- 1Department of Earth Sciences, Uppsala University, Uppsala, Sweden
- 2Department of Geology, Lund University, Lund, Sweden
- 3Department of Geology, United Arab Emirates University, Al Ain, United Arab Emirates
- 4Tandem Laboratory, Uppsala University, Uppsala, Sweden
Understanding the transport and deposition of the cosmogenic isotope 10Be is vital for the application of the isotope data to infer past changes of solar activity, to reconstruct past Earth’s magnetic field intensity and climate change. Here, we use data of the cosmogenic isotope 10Be from the Greenland ice cores, namely the NEEM and GRIP ice cores, to identify factors controlling its distribution. After removing the effects of the geomagnetic field on the cosmogenic radionuclide production rate, the results expose imprints of the 20–22 ka precession cycle on the Greenland 10Be records of the last glacial period. This finding can further improve the understanding of 10Be variability in ice sheets and has the prospect of providing better reconstructions of geomagnetic and solar activity based on cosmogenic radionuclide records.
Introduction
The cosmogenic radionuclides (e.g., 10Be) from different natural archives can provide important information of past changes in the solar and geomagnetic field (e.g., Muscheler et al. (2005a), Muscheler et al. (2007), Muscheler et al. (2005b), Zheng et al. (2021a)) and climate changes (e.g., Beck et al., 2018). Consequently, understanding factors controlling the cosmogenic radionuclide deposition on long-time scales is important for its applications. Effects of Earth’s orbital forcing, including precession (change in the direction of the Earth’s axis with a cycle of about 19–23 ka), obliquity (the axial tilt which changes between 22.1° and 24.5°with a cycle of about 41 ka) and eccentricity (how round or elliptic the Earth’s orbit is and consists of cycles of 413, 125 and 95 ka that loosely combine into a 100°ka cycle) on the Earth’s climate have been known for a long time (Supplementary Figure S1 and details therein). These changes, which are termed as Milankovitch cycles, result in variability of the insolation (W/m2) of solar irradiance at the top of the atmosphere dependent on positions on Earth with time (Berger, 1988; Berger and Loutre, 1991). The summer insolation at 65°N is considered critical to the growth and decay of the northern hemisphere ice sheets. Reproduction of the Milankovitch orbital forcing cycles in the Earth’s natural climate archives has been crucial for the understanding of the driving forces of climate change over the last million years. In addition to these long term climatic changes, the climate records of the last glacial period are punctuated by shorter-term events, such as the Heinrich and the Dansgaard-Oeschger events, at variable amplitudes (Alley, 2000; Barker et al., 2011).
As galactic cosmic rays (GCR) interact with nitrogen and oxygen in the atmosphere 10Be is produced through spallation (Lal and Peters, 1967). It is well known that the 10Be production rate depends on the solar- and geomagnetic modulation of GCR reaching the Earth’s atmosphere. The effects of these modulations are found in the 10Be records as solar cycles (Muscheler et al., 2007; Berggren et al., 2009) and geomagnetic events (such as the Laschamps geomagnetic excursion; e.g. Aldahan and Possnert, 1998; Bonhommet and Zahringer, 1969; Raisbeck et al., 1987). Nevertheless, the transport processes from the stratosphere where about 65% of the production of 10Be occurs and the transport within the troposphere are still major uncertainties for the interpretation of 10Be records. Furthermore, the removal of 10Be from the troposphere via wet and dry deposition is expected to change significantly with large changes in climate. Milankovitch orbital forcing could play a major role in this system as it affects atmospheric circulation driven by different heating rates in the atmosphere through local insolation differences.
Here we assess the effects of orbital forcing cycles on 10Be records of the last glacial period from the NEEM and GRIP ice cores in Greenland. The results are discussed in terms of causes of changes in the 10Be signals, the reliability of the obtained correlations with orbital forcing cycles and implications for a better understanding of aerosol transport and deposition.
