Such a distribution of geosites is conditioned by several variables: first of all, the knowledge and experience of the people who created the databases and their subjective assessment of the objects they placed in them, which include communication accessibility, scientific and educational value of geosites, esthetic and economic value, etc. For example, a large number of geosites located within the Beskid Niski Mountains and the Pogórze Dynowskie Foothills is related not only to the geological structure and relief but also to remnants of mining activities and oil extraction, which have become the main attraction of a geopark that is being planned in this region: the Geopark Kraina Wisłoka–The Polish Texas (Wasiluk, 2013; Wasiluk et al., 2016).
Discussion
This article shows for the first time the geodiversity approach for the entire province (in terms of the physical and geographical division) of the Western Carpathians. This article covers several aspects that we now want to address in the discussion. They were divided into three parts: 1) discussion of the method in the light of the cited literature; 2) strengths and weaknesses of the method application in relation to the obtained results; and 3) the importance of geodiversity in geotourism.
Discussion of the Method in the Light of the Cited Literature
As presented at the beginning, this method is the result of studies and an attempt to modify the method proposed by Zwoliński (Zwoliński, 2004, 2009, 2009, 2010) and is a qualitative–quantitative method (Zwoliński et al., 2018). The modification proposed by the authors manifests in several aspects. First of all, hexagons were used as the basic field for the analysis of GIS, and these have so far usually been used in biodiversity analyses (e.g., Jurasinski and Beierkuhnlein, 2006), but have also been mentioned by Kot (Kot, 2015, 2018). The authors decided to use hexagons in the analysis for one main reason, the hexagons filling all the areas by replication; it provides less variations on distances between its center and its outer limit (Zhang, 2005), and this is also important for the data presentation in the final maps. Also, hexagon is a shape that is common in nature and that precisely fills an analyzed area (e.g., honeycombs, basalt columns, insects multiple vision, snowflakes, etc.).
Another element is the component maps of the synthetic geodiversity map. For the analysis of geodiversity, several components are most often used, which are primarily relief models, land cover, lithology, soil cover, hydrographic network, etc. (Zwoliński, 2009, 2010; Zwoliński and Stachowiak, 2012; Kot, 2015; Fernández et al., 2020).
It is still a controversial issue to assign appropriate valuation classes and their criteria. In all the described quantitative and qualitative methods (Zwoliński et al., 2018), these classes and their criteria are determined by expert analysis. This is usually done by one or more people (experts) through discussions, using their knowledge and experience to agree on the most appropriate values. This is also how it was done in this article, where the knowledge and experience of the authors were additionally supported by the analysis of the literature and the values that Zwoliński (2009) used in his analysis of the geodiversity of the Polish part of the Carpathians.
The use of the qualitative questionnaire method to examine the views of a larger group of experts and to select classes and criteria in a more objective way may turn out to be an innovative concept here (Jankowski et al., 2020).
The next element concerns the selection of elements–relief types from the digital terrain model. In this case, the authors used another innovative tool–geomorphons. Determining the value of geodiversity for individual categories also carries a certain degree of subjectivity. However, in combination with the energy of the relief, the layer of geomorphons proved to be a suitable tool for assessing the geodiversity of large areas.
Creating a synthetic map of geodiversity for such a large area as the Western Carpathians is not easy, mainly due to the generalization of component maps, where it is not always possible to notice small elements that may undoubtedly be important from the point of view of geotourism. However, such maps are also needed to designate areas predisposing for more detailed geodiversity analyzes or the creation of geoparks, such as in Brazil (de Paula Silva et al., 2021; Dias et al., 2021), Hungary (Pál and Albert, 2021), Portugal (Pereira et al., 2012), or South Africa (Kori et al., 2019). It is true that the methodology of the above-mentioned analyzes is quite different from the one presented in this article, but in all the examples, it can be seen that the relief diversity plays a major role in creating a geodiversity map.
The most problematic seems to be the geological (lithological) analysis, because so far no way has been found to objectively classify rock types according to the relevant criteria. The criteria used in the lithological geodiversity map in this article somehow refer to those proposed by, for example, Zwoliński and Stachowiak (2012), de Paula Silva et al. (2021), or Fernández (2020). However, it is worth mentioning here a fairly important element, which is not only determining the criteria of individual lithological forms on the basis of their characteristic features and assigning them appropriate values but also reflecting on their diversity resulting from the number of different types of rocks in a given unit (e.g., tectonic) (Chrobak, 2018).
To sum up, the methodology of creating a geodiversity map is subject to constant changes and improvements, but there are still elements that need improvement.
The Importance of Geodiversity in Geotourism Development in the Western Carpathians
Geotourism as a form of cognitive tourism, where the main purpose of traveling is to discover and learn about forms and phenomena related to abiotic nature (e.g., Hose, 1995, 2000, 2008, 2011; Joyce, 2006; Newsome and Dowling, 2006, 2010; Ólafsdóttir, 2019). is rapidly developing in mountainous areas, including in individual parts of the Western Carpathians (e.g., Krobicki and Golonka, 2008; Golonka et al., 2012; Štrba et al., 2014; Chrobak, 2015, 2016; Kubalíková and Kirchner, 2016; Szczęch et al., 2016; Chrobak and Bąk, 2019; Kubalíková, 2019). Undoubtedly, the geodiversity map can be an ideal background for the elements of abiotic nature that are attractive for tourism and are characterized by high cognitive, educational, esthetic, ecological, and economic values. Interpretation of these elements (geosites), despite the fact that they are subject to separate evaluation, often refers to a broader geological background, which is characteristic of a mountain massif. Thus, the description of a geological site must be located at the appropriate geological time and enriched with explanations of the entire spectrum of endogenous and/or exogenous processes that led to the formation of this geological site. Thus, when describing the background, we simultaneously characterize the geodiversity of the wider area in which a given geohabitat is located, taking into account its cognitive and educational values (Schumann et al., 2015).
