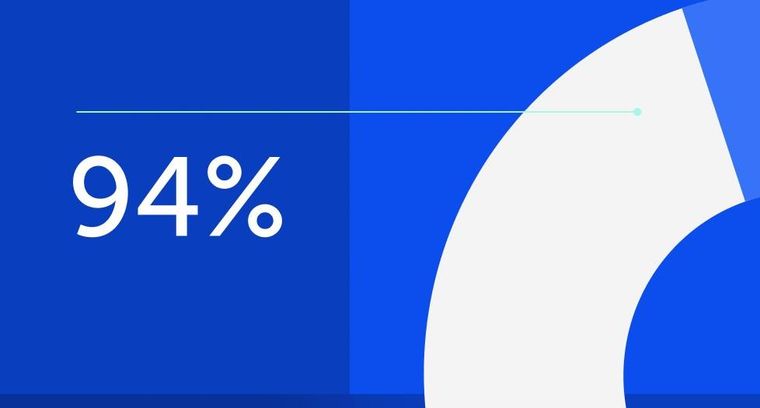
94% of researchers rate our articles as excellent or good
Learn more about the work of our research integrity team to safeguard the quality of each article we publish.
Find out more
ORIGINAL RESEARCH article
Front. Earth Sci., 19 August 2021
Sec. Sedimentology, Stratigraphy and Diagenesis
Volume 9 - 2021 | https://doi.org/10.3389/feart.2021.733397
This article is part of the Research TopicAdvances in Geology of Unconventional Hydrocarbon ResourcesView all 17 articles
Sichuan Basin is the only successful basin for shale gas exploration in China. In addition to the main shale in the Lower Silurian Longmaxi formation, the lower Cambrian Qiongzhusi shale is an important potential formation. However, it was once considered that shale gas is difficult to enrich because of its poor sealing conditions and hydrocarbon migration to adjacent reservoirs. With the increasing research on hydrocarbon generation and reservoir in shale of Qiongzhusi Formation in recent years, it has become an important exploration target in Sichuan Basin. The enrichment of oil and gas is closely related to fluid activities. Limited by the degree of exploration, there is little analysis of fluid activities in Qiongzhusi Formation, and there is little analysis of shale gas enrichment potential from the perspective of fluid. The hydrocarbon generated from Qiongzhusi shale in the rift could migrate laterally to the uplift area and form a reservoir in Dengying Formation. The fluid activities from source rock to reservoir are basically the same. Therefore, this paper reconstructed the history of hydrocarbon activities in Dengying reservoirs based on fluid inclusion analysis. Then the fluid activity process in Qiongzhusi shale was studied, and its enrichment conditions of shale gas was discussed. The results show that the hydrocarbon activities of Dengying Formation can be divided into three stages: 1) oil charging stage, 2) oil cracking gas generation stage and 3) gas reservoir adjustment stage. The first stage is under normal pressure, and the second and third stages developed overpressure with pressure coefficients of 1.3 and 1.2, respectively. High pressure coefficient is an important indicator of shale gas enrichment. Because the source rock of Qiongzhusi Formation has always been the main source rock of Dengying Formation, it can supply hydrocarbon to Dengying Formation only with overpressure in gas generation stage. Therefore, overpressure in the last two stages of gas generation indeed existed. As long as the sealing condition of shale itself is not particularly poor, shale gas “sweet points” would be formed. Therefore, the thick shale in Deyang-Anyue rift is the focus of shale gas exploration in Qiongzhusi Formation.
Shale gas is a kind of unconventional natural gas and has been successfully developed commercially abroad. It has become an important new energy (Han, et al., 2016). However, China started late and made a breakthrough in Sichuan Basin until 2010, when shale gas was drilled in Wufeng Formation of Upper Ordovician and Longmaxi formation of Lower Silurian (Huang et al., 2012; Jin et al., 2016). After more than a decade of exploration, China has made important progress in Yongchuan, Fuling, Weiyuan, Changning and other places, developing the shale gas fields (Zou et al., 2020). Sichuan Basin has become the second largest shale gas production area after the United States (Yang et al., 2021). So far, China has started to exploit the shale gas in marine strata with the depth shallower than 3500 m in the southern Sichuan Basin (Yang et al., 2021). In addition, some petroleum companies has begun to focus on the deeper layers of the basin, including the deeper buried Wufeng and Longmaxi shale, the third member of the Sinian Dengying Formation and the lower Cambrian Qiongzhusi shale. In particular, the Qiongzhusi shale is adequately thick, mature and has great potential for shale gas (Huang et al., 2012; Potter, 2018). Although only a few wells were designed to explore the Qiongzhusi shale at present, it was really successful because some of them successfully produced natural gas after fracturing. However, because of low degree of exploration, the research on Qiongzhusi shale gas is not enough. Previous studies mainly focused on the source rock qualities (Huang et al., 2012; Zheng et al., 2014; Wei et al., 2015; Yang et al., 2020), and some of them preliminarily analyzed the reservoir characteristics (Gao et al., 2020; Wang et al., 2016). The history of hydrocarbon evolution is still not clear, which restricts the further exploration.
