- 1Institute of Global Environmental Change, Xi’an Jiaotong University, Xi’an, China
- 2SKLLQG, Institute of Earth Environment, Chinese Academy of Sciences, Xi’an, China
- 3School of Land Engineering, Chang’an University, Xi’an, China
- 4Yunnan Key Laboratory of Earth System Science, Yunnan University, Kunming, China
- 5College of Grassland Science, Shanxi Agricultural University, Taigu, China
Because the shell substance of an ostracod is derived entirely from the water body where it lives, its chemical compositions are sensitive to aquatic environment and thus have been used to reconstruct past climatic and environmental changes. However, there is controversy about the controlling factors for the different compositions of ostracod shells from various water bodies. In this study, seasonal and interannual variations in daily flux and stable oxygen-carbon isotopic compositions (δ18O, δ13C) for two species of ostracod shells (Limnocythere inopinata and Eucypris mareotica) and their controlling factors are discussed, based on ostracod shell samples collected from a time-series sediment trap from July 2010 through September 2012 and from surface sediments in Lake Qinghai, which were correlated with the state-of-the-art sensing data of the lake water. The results show that the daily flux of L. inopinata shells is an order of magnitude higher than that of E. mareotica. The δ18O and δ13C of both L. inopinata and E. mareotica shells have distinctly interannual and seasonal variations, with species differences. Interannual differences of δ18O for the two species of ostracod shells directly reflect the systematic differences of the summer water temperature between 2010 and 2012. We propose that seasonal variations of both δ18O and δ13C for the two species are affected by the precipitation of authigenic carbonates in microenvironment induced by high water temperature in summers, highlighting their environmental implications in Lake Qinghai.
Introduction
As small, bivalved crustaceans in various water bodies, ostracod shells consist of low magnesium (Mg) calcite, which is derived almost entirely from the water body where they live, and are often well preserved in sediments. The chemical compositions of ostracod shells are, therefore, ideally used to trace limnological conditions and to reconstruct (paleo)environment, because they are sensitive to water chemistry (e.g., Chivas et al., 1985; Chivas et al., 1986; Holmes, 1996; Holmes et al., 1998; Boomer et al., 2003). In most cases, the stable oxygen and carbon isotopic compositions (δ18O, δ13C) of lacustrine ostracod shells are generally considered to reflect those of the lake water (Stuiver, 1970; Holmes, 1996; von Grafenstein et al., 1999; Jin et al., 2009; Caporaletti, 2011). However, in addition to the water chemistry, there are other factors controlling the variations in both δ18O and δ13C of lacustrine ostracod shells, such as water temperature, authigenic carbonate precipitation, and/or isotopic fractionation between the shell and water, particularly for δ13C (e.g., Mckenzie, 1985; Herczeg and Fairbanks, 1987; Heaton et al., 1995; von Grafenstein et al., 1999; Keatings et al., 2002; Decrouy et al., 2011; Devriendt et al., 2017). These factors make the current explanations about the controlling factors for the isotopic compositions of ostracod shells controversial and uncertain.
For Lake Qinghai, the largest lake located on the northeastern Tibetan Plateau, ostracod shells in its sediments have been used to reflect lake water conditions and, thus, Asian summer monsoon change. For example, Zhang et al. (1994) analyzed δ18O and Sr/Ca ratios of ostracod shells from Lake Qinghai and discussed changes in salinity, lake water level, and temperature for the past 12000 years. The following ostracod studies of Lake Qinghai showed that the δ18O ratios can reflect lake water level and, thus, the precipitation/evaporation (P/E) budget associated with the Asian summer monsoon system (Lister et al., 1991; Liu et al., 2007; An et al., 2012). Meanwhile, other studies have shown that the isotopic composition of ostracod shells in Lake Qinghai reflects summer bottom-water conditions, with species difference (Henderson et al., 2003; Liu et al., 2009), whereas their δ13C is affected by the δ13C of dissolved inorganic carbon (DIC) and can reflect lake water salinity change (Li et al., 2012; Li and Liu, 2014). Additionally, these isotopic analyses used samples of several ostracod valves from sediments, which might be comprised of shells moulting at different seasons and years, since sedimentation rate is averaged at 1.0 mm/yr in Lake Qinghai (Henderson et al., 2003). Being considered dramatic seasonal to interannual limnological change, it is essential for the understanding of seasonal variations within and between populations of ostracods and association with limnological processes (Decrouy et al., 2011). There is currently little information on the seasonal variations in δ18O and δ13C of lacustrine ostracod shells in Lake Qinghai.
In this study, we report the seasonal δ18O and δ13C of modern ostracod shells collected from a time-series sediment trap from July 2010 through September 2012 in Lake Qinghai. The limnological conditions controlling both seasonal δ18O and δ13C of modern ostracod shells are discussed, through correlation with the state-of-the-art sensing data of lake water.
Materials and Methods
As the largest lake with brackish water on the northeastern Tibetan Plateau (Figure 1A), Lake Qinghai (36°32′–37°15′N, 99°36′–100°47′E) is located within an intermontane depression at the tectonically active margin of the plateau. Today, Lake Qinghai, at an altitude of 3,194 m above sea level (a.s.l.), has a water volume of 71.6 km3 and a catchment area of about 29,660 km2. As a hydrologically closed lake, it is of particular interest due to its unique geographical/geological settings and dramatic seasonal to interannual limnological change. The lake lies in a transitional zone where climate is sensitive to the East Asian and Indian monsoons and the Westerly Jet Stream. Mean annual precipitation (1951–2000) is 357 mm, but evaporation is 3–4 times higher than precipitation (Li et al., 2007). Average annual air temperature (1951–2007) is ∼1.2°C.
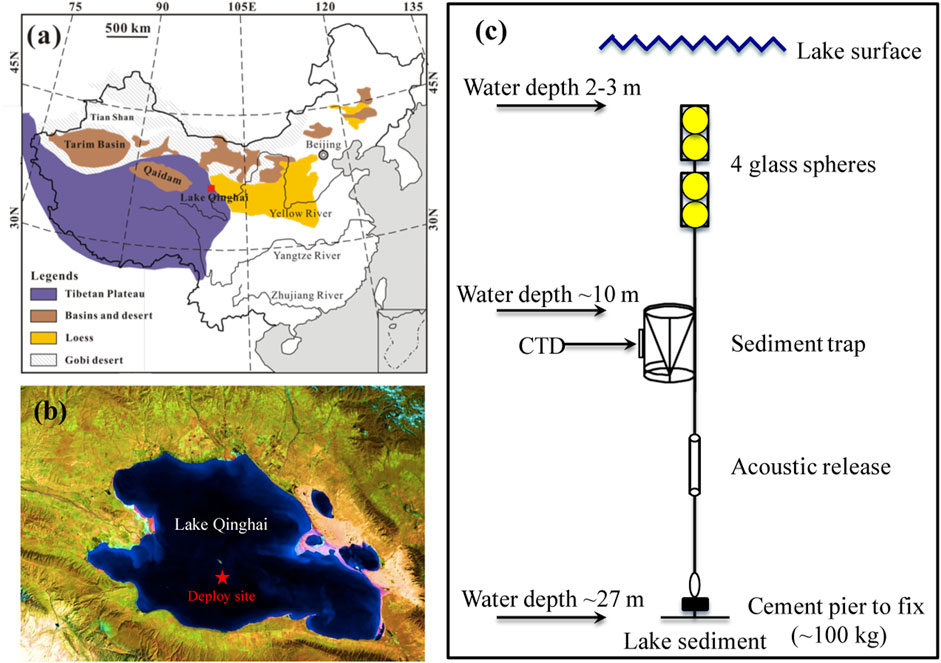
FIGURE 1. (A) Sketch map showing the location of Lake Qinghai (red square) and the surrounding region, including the Tibetan Plateau, the Chinese Loess Plateau, the Qaidam and Tarim Basins, and the Gobi desert. (B) The deployed (red solid star) site of the Mark 8–13 time-series sediment trap in Lake Qinghai. (C) Underwater mooring design of Mark 8–13 sediment trap in Lake Qinghai.
