- 1Key Laboratory of Comprehensive and Highly Efficient Utilization of Salt Lake Resources, Qinghai Institute of Salt Lakes, Chinese Academy of Sciences, Xining, China
- 2Key Laboratory of Salt Lake Geology and Environment of Qinghai Province, Xining, China
- 3University of Chinese Academy of Sciences, Beijing, China
- 4Qaidam Integrated Geological Exploration Institute of Qinghai Province, Golmud, China
- 5Key Laboratory of Salt Lake Resources Exploration in Qaidam Basin, Golmud, China
Lithium ore deposits are divided into pegmatite and brine deposits. The Puna Plateau and the Qinghai–Tibetan Plateau (QTP) are home to the most abundant brine lithium deposits worldwide. Very few studies have investigated the chronology of brine lithium deposits. This paper reports the Optically Stimulated Luminescence (OSL) dating measurements for typical brine lithium deposits at QTP, including East Taijnar Salt Lake, West Taijnar Salt Lake, and Yiliping Salt Lake in the central Qaidam Basin. Combining the results of OSL dating with previous studies and mineral composition obtained by X-ray diffraction analysis (XRD), this study summarizes the age and characteristics of the climatic environment during the formation of brine lithium deposits in the Qaidam Basin. The main results are: 1) Brine lithium deposits in the Qaidam Basin began to form since 40 ka. Brine lithium deposits in South America formed during the middle Pleistocene and late Pleistocene, and are older than the deposits in the Qaidam Basin. The lithium deposits of Tibet formed around 4 ka, are the youngest. 2) The climate in East Taijnar Salt Lake and West Taijnar Salt Lake was extremely cold and dry during 27–4.6 ka, with a relatively humid climatic condition at ∼10 ka. After 4.6 ka, the environment was comparatively more humid around both lakes. Yiliping Salt Lake had a dry climate since 38.09 ka, and the climate in the Three Lakes area is mainly controlled by the westerlies in the Holocene; and 3) East Taijnar Salt Lake, West Taijnar Salt Lake and Yiliping Salt Lake were located in the same secondary basin during the late Pleistocene. However, tectonic activity around 40 ka led to the evolution of Yiliping Salt Lake into an independent basin. East Taijnar Salt Lake and West Taijnar Salt Lake separated around 27 ka, and then deposited the lower salt layers until the Holocene. The substantial amount of detrital minerals that the Nalinggele River brought during the Holocene led to a brief desalination of East Taijnar Salt Lake. The upper salt layer was deposited in East Taijnar Salt Lake and West Taijnar Salt Lake during this period due to the extremely dry climate.
Introduction
Lithium, a scarce strategic resource, is widely used in ceramics and glass industries, batteries, lubricating greases, air treatment, and primary aluminum production. Global lithium deposits are estimated at over 17 Mt (million tons) (Yang et al., 2019). There are three main types of lithium deposits: 1) brine deposits in lacustrine evaporite deposits. The most abundant lithium brine deposits are found in South America (Salar de Uyuni and Salar de Atacama) and China (West Taijnar Salt Lake, East Taijnar Salt Lake, and Yiliping Salt Lake); 2) pegmatite deposits in the vein of pegmatite. For example, the Greenbushes lithium deposit in Australia; and 3) sedimentary rock deposits in Cainozoic clay, such as Jadar lithium deposits in Serbia and La Ventana lithium deposits in Mexico (Wang et al., 2015; Zhang et al., 2020). Brines containing lithium account for 66% of the world’s lithium resources (Gruber et al., 2011), primarily in the central Andes and China, with smaller deposits in the western United States and northern Africa (Kesler et al., 2012).
Several existing studies have conducted Quaternary geochronological studies on the Salar de Atacama and the Salar de Uyuni, the two largest brine-producing deposits. However, most studies focus on alluvial fans, deserts, or ice cores nearby these two Salars (Abbott et al., 1997; Klein et al., 1999; Rodbell et al., 1999; Thompson et al., 1999; Baker et al., 2001a; Pfeiffer et al., 2018; Diederich et al., 2020; Walk et al., 2020). Limited research has concentrated on Quaternary geochronology in the Salar de Atacama and the Salar de Uyuni (Baker et al., 2001b; Bobst et al., 2001; Fornari et al., 2001). Additionally, the above-mentioned studies mainly investigated the paleoclimate and evolution of the Salar de Atacama and the Salar de Uyuni. However, the age of formation of lithium deposits remains unconfirmed. The age of brine lithium deposits can help a deeper and broader understanding of the mechanism of mineralization.
