- 1State Key Laboratory of Shale Oil and Gas Enrichment Mechanisms and Effective Development, Beijing, China
- 2Petroleum Exploration and Production Research Institute, SINOPEC, Beijing, China
- 3Institute of Energy, Peking University, Beijing, China
The lacustrine shale in the Dongyuemiao Member of the Fuling area, Sichuan Basin, is widely distributed and has huge shale oil resource potential. It is one of the important replacement areas for shale oil exploration in China. To investigate the key shale oil evaluation well, Well FY10, in the Fuling area, X-ray diffraction (XRD) mineral analysis, Rock-Eval, argon ion polishing-scanning electron microscope (SEM), Mercury injection capillary pressure (MICP), and low pressure nitrogen adsorption were launched to determine the heterogeneity of the pore system in the lacustrine shale of the Dongyuemiao Member. The mineral composition exhibits a high degree of heterogeneity, and the shale can be divided into two main lithofacies: argillaceous shale and mixed shale. The porosity ranges from 2.95 to 8.43%, and the permeability ranges from 0.05 to 1.07 × 10−3 μm2. The physical properties of mixed shale are obviously better than those of argillaceous shale. Inorganic mineral pores, such as linear pores between clay minerals and calcite dissolution pores, are mainly developed, while a small amount of organic pores can be observed. The average total pore volume (Vp) is 0.038 ml/g with an average specific surface area of 5.38 m2/g. Mesopores provide the main Vp (average 61.72%), and micropores provide mostly specific surface area. TOC imposes a strong controlling effect on the development of micropores. Clay minerals are the main contributors to mesopores and macropores. The organic-inorganic interaction during the process of diagenesis and hydrocarbon generation controls the formation of shale pore systems.
Introduction
With the commercial exploitation of shale oil in the Bakken Formation in the Williston Basin, the Eagle Ford Formation in Southern Texas, and the Barnett Formation in the Fort Worth Basin, shale oil has altered the American energy structure and imposes a profound impact on the world energy pattern (Hill et al., 2007; Zhang et al., 2017; Saputra et al., 2019). In recent years, with the rapid development of China’s economy, the demand for oil and gas has increased substantially, and the degree of dependence on foreign oil and natural gas has continued to increase. There is an urgent requirement to find new types of oil and gas resources, achieve theoretical and technological breakthroughs, and form a contribution to domestic oil and gas production (Jia et al., 2012; Zou et al., 2020). Among the known new resource types, shale oil is one of the most realistic exploration and development targets (Zou et al., 2013; Jin et al., 2019). Shale oil refers to the oil contained in the organic-rich shale strata (including the thin interbedded tight carbonate and clastic rock layers in the shale). There is no production capacity under natural conditions, and unconventional technology is required to realize economies of scale exploitation (Jin et al., 2019).
Organic rich shale is widely distributed in China, which not only provides abundant conventional oil and gas resources, but also contains plentiful shale oil and gas resources (Jin et al., 2019; Zou et al., 2019). Many breakthroughs have been achieved in the Permian Lucaogou Formation in the Junggar Basin, Triassic Yanchang Formation in the Ordos Basin, Cretaceous Qingshankou Formation in the Songliao Basin, middle-lower Jurassic formations in the Sichuan Basin, and Paleogene Shahejie Formation in the Bohai Bay Basin, and industrial production has been realized in some regions (Zhang et al., 2018; Liu et al., 2019; Song et al., 2020; Fu et al., 2021; Liu et al., 2021; Zhang et al., 2021).
Lacustrine shale is characterized by very developed strata, wide distribution, and massive exploration potential (Jia et al., 2012; Jin et al., 2019; Zou et al., 2019; Liu et al., 2021). However, there are still some unresolved problems such as strong heterogeneity, an unclear occurrence mechanism, and the main controlling factors of enrichment (Guo et al., 2016; Li et al., 2020; Song et al., 2020). Based on the comprehensive study of the geological conditions of Dongyuemiao shale of Jurassic Ziliujing Formation in Sichuan Basin, the heterogeneity of lacustrine shale under lithofacies constraints in Fuling area is studied, in order to provide support for promoting the exploration and development process of lacustrine shale oil and gas in the Sichuan Basin and enrich the geological theory of lacustrine shale oil and gas.
Geological Setting
The Jurassic formation in the Sichuan Basin is a set of important petroleum-bearing strata containing both oil and gas (Liu et al., 2019). During the early and middle Jurassic, most of the Sichuan Basin was composed of shore shallow lacustrine and semi-deep lacustrine sediments (Li and He, 2014). Vertically, three sets of organic rich shales are developed in the Dongyuemiao Member, Da’anzhai Member of Ziliujing formation, and Lianggaoshan Formation (Zheng et al., 2013). During the deposition period of Dongyuemiao Member, the sedimentary center of Sichuan Basin is mainly located around Liangping-Guang’an-Fuling area in the east of Sichuan Province. The black shale exhibits a relatively high abundance of organic matter and a wide distribution area (Shu et al., 2021).
The Fuling area is located in the eastern of the Sichuan Basin (Figure 1). During the Dongyuemiao sedimentary period, the Fuling area experienced a large-scale lake transgression, depositing a set of shore shallow lacustrine to semi-deep lacustrine black shale interbedded with thin shell limestone, with regional thickness of 60–70 m. The Dongyuemiao Member in the area can be further divided into three sub members: the first sub member is black shale interbedded with thin layers of shell limestone, with a thickness of 28 m. The second sub member is composed of black argillaceous limestone with a thickness of 6.5 m. The third sub member is dark mudstone with a thickness of 27 m. In general, the lacustrine water body of the Dongyuemiao Member in the study area has experienced a change process from shallow to deep to shallow.
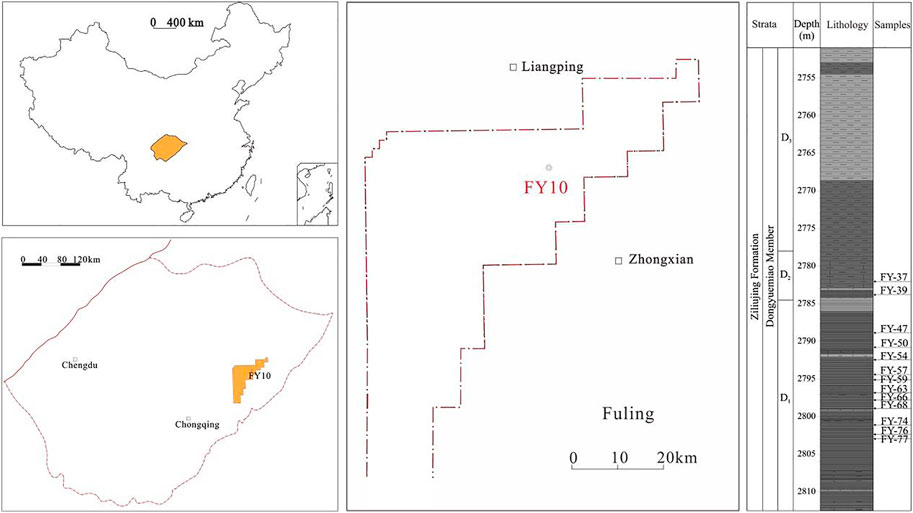
FIGURE 1. Geologic map and stratigraphic column of the Dongyuemiao shale and the sampling sites in the Fuling area in the eastern Sichuan Basin, China.
