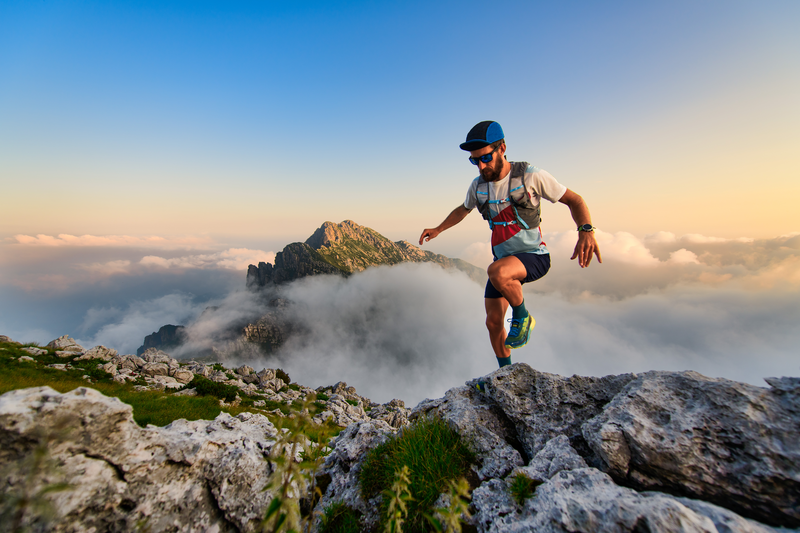
95% of researchers rate our articles as excellent or good
Learn more about the work of our research integrity team to safeguard the quality of each article we publish.
Find out more
REVIEW article
Front. Earth Sci. , 31 January 2022
Sec. Interdisciplinary Climate Studies
Volume 9 - 2021 | https://doi.org/10.3389/feart.2021.717420
This article is part of the Research Topic Late Quaternary Indo-Pacific Climate: Monsoons and Warm Currents View all 11 articles
A proper understanding of the paleoclimatic variability in the Southern Peninsular India demands a high-resolution paleoclimate reconstruction record. Hence, in this paper, we compiled all the available paleoclimate records from various locations across the Southern India that receives the southwest and the northeast monsoon. We delineated the southwest monsoon (SWMR) and Northeast monsoon (NEMR) variations within the Holocene Period based on the existing paleoclimatic record from this region. Paleoclimate records are primarily based on various paleoclimate archives and proxies. A detailed study of these variations unravels, (i) that SWMR gradually intensified since the younger dryas during the Early Holocene period. On the contrary, NEMR records indicate a dry phase during the same period. (ii) During the mid-late Holocene, precipitation in SWMR-dominated regions decreased, whereas precipitation in NEMR-dominated regions shows an increasing trend. (iii) Then, in the late Holocene Period, SWMR exhibits an overall wet phase with a few drier periods, and NEMR shows an intense dry period with shorter wet phases, which can be correlated to the demise of Chola dynasty, in the Southern India.
The tropical climate system in India is dominated by the Indian Summer Monsoon (ISM), which is characterised by regional irregularities in the general circulation of the atmosphere caused by land-sea contrasts and seasonal reversal in wind direction. The primary cause for monsoonal variation in the Indian subcontinent is differential heating of land and sea and/or manifestation of the seasonal migration of the intertropical convergence zone (ITCZ) (Charney, 1969). However, during the winter dry cold winds from Asia blow offshore (Colin et al., 1998). The interaction of these moisture regimes with various teleconnections (El Niño, La Nina, and IODM) have resulted in decadal to millennial-scale precipitation variations over India (Singhvi and Kale, 2010; Rajeevan et al., 2012).
The southwest or the summer monsoon (SWMR) and the northeast or winter monsoon (NEMR) are the two monsoons that bring copious amount of rain to South Asia. While the summer monsoon accounts for the majority of annual rainfall in India. Rainfall obtained during the northeast monsoon is also significant, particularly along the Coromandel Coast, South India, and Sri Lanka (Figure 1). During the summer monsoons withdrawal phase, lower-level winds in South Asia migrate from southwest to northeast (Rajeevan et al., 2012). This shift can be attributed to the southern trend of the tropical convergence zone. Hence, low-level winds over India switch their direction from southwesterly to northeasterly during the NEMR, and the region becomes a subset of the northeast trades, which is dry, persistent, and has a smaller vertical extent than the southwest monsoon. Hence, NEMR has a significant impact on agricultural practises in southern peninsular India (Rao Krishna and Jaganathan, 1953).
FIGURE 1. (A) Represents Large-scale topographical maps with dominant seasonal hemispherical airflows (after Mishra 2014, Resmi and Achyuthan, 2018). (B) Geographical location of the selected site.
On a regional and temporal scale, several studies have been attempted to reconstruct the Holocene paleoclimatic variability (Stubwasser et al., 2003; Trauth et al., 2003; Ponton et al., 2012). From the Indian subcontinent, various records viz. lacustrine (Rajagopalan et al., 1997; Juyal et al., 2004; Prasad et al., 2014; Sarkar et al., 2015; Rajaminkam et al., 2016), marine (Gupta et al., 2003; Thamban et al., 2007; Achyuthan et al., 2014), and fluvial records (Kale, et al., 2010; Resmi et al., 2016; Resmi and Achyuthan, 2018) have been used to reconstruct the paleomonsoon variability during the Holocene period.
Geographic distribution in the monsoon rainfall are of particular implication; for instance, the southern region of India which receives both SWMR and NEMR, perhaps, is the only region that experiences both the monsoons (Figures 1A,B, 2A,B). In the Holocene period, the southern peninsular India witnessed multiple cultural and adaptive innovations, transitions, as well as the rise of several civilizations (Figure 2C; Gupta et al., 2003; Walker et al., 2012). These ancient civilizations developed and disappeared in tandem with climate shifts, the monsoons have caused a significant socioeconomic ramification.
FIGURE 2. (A) Maps showing the monsoon precipitation during (A) June−September and December- February. (B) Monsoonal History (SWMR & NEMR) (C) Tanks and climate in India, District-wise ratio of tank irrigation to net sown area. The Chola and Pallava tank kingdoms mostly expanded across regions (modified after Gunnell et al., 2007).
Climatic reconstructions data considered for the present study is based on the following criteria: (1) The sites are located only in the Southern Peninsular India which is experiencing either NE or SW monsoon. (2) The proxy data were interpreted originally in terms of dry phase or wet phase (3) The records are within the Holocene period. However, owing to the paucity of high-resolution climatic records from the southern peninsular region, chronological uncertainty was not considered as the criteria for selection. We included and compiled all the records of lacustrine, fluvial and marine sediments. All records used in the compilation met the above-said criteria. To enable the comparison, the precipitation records were subdivided into the Southwest monsoon (SWMR) dominated region and Northeast monsoon (NEMR) dominated region. This strategy was adopted in the present study because of the spatial variability of SWMR and NEMR in the southern Peninsular India. Some of the precipitation records include isotope data, pollen data, sedimentation rate, geochemical records, clay mineralogy etc. We solely used proxy records of inferred climate shifts documented in previous records for the present study, and we did not reinterpret any original proxy data to a climate signal.