Other lithium-rich lacustrine evaporites and brines are found on the Qinghai–Tibet plateau, most of which are distributed in the Qaidam Basin. The Qaidam Basin, at an elevation of 2,800 m a.s.l, is the largest basin on the northeastern Tibetan plateau, covering an area of 2.4 × 105 km2. Thanks to the “higher mountain and lower basin” circumstances and hyper-arid climate (Yuan et al., 1983; An et al., 2012; Yu et al., 2013), the basin has developed a vast area of dry playa capped with salt crust, leading to the deposition of abundant brine resources (Zhang, 1987). Previous research suggests the preservation of a mega–paleolake in the Qaidam Basin before the late Pleistocene (Zhang, 1987; Zhu et al., 1989). Several recent studies (Yu and Lai, 2012; Lai et al., 2014; Yu and Lai 2014) have confirmed the fragmentation of the mega-paleolake to the western (East Tijinaier Salt Lake, West Taijnar Salt Lake, and Yiliping Salt Lake) and eastern parts (Qarhan Salt Lake) (Zhang, 1987; Owen et al., 2006; Zhang et al., 2007) as a result of tectonic evolution and climate change.
Most previous Quaternary chronological studies in the Qaidam Basin primarily used U-series disequilibrium dating methods and radiocarbon dating (14C) (Figure 1 and Supplementary Table S1). Chronological data of salt lakes in the Qaidam Basin over the past 250 ka and 10 ka are shown in Figure 1 (Shen et al., 1993; Liang and Huang, 1995; Huang and Han, 2007; Fan et al., 2010; Sun et al., 2010; Ma et al., 2011; Fan et al., 2013; Liang and Han, 2013; Han et al., 2014; Chen et al., 2017; Zeng and Xiang, 2017; Wang et al., 2019; Wang, 2020; Zhang and Liu, 2020). On the one hand, previous chronological works have focused especially on the east Qaidam Basin, while the central and western Qaidam Basins are still insufficiently studied. On the other hand, most previous chronological analyses mainly used 14C dating and 230Th dating. Chronological studies generally use different types of methods to verify each other, thereby infer the geological evolution accurately. There are 24 OSL ages for the salt lakes in the Qaidam Basin, in which there are only 6 ages for the central Qaidam Basin. The limited ages data has hiatus the understanding of the formation and evolution of salt lakes at the Qaidam Basin. In this study, we excavated three sections in the center of the Qaidam Basin. The sections were suitable for OSL dating due to the thick deposition of detrital minerals, including quartz. Our study demonstrates the applicability of OSL dating in salt lakes and complements data on the age of salt lakes in the central Qaidam Basin, providing key information on the origin and evolution of lakes.
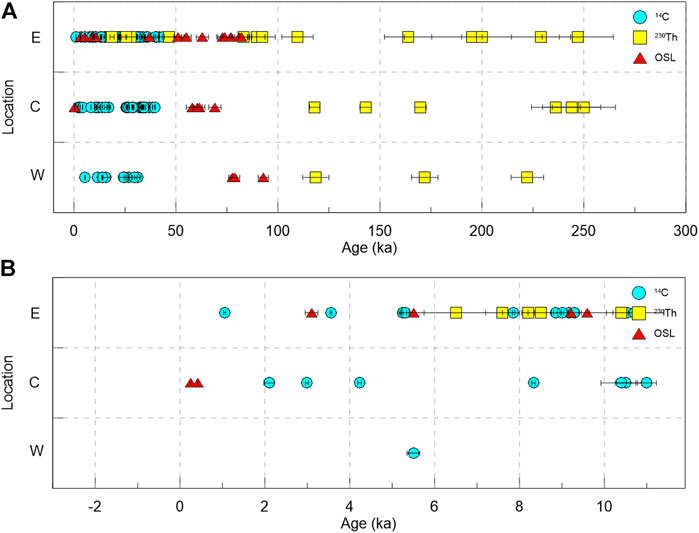
FIGURE 1. Summary of published chronological data of the salt lakes in the Qaidam Basin over the past 250 ka (A) and 10 ka (B). The records are mainly from the east (E), center (C), and west (W) of the Qaidam Basin. For more detailed information, see Supplementary Table S1 in the supplementary material.
The lithium content in brines in the Qaidam basin is concentrated and has the highest values in West Taijnar Salt Lake, East Taijnar Salt Lake and Yiliping Salt Lake, collectively called “The Three Lakes”. Studies on the origin and formation of brine lithium deposits in the Qaidam Basin are scarce. Three different hypotheses can be drawn from previous research. The first hypothesis suggests a multi-source lithium contribution to the resource with two possibilities: 1) the residual brines formed during the most recent Pliocene in the saline lakes located in the western Qaidam Basin and substantially contributed to the lithium deposits; and 2) the residual brine interacted with Li-rich waters from deep underground connate brines, facilitating lithium enrichment in the salt lakes since the late Pleistocene (Zhang, 1987). The second hypothesis emphasizes the important contribution of lithium from two ancient lakes, Nalinggele Lake and Kunlun Lake. These lakes possibly existed as large intermontane lakes in the Kunlun Mountains until 30 kaBP. The former was in the Nalinggele River valley, while the latter was south of Nalinggele Lake, receiving hot spring water rich in Li, B, and K (Zhu et al., 1989). The tectonic activity induced drainage of these two ancient saline lakes and several others in the eastern Kunlun Mountains in the Qaidam Basin probably led to evaporite deposition, eventually becoming the source of Li, B, and K. The third view from Yu et al. (2013) reported that the hydrothermal fields where the two active faults converged in the upper reach of the Hongshui River are a source of lithium in the Qaidam Basin. Overall, regardless of the source of lithium, it was enriched simultaneously with the evaporite formation, which implies that both were of identical age.