In March 2020, the FY10 well deployed by Sinopec Jianghan Oilfield Company was successfully fractured and became the first production well of shale oil and gas in the area (Figure 1). It obtained a daily gas production of 5.58 × 104 m3 and oil production of 17.6 m3. The total organic carbon (TOC) content of the favorable interval of Well FY10 is 2.11%, the porosity is 6.11%, and the permeability is 0.127 × 10−3 μm2, showing good reservoir performance in general. The Ro of the shale in Dongyuemiao Member is between 1.23 and 2.09%. The organic matter has evolved to a high mature stage, that is, the condensate to wet gas stage (Shu et al., 2021).
Samples and Methods
A total of 13 shale samples were carefully selected from the shale strata in the Dongyuemiao Member of Well FY10. Each sample is divided into several parts for different analyses.
Experimental Method
The analyses in this study were all launched at the Wuxi Research Institute of Sinopec Petroleum Exploration and Development Research Institute. First, 13 samples were analyzed for TOC content, rock pyrolysis, and XRD mineral composition. The analyses process comply with the operating steps outlined in the National Standard (SY/T) 14506.28-2010 and Chinese Oil and Gas Industry Standard (GB/T) 19145-2003. Nine of the samples were selected for thin slice identification analysis. Meanwhile, eight shale samples were launched for argon ion polishing-scanning electron microscopy analysis to observe the development characteristics of pores.
To quantitatively characterize the full-scale pore size distribution characteristics of shale, eight samples were selected for Mercury injection capillary pressure (MICP) and low pressure nitrogen adsorption, under the experimental procedure proposed in the National Standard (GB/T) 21650.1-2008 and (GB/T) 19587-2017 (Li et al., 2016, 2017). Theoretically speaking, the measurement range of the nitrogen adsorption method is from 0.35 to 500 nm. Due to the limitations of various formulas and models, the nitrogen adsorption method only has high measurement accuracy for pores below 50 nm, while the precision of the test results for large pores is reduced. The measurement range of the MICP method is from 6.5 nm to 63 μm. Because high pressure can damage the shale structure and then affect the results, the MICP has high measurement accuracy for pores above 50 nm (Li et al., 2017). In general, only by combining the two methods can it get the continuous pore distribution of shale. The method used in this paper is to combine the nitrogen adsorption data below 50 nm with the MICP data above 50 nm. In the meantime, the Brunauer Emmett Teller (BET) method was applied to calculate the specific surface area.
Lithofacy Classification Method
According to the quantitative analysis of the total mineral composition of lacustrine shale, the two-level lithofacies’ division is carried out on the basis of the graphic method of clay minerals, QMF (quartz, mica, and feldspar), and carbonate minerals as three terminal elements. In the first-level division process, the traditional petrological classification method is respected, and the name of vary rock is determined with 50% as the boundary. At the three ends of the triangle diagram, the shale area (I), sandstone area (III), and limestone area (IV) are determined, respectively. In the meantime, the middle area where the content of the three components does not exceed 50% is named the mixed rock area (II) (Figure 2A). On this basis for the I, III, and IV areas, taking the mineral content of 25% as the boundary, the secondary division is carried out. For example, the shale area (I) is further divided into three secondary areas: argillaceous shale, silty shale, and carbonate shale. For the mixed rock area, it is named mixed shale (Figure 2B).
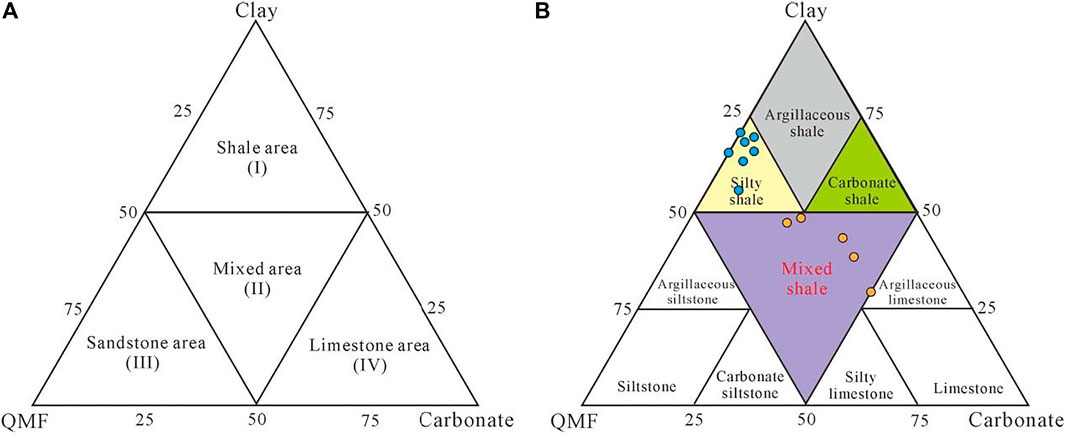
FIGURE 2. Lithofacies of the Dongyuemiao shale in the Fuling area according to the ternary diagram; QMF: quartz, mica, and feldspar. (A) first-level division of lithofacies; (B) secondary division of lithofacies.
On the basis of the above-mentioned three-terminal division, it is also necessary to identify the structure (including grain structure and morphology), genesis, and related sedimentary characteristics of the two non-clay minerals, quartz and calcite in shale, to improve the division of lithofacies.
Results
Mineral Contents
The detailed results of XRD mineral analysis are shown in Table 1. The mineral composition of the shale in the Dongyuemiao Member is highly heterogeneous, mainly including clay minerals, quartz, calcite, and a small amount of feldspar, dolomite, and pyrite. The content of clay minerals ranges from 25.9 to 69.0%, with an average value of 55.0%. The quartz content ranges from 17.8 to 29.4%, with an average value of 23.5%. The calcite content is between 0 and 51.2%, with an average value of 14.0% (Table 1). The three primary minerals accounted for 92.4% of the contents of the total minerals. Except for the relatively stable quartz content, the contents of clay minerals and calcite are both highly heterogeneous and fluctuate with each other.
Types of Lithofacies
On account of the ternary diagram of the lithofacies classification, two lithofacies of the Dongyuemiao shale can be identified, namely, mixed shale and silty shale (Figure 2). But for quartz, thin-section observation shows that the grain size structure of quartz in the shale is different from that of normal sandstone. Taking the two samples of the same silty shale (FY-74 and FY-77) as an example, the clay mineral contents are greater than 50%, and the quartz contents are 21 and 25.5%, respectively, which are all argillaceous structures, and it is difficult to identify obvious quartz particles (particle size <0.039 mm) (Figures 3A,B). Obviously, it is inappropriate to define it as silty shale, and it is more suitable to identify it as argillaceous shale. For the calcite mineral in mixed shale, core observation and thin-section identification analysis exhibit that it is mainly the product of calcification and cementation of the original biological shell, which is caused by bio-chemical sedimentation. Under the microscope, two-stage calcite cementation can be seen in individual shells (Figure 3C), and there exist different degrees of recrystallization (Figure 3D), but in most cases the original biological structure is still better.