The Corg/N and δ13C ratio help to differentiate the paleoenvironment in the catchment of a lake region (Chen et al., 2002). Corg/N ratio records are available from Shantisagara Lake (Sandeep et al., 2007) and Kukkal Lake (Rajamanickam et al., 2016) spanning the Holocene period. Lake sediments from Shantisagara, Sandynallah, Parsons Valley, and Akalagavi Cave Deposits have been assessed for δ13C value (Sukumar et al., 1993; Rajagopalan et al., 1997; Yadava et al., 2004; Sandeep et al., 2017; Raja et al., 2018a). High rainfall periods are characterized by depleted δ13C values (C3 plants), whereas low rainfall periods are marked by less negative or enriched δ13C values (C4 plants). Speleothem records are available from Akalagavi cave, δ18O value of the speleothem layers are a proxy for the past variations of precipitation (Yadava et al., 2004). Enrichment and depletion in δ18O are the indicative of the past deficiency and excess in rainfall of Akalagavi cave deposit (Table 1). δ18O variation in foraminifera is also used as proxy indicator for paleoclimatic studies (Thamban et al., 2001). Intensity of Chemical weathering (CWI) is also a proxy for understanding precipitation (Sun et al., 2010). In this review, CWI records from the Palar River (Resmi and Achyuthan, 2018) and Kukkal Lake (Rajamanickam et al., 2016) are also included. CWI is often higher in warm and humid climates than in cold and dry climates. The Cao/MgO, Rb/Sr records were used in this review: Under warm and dry climate conditions, increased CaO/MgO values in the Palar River (Resmi and Achyuthan, 2018) and Kukkal Lake (Rajamanickam et al., 2016) demonstrated higher carbonate of authigenic origin (Wang et al. 2004). Magnetic susceptibility (Xlf) is a major tool for assessing climatic variations (Da Silva et al., 2014). Low (Xlf) values suggest less rainfall, and vice versa (Bhattacharyya et al., 2015). Magnetic susceptibility (Xlf) data are available from Shantisagara Lake and Thimmannanayakanakere tank (Table 1).
TABLE 1. List of proxy records (major) from terrestrial and marine climate archives compiled in this study.
Pollen inferred climate records from Shantisagara Lake, Pookode Lake, Ashtamudi- Sasthamkotta Lake, Kukkal Lake, Sandynallah Basin, Parsons Valley, Peat deposition in Kerala and Konkan coast and mangrove vegetation of Northern Konkan region provide a high-resolution paleoclimate data during Holocene (Sukumar et al., 1993; Rajagopalan et al., 1997; Kumaran et al., 2005; Nair et al., 2010; Limaye and Kumaran, 2012; Rajamanickam et al., 2016; Sandeep et al., 2017; Raja et al., 2018a) (Table 1). Arboreals and non-arboreals pollen provides insight into its climatic conditions of the past. Prevalence of non-arboreal pollen with sparce vegetation suggests wet, dynamic, and non-static climatic conditions, and vice versa. Clay mineral assemblages can be used to deduce paleoclimate. This review also includes clay mineralogy records from the Bay of Bengal and Kaliveli Lake (Pattanaik, 2009; Chauhan et al., 2010) Thecamoebians record from the Pichavaram Estuary used in this review, A high abundance of thecamoebians indicates a better marine environment, and vice versa (Srivastava et al., 2011) (Table 1).
Sukumar et al. (1993), Rajamanickam et al. (2016), and Sandeep et al. (2017) presented a detailed southwest monsoon variability on peat and lake sediments from southern Indian Peninsula spanning the Holocene period. Based on a high content of carbonate, Corg/N ratio, Corg, N, and depleted δ13C values along with dominant C4 plants indicate an overall dry phase as presented in Shanti Sagara Lake in the initial stage of the early Holocene period (11.1–10.7 ka) (Sandeep et al., 2017) (Figure 3) (Table 1). This decreasing tendency was perhaps due to the gradual transition from the Younger Dryas to the early Holocene wet phase (Figure 3). Subsequently, enhanced precipitation phase is marked during 10.7–8.6 ka, reflecting high abundance of C3 plants as studied in the Shanti Sagara Lake (Sandeep et al., 2017), Kukkal Lake (Rajamanickam et al., 2016) (Figure 3), and peat deposits in the Nilgiris (Sukumar et al., 1993). The interpolated ages from the pollen data of Parsons Valley Lake, Nilgiris, indicate a dominance of arboreal, with a subsequent shift to a significant increase in non-arboreal, suggests a decrease in precipitation in the early Holocene (Raja et al., 2018a) (Table 1). Based on the δ13C record, Sukumar et al. (1993) have marked a high dominance of C3 vegetation at ∼10.6 ka which indicate an intensified rainfall during this period, followed by a predominance of C4 vegetation suggesting a dry period from 10 to 6 ka. Various terrestrial proxies, such as magnetic susceptibility and element concentration in marine core sediments extracted from the Arabian Sea (Thamban et al., 2007), have observed a sudden rise in the intensity of SWMR during 9.5–8 ka (Table 1), which is coeval with the maxima in the Kaveri River discharge (Kale et al., 2010) and the formation of thermocline anoxia in the Arabian Sea (Staubwasser et al., 2002). Sedimentological, palynological, and stable isotopes of sediments from the Kallada Bay Delta suggest a rise in sea level during the early Holocene period due to intense rainfall (Padmalal et al., 2013). The beginning of early Holocene, optimum ∼ 10.65 ka, can be manifested from several parts of southern India (Sukumar et al., 1993; Rajagopalan et al., 1997; Caner et al., 2007; Kumaran et al., 2008; Sandeep et al., 2017; Raja et al., 2018b). Thamban et al. (2007) observed the cessation of the early Holocene climatic optimum (HCO) event occurred gradually at ∼8.5 ka. Summer monsoons were intense in the early Holocene times, as shown by lake (Rawat et al., 2015a, 2015b; Sarkar et al., 2015) and sea (Kessarkar et al., 2013; Saraswat et al., 2016) sediments from the other part of Indian subcontinent. High-resolution speleothem records from northern Oman and the Oman margin support the view that Early Holocene is marked by intense precipitation (Neff et al., 2001; Gupta et al., 2003; Fleitmann et al., 2007).
FIGURE 3. Schematic diagram of climate variability during the Holocene from records in the region which experiences SWMR.
During 8.6–4.5 ka, the high Corg/N ratio and the prevalence of C4 plants indicate an overall decreasing trend of monsoon during the mid-Holocene period, which corresponds to the 8.2 ka cold event (Sandeep et al., 2017). Moreover, southward shift of the Inter-Tropical Convergence Zone (ITCZ) can be linked to a long-term drop in precipitation during the mid-Holocene (Fleitmann et al., 2007) (Figure 3). The period between 8 and 6 ka is marked a rise in sea level along the Kerala Coast and sinking areas of the mangroves which were flourished at that time, followed by a major regression at ∼5 ka (Cronin et al., 2007).