Experts describing the values and tourist attractions of individual mountain ranges or defined areas in the Western Carpathians, e.g., the Tatra Mountains (Zwoliński and Stachowiak, 2012); the Polish part of the Carpathians (Zwoliński, 2009, 2010); the Pieniny Mts. (Golonka et al., 2012), Podtatrze (Chrobak, 2016, 2018; Chrobak and Bąk, 2019; Chrobak et al., 2020); Spiš (Štrba et al., 2014); Moravia (Kubalíková, 2019), etc., have repeatedly characterized the geodiversity of these areas in order to draw attention to individual elements visible in the places they describe.
The synthetic geodiversity map allows comprehensively to assess the geodiversity of the research area. This map can also serve a starting point to indicate areas of exceptional natural values and then to select specific objects that could constitute a tourist attraction, geosites. So far, however, the assessment of geodiversity (surface) and assessment of geosites (point) have not been analyzed together. Geosites were assessed using other valuation methods related to the assessment of a specific site and not the entire area (e.g., Mucivuna et al., 2019, and references there in). Therefore, the lack of correlation between these variables (r = ˗0.0091) is not strange, but it does not mean that in places with very high geodiversity, there are no interesting places that could become geosites. In such a large area as the Western Carpathians, it is difficult to objectively assess the geotourist potential. From the above analyses, it can be described as average, but when we take into account individual mesoregions, there are areas with a very high geotouristic potential, with a high geodiversity and a large number of geosites (e.g., the Nízke Tatry Mts., the northern part of the Tatra Mts., the Malá Fatra Mts., the Slovenský raj (Stracenskie) Mts., and the Muránska planina Mts.), mesoregions with a low geodiversity, but a large number of geosites resulting from local conditions that are not visible on such a small scale (the Beskid Niski Mountains, the Pogórze Dynowskie Foothills, the Orawsko-Nowotarska Basin, and the Pogórze Śląskie Foothills), as well as mesoregions with a low geodiversity and a small number or lack of geosites (the Rimavská kotlina Basin, the Košická kotlina Basin, the Ipeľská kotlina Basin, and the Hornomoravský úval Basin).
Areas with exceptional natural features, which are also not best included in this geodiversity analysis due to the too small scales and large generalization of the map, are karst areas (Slovenský kras Karst Area, Spišsko-gemerský kras Karst Area, as well as the karst on the Mesozoic formations within Nízke Tatry Mts., Tatra Mts., Veľká Fatra Mts., and Malá Fatra Mts.). These areas are currently one of the most popular tourist destinations and require special protection. Groundwater, which created picturesque karst forms, is in great danger when it comes to anthropogenic impacts; therefore, sustainable development in the creation of new geoattractions in this area must be based on the principles of geoethics, because humanity in the future may depend much more on these water reserves (Gorelick et al., 1983; Kačaroğlu, 1999; Mammola et al., 2019).
Nevertheless, the presentation of general geodiversity for a large mountain area indicates those places that are worth exploring in more detail and analyzing in more detail. Certainly, further research by the authors of this article will focus on checking and perhaps increasing the correlation between the geodiversity of a research area and its geotourist potential, expressed primarily in the number of geosites.
The area of the Western Carpathians is very diverse. There are many valuable natural elements that must be properly protected, and the local authorities must base themselves on the principles of sustainable development and geoetics when creating new geotourist products. A positive example of this type of activity is the creation of geoparks that combine nature protection with rational management of its resources but also support local people by promoting culture and local products (Henriques and Brilha, 2017). There is currently only one geopark in the Western Carpathians, which has been included in the UNESCO Global Geoparks List, Novohrad-Nógrád UNESCO Global Geopark. Several other geoparks have the status of national geoparks: Banskoštiavnický Geopark, Banskobystrický Geopark, and Geopark Malé Karpaty (https://www.geopark.sk/). Other geoparks are also planned: Pieniński Geopark (Golonka et al., 2012, 2014) or Zemplinski Geopark (https://www.geopark.sk/). An area of exceptional natural values, which has been confirmed by a high geodiversity index, rich in folklore, culture, and history, which could also claim to be a geopark, is the Podtatrze area (Chrobak, 2014, 2016; Chrobak and Bąk, 2019).
Therefore, it can be clearly stated that the analysis of geodiversity of a given area is an essential element in creating further geo (tourist)-products.
Conclusion
The multifaceted analysis and discussion of geodiversity and geotourist potential arrived at the following conclusions:
- The geodiversity map presented in the article is another modification of the map algebra proposed by Zwoliński (2009).
- The use of geomorphons, hexagons, and the AHP calculator reduces the subjectivity resulting from expert analysis, but does not eliminate it completely.
- Geological analysis, which is 100% based on the knowledge and experience of experts, is still subjective.
- The geodiversity analysis for such a large area as the Western Carpathians is the first step in delineating areas requiring more detailed analysis of geotourist potential.
- There is no correlation between geodiversity and geotourist potential as determined by number and location of geosites.
- The geodiversity map is also very good background for the distribution of geotourist sites.
- The applied geodiversity map, although still subjective, is sufficiently optimal that it can also be used in other mountain areas by adjusting the class intervals accordingly.
- The creation of new geotourist attractions or better sharing of already existing geosites should be based on the principles of sustainable development in geoethics.
Data Availability Statement
The raw data supporting the conclusions of this article will be made available by the authors, without undue reservation.
Author Contributions
AC is the main author who deals with geodiversity in this research. JN is a geomorphologist. He made a physical and geographical description of the research area and statistical analyzes for geodiversity maps and also contributed significantly to writing and editing the paper. PS is the main developer of geodiversity maps in GIS programs.
Funding
The research was funded by Pedagogical University of Krakow, grants: BN.610-408/PBU/2020 and BN.711-183/PBU/2021, and by Vedecká Grantová Agentúra MŠVVaŠ SR a SAV, grant: 2/0019/21. The open-access publication fees were funded by Pedagogical University of Krakow, grants: BN.610-408/PBU/2020 and BN.711-183/PBU/2021.
Conflict of Interest
The authors declare that the research was conducted in the absence of any commercial or financial relationships that could be construed as a potential conflict of interest.