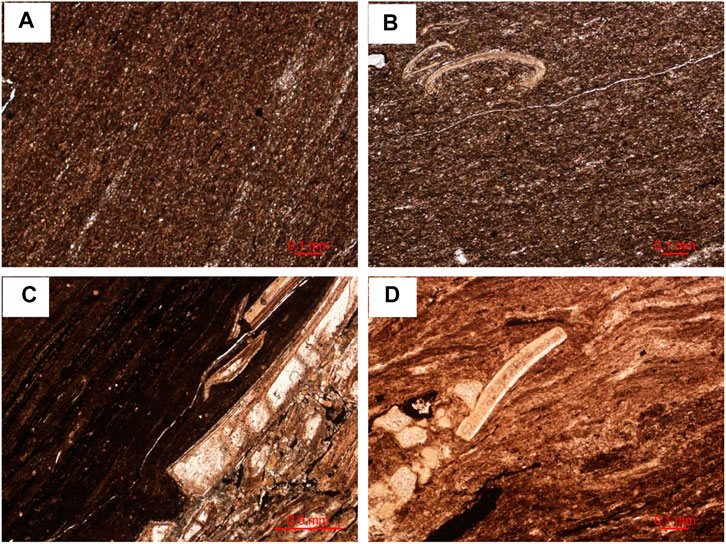
FIGURE 3. Microscopic Features of Main Lithofacies in Dongyuemiao shale of Well FY10 (A) argillaceous shale, 2,801.11 m; (B) argillaceous shale, 2,802.97 m; (C) mixed shale, 2,790.79 m; (D) mixed shale, 2,792.46 m.
By determining the structure and genesis of minerals, the definition of certain rocks can be effectively corrected, and the lithofacies of the same interval in the triangle diagram of mineral quantitative analysis can be distinguished. According to the results of XRD mineral analysis and thin slice observation, the Dongyuemiao shale of Well FY10 mainly develops argillaceous shale and mixed shale. The vertical lithofacies change and the water depth fluctuation have a certain synergism.
Organic Geochemical and Physical Characteristics
Total organic carbon (TOC) is usually the main indicator for evaluating the abundance of source rocks (Li et al., 2016; Liu et al., 2019; Feng et al., 2020). The TOC content of the shale in the Dongyuemiao shale of Well FY10 ranges from 0.77 to 3.13%, with an average value of 1.67% (Table 2). Rock pyrolysis hydrocarbons are composed of adsorbed hydrocarbons (S1) and pyrolysis hydrocarbons (S2). Their contents vary with the abundance and type of organic matter, and are direct indicators for evaluating the quality of source rocks (Huang et al., 2020). S1 ranges from 0.48 to 1.10 mg/g, with an average value of 0.70 mg/g. Hydrocarbon generation potential (PG = S1+S2) is an important indicator to characterize the abundance of organic matter (Huang et al., 2020). With the increase of maturity, the PG will obviously decrease. The PG of the Dongyuemiao shale of Well FY10 ranges from 1.16 to 3.08 mg/g, with an average value of 1.91 mg/g, which makes it a generally medium source rock. PG and TOC exhibit an obviously positive relationship, but there exist certain differences between argillaceous shale and mixed shale (Figure 4). The range of PG and TOC of the argillaceous shale is small, showing a certain degree of homogeneity. In contrast, the distribution of PG and TOC of the mixed shale is large and exhibits strong heterogeneity.
To express the oiliness of shale, Jarvie (2012); Jarvie (2014) proposed the Oil Saturation Index (OSI), that is, the ratio of the TOC of the shale to its pyrolysis S1 (S1/TOC). The OSI of shale in Dongyuemiao Member of Well FY10 ranges from 30.85 to 62.34 mg/gTOC, with an average value of 43.92 mg/gTOC, and there exist no significant differences between different types of shale. The analysis suggests that S1 reflects the remaining oil in the source rock, but is affected by sampling conditions, evolution stages, and oil and gas migration. The shale in the study area has evolved to a high maturity stage of the condensate to wet gas stage, so the light hydrocarbon components are easily lost, which has a certain impact on the results. The δ13C of kerogen ranges from −25.0‰ to −30.7‰, which indicates that type II kerogen is dominant in organic matter.
Through physical experiments, the porosity and permeability of the Dongyuemiao shale can be obtained. The porosity ranges from 2.95 to 8.43%, with an average value of 6.84%; the permeability ranges from 0.05 to 1.07 × 10−3 μm2, with an average value of 0.26 × 10−3 μm2 (Table 2). The physical properties of mixed shale are obviously better than those of argillaceous shale, but the relation between porosity and permeability of mixed shale is unclear, and the porosity and permeability of argillaceous shale exhibit an obvious positive correlation (Figure 5).
Pore Types and Full-Scale Pore Distribution
A combination of argon ion polishing-SEM, low-temperature nitrogen adsorption, and MICP was launched to carry out qualitative observation and quantitative analysis of shale micro-pores. To fully consider the contact and genetic relationship between inorganic mineral particles, organic matter, and pores, the pores of the Dongyuemiao shale in the study area are finally divided into organic pores and inorganic pores. Inorganic pores can be further divided into clay mineral pores and calcite-related pores.
Pore Morphology From Scanning Electron Microscope
Inorganic Pores
The development of inorganic pores, in essence, is similar to conventional reservoirs. There are two main formation mechanisms for the development of inorganic pores. One is the residual pores developed after the early intergranular pores undergo diagenetic compaction and cementation, and the other is the dissolution pores formed by the later dissolution modification (which can occur between and within grains); in addition, some pores also develop during mineral diagenetic alteration. The inorganic mineral pores in the shale of Dongyuemiao Member are mainly calcite dissolved pores and linear pores between clay minerals.
Compared with the marine Wufeng-Longmaxi shale (Dai et al., 2014; Feng et al., 2016; Nie et al., 2018), the Jurassic lacustrine shale has a higher content of clay minerals (>50%), and the average content of clay minerals in the Dongyuemiao shale of Well FY 10 can reach 55.0%. SEM observation reveals that the shale of Dongyuemiao Member widely develop linear micropores related to clay minerals (Figures 6A,B), that mainly exist between illite flakes or illite and mineral particles. Linear pores vary in length and width, and some of the pores are filled with solid asphalt. Therefore, the organic matter content in different samples has a significant effect on the development of linear pores. This type of pore is mainly composed of primary pores between early clay minerals. With the continuous increase of burial depth, due to the weak anti-compaction ability between clay minerals, the pores rapidly shrink to form residual linear pores. This type of pore basically develops along the cleavage direction of clay minerals, forming slit-shaped or linear pores, which can extend several microns.