Increased carbon (TOC), total nitrogen (TN), and the C/N ratio of Kukkal Lake are pointing towards an aridity at ∼8 ka (Figure 3). On the contrary, the paleoclimatic data from the flood plain of the upper Kaveri River indicate that a major flood occurred at ∼8 ka (Kale et al., 2010) (Figure 1) (Table 1). Enriched C/N ratio and dominant C3 terrestrial input indicate an intense rainfall during 8.4–4.9 ka with short drier phases (Raja et al., 2018b). As a continuation of early climatic optimum in the Sandhyanallah Basin experienced an intense rainfall till 5 ka, gradually an increase in C4 vegetation indicating a decreasing trend of monsoon during mid-Holocene (Sukumar et al., 1993; Rajagopalan et al., 1997) (Figure 3). High CaO and CaO/Al2O3 values indicated a lagoonal and/ or estuarine conditions between 6400 and 2600 cal year BP, which later on shifted to fresh water conditions at 2600 cal yr BP (Banerji et al., 2021).
Intense rainfall in southwest coastal plains during 6.5 ka leads to the enormous growth of forest in the abandoned river channels. The occurrence of pollen and peat deposits in these palaeoforests along the southwest coast of India suggests a warmer climate, which is consistent with a mid-Holocene thermal maximum (Kumaran et al., 2014). Studies based on geochemical proxies such as Fe, Al, and Ti, indicated that a most significant weaker monsoon during 6–5 Ka (Thamban et al., 2007) that corresponds with the foraminiferal oxygen isotope data from the sediment cores studied from the southwest coast of India (Sarkar et al., 2000; Thamban et al., 2001).
A substantial decrease in summer monsoon rainfall is also marked by a decrease in the kaolinite/chlorite ratio during 5.6 ka (Thamban et al., 2001; Table 1). In the mangroves of southern Konkan, Limaye and Kumaran (2012) suggested an increased rainfall and higher sea level from 5.6 to 5.33 ka. The marine sediment samples of Bay of Bengal marked a decrease in the intensity of SWM from 5 to 3.6 ka, which at ∼4.8 ka signify an arid climate conditions (Chauhan and Suneethi 2001).
Southern Indian palaeo-monsoonal records indicate a dry climate phase throughout the mid-Holocene (Sukumar et al., 1993) followed by wet spells and a high sedimentation rate during late Holocene (Padmalal et al., 2013). Short-term abrupt climatic fluctuations of enhanced monsoon have been inferred at 8.5, 8.4, and 8.2 ka (Thamban et al., 2007; Kale et al., 2010; Limaye and Kumaran, 2012). However, the 8.2 ka cold event relates to the abrupt weakening of ISM and during this time, solar insolation shows a decreasing tendency (Sandeep et al., 2017).
Southwest monsoon paleo-records from the other part of the Indian subcontinent are also characterized by decreasing monsoonal precipitation during the mid-Holocene. Eventhough the records reveals that during the mid-Holocene, the monsoon precipitation was waning, a few climate records show an increasing trend in monsoon strength (Prasad and Enzel, 2006; Staubwasser and Weiss, 2006). Construction of man-made dams, ponds, and other structures along the slope of the river or on tributary courses allowed water to be stored during the drier periods of the SWM-dominated regions. Around 5 ka, archaeological evidence indicates that local populations were affected, and providing alternatives to rice which was the main staple, alternative development of tilling-based agriculture caused a surge in sedimentation rates near the foot of the continental slope in the Arabian Sea. It is striking that the change in sedimentation rates roughly corresponds to the early Bronze Age’s significant cultural shifts and massive population migrations (Gourlan et al., 2020).
According to speleothem studies conducted by Fleitmann et al. (2007), the ITCZ migrated southward during the middle to late Holocene, and monsoon precipitation decreased gradually in response to decreased solar insolation; the same trend is also evident from the Indian and East Asian monsoon areas. Regardless of the fact that all monsoon records reveal unexpected and extreme monsoon events, they are short and dramatically superimposed on the consistent pattern of decreasing monsoon precipitation during mid- Holocene.
The high Corg/N ratio during 4.5–3.3 ka indicates a constant shift from plankton-dominated deep-water environments to entirely terrestrial vegetation as observed in the Shanti Sagara Lake. The paleomonsoon records of the Shanti Sagara Lake indicate a fluctuating monsoon condition from the beginning of late Holocene and decreasing trend from 4.5 ka, which corresponds to the 4.2 ka cold aridity event (Sandeep et al., 2017) (Figure 3 and Table 1). In Kukkal Lake, an increase in the intensity of chemical weathering, together with enhanced preservation of arboreal pollens and ferns, suggests a period of increasing rainfall during late Holocene ∼3.5 ka (Figure 3). Subsequently, a decrease in the sand deposition at ∼1.7 ka and replacement of forest vegetation largely by grasslands point towards a rapid increase in SWM intensity during this period. This is further corroborated by the establishment of Shola Forest during 3.5–1.70 ka (Rajamanikam et al., 2016) (Figure 3). Veena et al. (2014) noticed an increase of evergreen and semi-evergreen pollen taxa like Apocynaceae, Arenga, Artemisia (2.6%), and Calophyllum (2.5%) and an increase of panicoid, chloridoid, and festucoid suggesting a wet climate phase during 1.4–0.760 ka (Figure 3). Bhattacharyya et al. (2015) suggest that the significant high Xlf along with high influx of sand and low influx of clay in Pookot Lake during 3.1–2.5 ka indicate high precipitation during this period. Pookot Lake also indicates an overall dry period during 2.5–1 ka, with a brief episodes of intense monsoon as indicated by a rise in Xlf, sand deposition, and a reduction in clay content. The high-rainfall environments in Pookot Lake from 1 to 0.5 ka enhanced the growth of vegetation, which resulted in increased pollen formation and preservation; this time period is most likely the Medieval Warm Period (MWP) in Pookot Lake. The 0.6–0.3 ka period is marked by lower Xlf values, possibly indicating LIA with a cold and arid climate. Pookot Lake witnessed substantial rainfall during the MWP and low rainfall during the LIA, the SWMR becomes more intense after the LIA (Bhattacharyya et al., 2015). Shankar et al. (2006) marked that aridity conditions prevailed between 1.55 and 2.5 ka in the Nilgiris region, while an intense SWMR is noted at ∼1.7 ka BP (Raja et al., 2018b). Rajagopalan et al. (1997) noted the abundance of C3 plants in the Sandynallah Basin, Nilgiris, while the lake sediment samples from Parsons Valley Lake indicate a period of weakened SWM.
Along the Kerala–Konkan Coast during the beginning of the late Holocene period, ∼4 Ka, a decline in the distribution of the mangrove pollen, especially of Cullenia exarillata, and decrease in the organic matter content signifies an intense arid conditions (Kumaran et al., 2005) (Table 1). Recent studies carried out on mangrove vegetation from Northern Konkan suggest a reduced rainfall and drier climatic conditions (Limaye and Kumaran, 2012) in the late Holocene period (Figure 3).