The Sinian Dengying Formation is dominated by dolomite in Sichuan Basin. There is an unconformity between it and the overlying Qiongzhusi Formation. This unconformity provided a good pathway for oil migration from Qiongzhusi shale to the top of Dengying Formation (Zhou et al., 2016). In the Anyue gas field discovered in Gaoshiti-Moxi area, the Sinian Dengying Formation and Lower Cambrian formed the petroleum system, and the natural gas in the Dengying Formation was mainly generated from the Qiongzhusi Formation (Han et al., 2013; Zheng et al., 2014; Wei et al., 2015). Consequently, if lacking of data and being unable to directly study Qiongzhusi shale, the hydrocarbon evolution of Qiongzhusi shale could be interpreted by analyzing the hydrocarbon activities of the Dengying reservoirs. Therefore, using the fluid inclusions bearing in Dengying reservoirs, this paper studied the history of fluid activities, and then analyzed the hydrocarbon evolution of Qiongzhusi shale. The results can provide reference for further shale gas exploration of Qiongzhusi shale in Sichuan Basin and surrounding areas.
Sichuan Basin is located in Southwest China. It is the most important natural gas producing area and the only shale gas producing area in China. Gaoshiti-Moxi area, where the Aanyue gas field was discovered, is located in the middle of Sichuan Basin. It is the core of a successively developing uplift (Figure 1A). The Dengying Formation included four members numbered 1–4 respectively from bottom to top. The second and fourth members were relatively rich in cyanobacteria and formed algal mounds and granular beaches on carbonate platform (Li et al., 2013; Zhou et al., 2014). They were also the main reservoirs of Dengying Formation. At the end of Sinian, the basement of Sichuan Basin was uplifted due to Tongwan movement, and the top of Dengying Formation was extensively eroded. Two regional unconformities were formed at the top of the second member (Deng 2) and the fourth member (Deng 4) respectively. Meanwhile, two paleogeomorphic highlands, Gaoshiti-Moxi and Weiyuan-Ziyang, were formed in the same process (Du et al., 2014). Between the Highlands was Deyang-Anyue rift. The Cambrian system deposited over the erosion surface (Zou et al., 2010). The stratigraphic column of the paleouplift area is shown in Figure 1B. The Sinian Dengying formation (member 2 and 4) and the lower Cambrian Longwangmiao Formation are the main reservoirs, while the lower Cambrian Qiongzhusi Formation is the main source rock. The thickness of Qiongzhusi Formation is generally 120–150 m, while it is up to 350 m in the rift (Zou et al., 2020). The lower part of the Qiongzhusi Formation is dark gray carbonaceous shale and silty shale, and the color gradually becomes lighter upward. Some gray calcareous fine sandstone or siltstone are stuck in the middle, and the top part is yellow green shale. Its petrologic characteristics show that the Qiongzhusi Formation was formed in shallow or deep sea shelf (Yang et al., 2020). Previous studies suggested that the rift was the sedimentary center of high-quality source rocks in the lower Cambrian (Han et al., 2013; Wei et al., 2015; Zheng et al., 2014), the generated hydrocarbon could migrate laterally to the reservoirs on both sides of the rift (Figure 1C). Now, the Weiyuan and Anyue gas fields have been found on both sides of the rift, and shale gas is also under exploration (Zou et al., 2014, 2020; Wei et al., 2017; Chen, et al., 2021).
FIGURE 1. Geographical location (A), stratigraphic column (B) and a representative profile of the paleouplift (C) in Sichuan Basin.