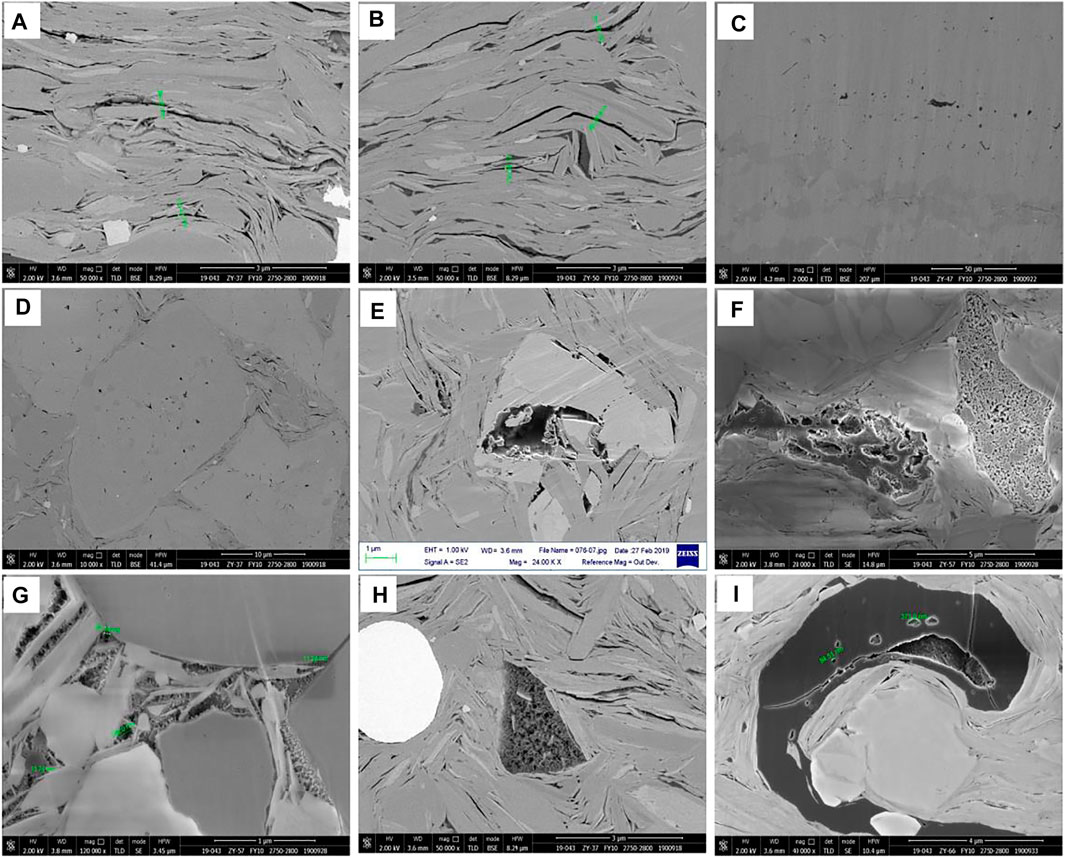
FIGURE 6. Pore morphology from SEM of Dongyuemiao shale (A) linear pores between clay minerals, 2,782.04 m; (B) linear pores between clay minerals, 2,790.79 m; (C) calcite dissolved pores, 2,788.87 m; (D) calcite dissolved pores, 2,782.04 m; (E) calcite dissolved pores, 2,802.37 m; (F) organic pores, 2,794.41 m; (G) Organic pores, 2,794.41 m; (H) organic pores, 2,782.04 m; (I) organic pores, 2,797.81 m.
Calcite dissolved pores are developed in the Dongyuemiao shale of Well FY10. Calcite is mainly composed of a crystalline granular structure, and the development of dissolution pores is various, mainly in three forms (Figures 6C–E). The first is the dissolution pores at the edge of calcite grains with irregular shapes. The second is the dissolution of macropores in the calcite grains, with various shapes, having clay minerals or dissolution residual calcite in the pores. The third is the dissolution needle-like pores in the calcite grains, and the individual is significantly smaller than the formers. The formation of calcite-related dissolved pores may be mainly related to the dissolution of organic acids during hydrocarbon generation.
Organic Pores
Observations of most shale samples from the Dongyuemiao Member reveal that the organic pores are well developed in the solid asphalt filled within inorganic minerals. The pore shapes vary, and include oval, irregular, slit, and honeycomb shapes (Figures 6F–H). The pore diameter is mainly in nanometers, but the size is not uniform, from tens of nanometers to hundreds of nanometers (the long axis of the pore size) are developed, and some samples show the development of micron-scale organic pores. The development of organic pores is mainly controlled by organic matter components. The organic pores in the solid asphalt are generally developed. For vitrinite, most of them did not develop organic pores except for a few which were modified by bacteria (Figure 6I).
Pore-Throat Structure Parameters and Pore Size Distribution
The entry pressure of the lacustrine shale is 0.46 MPa on average. The corresponding maximum pore-throat radius is 580.61 nm. The average median pressure reaches 20.88 MPa. The corresponding median radius is 8.09 nm. There exists no obvious difference in pore-throat structure parameters of different lithofacies shale.
The total pore volume (Vp) distribution range of Dongyuemiao shale is 0.0097–0.0163 cm3/g, the average value is 0.038 cm3/g, and the specific surface area ranges from 2.93 to 9.65 m2/g, with the average value of 5.38 m2/g (Table 3). According to the IUPAC pore classification standard, pores are classified into micropores (<2.0 nm), mesopores (2–50 nm), and macropores (>50 nm) according to their size (Loucks et al., 2012; Thommes et al., 2015). In general, the average proportions of micropores (<2 nm), mesopores (2–50 nm), and macropores (>50 nm) are 15.35, 61.72, and 22.93%, respectively. It can be seen that the proportion of mesopores is dominant and the proportion of macropores is also relatively high. The shale of the marine Wufeng-Longmaxi Formation is dominated by micropores and mesopores, the contents of which are similar, and together they can account for more than 95% of the total Vp (Li et al., 2016; Feng et al., 2018, 2019). In general, compared with the marine shale, the proportion of meso-macropores in the Dongyuemiao Member of the lacustrine shale is higher, which is very beneficial for improving the reservoir performance of shale.
From the full pore size distribution of shale, it can be detected that the distribution of different samples still has certain differences. The pore size distribution of the argillaceous shale is similar to that of the mixed shale. An obvious main peak of the pore size distribution exits and the main body is in the range of mesopores (2–20 nm) (Figures 7A,B). The pores of the two types of shale are mainly mesopores, which accounts for more than 60% on average. The proportion of micropores in mixed shale is slightly lower than that of macropores, with average values of 18.28 and 19.17%, respectively (Figure 7C; Table 3). The proportion of macropores in argillaceous shale is significantly higher than that of micropores, with average values of 25.18 and 13.60%, respectively (Figure 7D; Table 3). This difference in pore size distribution may be due to the different organic and inorganic mineral compositions of the two types of shale.