From July 13, 2010, to September 25, 2012, a McLane Mark 8–13 time-series sediment trap was deployed about 10 m under the lake water surface (Figure 1C) in the southern depocenter of Lake Qinghai (36°48′39.1″N, 100°8′12.0″E; Figure 1B). The Mark 8–13 sediment trap is a time-series instrument that uses 13 cup sample bottles to collect settling particles in situ. Due to the low sedimentation rate (∼1 mm/yr) and ice cover during winters, the sediment trap was recovered and deployed every 6 months, such that the collection time for each sample was 10–14 days. Meanwhile, an OS305 CTD (Conductance, Temperature, Depth) sensor was bound to the sediment trap to monitor lake water parameters every 30 min. These state-of-the-art sensing data recorded the limnological conditions. Owing to running out of battery, there lack sensing data between February 18 and June 10, 2011. In addition, 17 samples of the topmost 2 cm of sediment were collected from extensive sites within the lake.
In this study, a total of 45 trap sediment samples collected during July 2010 and September 2012 in Lake Qinghai were used to pick up ostracod shells. After removed lake water from the bottle, each sample was sieved using deionized water; >63 μm fraction was then dried on a petri dish; adult ostracod shells of both species (Limnocythere inopinata and Eucypris mareotica) were picked out from each sample using a fine paint-brush. The abundance of each species (valves/m2/d) was obtained using the number of adult ostracod shells, sediment weight, collection days, and surface area of the sediment trap (0.25 m2). Following the same procedure, adult ostracod shells of both species were picked out from 17 surface sediment samples.
Well-preserved adult ostracod shells were selected from the trap and surface sediment samples for stable isotopic analyses. Twenty to twenty five valves of L. inopinata and four to five valves of E. mareotica, respectively, were used for analyses of δ18O and δ13C. All specimens were then removed adhering detrital particles using a fine paint-brush, and allowed them to dry. Oxygen and carbon isotope analyses of ostracod shells were conducted using a Finigan MAT252 mass spectrometer with carbonate device (Kiel II). Meanwhile, carbonates in fine-grained fractions (<63 μm) of both trap and surface sediment samples were also analyzed for oxygen and carbon isotopic compositions. The <63 μm fraction was pretreated overnight with a 5% H2O2 solution to remove organic matter, and then reacted with anhydrous H3PO4 in vacuo overnight at a constant temperature of 25°C. The liberated CO2 was separated from water vapor and its stable isotope content measured also on a Finigan MAT252 mass spectrometer. All isotope results are reported in δ18O and δ13C notation per mil (‰) versus VPDB, based on calibration of the laboratory standards against NSB-19. Analytical reproducibility was better than ±0.10‰ (2σ) for δ18O and δ13C using both systems. All pretreatment and analyses were carried out at the State Key laboratory of Loess and Quaternary Geology (SKLLQG), Institute of Earth Environment, Chinese Academy of Sciences (IEECAS).
Meanwhile, the oxygen isotopic compositions of 13 lake water samples collected by the sediment trap during October 12 and September were analyzed using a Delta V IRMS mass spectrometer with Gas Bench II. For each analysis, 0.2 ml water samples were equilibrated for 18 h with instrument grade CO2 at 25°C. The equilibrated CO2 was measured for the oxygen isotopic compositions. The oxygen isotopic results are reported in δ18O notation permil (‰) deviations relative to the SMOW standard (Table 1). The analytical accuracy is better than 0.2‰. The oxygen isotopic analysis of water was carried out at the Institute of Tibetan Plateau Research, CAS.
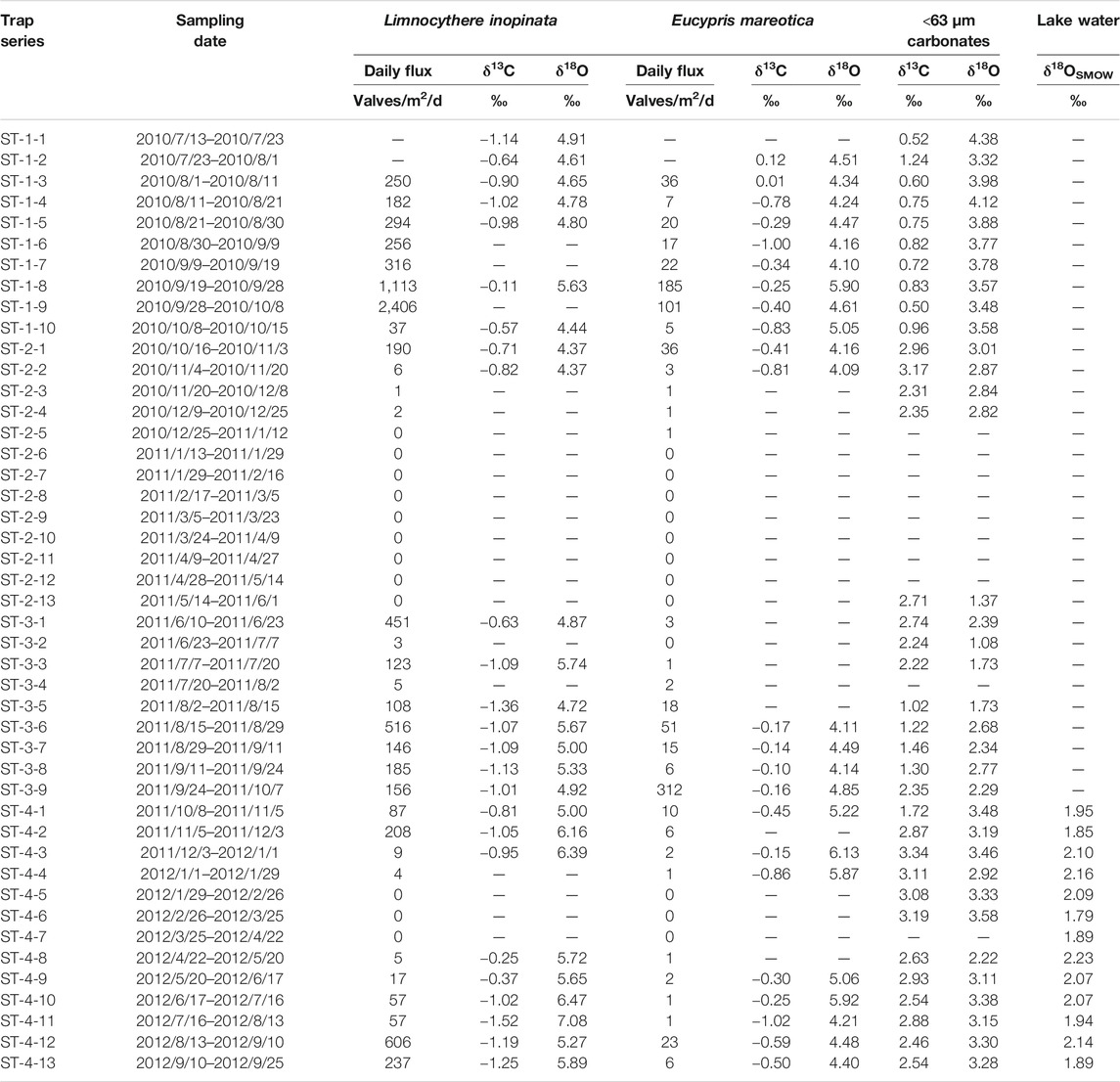
TABLE 1. Oxygen and carbon isotopic compositions for two species of adult ostracod shells (L. inopinata and E. mareotica) and carbonates of the <63 μm sediment fraction, and δ18O of lake water collected by a time-series sediment trap during July 2010 through September 2012 in Lake Qinghai.