Materials and Methods
The 10Be data used here are from the two longest Greenland 10Be records, namely from the NEEM (The North Greenland Eemian Ice Drilling; Zheng et al. (2021b)) and GRIP (The Greenland Ice Core Project; Adolphi et al. (2014), Muscheler et al. (2004), Vonmoos et al. (2006), Wagner et al. (2001), Yiou et al. (1997)) ice core projects (Supplementary Figure S2). The section of the GRIP record used here covers the period from 11.7 to 104 ka BP (before the present 1950 A.D.), and the NEEM data covers the period 11.7 to 108 ka BP. Yiou et al., 1997 found that some GRIP samples, which were filtered through a 0.45-micron mesh size filter before preparation, show a loss of meteoric 10Be on the filter. This loss of 10Be to total 10Be in the GRIP ice core is estimated at around 20% for the last glacial period (Baumgartner et al., 1997). To compensate for the average 10Be loss, we multiply the results for the samples filtered by 0.45-micron mesh size filter with 1.25. In addition to the 10Be concentration, the 10Be flux is calculated here by adapting calculated snow accumulation rates for the NEEM ice core from Rasmussen et al. (2013) and for the GRIP ice core from Johnsen et al. (1997) and Seierstad et al. (2014). All data are resampled at a 1000-years resolution to smooth out short-term variations.
We analyze the 10Be data in concert with the δ18O data from NEEM (NEEM community members, 2013; Schüpbach et al., 2018) and GRIP ice core (Johnsen et al., 1997; Rasmussen et al., 2014). To assess the common transport and deposition effects on aerosols and 10Be, we include the SO42- and
To investigate the climate effects on 10Be deposition, we need to correct the 10Be data for the geomagnetic field influence on the cosmogenic radionuclide production rates. We use the independent geomagnetic record PISO-1500 (Channell et al., 2009) and a marine 10Be/9Be stack (Simon et al., 2016). PISO-1500 is reconstructed through synchronizing and stacking 13 globally distributed and high-quality paleointensity records. PISO-1500 data is converted to the 10Be production signal (denoted as PISO1500 10Beprod) using the production model from Poluianov et al. (2016) with the local interstellar spectra by Herbst et al. (2017). For the calculations, we used a constant solar modulation parameter of 500 MeV. Finally, we averaged the normalized PISO1500 10Beprod and normalized marine 10Be/9Be stack to get the final production signal of 10Be (Supplementary Figure S3; denoted as 10Beprod). Also, it is important to mention that there are still large uncertainties in the geomagnetic field reconstructions as described by Panovska et al. (2019). The uncertainties in the geomagnetic data and the 10Be record likely affect the match between the two records. The 10Beprod shows high values over the 32, 40, 60–65 and near 95–100 ka BP which is related to the documented paleomagnetic excursions (Supplementary Figure S3). The 10Beprod is more comparable to the 10Be fluxes in terms of amplitude than the 10Be concentrations, which are influenced by the accumulation rates due to dilution effects.
The geomagnetic correction was performed by dividing the normalized 10Beprod from the normalized 10Be data (Savranskaia et al., 2021). The resulting records are denoted as 10Be concclimate and 10Be fluxclimate (Figure 1). We have used here linear regression Pearson correlation and factor analysis to decipher potential links between 10Be and the above-mentioned parameters. Some of the records have also been analyzed using the Fast Fourier transform (FFT) and wavelet transform methods to study periodic variations.