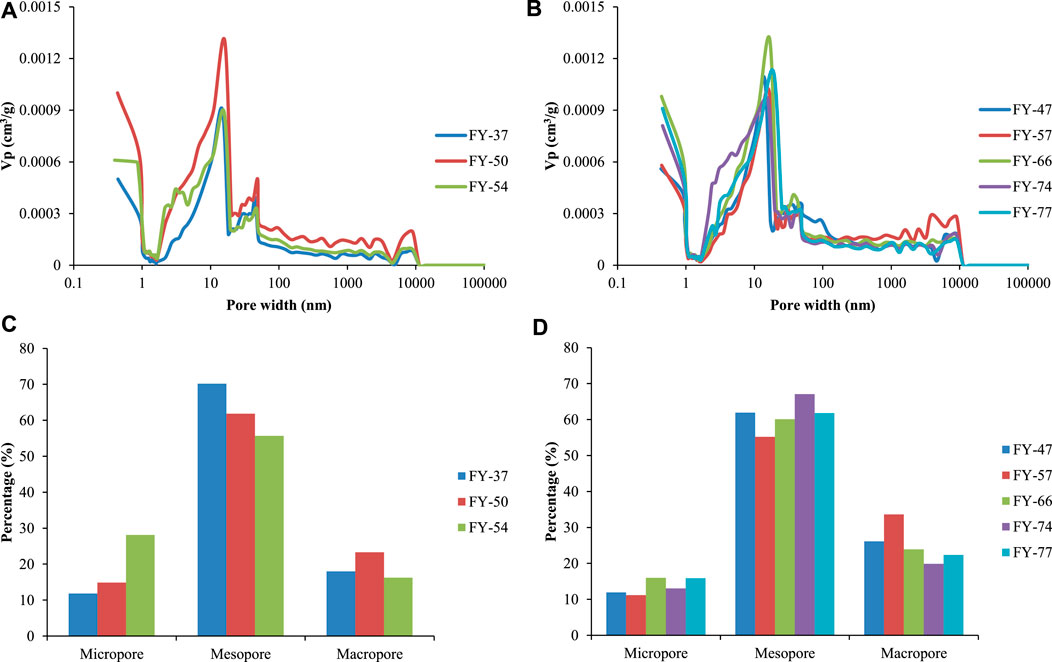
FIGURE 7. Pore size distribution characteristics of different lithofacies shale. (A) pore size distribution of mixed shale; (B) pore size distribution of argillaceous shale; (C) proportion characteristics of pores in different scales of mixed shale; (D) proportion characteristics of pores in different scales of argillaceous shale.
It can be detected from the intersection of pore volume and specific surface area that the total Vp and specific surface area have a certain positive correlation, but the correlation is not strong (Figure 8A). The relationship between the corresponding Vp of pores in different scales and specific surface areas is very different. The Vp of micropores and specific surface areas show a significant positive correlation, R2 can reach 0.9787 (Figure 8B), while Vp of mesopores and macropores have no obvious correlation with specific surface area (Figures 8C,D), indicating that micropores are the main contributor to the specific surface area, which is consistent with marine shale. Different types of shale also exhibit obvious differences. The Vp of micropores of mixed shale is only positively correlated with the specific surface area, while the Vp of micropores and mesopores of argillaceous shale exhibit an obvious positive correlation with specific surface area, indicating that the development of pores in argillaceous shale imposes a stronger controlling effect on surface area than mixed shale, which achieves more obvious regularity characteristics.
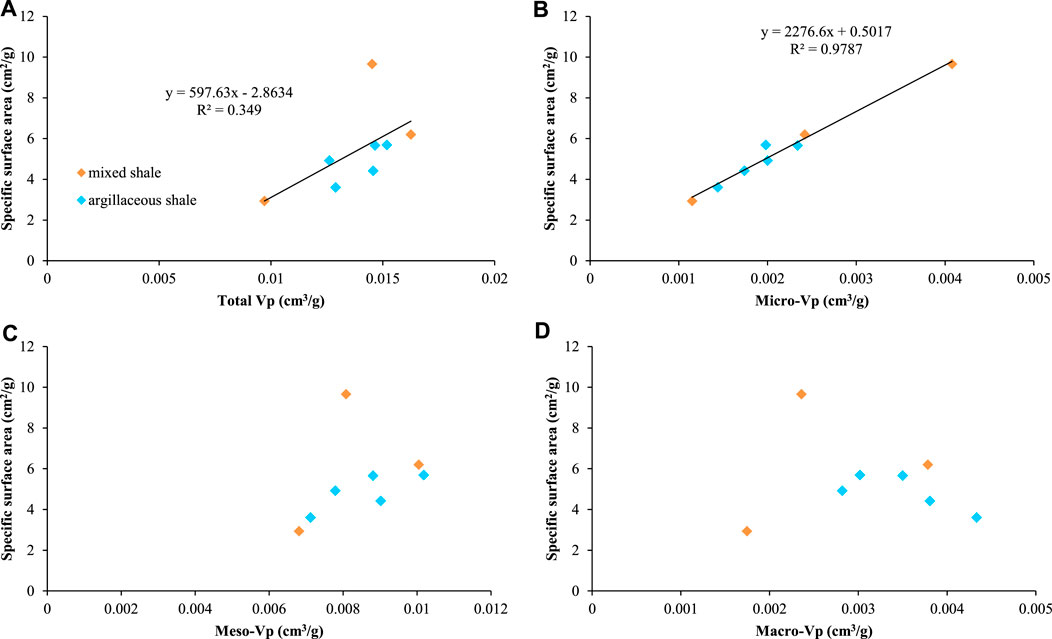
FIGURE 8. Intersection diagrams of pore volume and specific surface area. (A) total Vp and specific surface area; (B) micro-Vp and specific surface area; (C) meso-Vp and specific surface area; (D) macro-Vp and specific surface area
Discussion
To probe the differences between shale porosity and pore size distribution of different lithofacies, based on the material composition of shale, the reason for these differences is analyzed comprehensively from the composition of organic matter and inorganic mineral.
The Relationship Between Total Organic Carbon and Pore Structure Parameters
As an important part of the shale pore system, organic pores are formed during the process of hydrocarbon generation. The development of organic pores is of great significance to evaluate the gas storage capacity of shale intervals. Therefore, the formation mechanism of organic pores has always been a hot issue in the field of shale reservoirs. The development of organic pores in the Dongyuemiao shale is unique, which exhibits that some organic matter in the same sample is compact and uniform and develops no internal pores, while some organic matter has relatively developed internal pores. The organic matter with undeveloped pores is mostly in the shape of a strip or block. It is a microscopic component formed by the humification and gelation of the lignocellulosic tissue of higher plants (mainly vitrinite). The organic matter with organic pores is mostly unfixed, controlled by the original pore carrier (surrounding inorganic minerals), and is solid asphalt.
As shown in Figure 9, the Vp of micropores and specific surface areas are positively correlated with TOC content, indicating that micropores are mainly developed in organic matter. TOC content seems to be the main parameter controlling micropores’ development. However, there exists no obvious correlation between the Vp of mesopores and macropores and the TOC content, which indicates that the pores in organic matter are not the main contributors of mesopores and macropores.
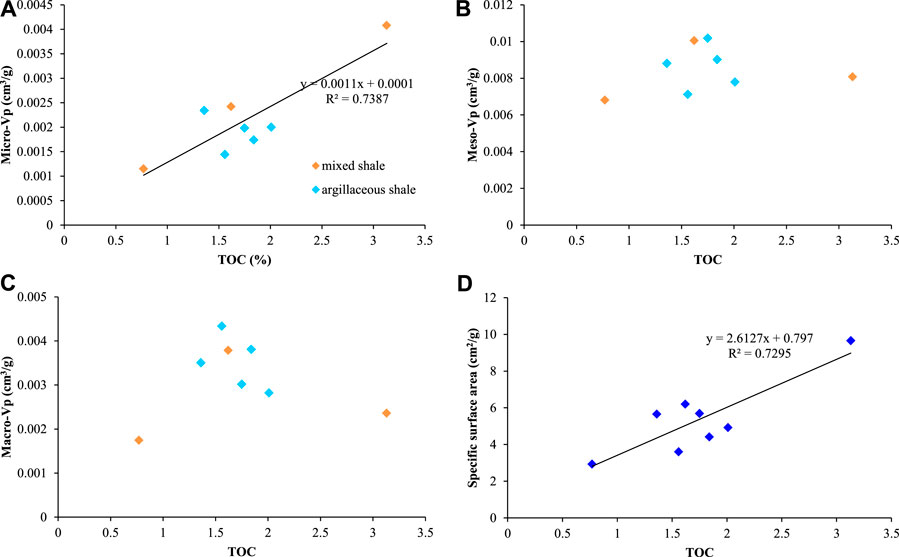
FIGURE 9. Intersection diagrams of TOC and pore structure parameters. (A) TOC and micro-Vp; (B) TOC and meso-Vp; (C) TOC and macro-Vp; (D) TOC and specific surface area.