Results
Limnological Conditions During 2010 and 2012
During the period of the sediment trap deployment (July 13, 2010, to September 25, 2012), Lake Qinghai experienced two entire limnological cycles as shown by lake water temperature, salinity, and level (Figures 2–4). The lake water temperature and salinity varied from −0.7°C to 15.4°C and from 12.08 to 13.16 PSU (Practical Salinity Units), respectively. Relative to the dry seasons, both parameters fluctuated largely during the summers, associated with monsoonal rainfall. For example, salinity had a 0.2 to 0.4 PSU change within one day. For the same period, water level in Lake Qinghai varied between 3,193.40 m and 3,194.53 m, with the highest water level occurring in summer 2012 (Figure 2). In general, salinity in the dry seasons was higher than that of the monsoonal seasons. As the lake water level increased during the monsoonal seasons, salinity tended to decrease. However, there was a negative relation between salinity and temperature (Figure 3), meaning that there was low salinity when temperature was high, even when the water level was low (Figure 2). The decreased salinity can be attributed to the removal of Mg2+ and Ca2+ by the precipitation of authigenic carbonates from the water column due to high temperature, because the Lake Qinghai water was supersaturated with respect to both calcite and dolomite (Jin et al., 2010; Jin et al., 2013a).
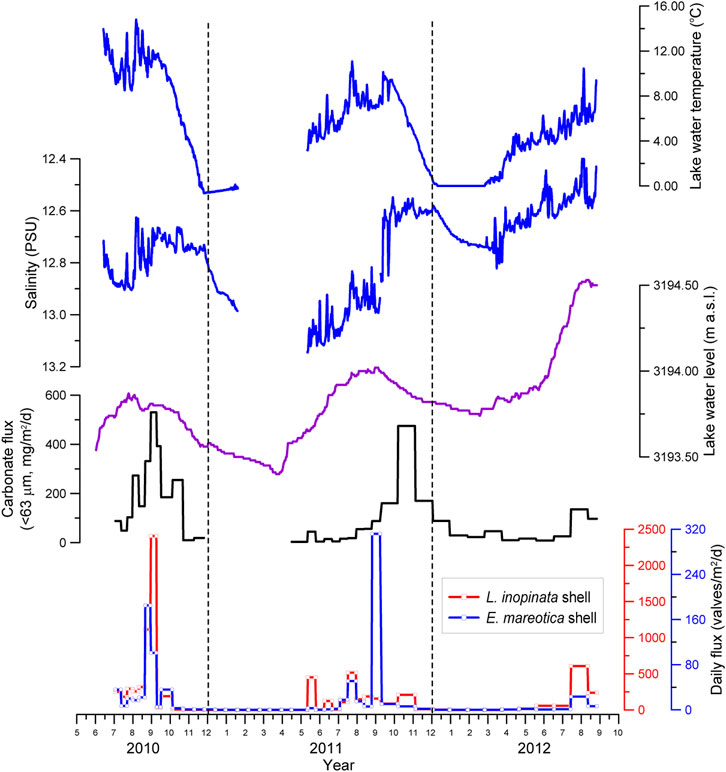
FIGURE 2. Variations in abundance for two species of adult ostracod shells (L. inopinata and E. mareotica) and carbonate flux from July 2010 to September 2012, along with water temperature, salinity, and water level of Lake Qinghai.
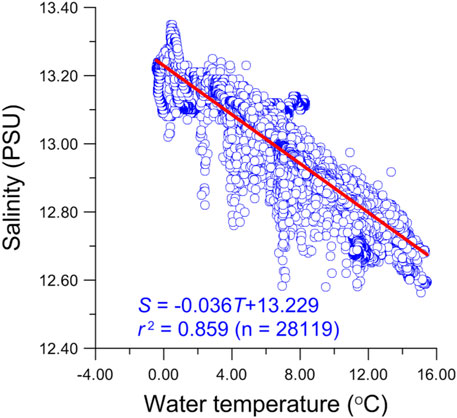
FIGURE 3. Linear relationship between water temperature and salinity in Lake Qinghai during July 2010 through September 2012.
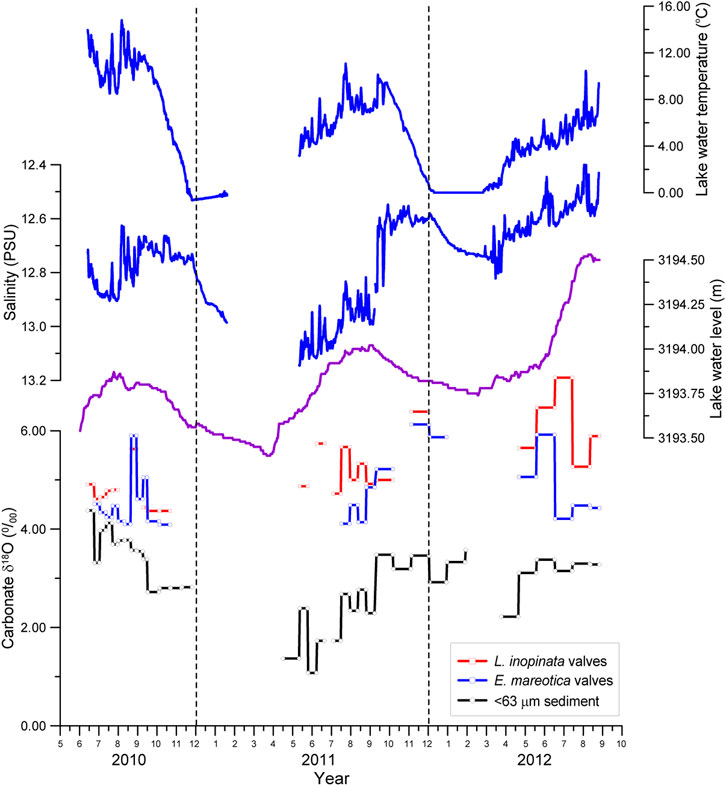
FIGURE 4. Seasonal and interannual variations in δ18O ratios for two species of adult ostracod shells (L. inopinata and E. mareotica) and carbonates of the <63 μm fraction in sediment from July 2010 to September 2012, along with water temperature, salinity, and water level of Lake Qinghai.
Seasonal Variation in the Daily Flux of Ostracod Shells in Lake Qinghai
There were two species of ostracods in the trap sediments collected during July 2010 and September 2012 in Lake Qinghai, i.e., L. inopinata and E. mareotica, both of which have been dominant in its lake sediments since the last glacial period (Lister et al., 1991; Zhang et al., 1994; Liu et al., 2007; An et al., 2012). In general, the daily flux of L. inopinata shell was an order of magnitude higher than that of E. mareotica, as listed in Table 1. There were dramatic seasonal variations in daily fluxes for both ostracods. High fluxes for both ostracods appeared during the monsoonal seasons, i.e., June through October (Figure 2). There were no or little shells found during winter and spring for both species. Flux peaks were 2,406 valves/m2/d for L. inopinata shells in late August 2010 and 312 valves/m2/d for E. mareotica in early September 2011 (Table 1).