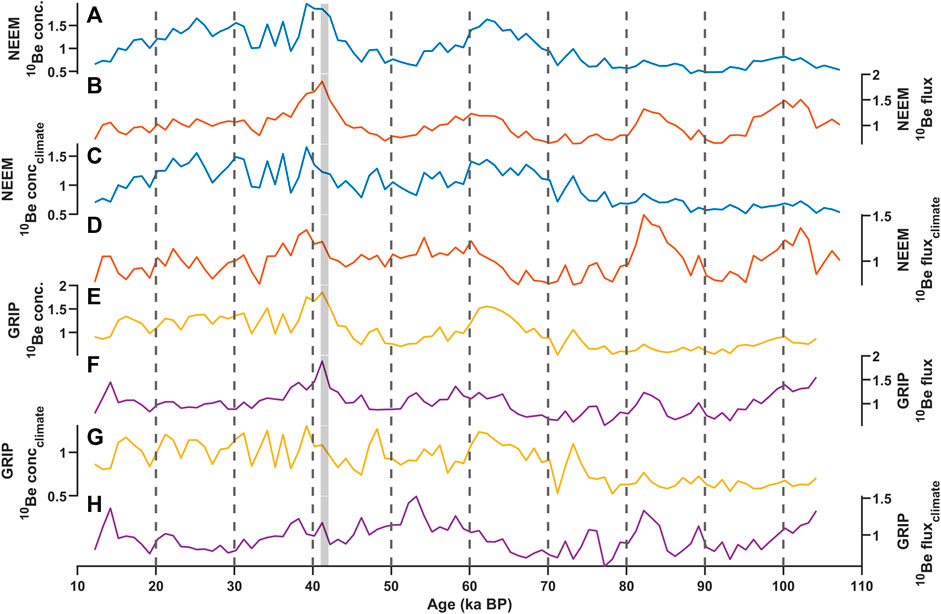
FIGURE 1. 10Be records of NEEM ice core showing concentrations (A), fluxes (B) and the corrected records for geomagnetic modulation referred to as 10Be concclimate(C) and 10Be fluxclimate(D) (E–H) the same for the record from the GRIP ice core. The grey bar shows the peak due to the Laschamps geomagnetic excursion during 41–42 ka BP. All data are resampled at a 1000-years resolution for the analysis (see text) and normalized for the overlap period.
Results and Discussions
The 10Be records of the NEEM and GRIP ice cores indicate similar concentrations and fluxes (Figure 1). The minimum and maximum values are 0.92 and 5.82 (x104 at/g ice) for the NEEM and 1.06 and 7.63 (x104 at/g ice) for the GRIP data with average values of 2.29 × 104 and 3 × 104 at/g ice, respectively (Table 1). The general profiles of the 10Be concentration and flux data are also comparable with the clear occurrence of the highest concentrations and fluxes zone around 40–42 ka representing the well-known Laschamps geomagnetic excursion. Another zone of high concentration occurs around 60–65 ka in both ice core records. The variability in δ18O of the two ice cores is similar to accumulation rates without unusual changes along with the Laschamps geomagnetic excursion (Supplementary Figure S4). The relatively strong and significant correlation (R = 0.91, p < 0.01, Supplementary Figure S5) between the 10Be concclimate of the NEEM and GRIP records and the well-defined occurrence of the Laschamps geomagnetic excursion (40–42 ka; Figure 1) in both records suggest regional patterns for 10Be deposition suitable for production and climate interpretations. However, there are some minor differences in the profiles of the production corrected records (10Be concclimate and 10Be fluxclimate) of the NEEM and GRIP ice cores (Figure 1). Those differences can be attributed to the uncertainties in 10Be measurements, different temporal resolution and ice core timescales, and most likely to different local climate influences at the two sites.

TABLE 1. Summary data for the raw data ranges of10Be concentration and flux and snow accumulation rate in the NEEM and GRIP ice cores.
Despite the differences in the two ice core records, a clear wave-like pattern is apparent in the 10Be fluxclimate profiles, which is comparable to the variations in the solar insolation (Figure 2). The correlation between the 10Be fluxclimate and insolation for the NEEM and GRIP records are R = 0.57 and 0.58, respectively (Supplementary Figure S5). In addition, there is a marked periodicity of 21 ka ± 3 ka in the 10Be fluxclimate of both ice cores (Supplementary Figure S6). Indication of the 21 ka periodicity also occurs in the δ18O data of both ice cores, but the 41–44 ka periodicity is more significant Supplementary Figure S6). Although one may argue that the 10Be climatic signal is an inherited snow accumulation signal, this effect is not extractable by the raw 10Be data without correction for the geomagnetic effect. In addition, there is a large difference in the accumulation rate between the NEEM and GRIP ice cores (Supplementary Figure S4), which is not manifested by the 10Be concentration records (Supplementary Figure S3).