Publisher’s Note
All claims expressed in this article are solely those of the authors and do not necessarily represent those of their affiliated organizations, or those of the publisher, the editors, and the reviewers. Any product that may be evaluated in this article, or claim that may be made by its manufacturer, is not guaranteed or endorsed by the publisher.
Acknowledgments
The authors would like to thank the reviewers for their comments that helped to improve the manuscript.
References
Abukari, H., and Mwalyosi, R. B. (2020). Local Communitie's Perceptions About the Impact of Protected Areas on Livelihoods and Community Development. Glob. Ecol. Conservation 22, e00909. doi:10.1016/j.gecco.2020.e00909
AHC (2002). Australian Natural Heritage Charter for the Conservation of Places of Natural Heritage Significance. Sydney: AHC1.
Albani, R. A., Mansur, K. L., Carvalho, I. d. S., and Santosdos, W. F. S. d. (2020). Quantitative Evaluation of the Geosites and Geodiversity Sites of João Dourado Municipality (Bahia-Brazil). Geoheritage 12, 46. doi:10.1007/s12371-020-00468-1
Antić, A., Peppoloni, S., and Di Capua, G. (2020). Applying the Values of Geoethics for Sustainable Speleotourism Development. Geoheritage 12, 73. doi:10.1007/s12371-020-00504-0
Argyriou, A. V., Sarris, A., and Teeuw, R. M. (2016). Using Geoinformatics and Geomorphometrics to Quantify the Geodiversity of Crete, Greece. Int. J. Appl. Earth Observation Geoinformation 51, 47–59. doi:10.1016/j.jag.2016.04.006
Balatka, B., and Kalvoda, J. (2006). Geomorfologické Členění Reliéfu Čech. Praha: Kartografie Praha.
Balon, J., Jodłowski, M., and Krąż, P. (2015). “Regiony Fizycznogeograficzne Karpat Zachodnich,” in Atlas Tatr - Przyroda Nieożywiona. Editors K. Dąbrowska, and M. Guzik (Zakopane: TPN).
Bandura, P., Minár, J., and Drăguţ, L. (2019). Morphometrical-Morphostructural Subdivision of the Western Carpathians by Object-Based Image Analysis. Geomorphol. Slovaca Bohem. 19, 1–102.
Bartuś, T., Bębenek, S., Doktor, M., Golonka, J., Ilcewicz-Stefaniuk, D., Joniec, A., et al. (2012). Katalog Obiektów Geoturystycznych w Obrębie Pomników i Rezerwatów Przyrody Nieożywionej. Editor T. Słomka (Kraków: Akademia Górniczo-Hutnicza).
Benito-Calvo, A., Pérez-González, A., Magri, O., and Meza, P. (2009). Assessing Regional Geodiversity: The Iberian Peninsula. Earth Surf. Process. Landforms 34, 1433–1445. doi:10.1002/esp.1840
Birkenmajer, K. (1986). Stages of Structural Evolution of the Pieniny Klippen Belt, Carpathians. Stud. Geol. Pol. 88, 7–32.
Bradbury, J. (2014). A Keyed Classification of Natural Geodiversity for Land Management and Nature Conservation Purposes. Proc. Geologist's Assoc. 125, 329–349. doi:10.1016/j.pgeola.2014.03.006
Braun, G., Mutke, J., Reder, A., and Barthlott, W. (2019). “Biotope Patterns, Phytodiversity and Forestline in the Andes, Based on GIS and Remote Sensing Data,” in Mountain Biodiversity. Editors C. H. Körner, and E. M. Spehn (Routledge: Taylor & Francis Group), 75–89.
Brilha, J. (2016). Inventory and Quantitative Assessment of Geosites and Geodiversity Sites: a Review. Geoheritage 8, 119–134. doi:10.1007/s12371-014-0139-3
Brilha, J. (2018). “Geoheritage: Inventories and Evaluation,” in Geoheritage. Assessment, Protection, and Management. Editors E. Reynard, and J. Brilha (Amsterdam: Elselvier), 69–85. doi:10.1016/B978-0-12-809531-7.00004-6
Burlando, M., Firpo, M., Queirolo, C., Rovere, A., and Vacchi, M. (2011). From Geoheritage to Sustainable Development: Strategies and Perspectives in the Beigua Geopark (Italy). Geoheritage 3, 63–72. doi:10.1007/s12371-010-0019-4
Carruana Herrera, D. I., and Martínez Murillo, J. F. (2020). Valoración de la geodiversidad del Parque Natural y Geoparque de las Sierras Subbéticas (Córdoba, sur de España). Cuaternario y Geomorfología 34, 5. doi:10.17735/CYG.V34I1-2.75529
Chrobak, A. (2014). “Geotourism in the Podhale Region as an Opportunity to Reduce the Tourist Overpopulation in the Polish Part of the Tatra Mountains,” in GEOTOUR & IRSE. Editor Ľ. Štrba (Košice: Technical University of Košice), 181–197.
Chrobak, A. (2015). Geotourism Potential in the Podhale, Orava, Liptov and Spiš Regions (Southern Poland/Northern Slovakia). Acta Geoturistica 6, 1–10.
Chrobak, A. (2016). Valorisation and Categorisation of the Geosites in the Podtatrze Area (Southern Poland). Geotour. 46-47, 3–26. doi:10.7494/geotour.2016.46-47.3
Chrobak, A. (2018). Analiza i Ocena Potencjału Geoturystycznego Podtatrza. Praca Dokt. Instytut Geografii: Kraków: Uniwersytet Pedagogiczny w Krakowie.
Chrobak, A., and Bąk, K. (2019). Poznawczo-edukacyjne Aspekty Atrakcji Geoturystycznych Podtatrza. Kraków: Wydawnictwo Naukowe UP.