Qiongzhusi Formation is one of the main source rocks in Sichuan Basin. In the uplift area, its TOC ranges from 0.5 to 8.49%, with an average of 1.95% (Wei et al., 2015, 2017). Within the rift, the TOC ranges from 0.5 to 25.7% (Zhu et al., 2006; Zou et al., 2010; Huang et al., 2012), organic rich carbonaceous shales were mainly developed in the lower part of the Qiongzhusi Formation, with TOC generally greater than 2%, and TOC decreases upward. The carbon isotopic of kerogen is generally light, ranging from −36.0‰ to −31.0‰, with an average of 33.3‰ (Wei et al., 2017). The black shale of Qiongzhusi Formation is mainly composed of sapropel macerals (more than 95%) and a small amount of humic macerals, which has great hydrocarbon generation potential. In addition, the mature degree of Qiongzhusi shale is very high. In the uplift area, the equivalent vitrinite reflectance is 1.84–2.42% (Wei et al., 2017), reaching the high or/and over mature stage. In the rift area, the equivalent vitrinite reflectance is generally greater than 2.5%, with an average of 3.5%, which is in the over mature stage (Zou et al., 2006; Wang et al., 2010; Huang et al., 2012). The pore structure and type of shale in Qiongzhusi Formation are similar to those in the Longmaxi Formation, the main shale gas procucing formation in Sichuan Basin (Wang et al., 2013). According to the analysis of drilling samples, the porosity of Qiongzhusi shale is 0.82–4.86%, with an average of 2.44%, and the permeability is 0.006 × 10–3∼0.158 × 10–3 μm2, with an average of 0.046 × 10−3μm2 (Huang et al., 2012). This indicates that the Qiongzhusi shale in the study area can be good reservoir for shale gas. Therefore, the hydrocarbon generation capacity of the Qiongzhusi shale was very strong, and part of the hydrocarbon was kept in the shale, and the other migrated from the source rock to the lateral Sinian Dengying reservoirs. The hydrocarbon activities related the source rock and reservoirs, which provides a theoretical basis for studying the hydrocarbon evolution of the Qiongzhusi Formation by analyzing the fluid activities of the Dengying reservoirs.
Fluid inclusions directly record the paleofluid, and is the main method to study paleofluid activities (Wang et al., 2020). In this paper, fluid inclusion analysis was used to describe the fluid evolution of reservoir, and then to analyze the fluid activities in source rocks. 12 samples were collected from 9 exploration wells such as GS21 in Gaoshiti-Moxi area, Sichuan Basin. The depth of the selected samples ranged from 5,010 m to 5,450 m. They were all from the fourth member of Dengying Formation. All samples were made into doubly-polished thick sections in order to observe and analyze fluid inclusions in transparent minerals. Bitumen often exists in the samples, filling in the intergranular pores and dissolution cavities. The dolomite crystals in the cavities is generally clean and bright, maybe resulting from recrystallization. Some cavities contain authigenic quartz, which are mostly wrapped by bitumen.
In order to clarify the characteristics of fluid activities and evolution of ancient oil reservoirs, we conducted a series of experiments of fluid inclusions based on microscope observation. Petrographic observation, microthermometric measurement and Raman spectroscopy were combined to study the fluid activities. All the experiments were conducted in the Lab of Fluid Inclusions in China University of Petroleum (East China). A polarizing/fluorescence microscope (Leica DM 2700p) was used for petrographic observation. Microthermometric measurements of fluid inclusions (FIs) were done on a heating/freezing stage (Linkam THMSG600) with an estimated uncertainty of ±0.1°C. Raman analyses were conducted with a laser Raman spectrometer (Horiba LabRam-010). Excitation was achieved using a laser tuned to 532 nm. The diameter of the confocal hole was 400 µm and the slit-width was 100 µm. The accumulation time was 10 s and each measurement was made twice. Raman shift was calibrated using a silicon wafer before collection.
Pores, cavities and fractures commonly occur in the dolomite of Dengying Formation. They are the main reservoir space of hydrocarbon. The cavities in the second member are generally larger (Figure 2A), while the ones in the fourth member are smaller (Figure 2B). The cavities are usually filled with bitumen and minerals, such as recrystallized dolomite, quartz and a little of fluorite. Microscopic photos show that bitumen is also widely developed among dolomite grains (Figure 2C), but the degree of filling is different, indicating that the carbonate reservoir has been filled with oil to varying degrees, and the oil has been fully cracked in a high degree of thermal evolution process. In the dissolution cavity, bright dolomite always occur along the wall. Some smaller pores can be completely filled with dolomite, but most of them are also filled with bitumen (Figure 2D), which is the residue of paleo-oil cracking. Some larger cavities also precipitate quartz (Figure 2E) or fluorite (Figure 2F), which are generally located in the center of the cavities and surrounded by bitumen. This phenomenon shows that the quartz or fluorite were formed after paleo-oil filling, and can record the evolution process after paleo-oil filling.
FIGURE 2. Photograps of filled pores in dolomite of Dengying formation in Sichuan basin. (A) The photo shows a big cavity filled with bitumen and quartz; (B) The photo shows three small cavities filled with bitumen; (C) shows the bitumen filled in the intergranular pores; (D) A cavity filled with recrystallized dolomite along the wall and bitumen in the center; (E) A cavity filled with recrystallized dolomite along the wall, quartz in the center and bitumen sandwiched between the two minerals; (F) A cavity filled with recrystallized dolomite, bitumen, quartz and fluorite. Bit = Bitumen; Qtz = Quartz; Dol = Dolomite; Fl = Fluorite.