There are few other Quaternary chronological studies for the Three Lakes area. Zhang et al. (2001) indicated that the salt was formed in East Taijnar Salt Lake during one of the driest periods of the Holocene. However, the sampling sites were not in the center of East Taijnar Salt Lake, which led to limitations in the evolution of the lake proposed in the study. Chen et al. (2017) discussed salt deposits and their relationship to the Quaternary glacial period and tectonic movement in Yiliping Salt Lake. However, the time scale was more than a million years, which is not suitable for researching the evolution and age of lithium in the lake since the late Pleistocene. Wang et al. (2019) dated two cores in West Taijnar Salt Lake using OSL and radiocarbon dating (AMS 14C). The condition experiments of OSL dating were conducted, and the feasibility of two methods was also discussed. However, the dating results are not enough to conclude the lake evolution. Zeng and Xiang (2017) studied the chronology of West Taijnar Salt Lake using OSL and AMS 14C. However, the analyzed part was located in the northeastern West Taijnar Salt Lake, near the Bayanwuer anticline, which might have perturbed the stratum sequence. Therefore, the dating results from this study are also not suitable for an accurate illustration of the lake evolution. Chang et al. (2017) dated the terraces of the Nalinggele River, where is the provenance of the Three Lakes. In general, very few studies have analyzed the Three Lakes, especially during the formation of the lithium deposits since the Holocene. The Three Lakes could provide significant insights into the formation and evolution of the central Qaidam Basin due to their location. This study aims to combine the dating results (OSL dating method), mineral compositions (using XRD), and previous studies to conclude the age of lithium deposits and geomorphic processes in the central Qaidam Basin.
Geological and Geographic Setting
The Qaidam Basin is the largest closed basin on the northeastern Tibetan Plateau. This hyperarid inland basin, with an area of 2.4 × 105 km2, is surrounded by the Altun-Qilian Shan Mountains (Mts) in the north, the Ela Shan Mts in the east, and the Kunlun Shan Mts in the south. In the basin, mean annual temperatures range from 0 to 5°C and mean annual precipitation (MAP) ranges from 100 mm in the east to less than 20 mm in the west. The potential mean annual evaporation is over 20 times higher than the MAP. Gravel desert, playa and salt crust, saline lakes and salt marshes and various yardang landforms are widespread in the basin, forming a striking desert landscape (Figure 2).
East Taijinar Salt lake, West Taijinair Salt lake and Yiliping Salt Lake lie in sub-depressions of low-lying land along the middle of the central Qaidam Basin in hyperarid conditions with precipitation limited to 15–35 mm/y and potential evaporation to precipitation ratio greater than 100:1. The Three Lakes in the Qaidam Basin, which is fed by the Nalinggele River, are significantly enriched with lithium and boron that are key resources for the regional economy (Zhang, 1987; Zhu et al., 1989; Yu et al., 2013). Before the late Pleistocene, the Three Lakes and the Qrahan salt lake were consolidated freshwater shallow lakes. Neotectonics led to the separation of the Three Lakes from Qarhan salt lake and the subsequent formation of the terminal lake at the end of the Nalinggele River. Quaternary sediments, extending several hundreds of kilometers, are the main deposits in the Three Lakes. The Taijnar tectonic belt and the Bayanwuer tectonic belt, including faults and anticlines, are located north of East Taijnar Salt Lake and West Taijnar Salt Lake. Few tectonic zones surround Yiliping Salt Lake, while some minor faults are in the south (Figure 3).