Furthermore, L. inopinata appeared during late May and early June, earlier than E. mareotica, which secreted shells during late July, indicating that E. mareotica grew one and half months later than L. inopinata. Meanwhile, there was distinct interannual variation in both species. Daily fluxes of both ostracods in 2010 were higher than those in 2012, though the highest flux of E. mareotica appeared in early September 2011.
Ostracod δ18O and δ13C in Lake Qinghai
The values of δ18O and δ13C for both species of ostracod shells collected from Lake Qinghai during July 2010 through September 2012 are listed in Table 1. There were no data for some samples due to no or limited shells, mainly for those collected during winters and springs. The δ18O values varied from 4.37‰ to 7.08‰ (averaging 5.30‰) for the adult L. inopinata shells and from 4.09‰ to 6.13‰ (averaging 4.72‰) for the adult E. mareotica (Table 1). It is obvious that L. inopinata shells had higher δ18O values than those of E. mareotica. There was a systematic difference for δ18O of 0.56‰ between the two species, with similar seasonal and interannual variations (Figure 4). In general, the δ18O values of both ostracod shells in 2010 were lower than those in 2011 and 2102, with high δ18O values occurring during the middle summers.
Contrary to the δ18O values, the δ13C values of L. inopinata shells were generally 0.53‰ lower than those of E. mareotica (Figure 5). The δ13C values varied from −1.52‰ to −0.11‰ (averaging −0.91‰) for the adult L. inopinata shells and from −1.02‰ to 0.12‰ (averaging −0.42‰) for the adult E. mareotica (Table 1). Another interesting observation was that the δ13C values of E. mareotica shells showed a general decreasing trend for each year, so do that of L. inopinata shells in 2012 (Figure 5). There were little interannual δ13C variations for both ostracods between 2010 and 2012.
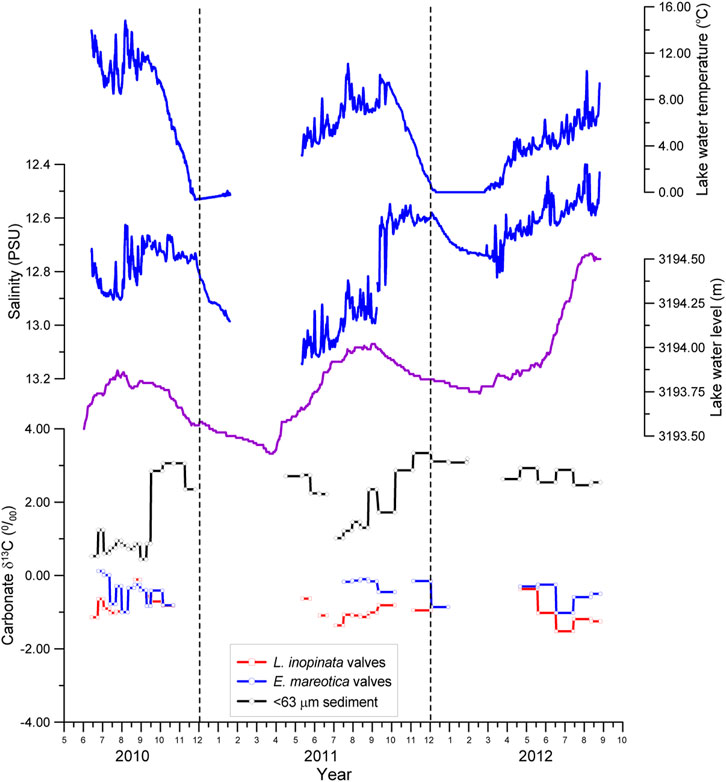
FIGURE 5. Seasonal and interannual variations in δ13C ratios for two species of adult ostracod shells (L. inopinata and E. mareotica) and carbonates of the <63 μm fraction in sediment from July 2010 to September 2012, along with water temperature, salinity, and water level of Lake Qinghai.
Relative to seasonal ostracod shells from the sediment trap, the ostracod δ18O and δ13C of both species from the surface sediment varied little, ranging from 3.64‰ to 4.79‰ for δ18O and −1.30‰ to −0.27‰ for δ13C of L. inopinata shells (Figure 6); corresponding values for E. mareotica shells were from 3.28‰ to 4.89‰ for δ18O and from −0.78 to 0.42‰ for δ13C, respectively (Table 2). Meanwhile, δ18O values of L. inopinata shells were slightly higher than those of E. mareotica, and the opposite was true for δ13C (Figure 6).
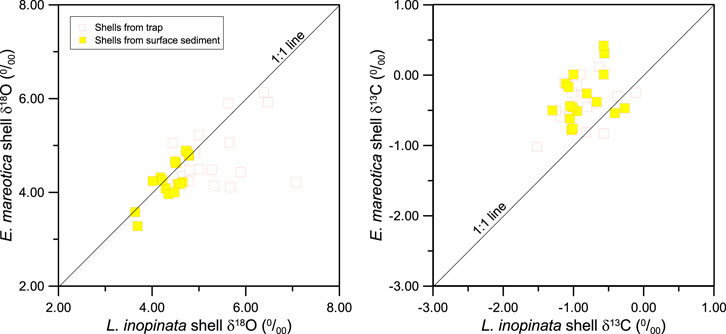
FIGURE 6. The correlations of (A) δ18O and (B) δ13C between two species of adult ostracod shells (L. inopinata and E. mareotica) from the sediment trap and surface sediment in Lake Qinghai.
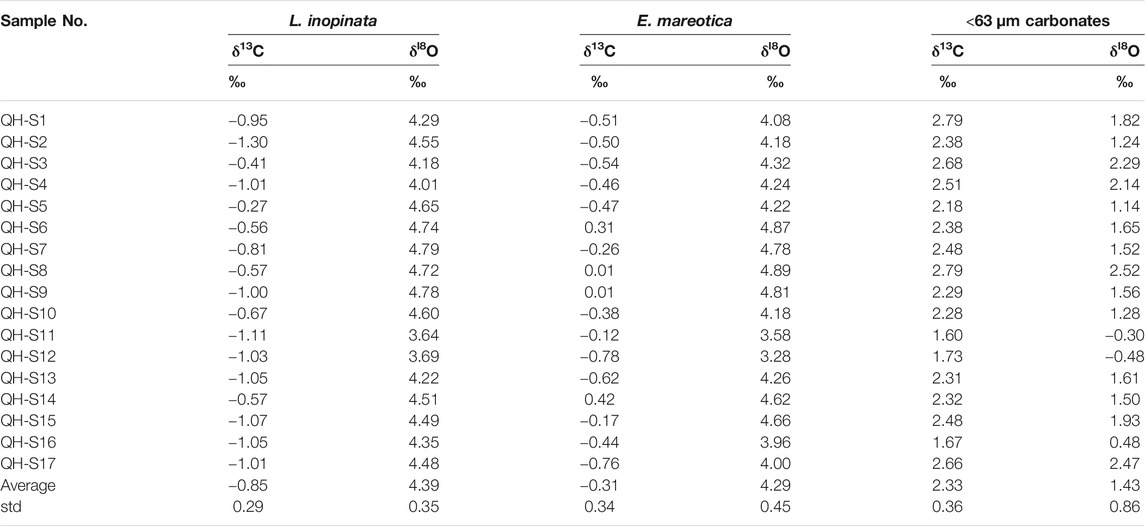
TABLE 2. Oxygen and carbon isotopic compositions of ostracod shells (L. inopinata and E. mareotica) and carbonate fraction (<63 μm) of surface sediments in Lake Qinghai.
