- 1Key Laboratory of Shale Gas and Geoengineering, Institute of Geology and Geophysics, Chinese Academy of Sciences, Beijing, China
- 2Institute of Earth Science, Chinese Academy of Sciences, Beijing, China
- 3University of Chinese Academy of Sciences, Beijing, China
- 4Institute of Earthquake Forecasting, China Earthquake Administration, Beijing, China
- 5Sino Petroleum Exploration and Production Research Institute, Beijing, China
- 6China University of Mining and Technology-Beijing, Beijing, China
Large areas of Yanshan period granites with high heat production values (3–10 μW/m3) and mantle plume around Hainan province co-exist in Igneous Rocks Regions of South China (IRRSC). Surface manifestations are mainly warm/hot springs with temperatures below 90 °C and no typical phenomenon of high temperature resources have been observed. The main objective of this paper is to discuss the existence of high temperature geothermal resources and their possible locations under this kind of geothermal and tectonic background by analysis of high temperature heat sources, borehole temperature measurement, and reservoir temperature estimation. Two possible partial melts of the magma chamber were detected as high temperature heat sources in the Southern Leizhou Peninsular and North Hainan Island at a depth of 8–15 km. Other low resistivity zones in the upper crust are more likely caused by fluid in the formations or faults but not high temperature heat sources. This was also verified by borehole temperature measurement in these two areas, with maximum formation temperatures of 211°C and 185°C found, respectively. Reservoir temperatures from fluid geothermometers show lower temperatures of between 110–160°C for typical geothermal fields over the IRRSC but not in the Southern Leizhou Peninsular and Northern Hainan Island. In all, high temperature geothermal resources may be found in the Southern Leizhou Peninsular and on Northern Hainan Island.
Introduction
Geothermal energy is a renewable and clean energy that widely exists in China. Compared with wind, solar power, and other renewable energies, it is safe, abundant, and stable (Wang et al., 2020). The China Geothermal Energy Development Report (2018) states that by the end of 2020, the annual utilization of geothermal energy in China was 70 million tons of standard coal equivalent (SCE), accounting for 1.46% of the total primary energy consumption (Lin et al., 2013). Geothermal energy could play an important role in the transformation of China’s energy structure in the context of global climate change.
The Igneous Rock Region of South China (IRRSC) mainly refers to areas with a wide amount of granite in Fujian, Guangdong, and Hainan Province where the economy is highly developed and the population is large and there is an urgent demand for clean energy. Granite in IRRSC has heat production values ranging from 2 to 10 μW/m3 and most of them are 4–7 μW/m3 which is much higher than that of other areas (Zhao et al., 1995; Wan et al., 2015; Zhou et al., 2016). In addition, geophysical interpretations indicate that there are lots of low resistivity zones in the upper and middle crust and low velocity zones in the lower crust and upper mantle, described by some researchers as partially melted (Liao et al., 1988; Xiong et al., 1991) and as disturbed mantle from asthenosphere upwelling, respectively.
This evidence indicates that there may be high temperature geothermal resources in these locations. However, surface manifestations in IRRSC are mainly warm/hot springs with a temperature lower than 90°C. Typical phenomena including geysers, boiling springs, or the hydrothermal explosion of high temperature geothermal resources have not been observed. The temperature revealed by geothermal wells is below 150°C and most of the geothermal wells in IRRSC are shallower than 1 km. In the past 5 years, several wells with a depth of 3–5 km have been drilled by the China Geological Survey (CGS), the details of which are listed in Table 1. Geothermal temperature readings revealed by an exploration well in Huizhou of Guangdong province and Zhangzhou in Fujian province were all below 150°C. The reported reading of 185°C from the exploration well of Hainan, also reported by the company, could be questionable because the temperature measured from adjacent wells is all around 150°C. However, petroleum exploration suggests a higher temperature of 211°C in the Xuwen and Guangdong area (Table 1). Fluid geothermometers are also good methods to evaluate reservoir temperature when there are no boreholes. Research on reservoir temperature prediction using geochemical methods shows that it will not exceed 150°C for the geothermal system in Fujian province while that of Guangdong and Hainan province range from 100 to 154°C (Pang, 1988; Li et al., 2013; Mao et al., 2020). In all, whether and where high temperature geothermal resources exist in IRRSC remains unknown.
This paper studies the existence and possible locations of high temperature geothermal resources in IRRSC from three aspects. Firstly, we discuss the possibility of a high temperature heat source based on geophysical interpretations and then analyze reservoir temperature using borehole temperature measurement. Finally, the reservoir temperature of typical geothermal fields is estimated by both borehole measurement and fluid geothermometers. These comprehensive analyses help examine whether there are high temperature geothermal resources and provide scientific evidence that could contribute to further development of geothermal resources in IRRSC.
Geological Settings of the Study Area
The study area of IRRSC is shown in (Figure 1A) (Yang, 2016). Tectonically, it is a part of the SouthE China Block (SCB), located at the southeastern margin of the Eurasian continent. Its northern margin is the Qinling-Dabie-Sulu collisional orogenic belt, which splices the SCB with the North China Block (NCB) (Figure 1A), while its southwestern margin is connected to the Indo-Chinese Block through the Red River Fault (Zhang et al., 2013). During the Mesozoic periods, a 1,300 km wide intra-land orogenic belt was formed (Liu et al., 2013). The area has been influenced by multiple phases of tectonic movements including the Caledonian, Indo-Chinese, and Yanshan phases, resulting in distributions of large areas of Mesozoic igneous rocks (Figure 1B). Typical igneous rocks include granite of the Triassic period of Indo-Chinese phase, granite and basalt of the Jurassic and Cretaceous period of the Yanshan phase, in which granite is the dominant rock type.
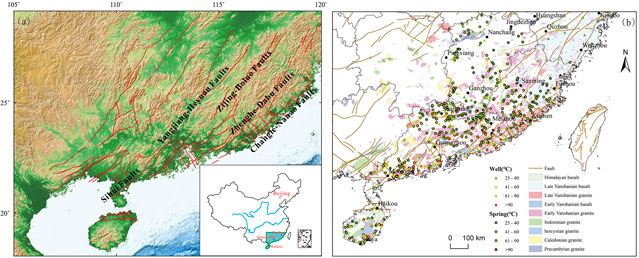
FIGURE 1. Location of the study area and main faults (A) and hot springs in the igneous rock areas (B).