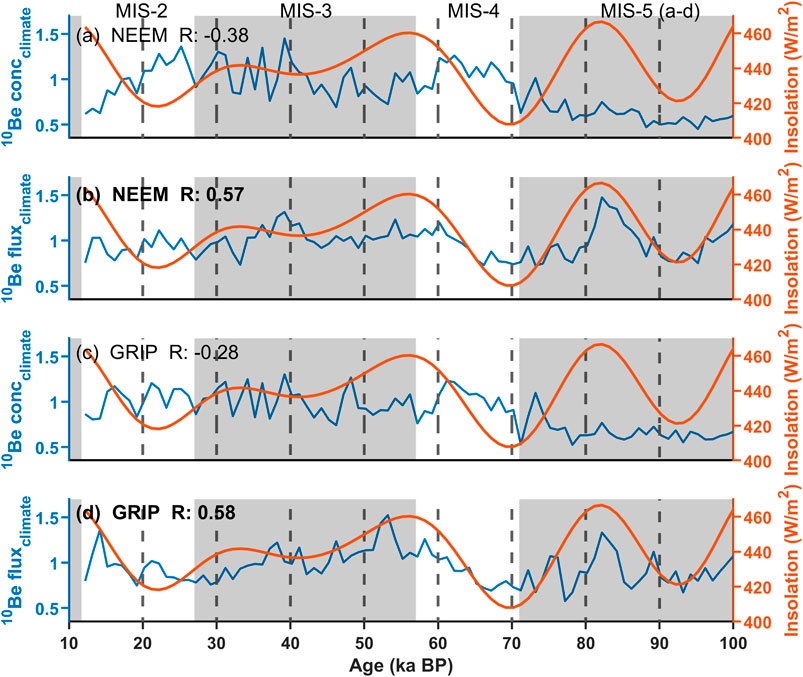
FIGURE 2. Comparison of the geomagnetic corrected 10Be records (10Be concclimate and 10Be fluxclimate) of NEEM and GRIP ice cores and summer insolation at 65°N over the last glacial period. The R-value is the Pearson correlation between 10Be and insolation. The R in bold indicates the significant value (p < 0.01, student t-test adjusted by the autocorrelation following (Hu et al., 2017)).
The cyclic pattern in the 10Be fluxclimate of both ice cores is paralleled with relatively good correlation values (R = −0.37 to −0.45) with the precession cycle (Supplementary Figure S5). The results from the Fast Fourier Transform (FFT) (Supplementary Figure S6) and wavelet transform (Supplementary Figure S7) further confirm a cyclicity around 20–22 ka (will be referred to as the 21 ka cycle) in both the NEEM and GRIP 10Be fluxclimate records that support the reflection of the orbital precession cycle.
It is well known that most atmospheric 10Be attaches to aerosols and would likely follow their transport pathways. To shed some light on this issue, we analyze SO42- and
To further discuss the climate signal in the 10Be data, we create a composite record by averaging the NEEM and GRIP 10Be fluxclimate (Figure 3). This stack record indicates a change around the midpart of MIS-4 (around 65 ka), which is also associated with an amplitude swing in the insolation record. Results of running correlation analysis between 10Be and δ18O and between 10Be and SO42- and
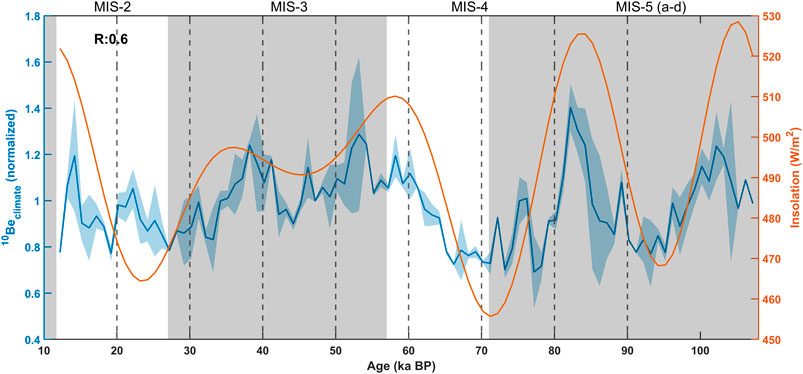
FIGURE 3. Stack 10Be record based on NEEM and GRIP 10Be fluxclimate records showing variability comparable to the summer insolation (TOA) at 65°N at a periodicity of 21°ka. The shaded area represents a 2-standard deviation error. The R in bold indicates the significant value (p < 0.01, student t-test adjusted by the autocorrelation following (Hu et al., 2017)).