Yadaava et al. (2004) noted that the stalagmite deposit of Akalagavi Cave shows an enrichment of δ18O values that indicate a deficient in rainfall events (which occurred during the years AD 1982, 1979, 1941, 1925, 1918, 1915, 1905, 1899, 1877, 1854, 1777, and 1796), and a depletion of δ180 designate an excess rainfall event (AD 1988, 1975, 1961, 1956, 1953, 1917, 1910, 1894, 1893, 1884, 1878, and 1664). The records shows that all deficient and excess rainfall years can be linked to countrywide rainfall as well as that the same extreme events occurring across the country (Figure 2B).
From the decadal averages, the SWMR shows a significant trend of increasing rainfall. From 1813–1820 to 1911–1920, there were 25 deficit years against 11 surplus years, but upto 2006 (86 years), there were 16 surplus years against seven deficit years, suggesting a trend of increasing rainfall than deficit. According to Verma and Bhatla (2021), the interannual variation in SWMR precipitation has been linked to El Nino in recent years, and this had a significant impact on the Eastern Ghats in the southern peninsular region. During the La Nina years, however, SWMR rainfall variation from the mean was large in the southern peninsular region. As a result of this, future flooding situation in the aforementioned places can be linked to the recurring and prolonged occurrences of La Nina events. Hari et al. (2020) suggested an increase in SWMR since 2002 because of the variations in the dynamics of the ITCZ that propagated northward since 2002.
Greenlandian Stage: Greenlandian Stage (Early Holocene): Resmi and Achyuthan (2018) studied the Palar River paleochannel sediments to reconstruct NEMR variability over the Southern Indian Peninsular spanning the Holocene period (Figure 1). It is observed that during the Early Holocene period ∼10 ka, a decrease in CWI values and higher CIA, Rb/Sr ratio of sediments indicate an enhanced NE monsoonal precipitation (Figure 4). During 10 to 4.83 ka suggests an increase in CWI values along with a decline in CIA values, and Rb/Sr ratio, pointing a decrease in rainfall in this period. However, some short pulses of enhanced NEM were also reported during this period (Resmi et al., 2017). Pollen data from the Cauvery delta sediments reveals that deposition occurred in a sub-aerial environment at the bottom of deltaic incised valleys between 11 and 9.3 ka (Mohapatra et al., 2021) (Table 1). This reveals that sea level was advanced during the LGM, as the region was 19 and 13 m below current mean sea level. From 9.3 to 6.5 ka, a rapid transgression occurred in this area, pushing the coastline 10 km inland.
FIGURE 4. Schematic diagram of climate variability during the Holocene from records in the region which experiences NEMR.
Northgrippian Stage (Mid Holocene): During ∼7.3 ka SE coast of India witnessed high stand sea level recorded from the emerged coral colony at Rameswaram (Banerjee, 2000). Later on, ∼6.5 to 5 ka mangroves had established in the nearby region might be due to stabilization of sea-level. Subsequently, an increase in NEMR precipitation is noted during ∼5.1 ka-2.5 ka from the high abundance of Poaceae, Cyperaceae along with the occurrence of aquatic taxa. Significant increase in CaO/MgO and CWI value are observed during 4.8-3.59 ka from the Palar River and its paleochannels implies a progressive rise in the precipitation. This inference is substantially corroborated by low Rb/Sr and Ba/Sr ratios (Resmi and Achyuthan, 2018).
Meghalayan Stage (Late Holocene): Resmi and Achyuthan (2018) noted a fluctuation in elemental concentrations with high sand percentage, Rb/Sr, K/Al, Ti/Al ratios and Lower CaO/MgO ratios during 3.59-3.26 ka indicating a rise in NEMR precipitation during this period. The period from 3.26 to 1.88 ka shows a significant decrease in elemental ratios of CaO/MgO, Ti/Al, CWI, and CIA values that indicate a reducing trend of NEMR. During 3.5 ka period, Srivastava et al. (2011) observed a decline in the abundance of thecamoebians studied from the Pichavaram Estuary (Figure 4). This was accompanied with significant tidal input from the Bay of Bengal and freshwater intake from the inland, indicating an increase in the monsoon conditions. This is further corroborated by smectite/kaolinite and Lower 10Be content in Kaluveli Lake representing a semi-arid to humid climate around 3.4 kyrs and humid to present day semi-arid conditions at ∼2.24 ka (Patnaik, 2009; Figure 4). Chauhan et al. (2010) inferred that high contribution of chlorite and kaolinite into the Bay of Bengal during NEMR. Hence an enhanced flux of chlorite and reduced K/C ratio indicate a high monsoon precipitation during 2.2-1.8 ka, ∼1 ka, ∼0.45 ka-0.6 ka. Likewise, a weakening of NEMR is noted from 1.8-1.1 ka BP to 1.44 ka (Figures 4, 5).
Only a few studies have been conducted around the globe to reconstruct the NEMR (winter) monsoon. Using chemical, biological, mineralogical, and physical proxies in coastal sediments from the Panama, Okanda, and Kirinda estuaries, Ranasinghe et al. (2013) noticed that intervals from >7.3 to 6.75, 4 to 3, 1.1 to 0.5 ka were marked by decreased NEMR, a short-wet interval around 6.5–6.25 ka, and a semi-arid interval between 6.25 and 4.6 ka were marked by decreased NEMR (Figure 4).
The decrease in NEMR intensity has a significant influence on paddy farming, mainly in the Cauvery delta. The Grand Anicut, also known as Kallanai, is a historic dam that spanning the Cauvery River in Srirangam in the Thiruchirapalli District. During the Sangam period (Singh, 2003; Hill, 2008), the dam was built to manage water for paddy agriculture and irrigation during the reign of Chola king Karikalan (c. 100 BC–c. 100 AD). It is India’s oldest and still operational water-regulator construction. With the reduction in NEMR during the Pallava period in the 9th and 10th centuries AD, the region around Chennai, Kanchipuram, and Chengapttu witnessed warmer conditions (1000 AD). Further, the impacts of rainfall on the Chola Kingdom’s wealth and prosperity (850–1280 ce) can correlate well with the Medieval Warm Period. However, instrumental and proxy climate data reveal that recurring El Nino-like events occurred between 850 and 1300 CE, which can be linked to a rise in NEMR, although SWMR suffered severe deficit during this time. The development of water harvesting infrastructure in the Chola Kingdom was concentrated in the NEMR-dominated area of southeastern India, with construction peaked during El Nino-dominated intervals. Because of the monumental architecture and proliferation of tanks in South India (Figure 2C), the Chola rulers are widely regarded as great supporters of culture, art, and architecture, and their dynasty is known as the ‘Golden Age of Tanks’ (Barah, 1996). Temple tanks were built to collect rainwater and supply it for home and agricultural use during the non-rainy season (Pandey et al., 2003; Ramachandran, 2006; Rajan, 2013; Meter et al., 2014). In addition to temple tanks, the Chola rulers favored the construction of multifunctional man-made lakes, also known as tanks, which were primarily used to collect runoff water for irrigation for example Veeranam Lake and Chembarambakkam Lake are two major man-made water bodies during the Chola period (Shanmugasundaram et al., 2017). Overall, the Chola’s territory strengthened and adapted to the declining NEMR conditions. As a result, it can be concluded that the Chola Dynasty’s water management system and infrastructure helped them to sustain extreme climatic conditions such as droughts and floods in the later part of its history.