Chrobak, A., Ugolini, F., Pearlmutter, D., and Raschi, A. (2020). Thermal Tourism and Geoheritage: Examining Visitor Motivations and Perceptions. Resources 9, 58. doi:10.3390/resources9050058
Coratza, P., Reynard, E., and Zwoliński, Z. (2018). Geodiversity and Geoheritage: Crossing Disciplines and Approaches. Geoheritage 10, 525–526. doi:10.1007/s12371-018-0333-9
CORINE Landcover map (2018). Available at: https://land.copernicus.eu/pan-european/corine-land-cover (Accessed October 15, 2020).
de Paula Silva, J., Alves, G. B., Ross, J. L. S., de Oliveira, F. S., do Nascimento, M. A. L., Felini, M. G., et al. (2021). The Geodiversity of Brazil: Quantification, Distribution, and Implications for Conservation Areas. Geoheritage 13, 75. doi:10.1007/s12371-021-00598-0
de Paula Silva, J., Rodrigues, C., and Pereira, D. I. (2015). Mapping and Analysis of Geodiversity Indices in the Xingu River Basin, Amazonia, Brazil. Geoheritage 7, 337–350. doi:10.1007/s12371-014-0134-8
De Waele, J., Di Gregorio, F., and Pala, A. (2005). Karst Geomorphosites of Monte Albo (north-east Sardinia).Il. J. Quat. Sci. 18 (1), 143–151.
Dias, M. C. S. S., Domingos, J. O., dos Santos Costa, S. S., do Nascimento, M. A. L., da Silva, M. L. N., Granjeiro, L. P., et al. (2021). Geodiversity Index Map of Rio Grande Do Norte State, Northeast Brazil: Cartography and Quantitative Assessment. Geoheritage 13, 10. doi:10.1007/s12371-021-00532-4
Dixon, G. (1996). Geoconservation: An Intrenational Review and Strategy for Tasmania.,” in Parks and Wildlife Service. Tasmania: Ocasional Paper 35.
Fernández, A., Fernández, T., Pereira, D. I., and Nieto, L. M. (2020). Assessment of Geodiversity in the Southern Part of the Central Iberian Zone (Jaén Province): Usefulness for Delimiting and Managing Natural Protected Areas. Geoheritage 12, 1–16. doi:10.1007/s12371-020-00447-6
Forte, J. P., Brilha, J., Pereira, D. I., and Nolasco, M. (2018). Kernel Density Applied to the Quantitative Assessment of Geodiversity. Geoheritage 10, 205–217. doi:10.1007/s12371-018-0282-3
Franko, O., and Melioris, L. (2000). Minerálne a Termálne Vody Slovenska - Vznik a Rozšírenie. Podzemn. Voda. 6, 5–28.
Froitzheim, N., Plašienka, D., and Schuster, R. (2008). “Alpine Tectonics of the Alps Ans Western Carpathians,” in The Geology of Central Europe: Mesozoic and Cenozoic. Editor T. McCann (London: The Geological Society), 1141–1232.
Golonka, J., Doktor, M., Krobicki, M., Miskiewicz, K., Bartuś, T., Stadnik, R., et al. (2012). “Transgraniczny Geopark Pieniński Jako Stymulator Rozwoju Regionu,” in Rozwój Turystyki Przyrodniczej i Kulturowej na Pograniczu Polsko-Słowackim, Nowy Targ, 45–54.
Golonka, J., Doktor, M., Miśkiewicz, K., Krobicki, M., and Słomka, T. (2014). Selected Geosites Within a Proposed New Trans-Border Pieniny Geopark (Polish-Slovakian). Acta Geoturistica 5, 46–63.
González-Amuchastegui, M., and Serrano, E. (2018). An Essential Tool for Natural Heritage Management: The Geomorphological Map of Valderejo Natural Park. Geosciences 8, 250. doi:10.3390/geosciences8070250
Gordon, J. E., Barron, H. F., Hansom, J. D., and Thomas, M. F. (2012). Engaging With Geodiversity-Why it Matters. Proc. Geologist's Assoc. 123, 1–6. doi:10.1016/j.pgeola.2011.08.002
Gordon, J. E. (2019). Geoconservation Principles and Protected Area Management. Int. J. Geoheritage Parks 7, 199–210. doi:10.1016/j.ijgeop.2019.12.005
Gorelick, S. M., Evans, B., and Remson, I. (1983). Identifying Sources of Groundwater Pollution: An Optimization Approach. Water Resour. Res. 19, 779–790. doi:10.1029/wr019i003p00779
Gray, M. (2005). Geodiversity and Geoconservation:What, Why, and How? George Wright Forum 22 (3), 4–12.
Gray, M. (2008). Geodiversity: The Origin and Evolution of a Paradigm. Geol. Soc. Lond. Spec. Publications 300, 31–36. doi:10.1144/SP300.4
Gray, M. (2013). Geodiversity: Valuing and Conserving Abiotic Nature. 2nd Edition. Chichester: Wiley-Blackwell.
Gray, M. (2018a). “Geodiversity,” in Geoheritage: Assessment, Protection, and Management. Editors E. Reynard, and J. Brilha (Amsterdam: Elselvier), 13–25. doi:10.1016/b978-0-12-809531-7.00001-0
Gray, M. (2018b). The Confused Position of the Geosciences within the “Natural Capital” and “Ecosystem Services” Approaches. Ecosystem Serv. 34, 106–112. doi:10.1016/j.ecoser.2018.10.010
Gray, M. (2019). Geodiversity, Geoheritage and Geoconservation for Society. Int. J. Geoheritage Parks 7, 226–236. doi:10.1016/j.ijgeop.2019.11.001
Guthrie, M. (2005). The Social and Economic Value of Geodiversity. Available at: www.geoconservation.com/EHWH/Conference/%0Apresentations/guthrie.htm
Halvorsen, R., Skarpaas, O., Bryn, A., Bratli, H., Erikstad, L., Simensen, T., et al. (2020). Towards a Systematics of Ecodiversity: The EcoSyst Framework. Glob. Ecol. Biogeogr. 29, 1887–1906. doi:10.1111/geb.13164
Henriques, M. H., and Brilha, J. (2017). UNESCO Global Geoparks: a Strategy Towards Global Understanding and Sustainability. Episodes 40, 349–355. doi:10.18814/epiiugs/2017/v40i4/017036
Herrera-Franco, G., Carrión-Mero, P., Mora-Frank, C., and Caicedo-Potosí, J. (2020). Comparative Analysis of Methodologies for the Evaluation of Geosites in the Context of the Santa Elena-Ancón Geopark Project. Int. J. Des. Nat. Ecodynamics 15, 183–188. doi:10.18280/ijdne.150207
Hjort, J., and Luoto, M. (2012). Can Geodiversity Be Predicted from Space? Geomorphology 153-154, 74–80. doi:10.1016/j.geomorph.2012.02.010
Hók, J., Šujan, M., and Šipka, F. (2014). Tektonické Členenie Západných Karpát - Prehl’ad Názorov a Nový Prístup. Acta Geol. Slovaca 6, 135–143.