Conclusion
10Be variabilities in the NEEM and GRIP ice cores from the last glacial period were analyzed to explore the link between 10Be transport and deposition to orbital forcing. After removing the effects of the geomagnetic field and solar modulation from the 10Be record, the results indicate imprints of the 21 ka precession cycles in the 10Be records. This finding might help to improve our understanding of the aerosol transport to the Greenland ice sheet during the last glacial.
Data Availability Statement
The original contributions presented in the study are included in the article/Supplementary Material, further inquiries can be directed to the corresponding authors.
Author Contributions
AS wrote the first manuscript in correspondence with RM, MZ and AA. AS and MZ performed the analysis. RM and AA initiated the study. All authors discussed and edited the manuscript.
Funding
The authors acknowledge funding from the Swedish Research Council and the Tandem Laboratory at Uppsala University. AA acknowledges the UAEU for the UPAR funding and the funding from the UAEU through the National Water and Energy Center project #31R192. MZ is supported by the strategic research program of ModEling the Regional and Global Earth system (MERGE) hosted by the Faculty of Science at Lund University and the Royal Physiographic Society of Lund (2017, 2018 and 2019, grants to MZ).
Conflict of Interest
The authors declare that the research was conducted in the absence of any commercial or financial relationships that could be construed as a potential conflict of interest.
Publisher’s Note
All claims expressed in this article are solely those of the authors and do not necessarily represent those of their affiliated organizations, or those of the publisher, the editors and the reviewers. Any product that may be evaluated in this article, or claim that may be made by its manufacturer, is not guaranteed or endorsed by the publisher.
Acknowledgments
We thank the many persons involved in logistics, drilling, and ice-core processing and analysis. NEEM is directed and organized by the Centre of Ice and Climate at the Niels Bohr Institute and US NSF, Office of Polar Programs. It is supported by funding agencies and institutions in Belgium (FNRS-CFB and FWO), Canada (NRCan/GSC), China (CAS), Denmark (FIST), France (IPEV, CNRS/INSU, CEA and ANR), Germany (AWI), Iceland (RannIs), Japan (NIPR), South Korea (KOPRI), The Netherlands (NWO/ALW), Sweden (VR), Switzerland (SNF), the United Kingdom (NERC) and the United States (US NSF, Office of Polar Programs) and the EU Seventh Framework programs Past4Future and Water under the Ice.
Supplementary Material
The Supplementary Material for this article can be found online at: https://www.frontiersin.org/articles/10.3389/feart.2021.743640/full#supplementary-material.