The δ18O and δ13C of Fine-Grained Carbonates
The fine-grained carbonates in modern Lake Qinghai were essentially dominated by authigenic aragonite and low-Mg calcite (Henderson et al., 2003; Jin et al., 2013a). The δ18O values of authigenic carbonates in the <63 μm fractions of the seasonal trap sediments had a large range, varying from 1.08‰ to 4.12‰ with an average of 3.03‰ (Table 1). Meanwhile, the δ18O values of authigenic carbonates were all lower than those of both ostracod shells for the same seasons (Figure 3). Similar to the seasonal and interannual variation in the ostracod δ18O, the δ18O values of authigenic carbonates varied little and decreased from summer to winter in 2010, whereas in both 2011 and 2012 the carbonate δ18O varied largely but increased from spring to summer and then remained at high values (Figure 3).
The δ13C of fine-grained carbonates varied from 0.50‰ to 3.34‰, averaging 1.97‰, with higher values in the dry seasons than in the monsoon seasons. Contrary to the δ18O values, the carbonate δ13C values were nearly all higher than those of both ostracod shells (Figure 4).
Relative to those of the trap sediments, δ18O and δ13C of fine-grained carbonates of the surface sediments varied little, ranging from −0.48‰ to 2.52‰ (1.43 ± 0.86‰) for δ18O and from 1.60‰ to 2.79‰ (2.33 ± 0.36‰) for δ13C, respectively (Table 2). Similar to the trap sediments, the δ18O values of authigenic carbonates were lower, but the δ13C values were higher than those of both ostracod shells.
Discussion
The Relation Between Ostracod Flux and Limnological Conditions
The occurrence and abundance of ostracod species are affected by various limnological conditions, including the water body nature (such as its size, shape, turbidity, and substrate), water temperature, salinity, ionic composition, food supply, and predation (Holmes, 1996). For a given water body, the distribution and flux of an individual ostracod species are closely related to the seasonal changes of water temperature and salinity, so that the flux of ostracod shells is used to reflect the variation in water temperature and/or salinity (De Deckker and Forester, 1988; Holmes et al., 1998; Lu and An, 2010). In Lake Qinghai, when the water temperature reached 4°C in spring, the first flux peak of L. inopinata shells occurred, whereas the peak of E. mareotica shells occurred when the water temperature reached 6°C (Figure 2). Based on the growth periods before reaching maturity of the two species (1 month for L. inopinata and one and half months for E. mareotica), both ostracods began to reproduce during the dry seasons when the temperature was higher than 2°C; there was no ostracod shell growth below 2°C. This indicates that water temperature was the key controlling factor for ostracod growth, though there was a negative correlation between temperature and salinity (Figure 3). Indeed, both ostracod species lived in a wide range of salinity. L. inopinata can live in fresh water to the Baltic Sea, but it becomes a dominant species in water bodies with low to middle salinity (0.5–20.0‰) (Meisch, 2000; Wang et al., 2021). E. mareotica also has a wide tolerance limit to salinity, living in fresh to saline water bodies with salinity up to 325‰ (Li et al., 2010); it becomes a dominant species in water bodies with high salinity (18–40‰) and ultra-high salinity (>50 g/L) (Huang, 1984). Similarly, Zhang et al. (2006) investigated the distribution of ostracod species within the Lake Qinghai area and demonstrated that L. inopinata dominated in low salinity waters but E. mareotica in relatively high salinity waters. In fact, the salinity of modern Lake Qinghai varied between 12.6 and 13.1 PSU during the trap deployment period (Figure 2) and was suitable for both ostracods living. As a result, salinity is not the controlling factor for ostracod habitat in Lake Qinghai, even though its limnic salinity is more suitable for L. inopinata, which is the reason why the daily flux of L. inopinata shells is an order of magnitude higher than that of E. mareotica.
In seasonal variations, the daily fluxes of both species of ostracod shells co-varied with lake water temperature (Figure 2), indicating the direct control of water temperature on ostracod reproducibility in Lake Qinghai (Chivas et al., 1985; Holmes, 1996). Thus, high temperature favors ostracod reproducibility. Along with the decrease of summer water temperature from 2010 to 2012, daily fluxes of ostracod shells of both species also decreased (Figure 2). Interannual variation in daily fluxes of ostracod shells further confirms the control of water temperature on ostracod reproducibility in Lake Qinghai.
The Control of Seasonal Ostracod δ18O in Lake Qinghai
The δ18O of lacustrine ostracod shells is controlled by water temperature and, more importantly in closed lakes, the isotope composition of the water at the time of carbonate precipitation. When lake water temperature increased, the δ18O of ostracod shells decreased, with a positive correlation with lake water δ18O (e.g., Heaton et al., 1995; Xia, 1996; Xia et al., 1997). The 2–3°C difference of summer water temperature between 2010 and 2011 resulted in the observed ostracod δ18O difference (0.45‰) exactly (Figure 4), assuming that temperature was the a single controlling factor alone, following a gradient change of 0.24‰ per 1°C for ostracod δ18O (O’Neil et al., 1969). Consequently, the interannual variation in ostracod δ18O in Lake Qinghai can be regarded as the direct response to lake water temperature, i.e., lower ostracod δ18O values for the two species during the warmer summer in 2010 than those in 2011 and 2012. There was a synchronous seasonal variation between ostracod δ18O and water temperature (Figure 4). In particular in 2010 and 2012, the highest ostracod δ18O values of both species appeared during the periods with high temperature. As a result, the seasonal variation of ostracod shells in Lake Qinghai was not a function of water temperature, rather than of the isotope composition of lake water.
The isotopic composition of lake water is controlled by the P/E ratio and, to a lesser extent, by the δ18O and the amount of rain, and local hydrological conditions (Lister et al., 1991; von Grafenstein et al., 1999; Leng and Marshall, 2004). Then, which factor dominates seasonal variation in the water δ18O of Lake Qinghai? First, there was a large range of rain δ18O, ranging between −18.6‰ and +0.6‰ in 2008, for example (Jin et al., 2013b). These values were lower than the δ18OSMOW of modern lake water. As a result, increasing water level in the past decade decreased the δ18O of lake water from 3.42 ± 0.25‰ in 2006 (Liu et al., 2009) to 2.01 ± 0.14‰ in 2012 (Table 1) in Lake Qinghai. The increased lake level in Lake Qinghai since 2005 was considered to be a result of increasing annual rainfall and/or changing rainfall pattern, rather than temperature and evaporation (Jin et al., 2013b; Fan et al., 2021). However, the lake water δ18OSMOW vary seasonally little (Table 1), so that the impact of annual rainfall (∼1.25 km3/yr) is minor for this huge lake with a water volume of 71.6 km3. Second, the δ18O values of river water fluctuated around −7.0‰ in the Buha River, the largest river within the Lake Qinghai catchment, with more than half of the annual runoff into the lake (Jin et al., 2013b), which excludes the impact of other water sources, such as glacial melt water (Zhang et al., 1988). On the contrary, the water loss due to strong evaporation under high temperature can lead to enriched-18O in lake water in summer. Therefore, the δ18O of lake water is controlled mainly by evaporation, i.e., the parameter E in the P/E ratio over annual and decadal timescales.