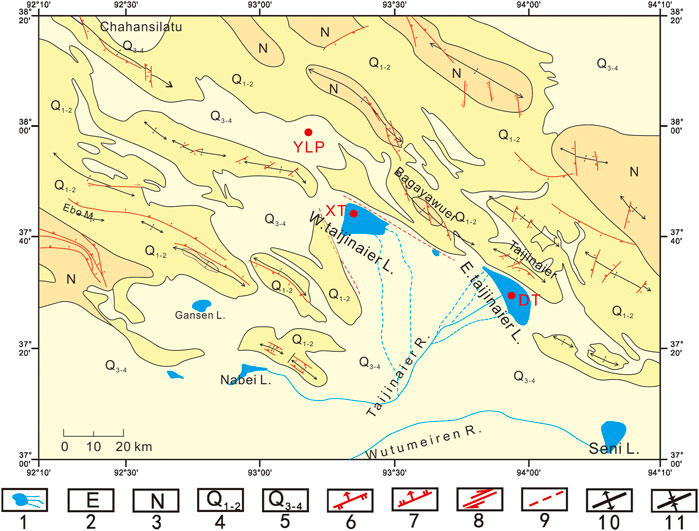
FIGURE 3. Map of major tectonic and sedimentologic features. Legend: 1. Lakes and rivers; 2. Neogene; 3. Palaeogene; 4. Early Pleistocene-Middle Pleistocene; 5. Epipleistocene–Holocene; 6. Reverse fault; 7. Normal fault; 8. Trike-slip fault; 9. Fault observed by remote sensing; 10. Anticline; and 11. Syncline. Study sites are marked with red dots.
Sample Collection
Fieldwork was conducted in 2017, and three sections were explored, including DT (in East Taijnar Salt Lake), XTW (in West Taijnar Salt Lake), and YLP (in Yiliping Salt Lake). The East Taijnar Salt Lake section, named the DT section (37°27’47.11” N, 93°57’09.71” E; 2,686 m a.s.l.), lies in the central part of the lake (Figure 1). The section was excavated to a depth of 13.4 m (Figure 2). The lithology of the DT section from top to bottom is as follows: salt crust from 0 to 0.2 m, with one XRD sample in this layer (0.1 m) and 45 other XRD samples taken for X-ray diffraction analysis (XRD) from 0.3 to 13.4 m, at a distance of 0.3 m; clay-silt from 0.2 to 8.4 m, with 16 optical dating samples collected at intervals of 0.5 m from 0.45 to 7.95 m (Figure 4A); clay-silt with white halite from 8.4 to 8.7 m; and white halite from 8.7 to 13.4 m.
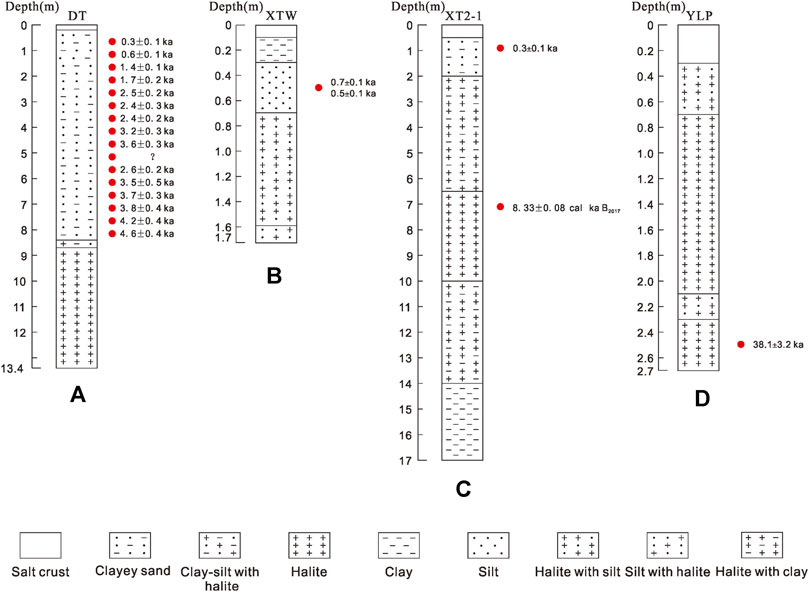
FIGURE 4. Stratigraphy, OSL sample site, and dating results for the Three Lakes: (A) Section DT in East Taijnar Salt Lake; (B) Section XTW in West Taijnar Salt Lake; (C) Section XT2-1 in West Taijnar Salt Lake (Wang et al., 2019); and (D) Section YLP in Yiliping Salt Lake.
The XTW section (37°43’54.98” N, 93°20’33.10” E; 2,686 m a.s.l.) lies west of West Taijnar Salt Lake. The section was excavated to a depth of 1.7 m. The XTW section is divided into 5 strata, which are: salt crust from 0 to 0.1 m; clay from 0.1 to 0.3 m; silt from 0.3 to 0.7 m, one sample was taken at 0.5 m and another at 0.6 m for OSL dating; white halite with silt from 0.7 to 1.6 m; and silt with halite from 1.6 to 1.7 m. Another sample for dating was taken at 1.65 m (Figure 4B).