The tectonics of SCB is mainly controlled by the NNE-oriented fault system that formed during the Yanshan phase. These faults are not only leftward sliding, but also have obvious extrusion characteristics and recoil pushing activities. Most of the southern section of the faults is deflected in the SW direction, and the accompanying faults are mainly NWW-oriented tensional and tensional-shear fault systems (Shu, 2012). There are six regional scale faults systems including the Jiangshan-Shaoxing (JS-SX), Sihui-Wuchuan (SH-WC), Yangjiang-Heyuan (YJ-HY), Zijin-Boluo (ZJ-BL) fault, Zhenghe-Dapu (ZH-DP), and Changle-Nanao (CL-NA) fault systems as shown in Figure 1A.
Previous investigations and studies show that geothermal resources in the region are abundant and widely distributed, mainly in the form of low-medium temperature hot springs. According to the statistics of CGS, there are a total of 553 hot springs and geothermal wells, and most of these geothermal waters are found in Guangdong Province 315) and Fujian Province (204) (Fujian Institute of Geological Survey, 2015; The Forth Geological Group of Geological Bureau of Guangdong Province, 2015; Hainan Institute of Geological Survey, 2016). Hot springs mainly spring at temperatures ranging from 40 to 60 °C which account for 47.9% of the total, while those ranging from 25 to 40°C and from 60 to 90°C account for 23.3 and 25.9%, respectively (Figure 1B). Geothermal resources with a temperature of 90–150°C are rare and only account for 2.9% of the total.
The main geothermal reservoirs can be divided into two types, including a fracture controlled banded reservoir and layered porous reservoir. Banded reservoirs are fractured zones of granite or metamorphic rocks while the layered reservoir is of sandstone.
Sampling and Data Collection
Sampling and Analysis
To study the reservoir temperature and the genesis models of geothermal systems, a total of 77 geothermal fluid samples were collected from November to December 2020 in typical geothermal fields in Guangdong province, including Maoming (MM), Xinzhou (XZ), Shenzao (SZ), Huizhou (HZ) and Fengshun (FS) geothermal field (Figure 1B). The pH, electrical conductivity (EC), alkalinity, temperature, and sulfide concentration measurement were measured for all the samples in the field. Samples for SiO2 analysis were diluted at a ratio of 1:4 to prevent SiO2 polymerization precipitation. Cations (Na+, K+, Mg2+, Ca2+) were acidified with ultra-pure nitric acid to make the pH below 2. Data for the geothermal fluid from Hainan province was collected from the geological survey report.
Borehole Temperature Measurement and Collection
Based on field measurement and data collection, a total of 19 T (Temperature)-D (Depth) curves and a number of borehole temperature measurement points were presented. The data points for the Southern Leizhou Peninsular and Northern Hainan Island were collected from the oil well and only refer to temperature measurements at a certain depth.
Borehole temperature measurement in Fujian province was mainly carried out by our institute and the Fujian Bureau of Geology and Minerals in 1992 (Fujian Institute of Geological Survey, 2015). The most recent temperature data of a 4 km deep well for HDR exploration was from the CGS (Lin et al., 2021). For Guangdong province, representative borehole temperature data were from exploration wells with a depth of about 1 km in XZ and 3 km in the HZ geothermal field, as well as the Southern Leizhou Peninsular from petroleum wells. Temperature measurement data in the Northern Hainan province were mostly referenced from petroleum boreholes in the Fushan depression and shallow wells of the Lingshui area. Data of the newly drilled HDR exploration well with depth more than 4 km by the HTAP Company was not analyzed because it was not available but showed on the T-P curves.
Interpretation Data Collection of Geophysical Exploration
In order to study whether there are high temperature heat sources like partial melt or magma chamber in the upper crust, data from several geophysical explorations, including Magnetotelluric (MT) and Artificial Blasting Earthquake (ABE) methods, were collected and analyzed. In addition, studies on the Hainan plume and its effects on the upper and medium crust were also analyzed.
Results and Discussions
Low Resistivity Zones in the Upper Crust and High Temperature Heat Source
Effects of the Hainan Plume
Geophysical exploration, geochemical characteristics, and petrological studies indicate that the Hainan plume exists. Evidence includes the fact that 1) the area 150–450 km beneath the IRRSC, which has strong heterogeneity laterally and low velocity anomaly, is only found around the Leiqiong volcano region (Hu et al., 2007; Zhao et al., 2012; Huang, 2014; Lv et al., 2017; Yang et al., 2021). 2) Cenozoic basalts in the Leiqiong area and basalt formed around 4–6 Ma and 1 Ma have Oceanic Island Basalts (OIB) characteristics, indicating it originated from a deep mantle with characters of MORB-OIB transitional zone (Castillo, 1998; Wang et al., 2012; Xu et al., 2012; Li et al., 2013; Yang, 2020). Based on alkaline rocks of the Cenozoic period at the surface, the influence scope of the Hainan plume is defined as the South China sea, Leqiong Peninsula, and Central South Peninsula, with an area of more than 400 km2 (Yan and Shi, 2007).
The plume extends from the top of the upper mantle to 410 km below the surface and is characterized by a low-velocity column with a diameter of about 160–200 km rising vertically that originated from the lower mantle (Huang, 2014; Wei and Chen, 2016; Wang, 2011; Chen, 2020). The low velocity anomalies at different depths have different locations, sizes, and geometries and are mainly located in the northeastern part of Hainan Island, covering the coastal area of Guangdong, the Leizhou Peninsula, and eastern and northeastern of Hainan Island. As shown in Figure 2, the low velocity anomaly at 300 km is mainly located in the eastern part of Hainan Island with complicated structural patterns, and from 300 to 260 km, the diameter gradually decreases. When the mantle plume reaches 220 km, the anomaly center shifts to the northeast of Hainan Island and upwells to the bottom of the lithosphere, and then starts to extend laterally (Chen, 2020). At depths of 50–90 km, there are large areas of low velocity which mainly exist in the Leizhou Peninsula and adjacent areas of the South Sea, the southeastern part of SCB, and the vicinity of the Pearl River Estuary.