The NEMR is connected to the ENSO and IOD ocean–atmospheric phenomena, and the variability of NEM rainfall reflects year-to-year change. NEMR rainfall variabilities reveals different period of high and low rainfall during the last two centuries. Furthermore, rainfall episodes near the tropical Indian Ocean be therefore a decade or more. As a result, there are no long-term trends in NEMR. In contrast, the variability of the Indian Ocean dipole moment (IODM) has shifted from a predominantly negative phase in previous decades to a predominantly positive phase in recent decades. The northeast monsoon activity is enhanced by the IODM’s positive phase. Winds are converging in the positive phase of the anomalous flow pattern, indicating moisture transport from the southeast Indian Ocean and the Bay of Bengal to southern India. In the negative phase, however, winds diverge and moisture is moved away from the southern Indian subcontinent. A negative correlation between Indian NEM and the Southern Oscillation Index (SOI), as well as a positive relationship between NEM and El Niño, which is opposite of ENSO’s relationship with Indian SWM rains. The anomalously warm SST in the western Indian Ocean, the cold SST in the eastern Indian Ocean, and the related large-scale convergence extending towards South India are all factors in the development of NEM rains during the positive dipole phase (Pattanaik and Mohapatra, 2017).
Overall, the NEMR and SWMR monsoon shows variability with important climatic events which demonstrates that the region’s geographical positions have a considerable influence on monsoon strength (Figure 5). Furthermore, our review suffers from a lack of temporal data in many regions, particularly those experiencing NEMR. When comparing multiproxy data from various archives from different locations, there can be discrepancies in interpretation. It may be due to the sensitivity of the proxies used.
To get a comprehensive picture of climatic variations of SWMR and NEMR in southern peninsular India, we have considered all available terrestrial and marine records. SWMR paleoclimatic record shows a wet period in the early Holocene and a gradual decline in monsoon precipitation in the Mid-Holocene period. However, the NEMR reveals a rise in precipitation at the beginning of the Early Holocene, followed by a decrease in the Early Holocene period. The NEMR-dominated region experienced a significant increase in precipitation during the Mid Holocene. Then, SWMR indicates an overall wet phase with a few drier episodes in the late Holocene Period, but NEMR shows an overwhelming dry period with shorter wet phases, which corresponds with the demise of the chola dynasty. During intense NEMR; IODM and El Nino exhibits a positive correlation. However, NEM shows a opposite relation to Southern Oscillation which is opposite to the relation that ENSO exhibits with the SWM rainfall. Hence, we inferred that since Holocene an antiphase relationship has been existed between NEMR and SWMR as stated by Resmi and Achythan (2018).
The SWMR records suggest that the globally known climate event ‘Younger Dryas’, HCO, MWP and LIA is reported from Southern India also but not evident in all paleoclimatic records. NEMR records, on the other hand, reveals little evidence of major climatic events apart from the MWP. Since the region is experiencing both monsoon (mostly SWMR) though intensity is less, NEMR also plays a major role in especially in the SE part of India but most of the paleoclimate reconstruction record from this region is predominately considering SWMR influence not NEMR. Hence, the present study is pointing to the need of reconstructing high-resolution NEMR and SWMR records using a multi-proxy approach, and the role of monsoon in the demise of major dynasties has yet to be explored.
RM (corresponding author) has taken a leading role. Other authors also contributed significantly.
The authors declare that the research was conducted in the absence of any commercial or financial relationships that could be construed as a potential conflict of interest.
All claims expressed in this article are solely those of the authors and do not necessarily represent those of their affiliated organizations, or those of the publisher, the editors, and the reviewers. Any product that may be evaluated in this article, or claim that may be made by its manufacturer, is not guaranteed or endorsed by the publisher.
Achyuthan, H., Nagasundaram, M., Gourlan, T. A., Eastoe, C., Ahmad, S. M., and Veena, M. P. (2014). Mid-Holocene Indian Summer Monsoon Variability off the Andaman Islands, Bay of Bengal. Quat. Int. 1e13. doi:10.1016/j.quaint.2014.07.041
Banerjee, P. K. (2000). Holocene and Late Pleistocene Relative Sea Level Fluctuations along the East Coast of India. Mar. Geology 167, 243–260. doi:10.1016/s0025-3227(00)00028-1
Banerji, U., Shaji, J., Arulbalaji, M., Vishnu, M., Dabhi, A. J., and Bhushan, R. (2021). Padmalal Mid-late Holocene evolutionary history and climate reconstruction of Vellayani lake, south India. Quat. Int. doi:10.1016/j.quaint.2021.03.018
Barah, B. C. (1996). Traditional Water Harvesting Systems: An Ecological Economic Survey. New Delhi: New Age International Limited.
Bhattacharyya, A., Sandeep, K., Misra, S., Shankar, R., Warrier, A. K., Weijian, Z., et al. (2015). Vegetational and Climatic Variations during the Past 3100 Years in Southern India: Evidence from Pollen, Magnetic Susceptibility and Particle Size Data. Environ. Earth Sci. 74, 3559–3572. doi:10.1007/s12665-015-4415-6
Caner, L., Seen, D. L., Gunnell, Y., Ramesh, B. R., and Bourgeon, G. (2007). Spatial Heterogeneity of Land Cover Response to Climatic Change in the Nilgiri highlands (Southern India) since the Last Glacial Maximum. The Holocene 17, 195–205. doi:10.1177/0959683607075833
Charney, J. G. (1969). The Intertropical Convergence Zone and the hadley Circulation of the Atmosphere. Proc. Wmo/iucg Symp. Numer. Weather Predict. Jpn. Meteorol. Agency III, 73–79.
Chauhan, O. S., Dayal, A. M., Basavaiah, N., and Kader, U. S. A. (2010). Indian Summer Monsoon Andwinter Hydrographic Variations over Past Millennia Resolved by clay Sedimentation. Geochem. Geophys. Geosyst. Res. Lett. 11, 12. doi:10.1029/2010gc003067
Chauhan, O. S., and Suneethi, J. (2001). 18 Ka BP Records of Climatic Changes, Bay of Bengal: Isotopic and Sedimentological Evidences. Curr. Sci. 81, 1231–1234.
Chen, J. a., Wan, G., Wang, F., Zhang, D. D., Huang, R., Zhang, F., et al. (2002). Environmental Records of Carbon in Recent lake Sediments. Sci. China Ser. D-Earth Sci. 45 (10), 875–884. doi:10.1360/02yd9086
Colin, C., Kissel, C., Blamart, D., and Turpin, L. (1998). Magnetic Properties of Sediments in the Bay of Bengal and the Andaman Sea: Impact of Rapid North Atlantic Ocean Climatic Events on the Strength of the Indian Monsoon. Earth Planet. Sci. Lett. 160, 623–635. doi:10.1016/s0012-821x(98)00116-2
Cronin, T. M., Vogt, P. R., Willard, D. A., Thunell, R., Halka, J., Berke, M., et al. (2007). Rapid Sea Level Rise and Ice Sheet Response to 8,200-year Climate Event. Geophys. Res. Lett. 34 (20), L20603. doi:10.1029/2007gl031318
Da Silva, A.-C., Whalen, M. T., Hladil, J., Koptikova, L., Chen, D., Spassov, S., et al. (2014). Application of Magnetic Susceptibility as a Paleoclimatic Proxy on Paleozoic Sedimentary Rocks and Characterization of the Magnetic Signal – IGCP-580 Projects and Events. Episodes 37 (2), 87–95.