West of Yiliping Salt Lake, a 2.7 m long section was discovered and named the YLP section (37°58’3.83” N, 93°13’7.80” E; 2,685 m a.s.l.). The YLP section lies in the western Yiliping Salt Lake. It consists, from top to bottom, of a salt crust layer, halite with silt layer, halite layer, halite with silt layer, and halite layer. We obtained a dating sample at 2.5 m (Figure 4D).
All OSL samples were collected by driving iron tubes (diameter 5 cm, length 25 cm) in newly cleaned vertical sections. The tubes were covered with a black cloth and sealed in black plastic bags, then wrapped with tape to avoid exposure to light. Each XRD sample, weighing about 50 g, was taken in clean bags.
Materials and Methods
Optically Stimulated Luminescence Dating
Sample preparation and OSL measurements were performed in the Luminescence Dating Laboratory of the Qinghai Institute of Salt Lakes, Chinese Academy of Sciences. First, two sides of each luminescence dating sample tube were removed to measure the concentration of the radioactive element and the water content. The unexposed middle part of the tube was used to extract minerals to determine the equivalent dose (De). All samples were treated with 10% HCl and 30% H2O2 to remove carbonates and organic matter. Thereafter, the samples were passed through a wet sieve to obtain a grain fraction of 38–63 μm. Polymineral grains of 4–11 μm were separated according to the Stokes’ Law settling. Then, the quartz fraction of 38–63 μm was treated with 35% hydrofluorosilicic acid (H2SiF6) for about two weeks, while the quartz fraction of 4–11 μm was treated with 35% H2SiF6 for 3–5 days. Both fractions were then treated with 10% HCl to remove any fiuorides. The purity of the extracted quartz was checked by IR stimulation. In case of obvious IR signals, quartz grains were retreated with H2SiF6 to avoid underestimation of age (Duller, 2003; Lai and Brückner, 2008). Samples of pure quartz were then mounted on stainless steel disks (diameter ∼0.97 cm), using silicone oil, with an area of about 0.7 cm in diameter.
The OSL signal was measured using an automated Risø TL/OSL-DA-20 reader. The reader was equipped with blue diodes (λ = 470 ± 20 nm) and IR laser diodes (λ = 830 nm). Laboratory irradiation was performed using 90Sr/Y90 sources mounted in the reader, with a dose rate of 0.089 Gy/s. Luminescence, stimulated by blue LEDs at 130°C for 40 s, was detected using a photomultiplier tube with an OSL signal passing through 7.5 mm thick U-340 filters. Equivalent De was measured using a single-aliquot regenerative-dose (SAR) (Murray and Wintle, 2000) and the standardized growth curve (SGC) method (Roberts and Duller, 2004; Lai, 2006). The sample was preheated to 260°C for 10 s and cut-heat to 220°C for 10 s. The signals of the first 0.64 s stimulation are integrated to build a growth curve after background subtraction (last 8 s).
The concentration of U and Th in all samples was measured by ICP-MS, while the K content was measured by ICP-OES. For grains of 38–63 μm, the alpha efficiency value was taken as 0.035 ± 0.003 (Lai et al., 2008). For grains of 4–11 μm, the alpha efficiency value was assumed to be 0.04 (Ree-Jones, 1995). The calcuation of the cosmic dose rate is based on Prescott and Hutton (1994). The water content (%) was determined by the ratio of the weight of water in the sediment to the weight of dry sediments. A 5% error was added to the water content when calculating the dose rate for each sample.
X-ray Diffraction Analysis
Mineral compositions of 45 samples, grounded into powder (<75 μm) using ball mill, were analyzed using a Panalytical X-pert Pro diffractometer at the Qinghai Institute of Salt Lakes, Chinese Academy of Sciences. The relative volume percentage of minerals was calculated using the formula from Chung (1974) based on the diffraction intensity of the sample.
Results and Discussion
The results of the OSL dating with their Equivalent dose (De) values are given in Table 1 and Figure 4. The obtained ages are generally in line with their geomorphic sequences (Figure 4). The OSL decay curves and the regenerative-dose growth curves fitted by a single saturation exponential plus linear function are presented in Figure 5. Mineral composition of section DT is summarized in Figure 6. Quartz, alite, muscovite, and chlorite were identified as detrital minerals in the DT section. The average content of these detrital minerals was approximately 49.71%. The average carbonate content was 9%, and consisted mainly of calcite and dolomite, while aragonite occurred in limited horizons. The evaporite salt minerals in section DT were mainly halite and gypsum, with a small amount of sylvine (10–13.4 m). Detrital minerals, carbonates and gypsum were generally abundant between 0.4 and 9.1 m. For comparison, halite appeared mainly at 9.4–13.4 m and its content exceeded 70% (Figure 6 and Supplementary Table S2).