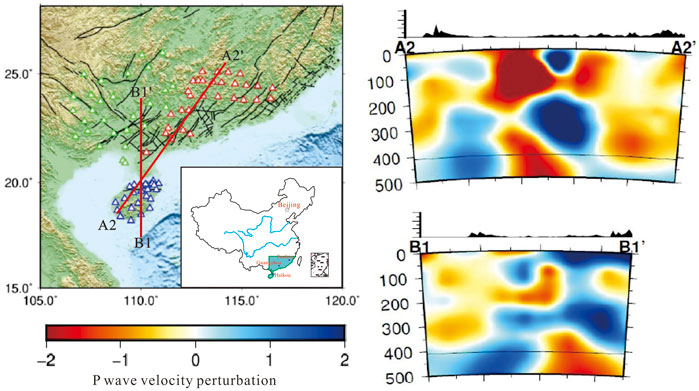
FIGURE 2. P wave velocity perturbation based on finite frequency tomography method. Red triangle-30 mobile station in Guangdong province, green triangle-27 stationary station in Guangxi province, blue triangle-27 stationary station in Guangxi province, A2-A2′ and B1-B1′represent NE-SW and N-S orientation sections (modified from Chen, 2020).
Meanwhile, low-velocity zones widely exist in the crust of coastal areas of the IRRSC. There are also three obvious low-velocity zones in the crust (about 12–20 km), which are consistent with an upper mantle low-velocity anomaly (Zhao et al., 2004). The plume provides energy for volcanic activity in Leizhou Peninsular and Northern Hainan Island, which reaches a peak in the early Pleistocene and is constrained by a NW-SE oriented fault system that provides conduits for magma ascent.
Thus, we conclude that the Hainan plume causes heat disturbance on the lower-middle crust and upper mantle in IRRSC but the question of whether a high temperature heat source could form under this environment requires further research.
Low Resistivity Zones in the Upper Crust and High Temperature Heat Source
The electrical structure of the lithosphere can be used to constrain heat sources such as partial melt or magma chamber. There are already many studies on the geophysical inversion of the structure of the upper-middle crust beneath IRRSC. This research found that the asthenosphere upwelling commonly exists in the NNE direction, resulting in small-scale discontinuous low velocity zones in the lower crust (20 km) and upper mantle (40 km) (Tian, 2014). Most of the small scale low resistivity zones in the crust are consistent with fault systems but not high temperature heat sources of melt.
The artificial explosion seismic profile in Fujian province shows that there is a stable low-velocity zone in the upper-middle crust (10–16 km) with a thickness of about 2.8 km and it is thought to be a partial melt. The Zhangzhou (ZZ) geothermal field with the highest reservoir temperature in the province is located just above this low-velocity zone. Therefore, it can be deduced that high temperature heat sources of partial melt exist in the ZZ geothermal field (Liao et al., 1988; Xiong et al., 1991). However, recent comprehensive studies indicate that the low velocity zone is more likely to be the slip surface of the Min-Tai spade fault and not partial melt, based on the evidence that 1) the volcanic rocks in this area developed in very small-scale and free of crustal mixing; 2) it is difficult for basaltic magma that rises rapidly to the surface adiabatically to stay inside the crust to form a magma chamber, which would be a heat source for geothermal activity; and 3) it is impossible that enough magma intrusion occurred 100 million years ago to maintain enough heat to generate a high-temperature geothermal system (Liao, 2012).
2D MT interpretations in the north of Guangdong province (Line 1, Figure 3) show that two large low resistivity zones exist at a depth of 50–150 km. They are thought to be channels for the subduction of mantle matter, resulting in a series of low resistivity layers in the crust and upper mantle. Recent 3D MT interpretation of the Meizhou-Shantou section (Line 2, Figure 3) shows that the low resistivity zones are mainly related to regional deep fault systems, rather than high-temperature heat sources of partial melt (Han, 2012; Cheng et al., 2021). 2D MT interpretations in the Huizhou (Line 3, Figure 3) and Yangjiang (Line 4, Figure 3) areas of Guangdong province indicate that low velocity zones exist at depths of 15–30 km and 35–60 km respectively. The intrusion of the asthenosphere occurs at the bottom of the crust, resulting in heat disturbance to the upper part (Lin et al., 2013). However, this kind of heat disturbance cannot provide enough heat to form partial melt or magma chamber in the crust.
In the Northern Hainan Island, based on 3D MT interpretations (Line 5, Figure 3), two N-S oriented linearly aligned low resistivity zone are found in the Fushan depression at depths of 4–10 km and >30 km (Liu et al., 2021). The shallower one is thought to have high salinity fluids or be magma volatile rather than a partial melt, while the deeper one is presumed to be partial melt in the crystal mushes. There is also a conduit between them that may provide a pass way for deep partial melt ascending to the shallower crust. Therefore, it can be concluded that partial melt may have existed, acting as a heat source in Northern Hainan Island.
Based on the above discussions, we conclude that there is probably no high-temperature heat source like partial melt in the upper-middle crust of IRRSC. However, there isE little geophysical data on the crust and mantle in tXW of the Leizhou Peninsula and Fushan Depression in northern Hainan and they are mostly influenced by the Hainan plume. Therefore, more studies are needed to identify whether there is a high temperature heat source like partial melt or a magma chamber in the upper crust, which could be the basis of the formation of high temperature geothermal resources.
Reservoir Temperature by Borehole Measurement
Reservoir Temperature Analysis for Fujian Province
Borehole temperature measurement is the most direct way to reveal the reservoir temperature and also can provide the most reliable evidence. T -D curves of representative geothermal wells in the Fujian area are shown in Figure 4 and the lithology and geothermal gradient information are listed in Table 2. Except for ZZ01 and ZZHDR01, geothermal systems in this area show obvious convection characteristics, reflecting the strong disruption by shallow groundwater activities. The temperature and geothermal gradient of the geothermal system can reach 80–120°C and more than 5 °C/100 m at a very shallow depth, respectively, but then stays at a constant value with a small gradient (Figure 4; Table 2). The ZZHDR01 well shows a conductive feature but the geothermal gradient is only 1.87 °C/100m, which is significantly lower than the global average value of 3°C/100 m. Therefore, we presume that there are no high temperature geothermal resources in Fujian province within the economical exploitation depth.