Usually, the δ18O of carbonate is mainly controlled by the δ18O and temperature of water body where and when the carbonate is precipitated. For a hydrological-closed system, low P/E ratios (high E) in summer would make δ18O positive for both lake water and ostracod shells in Lake Qinghai. However, the ostracod δ18O of both species tended to decrease during the peak summers, though there were some high ostracod δ18O values (Figure 4). In Lake Qinghai, the seasonal variation in ostraocd δ18O was approximately over 2.5‰ (Table 1), much larger than that of the lake water δ18O based on our monitoring water δ18O data from April to September in 2012 (Table 1). It indicates that variation in lake water δ18O is not the main factor for seasonal change of ostracod δ18O in Lake Qinghai. In fact, the ostraocd δ18O value should decrease with increasing water temperature when its host water δ18O is stable. Thus, the water temperature does also not control seasonal ostracod δ18O changes in Lake Qinghai.
Considering that the fine particles collected by the sediment traps were dominated by authigenic aragonite, we compared the δ18O values of ostracod shells with those of carbonates in the <63 μm fraction from the same traps. The results showed that they had a similar variation pattern, but the δ18O values of the carbonates (3.03 ± 0.77‰) were higher than that of the lake water (2.01 ± 0.14‰). The precipitation of authigenic and biogenic carbonates, both with high δ18O, would result in low δ18O lake water, as demonstrated by the δ18O–δ13C relationship between ostracod shells and carbonates (Figure 7). Indeed, the salinity also tended to decrease during the peak summers, as a direct result of the removal of Mg2+ and Ca2+ from the lake water column due to the precipitation of authigenic carbonates (Jin et al., 2013a). We therefore propose that the seasonal variation in ostracod δ18O was a result of decreasing lake water δ18O in microenvironment induced by the precipitation of authigenic carbonates. A similar δ18O variation of ostracod shells and carbonates was also observed in recent sediments (Henderson et al., 2003). These results showed a synchronous δ18O change between the ostracod shells and authigenic carbonates (<80 μm fraction of lake sediments), though carbonate δ18O values were always lower than those of ostracod shells for the same samples (Henderson et al., 2003). These observations further indicate the control of the precipitation of authigenic carbonates on δ18O of lake water and ostracod shells.
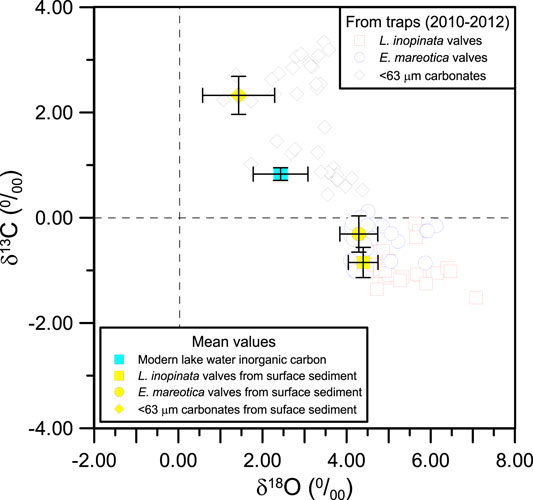
FIGURE 7. The relationship of δ18O and δ13C among total inorganic carbon in lake water, adult ostracod shells (L. inopinata and E. mareotica), and <63 μm carbonates from the sediment trap and surface sediment in Lake Qinghai. The data of the core QING 6 are cited from Henderson et al. (2003).
The Control of Seasonal Ostracod δ13C in Lake Qinghai
The carbon of lacustrine carbonates (including ostracod shells) mainly comes from DIC in the host water, which in turn is controlled by the catchment and within-lake processes. The sources of DIC are complex and affect its δ13C, including bedrock and soil carbon as catchment sources, the long-term balance of photosynthesis and organic decay within the lake (Mckenzie, 1985; Herczeg and Fairbanks, 1987; Kelts and Talbot, 1990; Cole et al., 1994). As a result, there were complex relationships between ostracod δ13C and water conditions. The variation ranges in δ13C of both ostracod shells and authigenic carbonates were smaller than those of δ18O, without obvious correlations with either water temperature or salinity (Figure 5). Previous study showed that the temperature effect of δ13C is weak for carbonates (Li et al, 2010), further supporting the complex relationships between water temperature and δ13C of both ostracod species in Lake Qinghai. Indeed, the seasonal δ13C change in authigenic carbonates was much larger than those of the ostracod shells.
In addition, opposite of δ18O, the ostracod δ13C values varied little, with slightly lighter δ13C of L. inopinata shells than those of E. mareotica (Figure 6). We propose that the systematic vital effects of δ18O and δ13C between ostracod species at the same seasons can be attributed to their living habitats. For example, photosynthesis of algae would result in different micro-environmental δ18O and δ13C values (Holmes, 1996; Li and Yu, 2001). L. inopinata prefers to creep on the benthos of the lake, while E. mareotica likes to swim and cling to algae (Lu and An, 2010; Li and Liu, 2014), which may result in slight differences of δ18O and δ13C values for the two ostracod species.
On the other hand, the precipitation of authigenic carbonates would control the δ13C of DIC and then of the ostracod shells as well, as demonstrated by the δ18O–δ13C relationship between ostracod shells and carbonates (Figure 7). It is also supported by the mirror variation in contemporaneous δ13C values for the <63 μm carbonates and ostracod shells from the traps (Figure 5). A similar variation was also observed in much higher δ13C values of the <80 μm carbonates than those of the ostracod shells in the past 300 years (Henderson et al., 2003). Therefore, we confirm that the seasonal variations in ostracod δ18O and δ13C is a result of decreasing δ18O but increasing δ13C in the lake water induced by the precipitation of authigenic carbonates. If so, chemical variations of ostracod shells in Lake Qinghai, particularly for δ18O, may provide an indication of water discharge and thus effective rainfall during monsoonal seasons (late May to September), because authigenic carbonate in Lake Qinghai sediments was largely controlled by solute fluxes into the lake by runoff within the catchment (Jin et al., 2010; Jin et al., 2015). Lake Qinghai water is supersaturated with respect to aragonite and calcite, where any available Ca2+ from rivers would be rapidly removed by the precipitation of authigenic carbonates, mainly during the summer months (Jin et al., 2010).
Conclusion
The seasonal samples collected by a time-series sediment trap provided insight into the controlling factors of interannual/seasonal variations in the flux and the chemical compositions of ostracod shells in Lake Qinghai on the northeastern corner of the Tibetan Plateau. Based on the daily flux, δ18O and δ13C of ostracod shell samples collected by a time-series sediment trap from southern basin of Lake Qinghai during July 2010 and September 2012, we found that both of L. inopinata and E. mareotica flourished during summer and early autumn, with a slight difference with growth periods and reproducibility between species. One of most important observations was that growth and flux of the two ostracod species were controlled directly by lake water temperature, which was correlated with the state-of-the-art sensing data of lake water. The interannual variation in ostracod δ18O mainly reflected the difference of lake water temperature, whereas their seasonal variation was controlled chiefly by the precipitation of authigenic carbonates induced by high water temperature, especially during the summer months. The precipitation of authigenic carbonates with higher δ18O than lake water resulted in low ostracod δ18O for both species. The factors affecting ostracod δ13C were complex, with a vital effect. Further information on the modern isotope systematics of the lake is needed to better understand the role of precipitation of authigenic carbonates on ostracod δ13C for different seasons.
Data Availability Statement
The original contributions presented in the study are included in the article, further inquiries can be directed to the corresponding author.