Hók, J., Kahan, Š., and Aubrecht, R. (2001). Geológia Slovenska. Bratislava: Univerzita Komenského. 1–48.
Hose, T. A. (2000). European Geotourism – Geological Interpretation and Geoconservation Promotion for Tourists. Geological Heritage: Its Conservation and Management. Editors D. Barretino, W. A. P. Wimbledon, and E. Gallego (Madrid: Instituto Tecnologico GeoMinero de Espana), 127–146.
Hose, T. A. (2008). Towards a History of Geotourism: Definitions, Antecedents and the Future. Geol. Soc. Lond. Spec. Publications 300, 37–60. doi:10.1144/SP300.5
Hose, T. A. (2011). The Genesis of Geotourism and its Management Implications. Geotourism a Variety of Aspects. Editor T. Słomka (Kraków), 23–38.
Interesting geosites of the Czech Republic (2018). Interesting Geosites of the Czech Republic. Czech Geol. Surv. Available at: https://mapy.geology.cz/geosites (Accessed October 15, 2020).
Jačková, K., and Romportl, D. (2008). The Relationship Between Geodiversity and Habitat Richness in Šumava National Park and Křivoklátsko PLA (Czech Republic): A Quantitative Analysis Approach. J. Landsc. Ecol. 1, 23–38. doi:10.2478/v10285-012-0003-6
Jankowski, P., Najwer, A., Zwoliński, Z., and Niesterowicz, J. (2020). Geodiversity Assessment With Crowdsourced Data and Spatial Multicriteria Analysis. ISPRS Int. J. Geo-Information 9, 716–16. doi:10.3390/ijgi9120716
Jasiewicz, J., and Stepinski, T. F. (2013). Geomorphons - a Pattern Recognition Approach to Classification and Mapping of Landforms. Geomorphology 182, 147–156. doi:10.1016/j.geomorph.2012.11.005
Jaskulska, A., Reynard, E., and Zwoliński, Z. (2013). Geodiversity Assessment for Environmental Management of Geomorphosites: Derborence and Illgraben, Swiss Alps. Geophys. Res. Abstr. 1.
Jedicke, E. (2001). Biodiversität, Geodiversität, Ökodiversität. Kriterien zur Analyse der Landschaftsstruktur – ein konzeptioneller Diskussionsbeitrag. Naturschutz und Landschaftsplan. 3, 59–68.
Joyce, B. (2006). Geomorphological Sites and the New Geotourism in Australia. Available at: web.earthsci.unimelb.edu.au.
Jurasinski, G., and Beierkuhnlein, C. (2006). Spatial Patterns of Biodiversity-Assessing Vegetation Using Hexagonal Grids. Biol. Environ. Proc. R. Irish Acad. 106, 401–411. doi:10.3318/bioe.2006.106.3.401
Kačaroğlu, F. (1999). Review of Groundwater Pollution and protection in Karst Areas. Water Air Soil Pollut. 113, 337–356. doi:10.1023/A:1005014532330
Kale, V. S. (2015). A 10-digit Geo-Coding System for Classification of Geomorphosites in India. EGU Gen. Assem., 1861.
Kirchner, K., and Kubalíková, L. (2014). “Geosite and Geomorphosite Assessment for Geotourism Purpose: A Case Study from the Vizovická Vrchovina highland, Eastern moravia,” in Public Recreation and Landscape Protection - With Man Hand in Hand? 2014 Conference Proceeding, 131–141.
Kori, E., Onyango Odhiambo, B. D., Chikoore, H., and Chikoore, H. (2019). A Geomorphodiversity Map of the Soutpansberg Range, South Africa. Landform Anal. 38, 13–24. doi:10.12657/landfana-038-002
Kostrzewski, A. (1998). “Georóżnorodność Rzeźby jako Przedmiot Badań Geomorfologii,” in Główne Kierunki Badań Geomorfologicznych w Polsce; Stan Aktualny i Perspektywy. IV Zjazd Geomorfologów Polskich (Lublin: UMCS), 260–277.
Kostrzewski, A. (2011). The Role of Relief Geodiversity in Geomorphology. Geogr. Pol. 84, 69–74. doi:10.7163/GPol.2011.S2.5
Kot, R. (2015). The point Bonitation Method for Evaluating Geodiversity: A Guide with Examples (Polish Lowland). Geografiska Annaler: Ser. A, Phys. Geogr. 97, 375–393. doi:10.1111/geoa.12079
Kot, R. (2018). A Comparison of Results from Geomorphological Diversity Evaluation Methods in the Polish Lowland (Toruń Basin and Chełmno Lakeland). Geografisk Tidsskrift-Danish J. Geogr. 118, 17–35. doi:10.1080/00167223.2017.1343673
Kot, R., and Leśniak, K. (2006). Ocena Georóżnorodności za Pomocą Miar Krajobrazowych-Podstawowe Trudności Metodyczne. Przegląd Geogr. 78, 25–45.
Kozłowski, S. (2004). Geodiversity. The Concept and Scope of Geodiversity. Prz. Geol. 52 (8/2), 833–837.