In order to interpret the relationship between the fillings in the cavities better, the occurrences of fluid inclusions hosted in different minerals were observed. In the recrystallized dolomite, fluid inclusions are very abundant, mainly gas-liquid two-phase inclusions, and only a few methane bearing aqueous inclusions are found. There is no bitumen in the grain, but the bitumen is only located among the crystals, which indicates that it may be formed earlier than the oil injection. Quartz is generally located in the center of the cavity and surrounded by bitumen (Figure 3A). Fluid inclusions hosted in quartz are also very abundant. There are various types of inclusions, including aqueous inclusions, pure methane gas inclusions (Figure 3B), bitumen and methane bearing gas inclusions (Figure 3C) and methane bearing aqueous inclusions (Figure 3D). A few fluorite grains are found in the cavities, but they contain a lot of fluid inclusions. The type is the same as that in quartz, which may precipitate in the same period as quartz.
FIGURE 3. Different kinds of fluid inclusions in quartz filled in the cavity of dolomite in Dengying formation. (A) A perfect crystal of quartz; Raman spectra respectively show (B) methane gas inclusion, (C) methane gas inclusion with bitumen, and (D) methane-bearing two-phase aqueous inclusion.
The microthermonetric results are summarized in Table 1. The fluid inclusions in recrystallized dolomite are almost all aqueous inclusions. Only one methane bearing inclusion was found, and its bubble didn’t change when the temperature was above 250°C, indicating that it is inhomogeneous and may be formed by mixing methane into the original fluid inclusion. The distribution of homogenization temperature is very wide, ranging from 71.9 to 186.6°C. Dolomite is carbonate rock with perfect cleavage and low hardness, which make it easy to be re-equilibrated under over heat and pressure. Therefore, the information recorded by fluid inclusions in dolomite may be changed due to the change of temperature and pressure in the later periods. This restricts its application. In particular, the homogenization temperature of fluid inclusions in quartz indicates that the reservoir has indeed experienced high temperature. However, quartz is relatively more stable than dolomite and not easy to be re-equilibrated. In addition, there are many methane bearing aqueous inclusions in fluorite. Due to their regular shape and pulsating bubbles without restriction, the bubbles run away when they are irradiated by laser under Raman spectroscope. It is not easy to completely identify their types. Their homogenization temperatures are very high and similar (Table 1), so it is difficult to quantitatively analyze them. Therefore, this paper only used the microthermometric data of fluid inclusions in quartz for paleofluid analysis.
TABLE 1. Microthermometric data and calculations based on fluid inclusions from Sinian Dengyingzu formation in Sichuan basin.
According to the above description, quartz crystallized after the earliest hydrocarbon charging. The coexistence of fluid inclusions in quartz, such as methane gas inclusions, methane-bearing aqueous inclusions, aqueous inclusions and even some bitumen inclusions, indicates that the precipitation of quartz is consistent with the process of early oil-gas transformation and the later natural gas activities. The cavities developed in some core samples deposited some bitumen and quartz, but are not filled completely. Growth striation can be found in some quartz crystals, and this can be interpreted that the precipitation of quartz is a continuous process. So the fluid activities after the early oil charging can be fully expressed through multi particle comprehensive measurements.
The homogenization temperature histogram of fluid inclusions in quartz is shown in Figure 4. Figure 4A shows the homogenization temperature histogram of aqueous inclusions in quartz, and Figure 4B shows the homogenization temperature histogram of methane-bearing inclusions. According to the distribution and occurrence of fluid inclusions in minerals, combined with their homogenization temperature and salinity, the fluid inclusions in quartz can be divided into three stages. The three stages of aqueous inclusions and methane-bearing aqueous inclusions correspond one by one. The data of homogenization temperatures and salinities are shown in Table 1. The methane-bearing aqueous inclusions are immiscible inclusions. We can assume that the methane is saturated during trapping, so the homogenization temperature equals to the trapping temperature. Combined with the homogenization temperatures and salinities data of aqueous inclusions, the trapping temperatures and pressures of fluid inclusions in different periods can be calculated. Microthermometric data were interpreted using the equations given by Bodnar and Vityk (1994) as implemented in the program “HokieFlincs” (Steele-MacInnis et al., 2012). The calculation results are also listed in Table 1. In addition, a small number of inclusions with low homogenization temperature hosted in some quartz and located near the wall, might be trapped at the beginning of early quartz precipitation.
FIGURE 4. Histograms of the homogenization temperatures of (A) aqueous inclusions and (B) methane-bearing inclusions in quartz filled in cavities of dolomite in Sinian Dengying Formation.