References
Adolphi, F., Muscheler, R., Svensson, A., Aldahan, A., Possnert, G., Beer, J., et al. (2014). Persistent Link between Solar Activity and Greenland Climate during the Last Glacial Maximum. Nat. Geosci 7, 662–666. doi:10.1038/ngeo2225
Aldahan, A., and Possnert, G. (1998). A High-Resolution 10Be Profile from Deep Sea Sediment Covering the Last 70ka: Indication for Globally Synchronized Environmental Events. Quat. Sci. Rev. 17, 1023–1032. doi:10.1016/s0277-3791(97)00089-9
Alley, R. B. (2000). Ice-core Evidence of Abrupt Climate Changes. Proc. Natl. Acad. Sci. 97, 1331–1334. doi:10.1073/pnas.97.4.1331
Barker, S., Knorr, G., Edwards, R. L., Parrenin, F., Putnam, A. E., Skinner, L. C., et al. (2011). 800,000 Years of Abrupt Climate Variability. Science 334, 347–351. doi:10.1126/science.1203580
Baumgartner, S., Beer, J., Wagner, G., Kubik, P., Suter, M., Raisbeck, G. M., et al. (1997). 10Be and Dust. Nucl. Instr. Methods Phys. Res. Section B: Beam Interactions Mater. Atoms 123, 296–301. doi:10.1016/s0168-583x(96)00751-3
Beck, J. W., Zhou, W., Li, C., Wu, Z., White, L., Xian, F., et al. (2018). A 550,000-year Record of East Asian Monsoon Rainfall from 10Be in Loess. Science 360, 877–881. doi:10.1126/science.aam5825
Berger, A., and Loutre, M. F. (1991). Insolation Values for the Climate of the Last 10 Million Years. Quat. Sci. Rev. 10, 297–317. doi:10.1016/0277-3791(91)90033-q
Berger, A. (1988). Milankovitch Theory and Climate. Rev. Geophys. 26, 624–657. doi:10.1029/rg026i004p00624
Berggren, A. M., Beer, J., Possnert, G., Aldahan, A., Kubik, P., Christl, M., et al. (2009). A 600-year Annual 10Be Record from the NGRIP Ice Core, Greenland. Geophys. Res. Lett. 36, L11801. doi:10.1029/2009gl038004
Bonhommet, N., and Za¨hringer, J. (1969). Paleomagnetism and Potassium Argon Age Determinations of the Laschamp Geomagnetic Polarity Event. Earth Planet. Sci. Lett. 6, 43–46. doi:10.1016/0012-821x(69)90159-9
Channell, J. E. T., Xuan, C., and Hodell, D. A. (2009). Stacking Paleointensity and Oxygen Isotope Data for the Last 1.5 Myr (PISO-1500). Earth Planet. Sci. Lett. 283, 14–23. doi:10.1016/j.epsl.2009.03.012
Herbst, K., Muscheler, R., and Heber, B. (2017). The New Local Interstellar Spectra and Their Influence on the Production Rates of the Cosmogenic Radionuclides 10Be and 14C. J. Geophys. Res. Space Phys. 122, 23–34. doi:10.1002/2016ja023207
Hu, J., Emile-Geay, J., and Partin, J. (2017). Correlation-based Interpretations of Paleoclimate Data - where Statistics Meet Past Climates. Earth Planet. Sci. Lett. 459, 362–371. doi:10.1016/j.epsl.2016.11.048
Igarashi, Y., Hirose, K., and Otsuji-Hatori, M. (1998). Beryllium-7 Deposition and its Relation to Sulfate Deposition. J. Atmos. Chem. 29, 217–231. doi:10.1023/a:1005921113496
Johnsen, S. J., Clausen, H. B., Dansgaard, W., Gundestrup, N. S., Hammer, C. U., Andersen, U., et al. (1997). The δ18O Record along the Greenland Ice Core Project Deep Ice Core and the Problem of Possible Eemian Climatic Instability. J. Geophys. Res. 102, 26397–26410. doi:10.1029/97jc00167
Lal, D., and Peters, B. (1967). “Cosmic Ray Produced Radioactivity on the Earth,” in Kosmische Strahlung II/Cosmic Rays II. Editor K. Sitte (Berlin, Heidelberg: Springer Berlin Heidelberg), 551–612. doi:10.1007/978-3-642-46079-1_7