Kozłowski, S., Migaszewski, M., and Gałuszka, A. (2004a). “Geodiversity Conservation-Conservating Our Geological Heritage,” in Geological Heritage Concept, Conservation and Protecion Policy in Central Europe. Editors A. Ber, and Z. Alexandrowicz (Polish Geological Institute Special Papers), 13–20.
Kozłowski, S., Migaszewski, M., and Gałuszka, A. (2004b). Znaczenie Georóżnorodności w Holistycznej Wizji Przyrody. Przegląd Geol. 52, 291–294.
Krobicki, M., and Golonka, J. (2008). Geotouristical Values of the Pieniny Klippen Belt and Tatra Mountains Regions (Poland). Przegląd Geol. 56, 670–711.
Kubalíková, L. (2019). Assessing Geotourism Resources on a Local Level: A Case Study From Southern Moravia (Czech Republic). Resources 8, 150. doi:10.3390/resources8030150
Kubalíková, L., and Kirchner, K. (2016). Geosite and Geomorphosite Assessment as a Tool for Geoconservation and Geotourism Purposes: a Case Study From Vizovická Vrchovina Highland (Eastern Part of the Czech Republic). Geoheritage 8, 5–14. doi:10.1007/s12371-015-0143-2
Lačný, A., Plašienka, D., and Vojtko, R. (2016). Structural Evolution of the Turňa Unit Constrained by Fold and Cleavage Analyses and its Consequences for the Regional Tectonic Models of the Western Carpathians. Geol. Carpathica 67, 177–193. doi:10.1515/geoca-2016-0012
Lexa, J., Bezák, M., Elečko, M., Mello, J., Polák, M., Potfaj, M., et al. (2000). Geological Map of Western Carpathians and Adjacent Areas, 1: 500 000. Bratislava: Geological Survey of Slovak Republic.
Lindner, L., Dzierżek, J., Marciniak, B., and Nitychoruk, J. (2003). Outline of Quaternary Glaciations in the Tatra Mts.: Their Development, Age and Limits. Geol. Q. 47, 269–280.
Liščák, P. (2012). Významné Geologické Lokality. Štátny Geol. Ústav Dionýza Štúra. Available at: http://apl.geology.sk/g_vglg (Accessed October 15, 2020).
Lóczy, D., Stankoviansky, M., and Kotarba, A. (2012). Recent Landform Evolution. The Carpatho-Balkan-Dinaric Region. Dordrecht: Springer.
Lukniš, M. (1972). “Reliéf,” in Slovensko 2 – Príroda. Editor M. Lukniš (Bratislava: Obzor), 124–202.
Maglay, J., Halouzka, R., Baňacký, V., Pristaš, J., and Janočko, J. (1999). Neotektonicka mapa Slovenska v mierke 1:500 000. Bratislava: Ministry of Environment of Slovak Republic.
Mammola, S., Cardoso, P., Culver, D. C., Deharveng, L., Ferreira, R. L., Fišer, C., et al. (2019). Scientist's Warning on the Conservation of Subterranean Ecosystems. Bioscience 69, 641–650. doi:10.1093/biosci/biz064
Mazúr, E., and Lukniš, M. (1978). Regional Geomorphological Division of the SSR. Geogr. Časopis 30, 101–125.
Mazúr, E. (1965). “Major Features of the West Carpathians in Slovakia as a Result of Young Tectonic Movements,” in Geomorphological Problems of Carpathians. Editors E. Mazúr, and O. Stehlík (Bratislava: Vydavateľstvo SAV), 9–54.
Micić Ponjiger, T., Lukić, T., Vasiljević, Ð. A., Hose, T. A., Basarin, B., Marković, S. B., et al. (2021). Quantitative Geodiversity Assessment of the Fruška Gora Mt. (North Serbia) by Using the Geodiversity Index. Geoheritage 13, 61. doi:10.1007/s12371-021-00572-w
Miklánek, P. (2012). “Rivers,” in Recent Landform Evolution. The Carpatho-Balkan-Dinaric Region. Editors D. Lóczy, M. Stankoviansky, and A. Kotarba (Dordrecht: Springer), 31–38. doi:10.1007/978-94-007-2448-8_3
Minár, J., Bielik, M., Kováč, M., Plašienka, D., Barka, I., Stankoviansky, M., et al. (2011). New Morphostructural Subdivision of the Western Carpathians: An Approach Integrating Geodynamics Into Targeted Morphometric Analysis. Tectonophysics 502, 158–174. doi:10.1016/j.tecto.2010.04.003
Mizgajski, A. (2001). “Odniesienie Georóżnorodności do Wybranych Pojęć w Naukach o Środowisku,” in Funkcjonowanie Geoekosystemów w Zróżnicowanych Warunkach Morfoklimatycznych. Monitoring, Ochrona, Edukacja. Editors A. Karczewski, and Z. Zwoliński (Poznań: Bogucki Wydawnictwo Naukowe), 369–375.
Mucivuna, V. C., Reynard, E., and Garciada, M. d. G. M. (2019). Geomorphosites Assessment Methods: Comparative Analysis and Typology. Geoheritage 11, 1799–1815. doi:10.1007/s12371-019-00394-x
Najwer, A., Borysiak, J., Gudowicz, J., Mazurek, M., and Zwoliński, Z. (2016). Geodiversity and Biodiversity of the Postglacial Landscape (Dębnica River Catchment, Poland). Quaest. Geogr. 35, 5–28. doi:10.1515/quageo-2016-0001
Najwer, A., Reynard, E., and Zwoliński, Z. (2014). GIS and Multi-Criteria Evaluation (MCE) for Landform Geodiversity Assessment. Geophys. Res. Abstr., 906. doi:10.13140/2.1.4412.0006
Najwer, A., and Zwoliński, Z. (2014). Semantyka i Metodyka Oceny Georóżnorodności - Przegląd i Propozycja Badawcza. Landform Anal. 26, 115–127. doi:10.12657/landfana.026.011
NASADEM (2020). Available at: https://earthdata.nasa.gov/esds/competitive-programs/measures/nasadem (Accessed October 15, 2020).