From the above analysis, it can be seen that quartz precipitation should be later than the earliest oil charging. Therefore, the lowest homogenization temperature of fluid inclusions in quartz can limit the latest time for the ancient reservoir to start charging. According to the burial history and thermal history of Gaoshiti-Moxi area (Wei et al., 2017; Shuai et al., 2021), the activity history of fluid can be obtained by projecting the calculated trapping temperature onto the burial history map (Figure 5). The burial depth of the formation bearing fluid inclusions at the trapping period can be obtained easily, and then the pressure coefficient can be calculated by dividing the trapping pressure by hydrostatic pressure.
FIGURE 5. The fluid evolution mode of the Sinian Dengying reservoirs in the Gaoshiti-Moxi area, Sichuan basin (modified from Wei et al., 2017 and Shuai et al., 2021).
According to the maturity of source rocks simulated in the burial history map, it can be deduced that the earliest generation and migration of hydrocarbon occurred in Ordovician. The lowest homogenization temperature of fluid inclusions in quartz indicates that the period of quartz precipitation may be Permian, when some hydrocarbon has been injected into the reservoirs.
Fluid inclusions in quartz mainly record three stages of fluid activities. The first stage occurred in the Late Permian or early Triassic, in the late Hercynian movement and early Indosinian movement. After a long period of uplift and erosion from Devonian to Carboniferous, the basin subsided again, burial deepened rapidly and temperature increased rapidly at the same time. The organic matter in the source rocks continued to mature and generate a large amount of hydrocarbon, which migrated and formed an ancient reservoir. All the methane-bearing inclusions in this period also contained bitumen in them, indicating that when the inclusions were trapped, they did not directly capture natural gas, but captured oil, and methane was the result of later transformation. The calculated pressure coefficient at this period was 1.0, indicating that it was normal pressure in the reservoir at this period. Fluid activity in this period was mainly oil generation, migration and accumulation, which was the largest oil activity and the last prosperity of oil activity, because with the rapid rise of temperature, oil was facing cracking.
The second stage of fluid activities recorded in quartz occurred in Jurassic, which belongs to the early Yanshanian movement. With the continuous increase of burial depth, the temperature increased rapidly, and it was close to 180°C. The early formed ancient oil reservoirs had begun to crack, release methane and remain bitumen, and the oil reservoirs had transformed into gas reservoirs. The oil in the cavities gradually changed into bitumen, and quartz precipitated in some cavities, which squeezed the space and made bitumen distributed at the edge of the cavities. The fluid activity in this period was the largest ancient reservoir transformation from oil to gas. The calculated reservoir pressure coefficient reached 1.3, indicating that the reservoir generally develops over pressure and the oil and gas activities had reached the peak.
The third stage of fluid activities occurred in the Cretaceous, which is the late Yanshanian. At this time, the buried depth reached the deepest, and the temperature exceeded 200°C. The oil in the reservoir had already been cracked. The pressure coefficient in the reservoir decreased to less than 1.2, indicating the leakage of natural gas in the reservoir. In addition to quartz, some pores were filled with a small amount of fluorite, which indicated that there were external hydrothermal fluids entering the reservoir. All these phenomena indicated that the gas reservoirs were reformed and adjusted again, which was closely related to the basin stopping subsidence and turning to uplift.
To sum up, fluid inclusions in quartz record the fluid activities of reservoir after initial hydrocarbon injection. We described the fluid evolution process of the formation, cracking and destruction of the ancient oil reservoirs and the reconstruction of the gas reservoirs in Dengying Formation.
Sichuan Basin is the only basin with successful shale gas exploration in China. The main stratum is upper Ordovician Wufeng Formation and Lower Silurian Longmaxi Formation, mainly distributed in the northeast of Sichuan Basin. In addition to this layer of shale, the most likely breakthrough stratum is the lower Cambrian Qiongzhusi Formation shale. The Qiongzhusi Formation is above the Sinian Dengying Formation with an unconformity, and its thickness greatly increases in the Deyang-Anyue rift. It is in lateral contact with the Dengying Formation and is basically at the same depth in the rift. The above description illustrates the evolution process of hydrocarbon fluid in the Sinian Dengying Formation, which also has a good indication for shale gas exploration in the Qiongzhusi Formation with the same buried depth, because the oil in the Dengying Formation is mainly from the mature hydrocarbon generation of the shale in the Qiongzhusi Formation. The thermal history evolution of Dengying Formation is the same as that of Qiongzhusi Formation.