Fleitmann, D., Burns, S. J., Mangini, A., Mudelsee, M., Kramers, J., Villa, I., et al. (2007). Holocene ITCZ and Indian Monsoon Dynamics Recorded in Stalagmites from Oman and Yemen (Socotra). Quat. Sci. Rev. 26, 170–188. doi:10.1016/j.quascirev.2006.04.012
Gourlan, T. A., Albarede, F., Achyuthan, H., and Campillo, S. (2020). The marine Record of the Onset of Farming Around the Arabian Sea at the Dawn of the Bronze Age. Holocene doi:10.1177/2F095968362090221810.1177/0959683620902218
Gupta, A. K., Anderson, D. M., and Overpeck, J. T. (2003). Abrupt Changes in the Asian Southwest Monsoon during the Holocene and Their Links to the North Atlantic Ocean. Nature 421, 354–357. doi:10.1038/nature01340
Gunnell, Y., Anupam, K., and Sultan, B. (2007). Response of the South Indian runoff-harvesting civilization to northeast monsoon rainfall variability during the last 2000 years: instrumental records and indirect evidence. The Holocene 17,2 (2007) pp. 207–215.
Hari, V., Villarini, G., Karmakar, S., Wilcox, L. J., and Collins, M. (2020). Northward Propagation of the Intertropical Convergence Zone and Strengthening of Indian Summer Monsoon Rainfall. Geophys. Res. Lett. 47, e2020GL089823. doi:10.1029/2020gl089823
Juyal, N., Chamyal, L. S., Bhandari, S., Maurya, D. M., and Singhvi, A. K. (2004). Environmental Changes during Late Pleistocene in the Orsang River basin, Western India. J. Geol. Soc. India 64, 471–479. doi:10.1016/j.palaeo.2004.07.017
Kale, V., Achyuthan, H., Jaiswal, M., and Sengupta, S. (2010). Palaeoflood Records from Upper Kaveri River, Southern India: Evidence for Discrete Floods during Holocene. Geochronometria 37, 49–55. doi:10.2478/v10003-010-0026-0
Kessarkar, P. M., Purnachadra Rao, V., Naqvi, S. W. A., and Karapurkar, S. G. (2013). Variation in the Indian Summer Monsoon Intensity during the Bølling-Ållerød and Holocene. Paleoceanography 28 (3), 413–425. doi:10.1002/palo.20040
Kumaran, K. P. N., Limaye, R. B., Nair, K. M., and Padmalal, D. (2008). Palaeoecological and Palaeoclimate Potential of Subsurface Palynological Data from the Late Quaternary Sediments of South Kerala Sedimentary Basin, Southwest India. Curr. Sci. 95, 515–526.
Kumaran, K. P. N., Nair, K. M., Shindikar, M., Limaye, R. B., and Padmalal, D. (2005). Stratigraphical and Palynological Appraisal of the Late Quaternary Mangrove Deposits of the West Coast of India. Quat. Res. 64, 418–431. doi:10.1016/j.yqres.2005.08.015
Kumaran, N. K. P., Padmalal, D., Nair, M. K., Limaye, R. B., Guleria, J. S., Srivastava, R., et al. (2014). Vegetation Response and Landscape Dynamics of Indian Summer Monsoon Variations during Holocene: An Eco-Geomorphological Appraisal of Tropical Evergreen Forest Subfossil Logs. PLoS One 9 (4), e93596. doi:10.1371/journal.pone.0093596
Laskar, A. H., Yadava, M. G., Ramesh, R., Polyak, V. J., and Asmerom, Y. (2013). A 4 Kyr Stalagmite Oxygen Isotopic Record of the Past Indian Summer Monsoon in the Andaman Islands. Geochem. Geophys. Geosyst. 14 (9), 3555–3566. doi:10.1002/ggge.20203
Limaye, R. B., and Kumaran, K. P. N. (2012). Mangrove Vegetation Responses to Holocene Climate Change along Konkan Coast of South-Western India. Quat. Int. 263, 114–128. doi:10.1016/j.quaint.2012.01.034
Meter, K. J. V., Basu, N. B., Tate, E., and Wyckoff, J. (2014). Monsoon Harvests: The Living Legacies of Rainwater Harvesting Systems in South India. Environ. Sci. Technol. 48 (8), 4217–4225. doi:10.1021/es4040182
Mishra, P. K. (2014). Late Quaternary climate variability in the Indian monsoon domain. PhD Thesis, Freie Universitt Berlin.
Mohapatra, P. P., Stephen, A., Prasad, S., and Anupama, K. (2021). Pollen based inference of Holocene Sea level changes, depositional environment and climatic history of Cauvery delta, Southern India, Catena 199, 105029. https://doi.org/10.1016/j.catena.2020.105029
Nagasundaram, M., Achyuthan, H., and Ahmad, S. M. (2014). Monsoonal Changes Inferred from the Middle to Late Holocene Sediments off Landfall Island, North Andaman. Arab J. Geosci. 7, 3513–3523. doi:10.1007/s12517-013-1010-6
Nair, K. M., Padmalal, D., Kumaran, K. P. N., Sreeja, R., Limaye, R. B., and Srinivas, R. (2010). Late Quaternary Evolution of Ashtamudi-Sasthamkotta lake Systems of Kerala, South West India. J. Asian Earth Sci. 37, 361–372. doi:10.1016/j.jseaes.2009.09.004
Neff, U., Burns, S. J., Mangini, A., Mudelsee, M., Fleitmann, D., and Matter, A. (2001). Strong Coherence between Solar Variability and the Monsoon in Oman between 9 and 6 Kyr Ago. Nature 411, 290–293. doi:10.1038/35077048
Padmalal, D., Kumaran, K. P. N., Nair, K. M., Baijulal, B., Limaye, R. B., and Mohan, S. V. (2011). Evolution of the Coastal Wetland Systems of SW India during the Holocene: Evidence from marine and Terrestrial Archives of Kollam Coast, Kerala. Quat. Int. 237 (1-2), 123–139. doi:10.1016/j.quaint.2010.12.021
Padmalal, D., Nair, K. M., Kumaran, K. P. N., Sajan, K., Mohan, S. V., Maya, K., et al. (2013). Climate and Sea Level Changes in a Holocene Bay Head Delta, Kerala, Southwest Coast of India. Clim. Change Isl. Coastal Vulnerability, 191–208. doi:10.1007/978-94-007-6016-5_13
Pandey, D. N., Gupta, A. K., and Anderson, D. M. (2003). Rainwater Harvesting as an Adaptation to Climate Change. Curr. Sci. 85 (1), 46–59.