In general, TOC content was positively correlated with micropore volume, indicating that organic matter could be used as the main index of micropore development. The higher the TOC content is, the more micropores and the larger the specific surface area in shale, which is consistent with the previous research results of marine shale (Loucks et al., 2012; Feng et al., 2019).
The Relationship Between Mineral Composition and Pore Structure Parameters
There are mainly two types of inorganic mineral pores in the Dongyuemiao shale of Well FY10, namely linear pores between clay minerals and calcite dissolved pores (Figure 6), which are closely related to the mineral composition of the shale. The average content of clay minerals in the Dongyuemiao shale is about 55%. The high clay content leads to weak compaction resistance, and the clay minerals are mostly oriented and distributed in layers, with residual clay minerals interlayers. The interlayer linear micropores of residual clay minerals are the main pores of lacustrine shale. Although the intergranular pores between clay minerals and mineral particles are developed, the number of pores is relatively small, and most of the large pores have been filled by solid asphalt. According to the statistics of the relationship between clay minerals and pore volume, it can be detected that with the increase of clay minerals, the Vp of mesopores and macropores tends to become larger, and the correlation coefficient with macropores is higher, showing a better relevance (Figure 10). It is shown that its contribution to micropores and mesopores is relatively apparent, and the linear pores between clay minerals provide the main reservoir space for the shale in the study area.
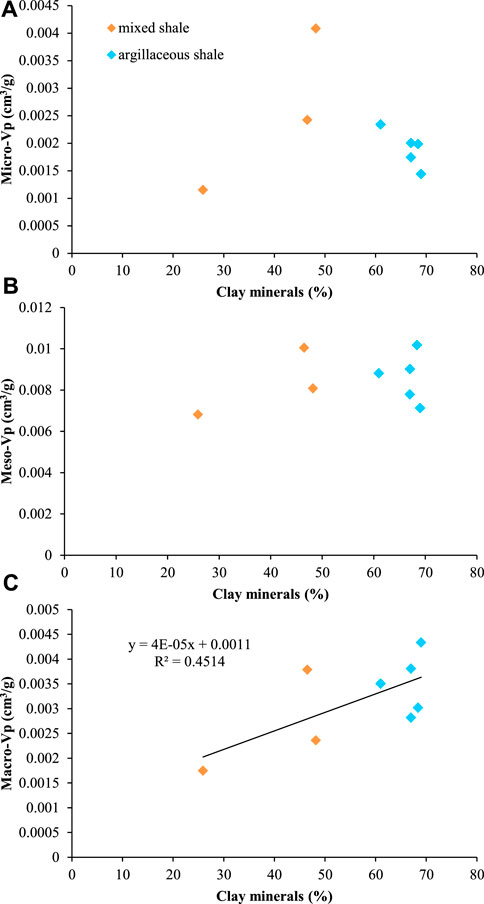
FIGURE 10. Intersection diagrams of clay minerals and pore structure parameters. (A) clay minerals and micro-Vp; (B) clay minerals and meso-Vp; (C) clay minerals and macro-Vp.
The calcite of Dongyuemiao Member is dominated by a crystalline granular structure with various dissolved pores, but there is no obvious regularity in its development. It is speculated that the formation of calcite-related dissolved pores mainly related to the organic acid dissolution during the hydrocarbon generation process. According to the statistics of the relationship between calcite and pore volume, it can be detected that, with the increase of calcite content, the Vp of mesopores and macropores tends to decrease, but there is no obvious correlation with the Vp of micropores. Although calcite may develop various types of pores, its contribution to pores in different sizes is relatively limited, so calcite dissolved pore is not the main contributor of reservoir space of lacustrine shale (Figure 11).
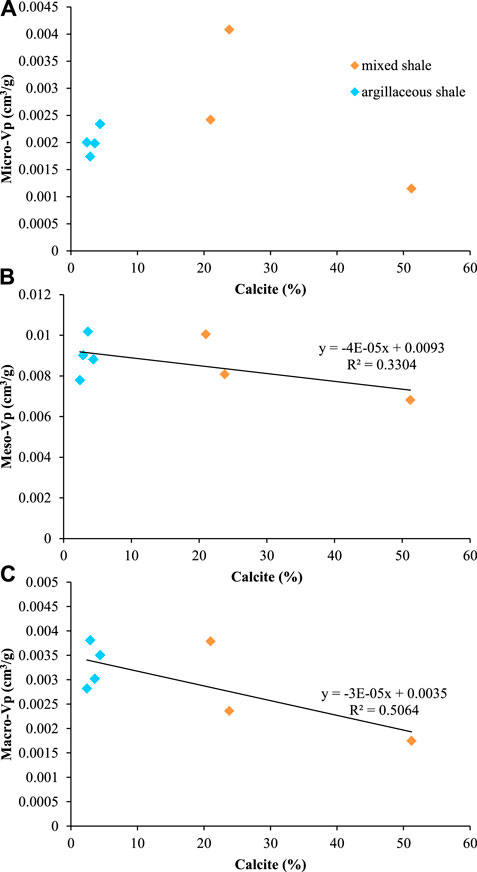
FIGURE 11. Intersection diagrams of calcite and pore structure parameters. (A) calcite and micro-Vp; (B) calcite and meso-Vp; (C) calcite and macro-Vp.
Diagenesis-Hydrocarbon Generation Controls the Formation and Evolution of Pores
The pore development and evolution of shale is complex, and is closely related to the compaction during the diagenesis process, the hydrocarbon generation and expulsion of organic matter, and the precipitation and dissolution of carbonate minerals. In addition to various inorganic minerals (clay minerals, quartz, feldspar, calcite, etc.), shale is also rich in organic matter, and organic pores that contribute to the accumulation of oil and gas are found inside. With the increase of the buried depth of the shale, the organic matter also evolves with the increase of the temperature in the process of the inorganic mineral diagenesis. Inorganic pores formed by the diagenetic evolution of inorganic minerals coexist with the pores formed by the hydrocarbon generation of organic matter, which reveals the formation and evolution of diagenesis, hydrocarbon generation, and pores as an integrated whole.
The shale in the Dongyuemiao Member of the Fuling area has entered the middle diagenetic stage B, with strong compaction; a large amount of montmorillonite was transformed into lamellar illite, the intergranular pores and intragranular pores have become smaller and the number decreased, but a large number of mesopores and macropores still formed, which are the main contributors to the shale reservoir space. The maturity (Ro) of shale is 1.5%, and it has entered a high evolutionary stage. Organic pores begin to form during the secondary pyrolysis of petroleum. Observation under the SEM exhibits that organic pores, mainly micropores, are widely developed in the asphalt. To some extent, it increases the porosity and contributes to the total porosity. Therefore, the difference in the reservoir space of different lithofacies is only a characteristic phenomenon. The organic-inorganic interaction controls the formation of shale pore systems in different lithofacies during diagenesis-hydrocarbon generation.