Figure 5 shows the hydrocarbon generation history of the source rocks. It mainly generated oil at the beginning. With the increase of burial depth and temperature, the source rocks reached the peak of oil generation, which corresponded to the fluid activity in the first stage of Dengying Formation. After that, the temperature exceeded 150°C and the oil began to crack. The kerogen and oil in the source rocks also began to crack, and the rapid transition from oil generation to gas generation corresponds to the second stage of fluid activity. Since then, the gas reservoir transformation and adjustment also occurred in the source rocks of the Qiongzhusi Formation. Because its lower part was in unconformity contact with the Dengying Formation, forming a good carrier layer, the natural gas generated in the shale was easy to escape along the unconformity surface. This may be an important factor that it is difficult to break through in the previous shale gas exploration of the Qiongzhusi Formation. However, the Qiongzhusi formation in the rift had large thickness, high TOC content and high maturity, so there is a great possibility of exploring “sweet point”.
According to the carbon isotope characteristics of natural gas and bitumen, it is considered that the natural gas in Dengying Formation mainly is generated from kerogen rather than oil cracking (Shuai et al., 2021). This indicates that during the second and third stage of fluid activities, the Qiongzhusi Formation has been generating natural gas and providing gas to the lower Dengying Formation and the upper Longwangmiao Formation. High pressure coefficient is an important indicator of shale gas enrichment. The pressure coefficient of Dengying Formation was 1.3 in the second stage, and 1.2 in the third stage of fluid activities. The Qiongzhusi Formation was still the main gas source of Dengying Formation, which indicates that the pressure coefficient of the Qiongzhusi Formation in some areas was more than 1.2, or even more than 1.3. Only in this way could it provide gas to Dengying Formation reservoirs. For example, the pressure coefficient of shale gas reservoirs in Longmaxi Formation, the main shale gas production layer in Sichuan Basin, is about 1.2. Hence the Qiongzhusi Formation has a well potential of shale gas exploration, especially in the rift area, where the upper and lower sealing ability is good enough, it is easier to form shale gas “sweet points” because of larger thickness. Therefore, the fluid evolution process of Dengying Formation reservoir shows that the Qiongzhusi shale in the rift has the conditions to form shale gas “sweet points”, and strengthening exploration is helpful to find them. In recent years, some petroleum companies have strengthened exploration efforts in the rift area. In the Weiyuan area, some industrial wells have been successfully drilled and gradually been put into production. It is believed that more shale gas “sweet points” will be found in the future.
1) Dolomite, bitumen, quartz and fluorite are filled in the pores or cavities of dolomite reservoir of Sinian Dengying Formation in Gaoshiti Moxi structure of Sichuan Basin. Fluid inclusions in quartz record the evolution process of fluid after the earliest oil injection. This process can be divided into three stages: 1) oil charging stage. Charging occurred with normal pressure. It is dominated by oil migration and accumulation, and also the peak of oil activities. 2) Stage of gas generation through oil cracking. The temperature had exceeded 150°C. Oil began to crack with the residual bitumen. The pressure coefficient were 1.3. 3) Adjustment stage of gas reservoir. The maximum temperature had exceeded 200°C. The basin subsided first and then uplifted, and the original gas reservoirs were destroyed, and began to transform and adjust to new conditions. The pressure coefficient was 1.2.
2) The lower Cambrian Qiongzhusi Formation is the main source rock of Dengying Formation. In the second and third stage of fluid activities, Dengying reservoirs developed over pressure, and the gas source were mainly kerogen cracking gas from Qiongzhusi shale. This indicates the higher hydrocarbon generation pressure in Qiongzhusi Formation. Together with its thickness, high TOC content and over mature, it has good shale gas exploration potential.
The original contributions presented in the study are included in the article/Supplementary Material, further inquiries can be directed to the corresponding author.
ZZ carried out conceptualization, methodology, writing original draft, and validation and software. XC conducted the microthermometric measurements and Raman spectroscopy. HX also conducted the microthermometric measurements and Raman spectroscopy.
This work was supported by the National Natural Science Foundation of China (Grant No. 41703060).
The authors declare that the research was conducted in the absence of any commercial or financial relationships that could be construed as a potential conflict of interest.
All claims expressed in this article are solely those of the authors and do not necessarily represent those of their affiliated organizations, or those of the publisher, the editors and the reviewers. Any product that may be evaluated in this article, or claim that may be made by its manufacturer, is not guaranteed or endorsed by the publisher.
Bodnar, R. J., and Vityk, M. O. (1994). “Interpretation of Microthermometric Data for H2O-NaCl Fluid Inclusions,” in Fluid Inclusions in Minerals, Methods and Applications. Editors B. De Vivo, and M. L. Frezzotti (Blacksburg, VA: Virginia Tech), 117–130.