Parthasarathy, B., Rupa Kumar, K., and Munot, A. A. 1993, Homogeneous Indian Monsoon Rainfall: Variability and Prediction. Proc. Indian Acad. Sci. (Earth Planet. Sci. 102 (1), 121–155.
Pattanaik, D. R., and Mohapatra, M. (2017). Active Northeast Monsoon over India during 2015 - an Assessment of Real-Time Extended Range Forecast. Curr. Sci. 112 (11), 2253–2262. doi:10.18520/cs/v112/i11/2253-2262
Pattanaik, J. (2009). Sr Isotope and Geochemical Studies on Kaveri, Palar And Ponnaiyar Rivers, Southern India and 10Be Isotope Studies on Quaternary Sediments of Kaluveli Lake. near Pondicherry, India. PhD Thesis: Pondicherry University.
Ponton, C., Giosan, L., Eglinton, T. I., Fuller, D. Q., Johnson, J. E., Kumar, P., et al. (2012). Holocene Aridification of India. Geophys. Res. Lett. 39 (3), n. doi:10.1029/2011gl050722
Prasad, S., Anoop, A., Riedel, N., Sarkar, S., Menzel, P., Basavaiah, N., et al. (2014). Prolonged Monsoon Droughts and Links to Indo-Pacific Warm Pool: a Holocene Record from Lonar Lake, central India. Earth Planet. Sci. Lett. 391, 171–182. doi:10.1016/j.epsl.2014.01.043
Prasad, S., and Enzel, Y. (2006). Holocene Paleoclimates of India. Quat. Res. 66, 442–453. doi:10.1016/j.yqres.2006.05.008
Prasad, S., Kusumgar, S., and Gupta, S. K. (1997). A Mid to Late Holocene Record of Palaeoclimatic Changes from Nal Sarovar: A Palaeodesert Margin lake in Western India. J. Quat. Sci. 12 (2), 153–159. doi:10.1002/(sici)1099-1417(199703/04)12:2<153:aid-jqs300>3.0.co;2-x
Prell, W. L., and Kutzbach, J. E. (1992). Sensitivity of the Indian Monsoon to Forcing Parameters and Implications for its Evolution. Nature 360, 647–652. doi:10.1038/360647a0
Raja, P., Achyuthan, H., Farooqui, A., Ramesh, R., Kumar, P., and Chopra, S. (2018b). Tropical Rainforest Dynamics and Paleoclimate Implications since the Late Pleistocene. India: Nilgiris. Quat. Res. doi:10.1017/qua.2018.58
Raja, P., Achyuthan, H., Geethanjali, K., Kumar, P., and Chopra, S. (2018a). Late Pleistocene Paleoflood Deposits Identified by Grain Size Signatures, Parsons Valley Lake, Nilgiris, Tamil Nadu. J. Geol. Soc. India 91, 517–644. doi:10.1007/s12594-018-0903-0
Rajagopalan, G., Sukumar, R., and Ramesh, R. (1997). Late Quaternary Vegetational and Climatic Changes from Tropical Peats in Southern India, an Extended Record up to 40,000 Years BP. Curr. Sci. 73, 60–63.
Rajan, P. (2013). Irrigation System, Water Management and Agrarian System in Southern Pennar valley under the Cholas. Indian Streams Res. J. 2 (12), 3–8.
Rajeevan, M., Unnikrishnan, C. K., Bhate, J., Niranjan Kumar, K., and Sreekala, P. P. (2012). Northeast Monsoon over India: Variability and Prediction. Met. Apps 19 (2), 226–236. doi:10.1002/met.1322
Rajmanickam, V., Achyuthan, H., Eastoe, C., and Farooqui, A. (2016). Early-Holocene to Present Palaeoenvironmental Shifts and Short Climate Events from the Tropical Wetland and lake Sediments, Kukkal Lake, Southern India: Geochemistry and Palynology. Holocene 27 (3), 404–417. doi:10.1177/0959683616660162
Ramachandran, K. (2006). Third World Water Forum – Water and Cultural Diversity – 2003. Tokyo: United Nations Educational Scientific and Cultural Organization, 113–130.
Ranasinghe, P., Ortiz, J., Smith, A., Griffith, E., Siriwardana, C., De Silva, S., et al. (2013). Mid- to late-Holocene Indian winter monsoon variability from a terrestrial record in eastern and southeastern coastal environments of Sri Lanka. Holocene 23, 945–960. doi:10.1177/0959683612475141
Rao Krishna, P. R., and Jagannathan, P. (1953). A Study of the Northeast Monsoon Rainfall of Tamilnadu. Indian J. Meteorology Geophys. 4, 22–43.
Rawat, S., Gupta, A. K., Sangode, S. J., Srivastava, P., and Nainwal, H. C. (2015b). Late Pleistocene-Holocene Vegetation and Indian Summer Monsoon Record from the Lahaul, Northwest Himalaya, India. Quat. Sci. Rev. 114, 167–181. doi:10.1016/j.quascirev.2015.01.032
Rawat, S., Gupta, A. K., Srivastava, P., Sangode, S. J., and Nainwal, H. C. (2015a). A 13,000 Year Record of Environmental Magnetic Variations in the lake and Peat Deposits from the Chandra valley, Lahaul: Implications to Holocene Monsoonal Variability in the NW Himalaya. Palaeogeogr. Palaeoclimatol. Palaeoecol. 440, 116–127. doi:10.1016/j.palaeo.2015.08.044
Resmi, M. R., Achyuthan, H., and Jaiswal, M. K. (2016). Middle to Late Holocene Paleochannels and Migration of the Palar River, Tamil Nadu: Implications of Neotectonic Activity. Quat. Int. 443, 211–222.
Resmi, M. R., and Achyuthan, H. (2018). Northeast Monsoon Variations during the Holocene Inferred from Palaeochannels and Active Channels of the Palar River basin, Southern Peninsular India. The Holocene 28 (6), 895–913. doi:10.1177/0959683617752839
Sandeep, K., Shankar, R., Warrier, A. K., Yadava, M. G., Ramesh, R., Jani, R. A., et al. (2017). A Multi-Proxy lake Sediment Record of Indian Summer Monsoon Variability during the Holocene in Southern India. Palaeogeogr. Palaeoclimatol. Palaeoecol. 476, 1–14. doi:10.1016/j.palaeo.2017.03.021
Sandeep, S., Chandrasekar, A., and Dash, S. K. (2007). On the Prediction of Tropical Cyclones over the Indian Region Using a Synthetic Vortex Scheme in a Mesoscale Model. Pure Appl. Geophys. 164 (8-9), 1443–1463. doi:10.1007/s00024-007-0231-6
Saraswat, R., Naik, D. K., Nigam, R., and Gaur, A. S. (2016). Timing, Cause and Consequences of Mid-holocene Climate Transition in the Arabian Sea. Quat. Res. 86 (2), 162–169. doi:10.1016/j.yqres.2016.06.001
Sarkar, A., Ramesh, R., Somayajulu, B. L. K., Agnihotri, R., Jull, A. J. T., and Burr, G. S. (2000). High Resolution Holocene Monsoon Record from the Eastern Arabian Sea. Earth Planet. Sci. Lett. 177, 209–218. doi:10.1016/s0012-821x(00)00053-4
Sarkar, S., Prasad, S., Wilkes, H., Riedel, N., Stebich, M., Basavaiah, N., et al. (2015). Monsoon Source Shifts during the Drying Mid-holocene: Biomarker Isotope Based Evidence from the Core 'monsoon Zone' (CMZ) of India. Quat. Sci. Rev. 123, 144–157. doi:10.1016/j.quascirev.2015.06.020
Shankar, R., Prabhu, C. N., Warrier, A. K., Vijaya Kumar, G. T., and Sekar, B. (2006). A Multidecadal Rock Magnetic Record of Monsoonal Variations during the Past 3700 Years from a Tropical Indian Tank. J. Geol. Soc. India 68, 447–459.