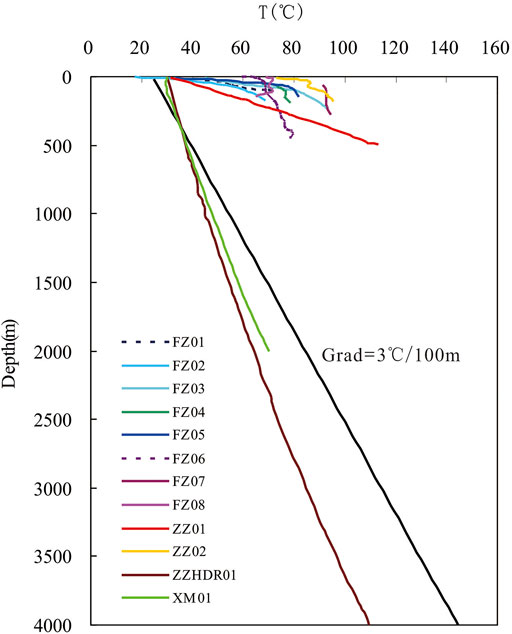
FIGURE 4. Representative borehole temperature measurement curves for Fujian province. FZ01-08 refer to boreholes from the Fuzhou area, ZZ01-02 refers to boreholes from the Zhangzhou area, ZZHSR01 refers to the hot dry rock exploration well by the China Geological Survey, XM01 refers to exploration well in the Xiamen area, the black line-geothermal gradient line with a global average value of 3°C/100 m
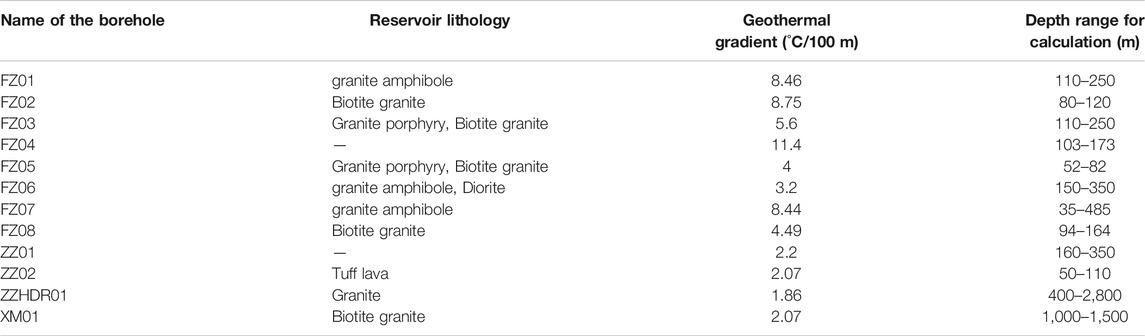
TABLE 2. Reservoir lithology and geothermal gradient of representative borehole temperature measurement curves (Fujian Institute of Geological Survey, 2015).
Reservoir Temperature Analysis for Guangdong Province
The T-P curves of Guangdong province are shown in Figure 5A. For the XZ geothermal field, the geothermal gradient is estimated to be 3.89–4.50°C/100 m based on the 1 km borehole temperature measurement, the lowest temperature of which is about 109°C. The reservoir lithology is medium-coarse grained biotite granite of the Yanshan phase, parts of which develop fracture zones for convection of geothermal fluid. The bottom temperature and geothermal gradient of the deepest HSD01 well that was drilled recently in the HZ area are 128°C and 3.5–4.0 °C/100 m respectively. Obvious convection properties also indicate that there may be no high temperature geothermal resources in the deep. Most of the geothermal gradient of Leizhou peninsular is 3.5–5.5°C/100 m and the maximum can reach 7.7°C/100 m. Existing temperature data from oil boreholes in the XW area of Southern Leizhou Peninsular show that the highest temperature measured is 211°C at a depth of more than 5.4 km (Table 1), and several data points indicate a temperature higher than 150°C at a depth deeper than 4 km. Therefore, we conclude that high temperature geothermal resources may exist in the deep reservoirs of the XW area of Southern Leizhou Peninsular.
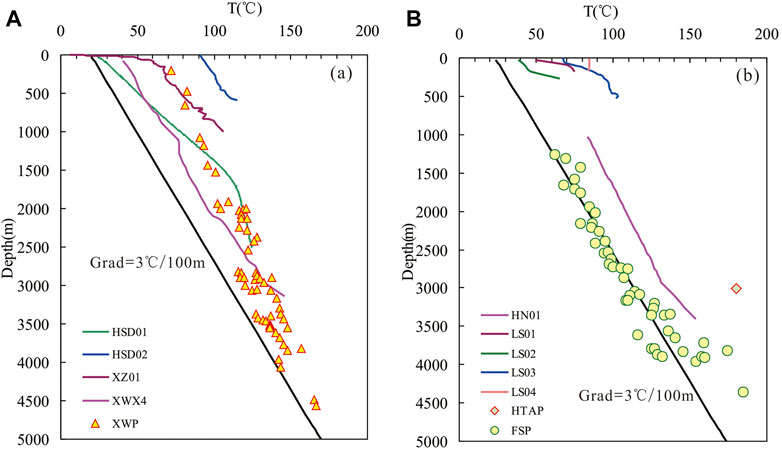
FIGURE 5. Representative boreholes temperature measurement curves for Guangdong (A) and Hainan province (B). HSD01, HSD02, and XZ01-scientific exploration wells for geothermal energy in Huangshadong and Xinzhou area, XW04- a well for oil exploration in XW area, XWP - bottom temperature of petroleum well measurement in Leizhou Peninsular, HN01–a well for oil exploration in Fushan depression, LS01-04-borehole from the Lingshui area of Hainan province, HTAP-the hot dry rock exploration well by HTAP Company, FSP- bottom temperature of petroleum well measurement in Fushan depression, the black line-geothermal gradient line with a global average value of 3°C/100 m
Reservoir Temperature Analysis for Hainan Province
The Hainan area can be divided into the sedimentary basin in the north and bedrock areas in the south from geological and geomorphological aspects. The sedimentary basin is dominated by conductive geothermal systems and geothermal anomalies are not obvious. Taking the Fushan depression as an example, temperature measurement from petroleum exploration shows a very good linear relationship and the geothermal gradient is about 3°C/100 m (Figure 5B). For many wells with a depth deeper than 4 km, the temperature reaches 150°C and the borehole drilled by the HTAP company reveals a temperature of 185°C at around 4.4 km. Therefore, we predict that it could be a potential site where high temperature geothermal resources can be found at depths greater than 4 km. However, the situation is different in the bedrock areas in the south. Taking the Lingshui area as an example, the temperature curve exhibits obvious convection properties and it is predicted that reservoir temperature will not exceed 100°C (Figure 4B).