Author Contributions
ZJ and FZ designed and managed the project and measured isotopes. ZJ, FZ, and XL developed interpretations and wrote the manuscript with help from all authors. JW and CJ picked the shells and helped to measure isotopes and data interpretation. All authors commented on the manuscript.
Funding
This work was supported by the National Natural Science Foundation of China (No. 41773149) and the CAS Strategic Priority Research Program (No. XDB40020100).
Conflict of Interest
The authors declare that the research was conducted in the absence of any commercial or financial relationships that could be construed as a potential conflict of interest.
Publisher’s Note
All claims expressed in this article are solely those of the authors and do not necessarily represent those of their affiliated organizations, or those of the publisher, the editors and the reviewers. Any product that may be evaluated in this article, or claim that may be made by its manufacturer, is not guaranteed or endorsed by the publisher.
Acknowledgments
We are grateful to F. Lu for their expert instruction on ostracod taxonomy. Thanks are extended to D. Wan, X. Qiu, L. Zhou, X. Zhang, and D. Xu for their supports for sediment deployment and Y. Li and L. Chen for their help for shell picking and laboratory analyses.
References
An, Z., Colman, S. M., Zhou, W., Li, X., Brown, E. T., Jull, A. J. T., et al. (2012). Interplay Between the Westerlies and Asian Monsoon Recorded in Lake Qinghai Sediments since 32 Ka. Sci. Rep. 2, 619. doi:10.1038/srep00619
Boomer, I., Horne, D. J., and Slipper, I. J. (2003). The Use of Ostracods in Palaeoenvironmental Studies, or What Can You Do with an Ostracod Shell?. Paleontol. Soc. Pap. 9, 153–180. doi:10.1017/s1089332600002199
Caporaletti, M. (2011). Ostracods and Stable Isotopes: Proxies for Palaeoenvironmental Reconstructions. Joannea Geol. Palaontol. 11, 345–359.
Chivas, A. R., De Deckker, P., and Shelley, J. M. G. (1986). Magnesium and Strontium in Non-Marine Ostracod Shells as Indicators of Palaeosalinity and Palaeotemperature. Hydrobiologia. 143, 135–142. doi:10.1007/bf00026656
Chivas, A. R., De Deckker, P., and Shelley, J. M. G. (1985). Strontium Content of Ostracods Indicates Lacustrine Palaeosalinity. Nature 316, 251–253. doi:10.1038/316251a0
Cole, J. J., Caraco, N. F., Kling, G. W., and Kratz, T. K. (1994). Carbon Dioxide Supersaturation in the Surface Waters of Lakes. Science. 265, 1568–1570. doi:10.1126/science.265.5178.1568
De Deckker, P., and Forester, R. M. (1988). “The Use of Ostracodes to Reconstruct Continental Palaeoenvironmental Records,” in Ostracoda in the Earth Sciences. Editors P De Decker, JP Colin, and J P Peypouquet, 175–199.
Decrouy, L., Vennemann, T. W., and Ariztegui, D. (2011). Controls on Ostracod Valve Geochemistry: Part 2. Carbon and Oxygen Isotope Compositions. Geochim. Cosmochim. Acta 75, 7380–7399. doi:10.1016/j.gca.2011.09.008
Devriendt, L. S., McGregor, H. V., and Chivas, A. R. (2017). Ostracod Calcite Records the 18O/16O Ratio of the Bicarbonate and Carbonate Ions in Water. Geochim. Cosmochim. Acta. 214, 30–50. doi:10.1016/j.gca.2017.06.044
Fan, C., Song, C., Li, W., Liu, K., Cheng, J., Fu, C., et al. (2021). What Drives the Rapid Water-Level Recovery of the Largest lake (Qinghai Lake) of China over the Past Half century?. J. Hydrol. 593, 125921. doi:10.1016/j.jhydrol.2020.125921
Heaton, T. H. E., Holmes, J. A., and Bridgwater, N. D. (1995). Carbon and Oxygen Isotope Variations Among Lacustrine Ostracods: Implications for Palaeoclimatic Studies. The Holocene. 5, 428–434. doi:10.1177/095968369500500405
Henderson, A. C. G., Holmes, J. A., Zhang, J. W., Leng, M. J., and Carvalho, L. R. (2003). A Carbon- and Oxygen-Isotope Record of Recent Environmental Change from Qinghai Lake, NE Tibetan Plateau. Chin. Sci. Bull. 48 (14), 1463–1468. doi:10.1360/02wd0272
Herczeg, A. L., and Fairbanks, R. G. (1987). Anomalous Carbon Isotope Fractionation between Atmospheric CO2 and Dissolved Inorganic Carbon Induced by Intense Photosynthesis. Geochim. Cosmochim. Acta. 51 (4), 895–899. doi:10.1016/0016-7037(87)90102-5
Holmes, J. A., Fothergill, P. A., Street-Perrott, F. A., and Perrott, R. A. (1998). A High–Resolution Holocene Record From Sahel Zone of Northeastern Nigeria. J. Paleolimn. 20, 369–380. doi:10.1023/a:1007923304411
Holmes, J. (1996). Trace-element and Stable-Isotope Geochemistry of Non-Marine Ostracod Shells in Quaternary Palaeoenvironmental Reconstruction. J. Paleolimnol. 15 (3), 223–235. doi:10.1007/bf00213042
Huang, B. R. (1984). Quaternary Ostracoda From Qinghai Lake Region (In Chinese With English Abstract). Bull. Nanjing Ins Geol. Palaeont Chin. Acad. Sci. 7, 187–236.