Conclusion
According to the mineral contents and thin slice analysis, the Dongyuemiao shale is divided into two main shale lithofacies: argillaceous shale and mixed shale. The porosity ranges from 2.95 to 8.43%, and the permeability ranges from 0.05 to 1.07 × 10−3 μm2. The physical properties of the mixed shale are obviously better than those of the argillaceous shale.
The shale in the Dongyuemiao Member is mainly composed of inorganic mineral pores, such as linear pores, between clay minerals and calcite dissolved pores, while a few organic pores are also developed. It is characterized by a high proportion of macropores and a predominance of mesopores. The mesopores provide the main pore volume (61.72% on average), while the micropores provide the main specific surface area.
The TOC content of the shale in the Dongyuemiao Member imposes the main parameter controlling the development of micropores. Clay minerals influence the development of mesopores and macropores, and the combined interaction of diagenesis and hydrocarbon generation controls the formation of pore systems in shale of different lithofacies.
Data Availability Statement
The original contributions presented in the study are included in the article/Supplementary Material, further inquiries can be directed to the corresponding authors.
Author Contributions
PL: Conceptualization, Methodology, Data curation, Writing-Reviewing and Editing. ZL: Supervision. HN: Reviewing and Editing. XL: Reviewing and Editing. QL: Validation. PW: Validation.
Funding
This work was supported by the National Natural Science Foundation of China (Grant Numbers 42090025, 41872124, U20B6001, 42002139), Strategic Priority Research Program of the Chinese Academy of Sciences, China (Grant Number XDA14010404), and the scientific research project of Sinopec Corporation (Grant Number P19017-2, P20046-1).
Conflict of Interest
All authors are employed by SINOPEC. The authors declare no additional commercial or financial relationships that could be construed as a potential conflict of interest.
Publisher’s Note
All claims expressed in this article are solely those of the authors and do not necessarily represent those of their affiliated organizations, or those of the publisher, the editors and the reviewers. Any product that may be evaluated in this article, or claim that may be made by its manufacturer, is not guaranteed or endorsed by the publisher.
Acknowledgments
The authors wish to acknowledge the Jianghan Oil Field for providing the drill cores herein.
References
Dai, J., Zou, C., Liao, S., Dong, D., Ni, Y., Huang, J., et al. (2014). Geochemistry of the Extremely High thermal Maturity Longmaxi Shale Gas, Southern Sichuan Basin. Org. Geochem 74, 3–12. doi:10.1016/j.orggeochem.2014.01.018
Feng, Z., Dong, D., Tian, J., Qiu, Z., Wu, W., and Zhang, C. (2018). Geochemical Characteristics of Longmaxi Formation Shale Gas in the Weiyuan Area, Sichuan Basin, China. J. Pet. Sci. Eng 167, 538–548. doi:10.1016/j.petrol.2018.04.030
Feng, Z., Dong, D., Tian, J., Wu, W., Cai, Y., Shi, Z., et al. (2019). Geochemical Characteristics of Lower Silurian Shale Gas in the Changning-Zhaotong Exploration Blocks, Southern Periphery of the Sichuan Basin. J. Pet. Sci. Eng 174, 281–290. doi:10.1016/j.petrol.2018.11.022
Feng, Z., Hao, F., Dong, D., Zhou, S., Wu, W., Xie, C., et al. (2020). Geochemical Anomalies in the Lower Silurian Shale Gas from the Sichuan Basin, China: Insights from a Rayleigh-type Fractionation Model. Org. Geochem 142, 103981. doi:10.1016/j.orggeochem.2020.103981
Feng, Z., Liu, D., Huang, S., Wu, W., Dong, D., Peng, W., et al. (2016). Carbon Isotopic Composition of Shale Gas in the Silurian Longmaxi Formation of the Changning Area, Sichuan Basin. Pet. Exploration Develop 43 (5), 769–777. doi:10.1016/s1876-3804(16)30092-1
Fu, S. T., Jin, Z. J., Fu, J. H., Li, S. X., and Yang, W. W. (2021). Transformation of Understanding from Tight Oil to Shale Oil in the Member 7 of Yanchang Formation in Ordos Basin and its Significance of Exploration and Development. Acta Petrolei Sinica 42 (05), 561–569.
Guo, X. S., Hu, D. F., Li, Y. P., Wei, X. F., Liu, R. B., Liu, Z. J., et al. (2016). Analyses and Thoughts on Accumulation Mechanisms of marine and Lacustrine Shale Gas: A Case Study of Longmaxi Formation and Da’anzhai Section of Ziliujing Formation in Sichuan Basin. Earth Sci. Front 23 (2), 18–28.
Hill, R. J., Zhang, E., Katz, B. J., and Tang, Y. (2007). Modeling of Gas Generation from the Barnett Shale, Fort worth basin, texas. Bulletin 91 (4), 501–521. doi:10.1306/12060606063
Huang, Z. K., Hao, Y. Q., Li, S. J., Wo, Y. J., Sun, D. S., Li, M. W., et al. (2020). Oil − Bearing Potential, Mobility Evaluation and Significance of Shale Oil in Chang 7 Shale System in the Ordos Basin:A Case Study of Well H317. Geology. China 47 (1), 210–219. doi:10.12029/gc20200117
Jarvie, D. M. (2014). Components and Processes Affecting Producibility and Commerciality of Shale Resource Systems. Geologica Acta 12, 307‒325. doi:10.1344/GeologicaActa2014.12.4.3
Jarvie, D. M. (2012). Shale Resource Systems for Oil and Gas: Part 2: Shale-Oil Resource Systems. AAPG Memoir 97, 89‒119. doi:10.1306/13321447M973489
Jia, C. Z., Zheng, M., and Zhang, Y. F. (2012). Unconventional Hydrocarbon Resources in china and the prospect of Exploration and Development. Pet. Exploration Develop 49 (8), 43–44. doi:10.1016/s1876-3804(12)60026-3
Jin, Z. J., Bai, Z. R., Gao, B., and Li, M. W. (2019). Has China Ushered in the Shale Oil and Gas Revolution? Oil Gas Geology 40 (3), 5–12.