Reservoir Temperature Estimation by Fluid Geothermometers
Geothermometers Selection
Fluid geothermometers are widely used to estimate reservoir temperature using geochemical data of hot springs and geothermal wells and can be divided into empirical and theoretical methods. Because geothermal fluids are widely occurring in igneous rock areas where faults or fractures are developed, they are susceptible to the effects of degassing and shallow groundwater mixing during ascent from the deep reservoir to the surface (Pang, 1988; Yuan, 2013; Wang, 2018; Mao et al., 2020). Therefore, the mixing model and multi-minerals equilibrium method are more applicable than other empirical geothermometers. The Na-K-Mg ternary diagram proposed by Giggenbach in 1988 can be used as a mixing model to estimate the reservoir temperature by extrapolating the mixing line to intersect with the full equilibrium line. Multi-mineral assemblage geothermometer refers to SI calculation based on chemical thermodynamic simulation under various temperatures. The temperature corresponding to the convergence point of multiple minerals is thought to be the reservoir temperature (Pang and Reed, 1998). This method can solve problems of degassing and mixing and has very good applications in estimating reservoir temperature (Fan, 2019; Malkemus et al., 2021).
Therefore, Na-K-Mg and multi-mineral assemblage geothermometers were chosen to calculate the reservoir temperature of the hydrothermal system.
Hydrogeochemical Properties of Geothermal Water From Typical Geothermal Fields
Concentrations of related ions and possible geochemical processes occurring from the reservoir to the surface are the basis of the application of fluid geothermometers (Xu et al., 2019a; Xu et al., 2019b). The geothermal water of the XZ, SZ, and MM geothermal fields are of Cl-Na and Cl-Na·Ca type and are affected by mixing with sea water, which has been verified by TDS (Table 3). On the other hand, the geothermal water of HZ and FS geothermal field are HCO3-Na type and different from that of XZ, SZ, and MM. Concentrations of Na, K, Mg, and SiO2 of geothermal fluid from XZ, SZ, and MM geothermal fields are in a narrow variability while that from HZ and FS is in a wide variety. The concentration of Na is high in the geothermal water from XZ, SZ, and MM geothermal fields and can reach 2,592 mg/L while the geothermal water of HZ and FS geothermal fields, reach much lower temperatures.
Reservoir Temperature Estimated by Na-K-Mg Geothermometer
The Na-K-Mg method is a cation geothermometer based on Na-K and K-Mg geothermometer and expressed in the form of a Na-K-Mg diagram. The diagram can also be used to evaluate the state of the geothermal fluid including fully equilibrium state, partially equilibrium or mixed state, and immature state. Based on the chemical data of samples, the Na-K-Mg diagram of geothermal fluids in the FS, HZ, MM, and XZ geothermal fields of Guangdong province were plotted as shown in Figure 6 (Arnorsson et al., 1998). The results show that for these geothermal systems, most of the samples are located in the partial equilibrated or mixing area and an obvious mixing trend can be found. The deep reservoir temperature is predicted to be about 110–120°C (Figure 6).
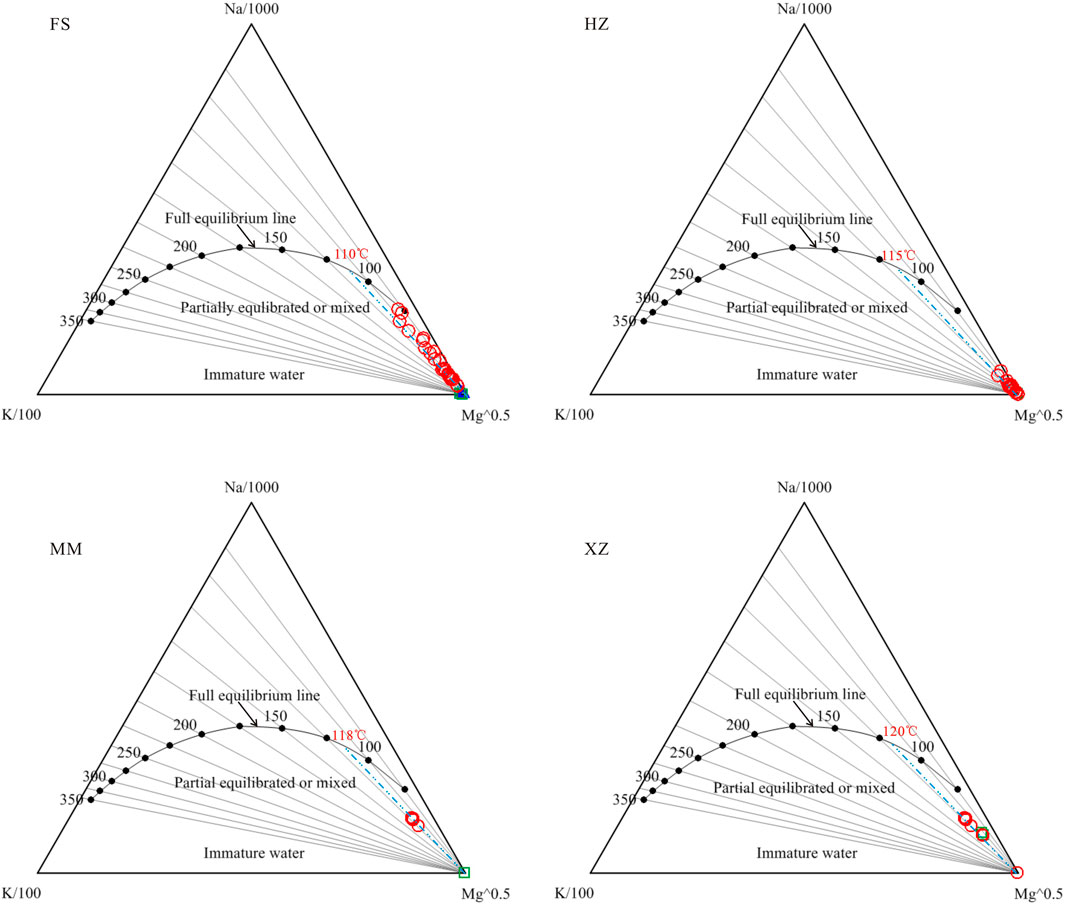
FIGURE 6. Na-K-Mg triangle diagrams for geothermal fluid from FS, HZ, MM, and XZ geothermal fields. Red circle-geothermal water, blue triangle-river water, green square-shallow groundwater.