Shanmugasundaram, J., Gunnell, Y., Hessl, A. E., and Lee, E. (2017). Societal Response to Monsoon Variability in Medieval South India: Lessons from the Past for Adapting to Climate Change. Anthropocene Rev. 4, 110–135. doi:10.1177/2053019617695343
Singh, V. P., and Ram Narayan, Y. (2003). Water Resources System Operation: Proceedings of the International Conference on Water and Environment, 508. Allied Publishers.
Singhvi, A. K., and Kale, V. S. (2010). Paleoclimate Studies in India: Last Ice Age to the Present. Indian Natl. Sci. Acad. IGBP-WCRP-SCOPE-Report Ser. 4.
Srivastava, J., Farooqi, A., and Hussain, S. (2011). Ecological Changes in Pichavaram Estuary during the Last 4 Millennium. Int. J. Geology Earth Environ. Sci. 1, 18–26.
Srivastava, J., Farooqui, A., and Hussain, S. M. (2012). Vegetation History and Salinity Gradient during the Last 3700 Years in Pichavaram Estuary, India. J. Earth Syst. Sci. 121 (5), 1229–1237. doi:10.1007/s12040-012-0215-5
Staubwasser, M., Sirocko, F., Grootes, P. M., and Erlenkeuser, H. (2002). South Asian Monsoon Climate Change and Radiocarbon in the Arabian Sea during Early and Middle Holocene. Paleoceanography 17, 15–21. doi:10.1029/2000PA000608
Staubwasser, M., Sirocko, F., Grootes, P. M., and Segl, M. (2003). Climate Change at the 4.2 Ka BP Termination of the Indus valley Civilization and Holocene South Asian Monsoon Variability. Geophys. Res. Lett. 30 (8). doi:10.1029/2002gl016822
Staubwasser, M., and Weiss, H. (2006). Holocene Climate and Cultural Evolution in Late Prehistoric-Early Historic West Asia. Quat. Res. 66 (3), 372–387. doi:10.1016/j.yqres.2006.09.001
Sukumar, R., Ramesh, R., Pant, R. K., and Rajagopalan, G. (1993). A δ13C Record of Late Quaternary Climate Change from Tropical Peats in Southern India. Nature 364, 703–706. doi:10.1038/364703a0
Sun, Y., Ding, Y., and Dai, A. (2010). Changing Links between South Asian Summer Monsoon Circulation and Tropospheric Land-Sea thermal Contrasts under a Warming Scenario. Geophys. Res. Lett. 37, L02704. doi:10.1029/2009gl041662
Thamban, M., Kawahata, H., and Rao, V. P. (2007). Indian Summer Monsoon Variability during the Holocene as Recorded in Sediments of the Arabian Sea: Timing and Implications. J. Oceanogr. 63, 1009–1020. doi:10.1007/s10872-007-0084-8
Thamban, M., Purnachandra Rao, V., Schneider, R. R., and Grootes, P. M. (2001). Glacial to Holocene Fluctuations in Hydrography and Productivity along the Southwestern continental Margin of India. Palaeogeogr. Palaeoclimatol. Palaeoecol. 165 (1-2), 113–127. doi:10.1016/s0031-0182(00)00156-5
Trauth, M. H., Deino, A. L., Bergner, A. G. N., and Strecker, M. R. (2003). East African Climate Change and Orbital Forcing during the Last 175 Kyr BP. Earth Planet. Sci. Lett. 206, 297–313. doi:10.1016/s0012-821x(02)01105-6
Veena, M. P., Achyuthan, H., Eastoe, C., and Farooqui, A. (2014). A Multi-Proxy Reconstruction of Monsoon Variability in the Late Holocene, South India. Quat. Int. 325, 63–73. doi:10.1016/j.quaint.2013.10.026
Verma, S., and Bhatla, R. (2021). Performance of RegCM4 for Dynamically Downscaling of El Nino/La Nina Events during Southwest Monsoon over India and its Regions. Earth Space Sci. 10, 1029. doi:10.1029/2020EA001474
Walker, M. J. C., Berkelhammer, M., Björck, S., Cwynar, L. C., Fisher, D. A., Long, A. J., et al. (2012). Formal Subdivision of the Holocene Series/Epoch: a Discussion Paper by a Working Group of INTIMATE (Integration of Ice-Core, marine and Terrestrial Records) and the Subcommission on Quaternary Stratigraphy (International Commission on Stratigraphy). J. Quat. Sci. 27 (7), 649–659. doi:10.1002/jqs.2565
Wang, H., Liu, H., Liu, Y., and Cui, H. (2004). Mineral Magnetism of Lacustrine Sediments and Holocene Paleoenvironmental Changes in Dali Nor area, Southeast Inner Mongolia Plateau, China. Palaeogeogr. Palaeoclim. Palaeoecol. 208, 175–193.
Keywords: southern India, SWMR and NEMR, MWP, LIA, ancient settlement
Citation: Resmi MR, Achyuthan H and Deopa H (2022) Holocene Monsoonal Variations Over Southern India: Looking Into Its Antecedents. Front. Earth Sci. 9:717420. doi: 10.3389/feart.2021.717420
Received: 30 May 2021; Accepted: 31 August 2021;
Published: 31 January 2022.
Edited by:
Xander Wang, University of Prince Edward Island, CanadaReviewed by:
Yu Li, Lanzhou University, ChinaCopyright © 2022 Resmi, Achyuthan and Deopa. This is an open-access article distributed under the terms of the Creative Commons Attribution License (CC BY). The use, distribution or reproduction in other forums is permitted, provided the original author(s) and the copyright owner(s) are credited and that the original publication in this journal is cited, in accordance with accepted academic practice. No use, distribution or reproduction is permitted which does not comply with these terms.
*Correspondence: M. R. Resmi, cmVzbWlhcnVuLm1yQGdtYWlsLmNvbQ==
Disclaimer: All claims expressed in this article are solely those of the authors and do not necessarily represent those of their affiliated organizations, or those of the publisher, the editors and the reviewers. Any product that may be evaluated in this article or claim that may be made by its manufacturer is not guaranteed or endorsed by the publisher.
Research integrity at Frontiers
Learn more about the work of our research integrity team to safeguard the quality of each article we publish.