Mayewski, P. A., Lyons, W. B., Spencer, M. J., Twickler, M. S., Buck, C. F., and Whitlow, S. (1990). An Ice-Core Record of Atmospheric Response to Anthropogenic Sulphate and Nitrate. Nature 346, 554–556. doi:10.1038/346554a0
Mayewski, P. A., Meeker, L. D., Twickler, M. S., Whitlow, S., Yang, Q., Lyons, W. B., et al. (1997). Major Features and Forcing of High-Latitude Northern Hemisphere Atmospheric Circulation Using a 110,000-Year-Long Glaciochemical Series. J. Geophys. Res. 102, 26345–26366. doi:10.1029/96jc03365
Muscheler, R., Beer, J., Kubik, P. W., and Synal, H.-A. (2005a). Geomagnetic Field Intensity during the Last 60,000 Years Based on 10Be and 36Cl from the Summit Ice Cores and 14C. Quat. Sci. Rev. 24, 1849–1860. doi:10.1016/j.quascirev.2005.01.012
Muscheler, R., Beer, J., Wagner, G., Laj, C., Kissel, C., Raisbeck, G. M., et al. (2004). Changes in the Carbon Cycle during the Last Deglaciation as Indicated by the Comparison of 10Be and 14C Records. Earth Planet. Sci. Lett. 219, 325–340. doi:10.1016/s0012-821x(03)00722-2
Muscheler, R., Joos, F., Beer, J., Müller, S. A., Vonmoos, M., and Snowball, I. (2007). Solar Activity during the Last 1000yr Inferred from Radionuclide Records. Quat. Sci. Rev. 26, 82–97. doi:10.1016/j.quascirev.2006.07.012
Muscheler, R., Joos, F., Müller, S. A., and Snowball, I. (2005b). How Unusual Is Today's Solar Activity? Nature 436, E3–E4. doi:10.1038/nature04045
NEEM community members (2013). Eemian Interglacial Reconstructed from a Greenland Folded Ice Core. Nature 493, 489–494. doi:10.1038/nature11789
Panovska, S., Korte, M., and Constable, C. G. (2019). One Hundred Thousand Years of Geomagnetic Field Evolution. Rev. Geophys. 57, 1289–1337. doi:10.1029/2019rg000656
Poluianov, S. V., Kovaltsov, G. A., Mishev, A. L., and Usoskin, I. G. (2016). Production of Cosmogenic isotopes 7Be, 10Be, 14C, 22Na, and 36Cl in the Atmosphere: Altitudinal Profiles of Yield Functions. J. Geophys. Res. Atmos. 121, 8125–8136. doi:10.1002/2016jd025034
Raisbeck, G. M., Yiou, F., Bourles, D., Lorius, C., Jouzel, J., and Barkov, N. I. (1987). Evidence for Two Intervals of Enhanced 10Be Deposition in Antarctic Ice during the Last Glacial Period. Nature 326, 273–277. doi:10.1038/326273a0
Rasmussen, S. O., Abbott, P. M., Blunier, T., Bourne, A. J., Brook, E., Buchardt, S. L., et al. (2013). A First Chronology for the North Greenland Eemian Ice Drilling (NEEM) Ice Core. Clim. Past 9, 2713–2730. doi:10.5194/cp-9-2713-2013
Rasmussen, S. O., Bigler, M., Blockley, S. P., Blunier, T., Buchardt, S. L., Clausen, H. B., et al. (2014). A Stratigraphic Framework for Abrupt Climatic Changes during the Last Glacial Period Based on Three Synchronized Greenland Ice-Core Records: Refining and Extending the INTIMATE Event Stratigraphy. Quat. Sci. Rev. 106, 14–28. doi:10.1016/j.quascirev.2014.09.007
Savranskaia, T., Egli, R., Valet, J.-P., Bassinot, F., Meynadier, L., Bourlès, D. L., et al. (2021). Disentangling Magnetic and Environmental Signatures of Sedimentary 10Be/9Be Records. Quat. Sci. Rev. 257, 106809. doi:10.1016/j.quascirev.2021.106809
Schüpbach, S., Fischer, H., Bigler, M., Erhardt, T., Gfeller, G., Leuenberger, D., et al. (2018). Greenland Records of Aerosol Source and Atmospheric Lifetime Changes from the Eemian to the Holocene. Nat. Commun. 9, 1476. doi:10.1038/s41467-018-03924-3