Jin, Z., Zhang, F., Li, F., Chen, L., Xiao, J., and He, M. (2013a). Seasonal and Interannual Variations of the Lake Water Parameters and Particle Flux in Lake Qinghai: A Time-Series Sediment Trap Study (In Chinese With English Abstract). J. Earth Environ. 4 (3), 1306–1313. doi:10.7515/JEE201303002
Jin, Z., Zhang, F., Wang, H., Bai, A., and Qiu, X. (2013b). The Reason of Rising Water Level in Lake Qinghai since 2005 (In Chinese With English Abstract). J. Earth Environ. 4 (3), 1355–1362. doi:10.7515/JEE201303008
Jin, Z., An, Z., Yu, J., Li, F., and Zhang, F. (2015). Lake Qinghai Sediment Geochemistry Linked to Hydroclimate Variability Since the Last Glacial. Quat. Sci. Rev. 122, 63–73. doi:10.1016/j.quascirev.2015.05.015
Jin, Z., Bickle, M. J., Chapman, H. J., Yu, J., Wang, S., and Chen, S. (2009). Early to Mid-Pleistocene Ostracod δ18O and δ13C in the central Tibetan Plateau: Implication for Indian Monsoon Change. Palaeogeogr. Palaeoclimatol. Palaeoecol. 280, 406–414. doi:10.1016/j.palaeo.2009.06.028
Jin, Z., You, C.-F., Wang, Y., and Shi, Y. (2010). Hydrological and Solute Budgets of Lake Qinghai, the Largest lake on the Tibetan Plateau. Quat. Int. 218, 151–156. doi:10.1016/j.quaint.2009.11.024
Keatings, K. W., Heaton, T. H. E., and Holmes, J. A. (2002). Carbon and Oxygen Isotope Fractionation in Non-Marine Ostracods: Results from a ‘natural Culture' Environment. Geochim. Cosmochim. Acta. 66, 1701–1711. doi:10.1016/s0016-7037(01)00894-8
Kelts, K., and Talbot, M. (1990). “Lacustrine Carbonates as Geochemical Archives of Environmental Change and Biotic/Abiotic Interactions,” in Large Lakes: Ecological Structure and Function. Editors M Tilzer, and C Serruya (Madison: Science & Technology Publishers), 288–315. doi:10.1007/978-3-642-84077-7_15
Leng, M. J., and Marshall, J. M. (2004). Palaeoclimate Interpretation of Stable Isotope Data from lake Sediment Archives. Quat. Sci. Rev. 23 (7-8), 811–831. doi:10.1016/j.quascirev.2003.06.012
Li, J., and Yu, J. (2001). Lacustrine Ostracodes as Environmental Change Indicators: Application and Advance (In Chinese With English Abstract). J. Lake Sci. 13 (4), 367–375. doi:10.18307/20010413
Li, X.-Y., Xu, H.-Y., Sun, Y.-L., Zhang, D.-S., and Yang, Z.-P. (2007). Lake-Level Change and Water Balance Analysis at Lake Qinghai, West China During Recent Decades. Water Resour. Manage. 21, 1505–1516. doi:10.1007/s11269-006-9096-1
Li, X., and Liu, W. (2010). Carbon Isotope Fractionation in Ostracod Eucypris Mareotica From Culture Experiments and its Implication for Palaeoenvironment Reconstruction. Mar. Geol. Quat. Geol. 30 (3), 127–132. doi:10.3724/sp.j.1140.2010.03127
Li, X., Liu, W., Zhang, L., and Sun, Z. (2010). Distribution of Recent Ostracod Species in the Lake Qinghai area in Northwestern China and Its Ecological Significance. Ecol. Indicat. 10 (4), 880–890. doi:10.1016/j.ecolind.2010.01.012
Li, X., and Liu, W. (2014). Water Salinity and Productivity Recorded by Ostracod Assemblages and Their Carbon Isotopes since the Early Holocene at Lake Qinghai on the Northeastern Qinghai-Tibet Plateau, China. Palaeogeogr. Palaeoclimatol. Palaeoecol. 407, 25–33. doi:10.1016/j.palaeo.2014.04.017
Li, X., Liu, W., and Xu, L. (2012). Carbon Isotopes in Surface-Sediment Carbonates of Modern Lake Qinghai (Qinghai-Tibet Plateau): Implications for lake Evolution in Arid Areas. Chem. Geol. 300-301, 88–96. doi:10.1016/j.chemgeo.2012.01.010
Lister, G. S., Kelts, K., Chen, K. Z., Yu, J-Q., and Niessen, F. (1991). Lake Qinghai, China: Closed-basin lake Levels and the Oxygen Isotope Record for Ostracoda since the Latest Pleistocene. Palaeogeogr. Palaeoclim. Palaeoecol. 84 (1-4), 141–162. doi:10.1016/0031-0182(91)90041-o
Liu, W., Li, X., Zhang, L., An, Z., and Xu, L. (2009). Evaluation of Oxygen Isotopes in Carbonate as an Indicator of Lake Evolution in Arid Areas: The Modern Qinghai Lake, Qinghai-Tibet Plateau. Chem. Geol. 268 (1-2), 126–136. doi:10.1016/j.chemgeo.2009.08.004
Liu, X., Shen, J., Wang, S., Wang, Y., and Liu, W. (2007). Southwest Monsoon Changes Indicated by Oxygen Isotope of Ostracode Shells From Sediments in Qinghai Lake Since the Late Glacial. Chin. Sci. Bull. 52 (4), 539–544. doi:10.1007/s11434-007-0086-3
Lu, F., and An, Z. (2010). Climatic and Environmental Significance of Ostracod Abundance and Their Shell Oxygen Isotope From Lake Qinghai Surface Sediments (In Chinese With English Abstract). Mar. Geol. Quat. Geol. 30 (5), 119–128. doi:10.3724/SP.J.1140.2010.05119
Mckenzie, J. A. (1985). “Carbon Isotopes and Productivity in the Lacustrine and Marine Environment,” in Chemical Process in Lakes. Editor W Stumm (New York: Wiley), 99–118.
O’Neil, J. R., Clayton, R. N., and Mayeda, T. K. (1969). Oxygen Isotope Fractionation in Divalent Metal Carbonates. J. Chem. Phys. 51, 5547–5558. doi:10.1063/1.1671982
Stuiver, M. (1970). Oxygen and Carbon Isotope Ratios of Fresh-Water Carbonates as Climatic Indicators. J. Geophys. Res. 75, 5247–5257. doi:10.1029/jc075i027p05247
von Grafenstein, U., Erlernkeuser, H., and Trimborn, P. (1999). Oxygen and Carbon Isotopes in Modern Fresh-Water Ostracod Valves: Assessing Vital Offsets and Autecological Effects of Interest for Palaeoclimate Studies. Palaeogeogr. Palaeoclim. Palaeoecol. 148 (1-3), 133–152. doi:10.1016/s0031-0182(98)00180-1
Wang, C., Kuang, X., Wang, H., Guo, G., and Song, G. (2021). Ostracods as a Proxy for Paleoclimatic Change: An Essential Role of Bioculture Experiment Taking Limnocythere Inopinata (Crustacea: Ostracoda) as an Example. Ecol. Indicators. 121, 107000. doi:10.1016/j.ecolind.2020.107000
Xia, J. (1996). Sable-Isotope and Trace-Element Composition on Ostracode Shells and Their Application to Paleoclimatic Reconstruction (In Chinese With English Abstract). Quat. Sci. 4, 345–352.
Xia, J., Ito, E., and Engstrom, D. R. (1997). Geochemistry of Ostracode Calcite: Part 1. An Experimental Determination of Oxygen Isotope Fractionation. Geochim. Cosmochim. Acta. 61 (2), 377–382. doi:10.1016/s0016-7037(96)00351-1
Zhang, L., Sun, Z., An, Z., Liu, W., and Li, X. (2006). A Preliminary Distribution Analysis on Ostracoda of Different Water Bodies from Qinghai Lake Area, NW China (In Chinese With English Abstract). Acta Micropalaeont Sinica. 23 (4), 425–436. doi:10.3969/j.issn.1000-0674.2006.04.007
Zhang, P., Zhang, B., Qian, G., Li, H., and Xu, L. (1994). The Study of Paleoclimatic Parameter of Qinghai Lake since Holocene (In Chinese With English Abstract). Quat. Sci. 14 (3), 225–238.
Keywords: ostracod shell, abundance, stable O-C isotopes, seasonal variation, controlling factor, Lake Qinghai
Citation: Jin Z, Zhang F, Li X, Wang J and Jin C (2021) Seasonal/Interannual Variation and Controlling Factors for Oxygen and Carbon Isotopes of Ostracod Shells Collected From a Time-Series Sediment Trap in Lake Qinghai. Front. Earth Sci. 9:727330. doi: 10.3389/feart.2021.727330
Received: 18 June 2021; Accepted: 30 July 2021;
Published: 11 August 2021.
Edited by:
Fang-Zhen Teng, University of Washington, United StatesReviewed by:
Cheng Zhao, Nanjing University, ChinaQiuli Li, Institute of Geology and Geophysics (CAS), China
Copyright © 2021 Jin, Zhang, Li, Wang and Jin. This is an open-access article distributed under the terms of the Creative Commons Attribution License (CC BY). The use, distribution or reproduction in other forums is permitted, provided the original author(s) and the copyright owner(s) are credited and that the original publication in this journal is cited, in accordance with accepted academic practice. No use, distribution or reproduction is permitted which does not comply with these terms.
*Correspondence: Zhangdong Jin, zhdjin@ieecas.cn