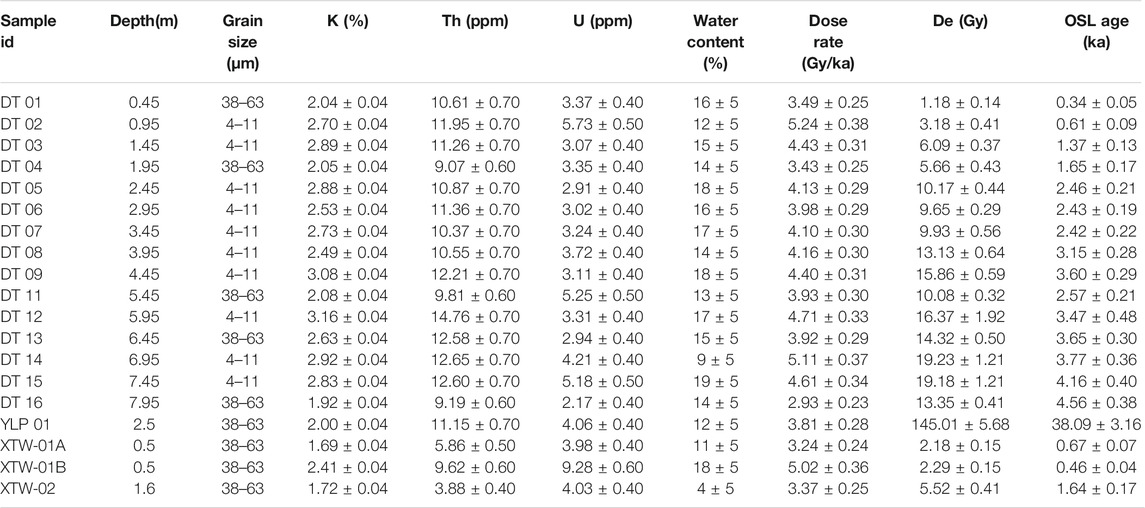
TABLE 1. | Results of the OSL dating of sections DT, XTW and YLP in the Three Lakes. The OSL age of the DT10 sample could not be analyzed due to quartz deficiency.
Salt Crust Formation in Central Western Qaidam
The salt crust of the DT section, located in East Taijnar Salt Lake, was 0.2 m thick. The result of OSL dating of DT01 (0.45 m at the DT section) revealed that the age of the salt crust in East Taijnar Salt Lake is about 0.35 ka (Figure 4A). The thickness of the salt crust in West Taijnar Salt Lake was recorded as 0.1 m in the section XTW. Dating samples (XTW01A and XTW01B) collected at a depth of 0.5 m were dated to about 0.7 ± 0.1 ka and 0.5 ± 0.1 ka, respectively (Figure 4B). These ages indicate that the salt crust in West Taijnar Salt Lake developed after 0.67 ka. The dating results of several sections analyzed by Wang et al. (2019) showed that the age of the salt crust in West Taijnar Salt Lake is younger than at least 0.4 ka, and the age of the crust is even younger than 0.3 ka in some sections. Combining these observations with our results, it can be concluded that the salt crust in West Taijnar Salt Lake developed for several years and is younger than hundreds of years. The results of Zeng and Xiang (2017) show that the age of the surface crust of West Taijnar Salt Lake is approximately 57.9 ka, which does not agree with the results of our study and with the results of Wang et al. (2019). This disagreement could be the result of the fact that the lake section analyzed by Zeng and Xiang (2017) was not in West Taijnar Salt Lake, but in the north of West Taijnar Salt Lake, near the Bayanwuer anticline (Figure 3). The YLP section, which lies at Yiliping Salt Lake, has developed a 0.2 m thick salt crust. A limited number of quartz grains resulted in a lack of dating samples, which prevented the assessment of the age of the salt crust. However, the age of the salt crust in Yiliping Salt Lake was obtained by analysis of Huang and Han (2007). This study analyzed the age of borehole 82CK1 in the center of Yiliping Salt Lake by radiocarbon dating and reported the age of the surface salt crust younger than 2.10 ± 0.25 ka.
The salt crust in Chahansilatu playa was explored by Han et al. (2014). Their work shows that the depth of the salt crust in Chahansilatu is about 2.0 m. The age of the three samples with depths of 0.5, 1.0, and 1.9 m was reported as 77.8 ± 4.0 ka, 78.9 ± 4.8 ka and 92.9 ± 5.1 ka, respectively, which confirms the formation of the salt crust in Chahansilatu at 90 ka. The salt crust is 0.21 m thick in Dalangtan salt lake. Ma et al. (2011) analyzed section D26 in Dalangtan salt lake. Their results showed that the salt crust in Dalangtan salt lake is 0.21 m thick. From the sample's age at a depth of 0.47 m it can be concluded that the salt crust in Dalangtan salt lake developed by 118 ka at most. The thickness of the salt crust in Kunteyi salt lake was recorded as 0.1 m, while the age is between 14.1 ka and 24.4 ka (Huang and Han, 2007). Previous studies definitely reveal the variability of salt crust thickness in the Qaidam Basin. The salt crust thickness is less than 0.2 m in the Three Lakes, which is thinner than the salt crust in the western Qaidam Basin, such as Chahansilatu and Dalangtan salt lake. In addition, the salt crust in Chahansilatu, Dalangtan, and Kunteyi salt lakes formed earlier than the salt crust in the Three Lakes.