Naveh, Z. (2007). From Biodiversity to Ecodiversity: A Landscape-Ecology Approach to Conservation and Restoration. Restoration Ecology 2: 180-189. Ecol. Restor. Ecol. 6, 117–134. doi:10.1007/1-4020-4422-4_4
Newsome, D., and Dowling, R. (2006). “The Scope and Nature of Geotourism”. In Geotourism. Editors R. K. Dowling, and D. Newsome (Oxford, Urlington, MA: Elsevier), 3–25. doi:10.1016/B978-0-7506-6215-4.50009-9
Newsome, D., and Dowling, R. K. (2010). Geotourism. The Tourism of Geology and Landscape. Oxford: Goodfellow Publishers Limited.
Pál, M., and Albert, G. (2021). Refinement Proposals for Geodiversity Assessment-A Case Study in the Bakony-Balaton UNESCO Global Geopark, Hungary. ISPRS Int. J. Geo-Information 10, 566. doi:10.3390/ijgi10080566
Panizza, M. (2009). The Geomorphodiversity of the Dolomites (Italy): A Key of Geoheritage Assessment. Geoheritage 1, 33–42. doi:10.1007/s12371-009-0003-z
Pellitero, R., Manosso, F. C., and Serrano, E. (2015). Mid‐ and Large‐scale Geodiversity Calculation in Fuentes Carrionas (Nw spain) and Serra Do Cadeado (Paraná, brazil): Methodology and Application for Land Management. Geografiska Annaler: Ser. A, Phys. Geogr. 97, 219–235. doi:10.1111/geoa.12057
Peppoloni, S., and Di Capua, G. (2015). Geoethics: The Role and Responsibility of Geoscientists. London: Geological Society of London, Special Publications 419. doi:10.1144/SP419
Peppoloni, S., and Di Capua, G. (2016). “Geoethics: Ethical, Social, and Cultural Values in Geosciences Research, Practice, and Education,” in Geoscience for the Public Good and Global Development: Toward a Sustainable Future. Editors G. Wessel, and J. Greenberg (Geological Society of America, special paper 520), 17–21. doi:10.1130/2016.2520(03
Peppoloni, S., and Di Capua, G. (2017). Geoethics: Ethical, Social and Cultural Implications. Geosciences in Geoethics: At the Heart of All Geosciences. Annals of Geophysics 60, Fast Track 7. Editors S. Peppoloni, G. Di Capua, P. Bobrovsky, and V. Cronin. doi:10.4401/ag-7473
Peppoloni, S., Bobrowsky, P., and Di Capua, G. (2015). “Geoethics: a challenge for Research Integrity in Geosciences,” in Integrity in the Global Research Arena, World Scientific. Editors N. Steneck, M. Anderson, S. Kleinert, and T. Mayer, 287–294. doi:10.1142/9789814632393_0035
Pereira, D. I., Pereira, P., Brilha, J., and Santos, L. (2013). Geodiversity Assessment of Paraná State (Brazil): An Innovative Approach. Environ. Manage. 52, 541–552. doi:10.1007/s00267-013-0100-2
Pereira, D., Santos, L., de Paula Silva, J., Pereira, P., Brilha, J., da Silva, J. M., et al. (2012). Mapping Regional Geodiversity in Brazil and Portugal. Geol. Dell’ Ambient. 3, 111–112.
Perotti, L., Carraro, G., Giardino, M., De Luca, D. A., and Lasagna, M. (2019). Geodiversity Evaluation and Water Resources in the Sesia Val Grande UNESCO Geopark (Italy). Water. 11, 2102. doi:10.3390/w11102102
PIG-PIB (2014). Centralny Rejestr Geostanowisk Polski/Polish Central Register of Geosites. Warszawa: Państwowy Inst. Geol. - Państwowy Inst. Badaw./Polish Geol. Inst. – Natl. Res. Institute.
Plašienka, D., Grecula, P., Putiš, M., Kováč, M., and Hovorka, D. (1997). “Evolution and Structure of the Western Carpathians: an Overview”, in Geological Evolution of the Western Carpathians. Editors P. Grecula, D. Hovorka, and M. Putiš (Bratislava: Mineralia Slovaca - Monogr.), 1–24.
Rypl, J., Kirchner, K., and Ryplová, R. (2019). Contribution to the Assessment of Geomorphosites in the Czech Republic (A Case Study of the North-Eastern Part of the Novohradské Mountains). Geoheritage 11, 427–439. doi:10.1007/s12371-018-0293-0
Saaty, T. L. (1990). Multicriteria Decision Making: The Analytic Hierarchy Process. Pittsburgh, PA: RWS Publications.
Schmid, S. M., Bernoulli, D., Fügenschuh, B., Matenco, L., Schefer, S., Schuster, R., et al. (2008). The Alpine-Carpathian-Dinaridic Orogenic System: Correlation and Evolution of Tectonic Units. Swiss J. Geosci. 101, 139–183. doi:10.1007/s00015-008-1247-3
Schumann, A., Muwanga, A., Lehto, T., Staudt, M., Schlüter, T., Kato, V., et al. (2015). Ugandan Geosites. Geology Today 31, 59–67. doi:10.1111/gto.12089
Seijmonsbergen, A. C., de Jong, M. G. G., Hagendoorn, B., Oostermeijer, J. G. B., and Rijsdijk, K. F. (2018). “Geodiversity Mapping in alpine Areas,” in Mountains, Climate and Biodiversity. Editors C. Hoorn, A. Perrigo, and A. Antonelli (Hoboken, NJ: John Wiley & Sons), 155–170.
Seijmonsbergen, A., de Jong, M. G. G., de Graaff, L. W. S., and Anders, N. S. (2014). Geodiversität von Vorarlberg und Liechtenstein. Bern: Haupt Verlang.
Serrano, E., and Ruiz-Flaño, P. (2007a). Geodiversity: a Theoretical and Applied Concept. Geogr. Helv. 62, 140–147. doi:10.5194/gh-62-140-2007
Serrano, E., and Ruiz-Flaño, P. (2007b). Geodiversity: Concept, Assessment and Territorial Application. The case of Tiermes-Caracena (Soria). Boletín la Asoc. Geógrafos Españoles 45, 389–393.