Chen, S., Gong, Z., Li, X., Wang, H., Wang, Y., and Zhang, Y. (2021). Pore Structure and Heterogeneity of Shale Gas Reservoirs and its Effect on Gas Storage Capacity in the Qiongzhusi Formation. Geosci. Front. 12, 101244. doi:10.1016/j.gsf.2021.101244
Du, J. H., Zou, C. N., and Xu, C. C. (2014). Theoretical and Technical Innovations in Strategic Discovery of a Giant Gas Field in Cambrian Longwangmiao Formation of central Sichuan Paleo-Uplift, Sichuan Basin. Pet. Exploration Dev. 41 (3), 268–277. doi:10.1016/s1876-3804(14)60035-5
Gao, B., Liu, Z. B., Shu, Z. G., Liu, H. T., Wang, R. Y., Jin, Z. G., et al. (2020). Reservoir Characteristics and Exploration of the Lower Cambrian Shale Gas in the Middle-Upper Yangtze Area. Oil Gas Geology. 41 (2), 284–294. doi:10.11743/ogg20200205
Han, C., Jiang, Z., Han, M., Wu, M., and Lin, W. (2016). The Lithofacies and Reservoir Characteristics of the Upper Ordovician and Lower Silurian Black Shale in the Southern Sichuan Basin and its Periphery, China. Mar. Pet. Geology. 75, 181–191. doi:10.1016/j.marpetgeo.2016.04.014
Han, H., Li, D., Ma, Y., Cheng, L., Qi, L., Wang, Q., et al. (2013). The Origin of marine Shale Gas in the Northeastern Sichuan Basin, China: Implications from Chemical Composition and Stable Carbon Isotope of Desorbed Gas. Shiyou Xuebao/Acta Petrolei Sinica 34, 453–459. doi:10.7623/syxb201303005
Huang, J. L., Zou, C. N., Li, J. Z., Dong, D. Z., Wang, S. J., Wang, S. Q., et al. (2012). Shale Gas Generation and Potential of the Lower Cambrian Qiongzhusi Formation in Southern Sichuan Basin, China. Pet. Exploration Dev. 39, 69–75. doi:10.1016/s1876-3804(12)60017-2
Jin, Z. J., Hu, Z. Q,, Gao, B., and Zhao, J. H. (2016). Controlling Factors on the Enrichment and High Productivity of Shale Gas in the Wufeng-Longmaxi Formation, southeastern Sichuan Basin. Earth Sci. Front. 23 (1), 1–10.
Li, L., Tan, X. C., and Zeng, W. (2013). Development Characteristics and Reservoir Significance of Mortar mounds in the Sinian Dengying Formation in Sichuan Basin. Pet. Exploration Dev. 40, 666–673. doi:10.1016/s1876-3804(13)60096-8
Potter, C. J. (2018). Paleozoic Shale Gas Resources in the Sichuan Basin, China. AAPG Bull. 102 (6), 987–1009. doi:10.1306/0828171607817072
Shuai, Y. H., Liu, K. Y., Hu, G. Y., Wang, T. S., Tian, X. W., Zhang, B., et al. (2021). Gas Accumulation Mechanisms of the Sinian Reservoir in the Sichuan basin and Their Significance for Deep Gas Exploration. Acta Geologica Sinica 95. doi:10.19762/j.cnki.dizhixuebao.2021141
Steele-MacInnis, M., Lecumberri-Sanchez, P., and Bodnar, R. J. (2012). HokieFlincs_H2O-NaCl : A Microsoft Excel Spreadsheet for Interpreting Microthermometric Data from Fluid Inclusions Based on the PVTX Properties of H 2 O-NaCl. Comput. Geosciences 49, 334–337. doi:10.1016/j.cageo.2012.01.022
Wang, D. F., Wang, Y. M., Dong, D. Z., Wang, S. Q., Huang, J. L., Huang, Y. B., et al. (2013). Quantitative Characterization of Reservoir Space in the Lower Cambrian Qiongzhusi Shale, Southern Sichuan Basin. Nat. Gas Industry 33 (7), 1–10.
Wang, F. Y., Chen, J. Y., and Gao, G. (2010). Reflectance of Macroalgae-Derived Vitrinite-like Macerals: An Organic Maturity Indicator for Pre-devonian marine Strata. Pet. Exploration Dev. 37 (2), 250–256.
Wang, M., Chen, Y., Bain, W. M., Song, G., Liu, K., Zhou, Z., et al. (2020). Direct Evidence for Fluid Overpressure during Hydrocarbon Generation and Expulsion from Organic-Rich Shales. Geology 48, 374–378. doi:10.1130/g46650.1
Wang, T., Xiong, L., Xu, M., Dong, X. X., Zhou, R. Q., and Fu, X. N. (2016). Shale Reservoir Characteristics of the Lower Cambrian Qiongzhusi Formation in the Southern Sichuan Basin. Peroleum Geology. Exp. 38 (2), 197–203. doi:10.11781/sysydz201602197210
Wei, G. Q., Wang, Z. H., and Li, J. (2017). Characteristics of Source Rocks, Resource Potential and Exploration Direction of Sinian and Cambrian in Sichuan Basin. Nat. Gas Geosci. 28 (1), 1–13.