Reservoir Temperature Estimated by Multi-Minerals Assemblage Method
The multi-mineral assemblage method has obvious advantages over empirical geothermometers because it relies on complete fluid compositions and a solid thermodynamic basis, rather than (semi-) empirical correlations, and thus in principle applies to any geochemical system (Palandri and Reed, 2001). This method consists of using full chemical analyses of fluid samples to compute the SI (log Q/K) of certain minerals over a range of temperatures (e.g., 25–300°C), and then the SI are plotted as a function of temperature and the converging point of clustering curves at any specific temperature is inferred to yield the deep reservoir temperature.
In this study, representative geothermal water from the FS, HZ, MM, and XZ geothermal fields of Guangdong province and the GX geothermal field of Hainan province were selected to estimate the reservoir temperature, shown in Table 4. The selected samples are all located in the partial equilibrated or mixed area in the Na-K-Mg diagram and have the highest SiO2 concentration or measured surface or wellhead temperature among the respective groups. Minerals including quartz, feldspar, microcline, calcite, laumonite, chalcedony, biotite, montmorillonite-Na, and montmorillonite-K were chosen when doing the SI calculation based on the mineral facies of typical granite over the IRRSC.

TABLE 4. Water chemistry of typical samples for FS, HZ, XZ, MM geothermal fields in Guangdong province and GX geothermal field in Hainan province.
The GEOT program developed by Spycher was used to do the calculation as correction of degassing and mixing/dilution can be performed (Spycher et al., 2014; Spycher et al., 2016).
Based on gas compositions and assumed mixing factor, the reservoir temperature of these geothermal fields are calculated and the results are shown in Figure 7. The results show that reservoir temperatures in the FS, HZ, XZ, MM, and GX geothermal fields are 140°C, 155°C, 160°C, 140°C and 150°C, respectively. Except for the FS and MM area, the reservoir temperature of all other areas reaches 150°C.
Discussions on Reservoir Temperature
In the above sections, the reservoir temperature of typical geothermal fields was assessed using Na-K-Mg and multi-mineral assemblage geothermometer and borehole temperature measurement. The temperature of geothermal fields in Fujian province is referred to in results from Pang (1988) and that in Hainan province is referred to from temperature from oil wells. All the temperature data is summarized in Table 5.
Reservoir temperature from Na-K-Mg ternary diagram is lower than that from multi-minerals assemblage geothermometer. The borehole temperature measurement refers to the temperature at the bottom of the well and existing values indicate there are high temperature geothermal resources in XW of Guangdong and north of Hainan province. Combined with evidence from geophysical exploration and interpretations, we conclude that high temperature geothermal resources may exist in the XW area of Southern Leizhou Peninsular in west Guangdong province and Northern Hainan province like Fushan depression.
Conclusion
This paper discusses whether there are high temperature geothermal resources in IRRSC based on a comprehensive understanding of the existence of high temperature heat sources and reservoir temperatures from borehole measurement and fluid geothermometers.
Geophysical interpretations of the upper and middle crust indicate that there is no high temperature heat source, such as partial melt in the upper crust for most areas of IRRSC but the Southern Leizhou Peninsula and Fushan Depression in Northern Hainan are probable exceptions. Reservoir temperature for typical geothermal systems in IRRSC range from 100°C to 160°C and the highest temperature is found in the HZ, XZ, and MM geothermal fields. Existing borehole temperature measurements indicate that there are no high temperature geothermal systems in Fujian province within the economical exploitation depth but in the XW area of the Leizhou Peninsula and Fushan Depression of Hainan Island within the depth of 4–6 km.
High temperature geothermal resources should exist in IRRSC and specifically, are probably found in the Southern Leizhou Peninsula and Northern Hainan Island. Further studies are required to identify the exact location and details of high temperature geothermal resources for future utilization.
Data Availability Statement
The raw data supporting the conclusions of this article will be made available by the authors, without undue reservation.
Author Contributions
YL proposes the idea and finishes the manuscript draft; JT carries out geothemrmal fluid sampling and gas analysis and provides good suggestions; YC finishes the MT data collection and interpretation of two sections and helps to understand the geophysical analysis about the upper crust; GJ carryies out borehole temperature measurement in Huizhou of Guangdong Province; YZ helps collecting the temperature data of petroleum wells in South of LP, KC helps draw most of the Figures in the paper and ZP provides some suggestions to improve the manuscript.
Funding
The study has been financially supported by the National Key R&D Program of China (Nos. 2019YFC0604901) which focuses on heat sources and genesis models of geothermal systems in igneous rock regions of South China and by the National Natural Science Foundation of China (Grant No. 42072328) which is dealing with reinjection problems during geothermal fluid exploitation.
Conflict of Interest
Author YZ is employed by Sino Petroleum Exploration and Production Research Institute.
The remaining authors declare that the research was conducted in the absence of any commercial or financial relationships that could be construed as a potential conflict of interest.
Publisher’s Note
All claims expressed in this article are solely those of the authors and do not necessarily represent those of their affiliated organizations, or those of the publisher, the editors and the reviewers. Any product that may be evaluated in this article, or claim that may be made by its manufacturer, is not guaranteed or endorsed by the publisher.
References
Arnorsson, S., Anderesdottir, A., Gunnarsson, I., and Stefansson, A. (1998). “New Calibration for the Quartz and Na/K Geothermometers-Valid in the Range 0-350°C,” in Proceeding of Geoscience Society of Iceland Annual Meeting, 42–43.
Castillo, P. (1998). The Dupal Anomaly as a Trace of the Upwelling Lower Mantle. Nature 336, 667–670.
Chen, Y. P. (2020). Hainan Mantle Plume from P Wave Finite Frequency Tomography. Dissertation of Master’s degree. Harbin: Harbin institute of Technology. (In Chinese with English abstract).
Cheng, Y., Han, B., Li, Y., Guo, J., and Hu, X. (2021). Lithospheric Electrical Structure beneath the Cathaysia Block in South China and its Tectonic Implications. Tectonophysics 814, 228981. doi:10.1016/j.tecto.2021.228981
Fan, Y. F. (2019). Study on Fluid Geochemistry of the High-Temperature Geothermal System of the Ganzi Basin, Western Sichuan Plateau. Dissertation of Doctor’s degree. Beijing: University of Chinese Academy of Sciences.