Li, M. W., Jin, Z. J., Dong, M. Z., Ma, X. X., Li, Z. M., Jiang, Q. G., et al. (2020). Advances in the Basic Study of Lacustrine Shale Evolution and Shale Oil Accumulation. Pet. Geology. Exp 42 (04), 489–505. doi:10.11781/sysydz202004489
Li, P., Jiang, Z., Zheng, M., Bi, H., and Chen, L. (2016). Estimation of Shale Gas Adsorption Capacity of the Longmaxi Formation in the Upper Yangtze Platform, China. J. Nat. Gas Sci. Eng 34, 1034–1043. doi:10.1016/j.jngse.2016.07.052
Li, P., Zheng, M., Bi, H., Wu, S., and Wang, X. (2017). Pore Throat Structure and Fractal Characteristics of Tight Oil sandstone: A Case Study in the Ordos Basin, China. J. Pet. Sci. Eng 149, 665–674. doi:10.1016/j.petrol.2016.11.015
Li, Y. Q., and He, D. F. (2014). Evolution of Tectonic-Depositional Environment and Prototype Basins of the Early Jurassic in Sichuan Basin and Adjacent Areas. Acta Petrolei Sinica 35 (2), 219–232. doi:10.7623/syxb201402002
Liu, B., Sun, J. H., Zhang, Y. Q., He, J. L., Fu, X. F., Yang, L., et al. (2021). Reservoir Space and Enrichment Model of Shale Oil in the First Member of Cretaceous Qingshankou Formation in the Changling Sag, Southern Songliao Basin, NE China. Pet. Exploration Develop 48 (03), 521–535. doi:10.1016/s1876-3804(21)60049-6
Liu, Q., Li, P., Jin, Z., Liang, X., Zhu, D., Wu, X., et al. (2021). Preservation of Organic Matter in Shale Linked to Bacterial Sulfate Reduction (BSR) and Volcanic Activity under marine and Lacustrine Depositional Environments. Mar. Pet. Geology 127, 104950. doi:10.1016/j.marpetgeo.2021.104950
Liu, Z. B., Liu, G. X., Hu, Z. Q., Feng, D. J., Zhu, T., Bian, R. K., et al. (2019). Lithofacies Types and Assemblage Features of continental Shale Strata and Their Significance for Shale Gas Exploration: A Case Study of the Middle and Lower Jurassic Strata in the Sichuan Basin. Natur. Gas Ind 39 (12), 10–21. doi:10.3787/j.issn.1000-0976.2019.12.002
Loucks, R. G., Reed, R. M., Ruppel, S. C., and Hammes, U. (2012). Spectrum of Pore Types and Networks in Mudrocks and a Descriptive Classification for Matrix-Related Mudrock Pores. Bulletin 96, 1071–1098. doi:10.1306/08171111061
Nie, H., Jin, Z., and Zhang, J. (20182018). Characteristics of Three Organic Matter Pore Types in the Wufeng-Longmaxi Shale of the Sichuan Basin, Southwest China. Sci. Rep 8 (1), 7014–1098. doi:10.1038/s41598-018-25104-5
Saputra, W., Kirati, W., and Patzek, T. (2019). Generalized Extreme Value Statistics, Physical Scaling and Forecasts of Oil Production in the Bakken Shale. Energies 12 (19), 3641. doi:10.3390/en12193641
Shu, Z. G., Zhou, L., Li, X., Liu, H. T., Zeng, Y., Xie, H. G., et al. (2021). Geological Characteristics of Gas Condensate Reservoirs and Their Exploration and Development prospect in the Jurassic continental Shale of the Dongyuemiao Member of Ziliujing Formation, Fuxing Area, Eastern Sichuan Basin. Oil Gas Geology 42 (01), 212–223. doi:10.11743/ogg20210118
Song, M., Liu, H., Wang, Y., and Liu, Y. (2020). Enrichment Rules and Exploration Practices of Paleogene Shale Oil in Jiyang Depression, Bohai Bay Basin, China. Pet. Exploration Develop 47 (2), 242–253. doi:10.1016/s1876-3804(20)60043-x
Song, Y., Gao, F. L., Tang, X. L., Chen, L., and Wang, X. M. (2020). Influencing Factors of Pore Structure Differences between marine and Terrestrial Shale Reservoirs. Acta Petrolei Sinica 41 (12), 1501–1512. doi:10.7623/syxb202012005
Thommes, M., Kaneko, K., Neimark, A. V., Olivier, J. P., Rodriguez-Reinoso, F., Rouquerol, J., et al. (2015). Physisorption of Gases, with Special Reference to the Evaluation of Surface Area and Pore Size Distribution (IUPAC Technical Report). Pure Appl. Chem 87, 1051–1069. doi:10.1515/pac-2014-1117
Zhang, P., Misch, D., Hu, F., Kostoglou, N., Sachsenhofer, R. F., Liu, Z., et al. (2021). Porosity Evolution in Organic Matter-Rich Shales (Qingshankou Fm.; Songliao Basin, NE China): Implications for Shale Oil Retention. Mar. Pet. Geology 130 (1), 105139. doi:10.1016/j.marpetgeo.2021.105139
Zhang, S., Liu, C., Liang, H., Wang, J., Bai, J., Yang, M., et al. (2018). Paleoenvironmental Conditions, Organic Matter Accumulation, and Unconventional Hydrocarbon Potential for the Permian Lucaogou Formation Organic-Rich Rocks in Santanghu Basin, NW China. Int. J. Coal Geology 185, 44–60. doi:10.1016/j.coal.2017.11.012
Zhang, T. W., Sun, X., Milliken, L. K., Ruppel, C. S., and Enriquez, D. (2017). Empirical Relationship between Gas Composition and thermal Maturity in eagle ford Shale, South texas. AAPG Bull 101 (8), 1277–1307. doi:10.1306/09221615209
Zheng, R. C., He, L., Liang, X. W., and Xu, W. L. (2013). Forming Conditions of Shale Gas (Oil) Plays in the Lower Jurassic Da’anzhai Member in the Eastern Sichuan Basin. Natur. Gas Ind 33 (12), 30–40. doi:10.3787/j.issn.1000-0976.2013.12.004
Zou, C. N., Yang, Z., Sun, S. S., Zhao, Q., Bai, W. H., Liu, H. L., et al. (2020). Exploring Petroleum inside Source Kitchen": Shale Oil and Gas in Sichuan basin. Sci. China Earth Sci 63 (7). doi:10.1007/s11430-019-9591-5
Zou, C., Yang, Z., Cui, J., Zhu, R., Hou, L., Tao, S., et al. (2013). Formation Mechanism, Geological Characteristics and Development Strategy of Nonmarine Shale Oil in china. Pet. Exploration Develop 40 (1), 15–27. doi:10.1016/s1876-3804(13)60002-6
Keywords: heterogeneity, lacustrine shale, lithofacies, dongyuemiao member, sichuan basin
Citation: Li P, Liu Z, Nie H, Liang X, Li Q and Wang P (2021) Heterogeneity Characteristics of Lacustrine Shale Oil Reservoir Under the Control of Lithofacies: A Case Study of the Dongyuemiao Member of Jurassic Ziliujing Formation, Sichuan Basin. Front. Earth Sci. 9:736544. doi: 10.3389/feart.2021.736544
Received: 05 July 2021; Accepted: 01 September 2021;
Published: 17 September 2021.
Edited by:
Guochang Wang, Saint Francis University, United StatesReviewed by:
Chao Liang, China University of Petroleum, ChinaJianhua Zhao, China University of Petroleum, China
Copyright © 2021 Li, Liu, Nie, Liang, Li and Wang. This is an open-access article distributed under the terms of the Creative Commons Attribution License (CC BY). The use, distribution or reproduction in other forums is permitted, provided the original author(s) and the copyright owner(s) are credited and that the original publication in this journal is cited, in accordance with accepted academic practice. No use, distribution or reproduction is permitted which does not comply with these terms.
*Correspondence: Haikuan Nie, bmllaGsuc3lreUBzaW5vcGVjLmNvbQ==; Xinping Liang, eGlucGluZy5saWFuZ0Bwa3UuZWR1LmNu