Age of Lithium Deposits Formation
The brine lithium deposits are distributed in the Three Lakes in the Qaidam Basin. The formation of lithium from evaporites indicates that the age of formation of the lithium deposits is the same as the age of salt formation. Stratigraphy of boreholes from south to north and from east to west in East Taijnar Salt Lake shows that lithium deposits can be classified into two types: 1) phreatic brine lithium deposits in the upper halite layer, and 2) confined brine lithium deposits in the lower halite layer.
Furthermore, Figure 7 reveals that the deposition center of the lake is close to the CK264 core, which is also the deposition center of the confined brine lithium deposits. The DT section is close to CK264, which implies that the dating results from the DT section can reflect the age of formation of the confined brine lithium deposits. The confined lithium brine deposits are found in the lower halite layer below 8.4 m in the DT section. The dating results of the clayey sand located above the lower halite layer reveal that the confined brine lithium deposits formed before 4.6 ka (Figure 4A). Liang and Han (2013) indicated that the age of the Upper Pleistocene sediments is 27 ka. Therefore, we infer that the age of the confined brine lithium deposits is between 27 and 4.6 ka. Since the depositional center of the phreatic lithium deposits is in the northwestern East Taijnar Salt Lake (Figure 7A), we can only conclude that it was formed after 4.6 ka at the most. According to the similarity of the evolution of West Taijnar Salt Lake and East Taijnar Salt Lake from the Late Pleistocene (Zhu et al., 1989), the age of formation of the brine lithium deposits of the two lakes is the same. Lithium deposits in Yiliping Salt Lake are found in surface halite above 2.3 m (Figure 4D). Wang (2020) revealed that the upper halite layer began to deposit at 40 ka, which is in line with our conclusions. This not only confirms the accuracy of our dating results, but also suggests that the lithium deposits formed after 40 ka.
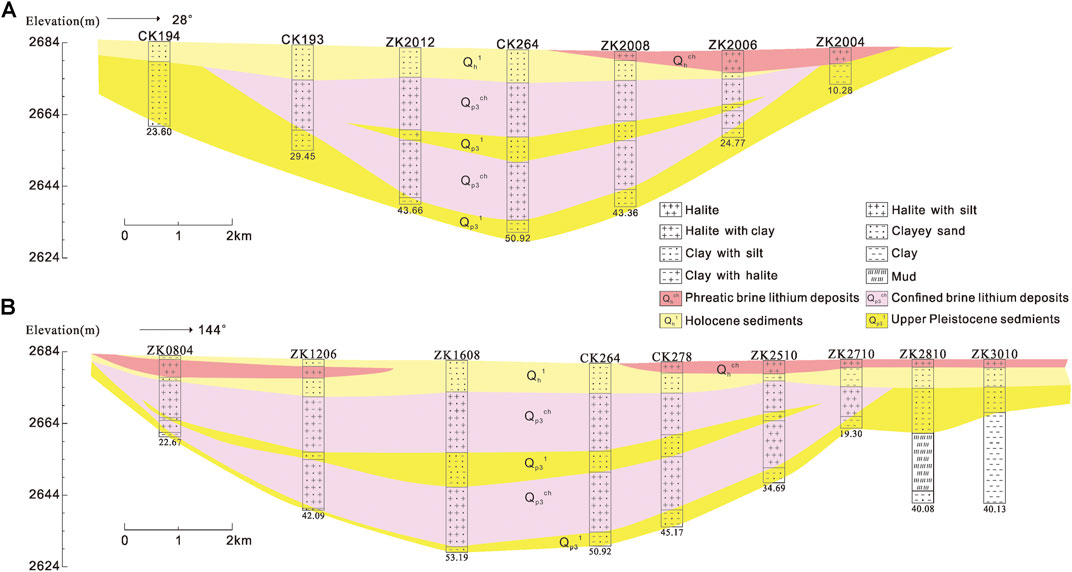
FIGURE 7. Stratigraphy and distribution of lithium deposits in East Taijnar Salt Lake: (A) from south to north, and (B) from west to east.