Fujian Institute of Geological Survey (2015). Report of Investigation, Evaluation and Regionalization for Geothermal Resources in Fujian Province.
Hainan Institute of Geological Survey (2016). Assessment and Design of Geothermal Resources in Hainan Provinces. (In Chinese).
Han, K. (2012). A Study on Crust/mantle Electrical Structure Characteristics and its Dynamic Background in southeastern of South China. Dissertation of Master’s degree. Beijing: China University of Geosciences. (In Chinese with English abstract).
Hu, J. C., Cheng, Y. Z., Bai, D. H., Wang, W. H., Wang, L. F., He, Z. H., et al. (2007). Magnetotelluric Surveying and Electrical Structure of the Deep Underground Part in Leiqiong Volcanic Area. South China J. Seismol. 27 (1), 1–7. (In Chinese with English abstract).
Huang, J. (2014). P- and S-Wave Tomography of the Hainan and Surrounding Regions: Insight into the Hainan Plume. Tectonophysics 633, 176–192. doi:10.1016/j.tecto.2014.07.007
Li, N., Yan, Q., Chen, Z., and Shi, X. (2013). Geochemistry and Petrogenesis of Quaternary Volcanism from the Islets in the Eastern Beibu Gulf: Evidence for Hainan Plume. Acta Oceanol. Sin. 32 (12), 40–49. doi:10.1007/s13131-013-0386-1
Liao, Q. L., Wang, Z. M., Wang, P. L., Yu, Z. K., Wu, N. Y., and Liu, B. C. (1988). Explosion Seismic Study of the Crustal Structure in Fuzhou-Quanzhou-Shantou Region. Acta Geophysica Sinica 31 (3), 270–280. (In Chinese with English abstract).
Liao, Z. J. (2012). Deep-Circulation Hydrothermal Systems without Magmatic Heat Source in Fujian Province. Geosciences 26 (1), 85–98. (In Chinese with English abstract).
Lin, W. J., Liu, Z. M., Wang, W. L., and Wang, G. L. (2013). The Assessment of Geothermal Resources Potential of China. Geol. China 40 (1), 312–321. (In Chinese with English abstract).
Lin, W. J., Wang, G. L., Shao, J. L., Gan, H. N., and Tan, X. F. (2021). Distribution and Exploration of Hot Dry Rock Resources in China: Progress and Inspiration. Acta Geol. Sin. 95, 1–10. (In Chinese with English abstract).
Liu, Q. Y., He, L. J., and Huang, F. (2013). Review of Mesozoic Geodynamics Research of South China. Prog. Geophys. 28 (2), 633–647. (In Chinese with English abstract).
Liu, Y., Du, J., Hu, Z., Hu, D., Luo, Y., and Chen, C. (2021). Crustal Magma Plumbing System beneath the Quaternary Volcanic Area (Northern Hainan Island, china) Revealed by Magnetotelluric Data. J. Volcanol. Geotherm. Res. 419 (1), 107362. doi:10.1016/j.jvolgeores.2021.107362
Lv, Z. Y., Qiu, X. L., Ma, X. J., and Fang, L. H. (2017). Teleseismic P-Wave Travel Time Tomography for the Leiqiong Volcano and Cathaysia Block. Chin. J. Geophys. 60 (12), 4569–4579. (In Chinese with English abstract).
Malkemus, D., Perkins, R. B., and Palmer, C. D. (2021). Geochemistry and Geothermometry of Breitenbush Hot Springs, Oregon, USA. Geothermics 95, 102134. doi:10.1016/j.geothermics.2021.102134
Mao, X. M., Zhu, D., Ndikubwimana, I., He, Y., and Shi, Z. (2020). The Mechanism of High-Salinity thermal Groundwater in Xinzhou Geothermal Field, south china: Insight from Water Chemistry and Stable Isotopes. J. Hydrol. 593. doi:10.1016/j.jhydrol.2020.125889
Palandri, J. L., and Reed, M. H. (2001). Reconstruction of In Situ Composition of Sedimentary Formation Waters. Geochim. Cosmochim. Acta 65 (11), 1741–1767. doi:10.1016/s0016-7037(01)00555-5
Pang, Z.-H., and Reed, M. (1998). Theoretical Chemical Thermometry on Geothermal Waters: Problems and Methods. Geochim. Cosmochim. Acta 62, 1083–1091. doi:10.1016/s0016-7037(98)00037-4
Pang, Z. H. (1988). Zhangzhou Basin Geothermal System-Genesis Model, Energy Potential and the Occurrence of Thermal Water. Dissertation of Doctor’s Degree. Beijing. (In Chinese with English abstract).
Shu, L. S. (2012). An Analysis of Principal Features of Tectonic Evolution in South China Block. Geol. Bull. China 31 (7), 1035–1053. (In Chinese with English abstract).
Spycher, N., Peiffer, L., Sonnenthal, E. L., Saldi, G., Reed, M. H., and Kennedy, B. M. (2014). Integrated Multicomponent Solute Geothermometry. Geothermics 51, 113–123. doi:10.1016/j.geothermics.2013.10.012
Spycher, N., Stefan, F., and Patrick, D. (2016). “New Developments in Multicomponent Geothermometry,” in Proceedings, 41st Workshop on Geothermal Reservoir Engineering Stanford University, Stanford, California, February 22-24, 2016. SGP-TR-209.
The Forth Geological Group of Geological Bureau of Guangdong Province (2015). Investigation and Assessment Report of Geothermal Resources in Guangdong Province. (In Chinese).
Tian, S. B. (2014). The Study on the Deep Electrical Conductivity Structure of the Heat-Controlling Structure in Middle and Northern of Guangdong. Dissertation of Master’s degree. Wuhan: China University of Geosciences. (In Chinese with English abstract).
Wan, J. J., Sun, Z. X., Hu, B. Q., Wang, A. D., Liu, J. H., Liu, C. D., et al. (2015). Radiogenic Geochemistry Investigation on Granitic Rocks from Fogang Complex, Northern Guangdong Province and its Implications for Hot Dry Rock Resource. J. East China Inst. Techn. (Nat. Sci.) 38 (4), 398–406.
Wang, C. Y. (2011). Mantle Transition Zone Structure Around Hainan by Receiver Function Analysis. Dissertation of master’s degree. Beijing: Institute of Earthquake Forecasting, China Earthquake Administration. (In Chinese with English abstract).