Seierstad, I. K., Abbott, P. M., Bigler, M., Blunier, T., Bourne, A. J., Brook, E., et al. (2014). Consistently Dated Records from the Greenland GRIP, GISP2 and NGRIP Ice Cores for the Past 104 Ka Reveal Regional Millennial-Scale δ18O Gradients with Possible Heinrich Event Imprint. Quat. Sci. Rev. 106, 29–46. doi:10.1016/j.quascirev.2014.10.032
Simon, Q., Thouveny, N., Bourlès, D. L., Valet, J. P., Bassinot, F., Ménabréaz, L., et al. (2016). Authigenic 10 Be/9 Be Ratio Signatures of the Cosmogenic Nuclide Production Linked to Geomagnetic Dipole Moment Variation since the Brunhes/Matuyama Boundary. J. Geophys. Res. Solid Earth 121, 7716–7741. doi:10.1002/2016jb013335
Taylor, K. C., Mayewski, P. A., Twickler, M. S., and Whitlow, S. I. (1996). Biomass Burning Recorded in the GISP2 Ice Core: a Record from Eastern Canada? The Holocene 6, 1–6. doi:10.1177/095968369600600101
Vonmoos, M., Beer, J., and Muscheler, R. (2006). Large Variations in Holocene Solar Activity: Constraints from 10Be in the Greenland Ice Core Project Ice Core. J. Geophys. Research-Space Phys. 111. doi:10.1029/2005ja011500
Wagner, G., Beer, J., Masarik, J., Muscheler, R., Kubik, P. W., Mende, W., et al. (2001). Presence of the Solar de Vries Cycle (∼205 years) during the Last Ice Age. Geophys. Res. Lett. 28, 303–306. doi:10.1029/2000gl006116
Yang, Q., Mayewski, P. A., Whitlow, S., Twickler, M., Morrison, M., Talbot, R., et al. (1995). Global Perspective of Nitrate Flux in Ice Cores. J. Geophys. Res. 100, 5113–5121. doi:10.1029/94jd03115
Yiou, F., Raisbeck, G. M., Baumgartner, S., Beer, J., Hammer, C., Johnsen, S., et al. (1997). Beryllium 10 in the Greenland Ice Core Project Ice Core at Summit, Greenland. J. Geophys. Res. 102, 26783–26794. doi:10.1029/97jc01265
Zheng, M., Adolphi, F., Sjolte, J., Aldahan, A., Possnert, G., Wu, M., et al. (2021a). Solar Activity of the Past 100 Years Inferred from 10Be in Ice Cores – Implications for Long-Term Solar Activity Reconstructions. Geophys. Res. Lett. 48, e2020GL090896.
Keywords: orbital forcing, 10Be, atmospheric transport, aerosol, ice core, Greenland, glacial period
Citation: Sturevik-Storm A, Zheng M, Aldahan A, Possnert G and Muscheler R (2021) The Influence of Orbital Forcing on 10Be Deposition in Greenland Over the Glacial Period. Front. Earth Sci. 9:743640. doi: 10.3389/feart.2021.743640
Received: 27 July 2021; Accepted: 27 August 2021;
Published: 15 September 2021.
Edited by:
Monika Korte, German Research Centre for Geosciences, GermanyReviewed by:
Ramon Egli, Central Institution for Meteorology and Geodynamics (ZAMG), AustriaNicolas Thouveny, CEREGE UM 34 Aix-Marseille UNIVERSITE-CNRS-IRD, France
Copyright © 2021 Sturevik-Storm, Zheng, Aldahan, Possnert and Muscheler. This is an open-access article distributed under the terms of the Creative Commons Attribution License (CC BY). The use, distribution or reproduction in other forums is permitted, provided the original author(s) and the copyright owner(s) are credited and that the original publication in this journal is cited, in accordance with accepted academic practice. No use, distribution or reproduction is permitted which does not comply with these terms.
*Correspondence: Minjie Zheng, bWluamllLnpoZW5nQGdlb2wubHUuc2U=; Ala Aldahan, YWFsZGFoYW5AdWFldS5hYy5hZQ==