Wei, G. Q., Xie, Z. Y., Song, J. R., Yang, W., Wang, Z. H., Li, J., et al. (2015). Features and Origin of Natural Gas in the Sinian-Cambrian of central Sichuan Paleo-Uplift, Sichuan Basin, SW China. Pet. Exploration Dev. 42 (6), 702–711. doi:10.1016/s1876-3804(15)30073-2
Yang, S. J., Wang, W. F., Zhang, D. L., Fu, X. D., and Zhang&Li, J. Y. W. Z. (2020). Distribution Characteristics and Formation Environment of High Quality Source Rocks of Qiangzhusi Formation in Northeastern Sichuan Basin. Nat. Gas Geosci. 31, 507–517.
Yang, Y. M., Chen, Y. L., Liu, S. Y., Deng, B., Xu, H., Chen, L. Q., et al. (2021). Status, Potential and prospect of Shale Gas Exploration and Development in the Sichuan Basin and its Periphery. NATURAL GAS INDUSTRY 41, 42–58. doi:10.3787/j.issn.1000-0976.2021.01.004
Zheng, P., Shi, Y., Zou, C., Kong, L., and Liu, J. (2014). Natural Gas Sources in the Dengying and Longwangmiao Fms in the Gaoshiti-Maoxi Area, Sichuan basin. Nat. Gas Industry 34 (3), 50–54.
Zhou, W., Xu, H., Yu, Q., Xie, R. C., and Deng, K. (2016). Shale Gas-Bearing Property Differences and Their Genesis between Wufeng-Longmaxi Formation and Qiongzhusi Formation in Sichuan Basin and Surrounding Areas. Lithologic Reservoirs 28 (5), 18–25.
Zhou, Z., Wang, X. Z., and Xie, L. (2014). Reservoir Characteristics and Physical Property Influencing Factors of Sinian Dengying Formation in central Sichuan Basin. Nat. Gas Earth Sci. 25, 701–708.
Zhu, G. Y., Zhang, S. C., and Liang, Y. B. (2006). The Characteristics of Natural Gas in Sichuan basin and its Sources. Earth Sci. Front. 13 (2), 234–248.
Zou, C. N., Du, J. T., Xu, C. C., Wang, Z. C., Zhang, B. M., Wei, G. Q., et al. (2014). Formation, Distribution, Resource Potential and Discovery of the Sinian-Cambrian Giant Gas Field, Sichuan Basin, SW China. Pet. Exploration Dev. 41 (3), 278–293. doi:10.1016/s1876-3804(14)60036-7
Zou, C. N., Zhang, G. Y., and Tao, S. Z. (2010). Geological Features, Major Discoveries and Unconventional Petroleum Geology in the Global Petroleum Exploration. Pet. Exploration Dev. 37 (2), 129–145.
Keywords: fluid inclusion, fluid activity, shale gas, Sichuan Basin, Qiongzhusi Formation
Citation: Zhou Z, Chen X and Xia H (2021) The Fluid Evolution of Ancient Carbonate Reservoirs in Sichuan Basin and Its Implication for Shale Gas Exploration. Front. Earth Sci. 9:733397. doi: 10.3389/feart.2021.733397
Received: 01 July 2021; Accepted: 09 August 2021;
Published: 19 August 2021.
Edited by:
Jon Jincai Zhang, Sinopec Tech Houston Center (STHC), United StatesReviewed by:
Yongqiang Yang, China University of Petroleum, ChinaCopyright © 2021 Zhou, Chen and Xia. This is an open-access article distributed under the terms of the Creative Commons Attribution License (CC BY). The use, distribution or reproduction in other forums is permitted, provided the original author(s) and the copyright owner(s) are credited and that the original publication in this journal is cited, in accordance with accepted academic practice. No use, distribution or reproduction is permitted which does not comply with these terms.
*Correspondence: Zhenzhu Zhou, emhvdXpoZW56aHUxOTgzQDE2My5jb20=; Xiaolan Chen, MTU3NTczMDUzQHFxLmNvbQ==
Disclaimer: All claims expressed in this article are solely those of the authors and do not necessarily represent those of their affiliated organizations, or those of the publisher, the editors and the reviewers. Any product that may be evaluated in this article or claim that may be made by its manufacturer is not guaranteed or endorsed by the publisher.
Research integrity at Frontiers
Learn more about the work of our research integrity team to safeguard the quality of each article we publish.