Wang, J. Y., Pang, Z. H., Kong, Y. L., Cheng, Y. Z., and Luo, J. (2020). Status and Prospects of Geothermal Clean Heating Industry in China. Innovation in China 16 (3), 294–297. (In Chinese with English abstract).
Wang, X.-C., Li, Z.-X., Li, X.-H., Li, J., Liu, Y., Long, W.-G., et al. (2012). Temperature, Pressure, and Composition of the Mantle Source Region of Late Cenozoic Basalts in Hainan Island, Se Asia: a Consequence of a Young thermal Mantle Plume Close to Subduction Zones? J. Petrol. 53 (1), 177–233. doi:10.1093/petrology/egr061
Wang, X. (2018). Formation Conditions and Hydrogeochemical Characteristics of the Geothermal Water in Typical Coastal Geothermal Field with Deep Faults, Guangdong Province. Dissertation of Doctor’s degree. Wuhan: China University of Geosciences. (In Chinese with English abstract).
Wei, S. S., and Chen, Y. J. (2016). Seismic Evidence of the Hainan Mantle Plume by Receiver Function Analysis in Southern China. Geophys. Res. Lett. 43, 8978–8985. doi:10.1002/2016gl069513
Xiong, S. B., Jin, D. M., Sun, K. Z., Zou, Y. S., Fan, X. B., and Du, X. G. (1991). Some Characteristics of Deep Structure of the Zhangzhou Geothermal Field and its Neighborhood in the Fujian Province. Acta Geophys. Sin. 34 (1), 53–65. (In Chinese with English abstract).
Xu, P. P., Li, M. N., Qian, H., Zhang, Q. Y., Liu, F. X., and Hou, K. (2019a). Hydrochemistry and Geothermometry of Geothermal Water in the Central Guanzhong basin, china: a Case Study in Xi'an. Environ. Earth ences 78 (3), 87. doi:10.1007/s12665-019-8099-1
Xu, P. P., Zhang, Q. Y., Qian, H., Li, M. N., and Hou, K. (2019b). Characterization of Geothermal Water in the piedmont Region of Qinling Mountains and Lantian-Bahe Group in Guanzhong Basin, China. Environ. Earth Sci. 78 (15), 442. doi:10.1007/s12665-019-8418-6
Xu, Y. G., Wei, J. X., Qiu, H. N., Zhang, H. H., and Huang, X. L. (2012). Opening and Evolution of the South China Sea Constrained by Studies on Volcanic Rocks: Preliminary Results and a Research Design. Chin. Sci. Bull. 57, 1863–1878. doi:10.1007/s11434-011-4921-1
Yan, Q. S., and Shi, X. F. (2007). Hainan Mantle Plume and the Formation and Evolution of the South China Sea. Geolog. J. China Univer. 13 (2), 311–322. (In Chinese with English abstract).
Yang, L. Z. (2016). The Radioactive Heat-Producing Characteristics and the Hot Dry Rock Resources Potential of Prototypes Granite Rocks in Zhangzhou Region. Dissertation of Master’s degree. East China University of Technology. (In Chinese with English abstract).
Yang, W. J. (2020). Mantle Sources and Magma Genesis of Late Cenozoic Basalts from Weizhou Island, Guangxi, China. Dissertation of Master’s degree. Beijing: Institute of Geology, China Earthquake Administration.
Yang, X., Yao, H., and Huang, B.-S. (2021). Crustal Footprint of Mantle Upwelling and Plate Amalgamation Revealed by Ambient Noise Tomography in Northern Vietnam and the Northern South China Sea. J. Geophys. Res. Solid Earth 126, e2020JB020593. doi:10.1029/2020jb020593
Yuan, J. F. (2013). Hydrogeochemistry of the Geothermal System in Coastal Areas of Guangdong Province, South China. Dissertation of Doctor’s degree. Wuhan: China University of Geosciences. (In Chinese with English abstract).
Zhang, G., Guo, A., Wang, Y., Li, S., Dong, Y., Liu, S., et al. (2013). Tectonics of South China Continent and its Implications. Sci. China Earth Sci. 56, 1804–1828. (In Chinese with English abstract). doi:10.1007/s11430-013-4679-1
Zhao, L., Allen, R. M., Zheng, T. Y., and Zhu, R. (2012). High-resolution Body Wave Tomography Models of the Upper Mantle beneath Eastern china and the Adjacent Areas. Geochem. Geophys. Geosyst. 13 (6), Q06007. doi:10.1029/2012gc004119
Zhao, M. H., Qiu, X. L., Ye, C. M., Xia, Z. Y., Huang, C. L., Xie, J. B., et al. (2004). Analysis on Deep Crustal Structure along the Onshore-Offshore Seismic Profile across the Binhai (Littoral) Fault Zone in Northeastern South China Sea. Chin. J. Geophys. 47 (5), 845–852. (In Chinese with English abstract). doi:10.1002/cjg2.573
Zhao, P., Wang, J. Y., Wang, J. A., and Luo, D. G. (1995). Characteristics of Heat Production Distribution in SEChina. Acta Petrol. Sin. 11 (3), 292–305. (In Chinese with English abstract).
Keywords: high temperature geothermal resources, south China, igneous rock, reservoir temperature, hainan plume
Citation: Li Y, Tian J, Cheng Y, Jiang G, Zhang Y, Chen K and Pang Z (2021) Existence of High Temperature Geothermal Resources in the Igneous Rock Regions of South China. Front. Earth Sci. 9:728162. doi: 10.3389/feart.2021.728162
Received: 28 June 2021; Accepted: 12 October 2021;
Published: 03 November 2021.
Edited by:
Haibing Shao, Helmholtz Centre for Environmental Research (UFZ), GermanyReviewed by:
Zheng-An Wei, China University of Geosciences, ChinaPanpan Xu, Chang’an University, China
Copyright © 2021 Li, Tian, Cheng, Jiang, Zhang, Chen and Pang. This is an open-access article distributed under the terms of the Creative Commons Attribution License (CC BY). The use, distribution or reproduction in other forums is permitted, provided the original author(s) and the copyright owner(s) are credited and that the original publication in this journal is cited, in accordance with accepted academic practice. No use, distribution or reproduction is permitted which does not comply with these terms.
*Correspondence: Jiao Tian, dGlhbmppYW9AbWFpbC5pZ2djYXMuYWMuY24=