Sharples, C. (2002). Concepts and Principles of Geoconservation. Tasmanian Park. Wildl. Serv. Website.
Šimo, E., and Zaťko, M. (2002). “Types of Runoff Regime 1:2 000 000,” in Atlas Krajiny Slovenskej Republiky, 103. Bratislava: Ministerstvo životného prostredia SR.
Solon, J., Borzyszkowski, J., Bidłasik, M., Richling, A., Badora, K., Balon, J., et al. (2018). Physico-geographical Mesoregions of Poland: Verification and Adjustment of Boundaries on the Basis of Contemporary Spatial Data. Geogr. Pol. 91, 143–170. doi:10.7163/gpol.0115
Stanley, M. (2004). Geodiversity – Linking People, Landscapes and Their Culture. Natural and Cultural Landscape – the Geological Foundation. Editor M. A. Parkes (Dublin: Royal Irish Academy), 47–52.
Stepinski, T. F., and Jasiewicz, J. (2011). Geomorphons - a New Approach to Classification of Landforms. Proc. Geomorphometry 109–112.
Štrba, L. u., Rybár, P., Baláž, B., Molokáč, M., Hvizdák, L., Kršák, B., et al. (2014). Geosite Assessments: Comparison of Methods and Results. Curr. Issues Tourism 18, 496–510. doi:10.1080/13683500.2014.882885
Štrba, Ľ., Kolačkovská, J., Kudelas, D., Kršák, B., and Sidor, C. (2020). Geoheritage and Geotourism Contribution to Tourism Development in Protected Areas of Slovakia-theoretical Considerations. Sustainability 12, 2979. doi:10.3390/su12072979
Szczęch, M., Cieszkowski, M., Chodyń, R., and Loch, J. (2016). Geotouristic Values of the Gorce National Park and its Surroundings (The Outer Carpathians, Poland). Geotourism 44–45. doi:10.7494/geotour.2016.44-45.27
Urbánek, J., Beták, J., Jakál, J., Lacika, J., and Novotný, J. (2009). Regional Geomorphological Division of Slovakia: Old Problem in New Perspectives in Slovak Geography at the Beginning of the 21st Century. Geogr. Slovaca 26, 237–259.
Urbánek, J., and Lacika, J. (1998). Morphostructures of the West Carpathians of Slovakia. Stud. Geomorphol. Carpatho-balcanica 32, 59–67.
Vasiljević, Đ. A., Vujičić, M. D., Božić, S., Jovanović, T., Marković, S. B., Basarin, B., et al. (2018). Trying to Underline Geotourist Profile of National Park Visitors: Case Study of NP Fruška Gora, Serbia (Typology of Potential Geotourists at NP Fruška Gora). Open Geosci. 10, 222–233. doi:10.1515/geo-2018-0017
Velázquez, V. F., Colonna, J., Sallun, A. E. M., Azevedo Sobrinho, J. M., Sallun Filho, W., and Paiva, P. C. A. (2014). The Colônia Impact Crater: Geological Heritage and Natural Patrimony in the Southern Metropolitan Region of São Paulo, Brazil. Geoheritage 6, 283–290. doi:10.1007/s12371-014-0121-0
Wasiluk, R., Radwanek-Bąk, B., Bąk, B., Kopciowski, R., Malata, T., Kochman, A., et al. (2016). A Conception of a Mountain Geopark in a SPA Region; Example of a Projected Geopark „Wisłok Valley - the Polish Texas”, in the Krosno Region. Geotour. 46-47, 43–52. doi:10.7494/geotour.2016.46-47.43
Zhang, Y. (2005). “The Cellular Concept – System Design Fundamentals,” in ECE 8708 Wireless Communications, 57–104. Available at: https://www.pearsonhighered.com/assets/samplechapter/0/1/3/0/0130422320.pdf
Zwoliński, Z. (2004). Geodiversity in Encycl. Geomorphol. Editor A. S. Goudie (New York: Routledge), 417–418. doi:10.1007/0-306-47818-8_43
Zwoliński, Z. (2009). The Routine of Landform Geodiversity Map Design Forthe Polish Carpathian Mts. Landf. Anal. 11, 77–85.
Zwoliński, Z. (2010). Aspekty Turystyczne Georóżnorodności Rzeźby Karpat. Kraj. A. Tur. Pr. Kom. Kraj. Kult. 14, 316–327.
Zwoliński, Z. (2018). Geodiversity Evaluation of the Słupsk Bank boulder Area. Bim. 33, 191–198. doi:10.5604/01.3001.0012.8088
Zwoliński, Z., and Stachowiak, J. (2012). Geodiversity Map of the Tatra National Park for Geotourism. Quaestiones Geographicae 31, 99–107. doi:10.2478/v10117-012-0012-x
Keywords: geodiversity, geoheritage, geotourism, mapping, assessment methods, Western Carpathians
Citation: Chrobak A, Novotný J and Struś P (2021) Geodiversity Assessment as a First Step in Designating Areas of Geotourism Potential. Case Study: Western Carpathians. Front. Earth Sci. 9:752669. doi: 10.3389/feart.2021.752669
Received: 03 August 2021; Accepted: 22 September 2021;
Published: 01 November 2021.
Edited by:
Dario Gioia, Istituto di Scienze del Patrimonio Culturale (CNR), ItalyReviewed by:
Aleksandar Antic, University of Novi Sad, SerbiaAlessia Pica, Sapienza University of Rome, Italy
Copyright © 2021 Chrobak, Novotný and Struś. This is an open-access article distributed under the terms of the Creative Commons Attribution License (CC BY). The use, distribution or reproduction in other forums is permitted, provided the original author(s) and the copyright owner(s) are credited and that the original publication in this journal is cited, in accordance with accepted academic practice. No use, distribution or reproduction is permitted which does not comply with these terms.
*Correspondence: Anna Chrobak, YW5uYS5jaHJvYmFrQHVwLmtyYWtvdy5wbA==