The most classic and abundant lithium brine deposits are distributed in South America and the Qaidam Basin, so we compare their age of lithium deposits. Previous chronological studies in South America can help us estimate the age of lithium deposits formation. The current lithium production comes from the Salar de Atacama in Chile, with an area of about 3,000 km2. The lithium-bearing brine fills the upper part of the salt layer at a depth of about 35 m (Garrett, 2004). From a chronological study of a 100 m long salt core in the central part of the Salar de Atacama (Bobst et al., 2001), it is assumed that the age of formation of lithium deposits in halite above 35 m is about 60.7 ka (Figure 8A). Another well-known brine lithium deposit in South America is located in the Salar de Uyuni. The Salar de Uyuni contained 11 lacustrine layers (L1-L11) and 12 salt layers (S1-S12) (Figure 8B). Ericksen et al. (1978) indicated that lithium deposit is located in the halite layer at depths of 0–10 m. Lithium levels at depths below 10 m have not been analyzed, which limited the analysis to salt layers above 10 m. Fornari et al. (2001) showed that the salt layers above 10 m formed since 16.1 ka (Figure 8B). In contrast, Baker et al. (2001b) indicated the age of the salt layer above 10 m is 18.92 ka BP, implying that the age of formation of lithium is at least 18.92 ka. Lithium deposits below a depth of 10 m may be older than 18.92 ka.
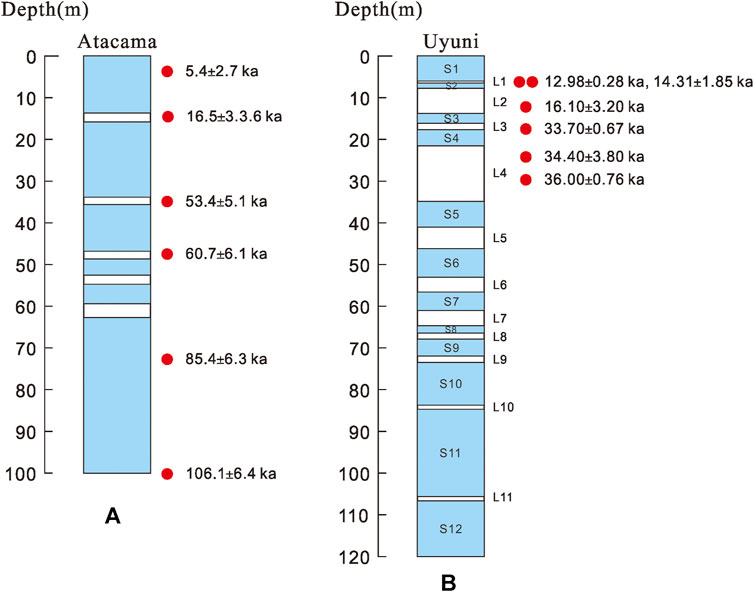
FIGURE 8. Stratigraphy, sample site, and dating results for salt lakes in South America: (A) core in the Salar de Atacama, the dating results being analyzed by the radiocarbon dating method Bobst et al. (2001); and (B) core in the Salar de Uyuni, for which the U-series disequilibrium dating method is used. The blue squares represent the salt layers and the white squares represent the lacustrine layers Fornari et al. (2001).
Zabuye Lake is located in the southwestern region of the Tibetan Plateau, which is another region with the brine lithium deposits in the surface lakes. Liu and Zheng (1999) hypothesized that Zhabuye Lake became a salt lake at 8.9 ka. Therefore, strong evaporation around 4 ka led to the deposition of brine lithium.
In summary, the brine lithium deposits in South America formed during the Middle Pleistocene. In contrast, the lithium deposits in the Qaidam Basin are younger as they formed between the Late Pleistocene and Holocene. The youngest lithium deposits in Tibet are 4 ka old.
Formation of Salt Deposits and Climate Change
There are two periods of salt formation in the Qaidam basin: 1) the first period is in the Late Tertiary Pliocene, and 2) the second period is in the Late Quaternary Pleistocene (Zhang, 1987). The Three Lakes evolved into salt lakes in the second salt-forming period (Zhu et al., 1989).
A previous investigation by the Qaidam Integrated Geological Exploration Institute of Qinghai Province reported two periods of salt formation in East Taijnar Salt Lake, during which two thick layers of halite were deposited due to dry climate. The climate briefly changed to relatively humid during the first period of salt formation, leading to the deposition of a thin layer of clay in the middle of the lower halite layer (Figure 7). Liang and Han (2013) reported that the age of the Upper Pleistocene clay below the lower halite layer is 27 ka, indicating that the lower halite layer formed after 27 ka in East Taijnar Salt Lake. Hence, the dry climate facilitated the deposition of halite since 27 ka. The halite layer below 8.4 m in the DT section represents the top of the lower halite layer. Abundant halite deposition below 8.4 m shows that this was a period of salt formation in East Taijnar Salt Lake. Analysis of OSL dating shows that this period of salt formation lasted from 27 to 4.6 ka, during which East Taijnar Salt Lake witnessed intensely dry conditions that led to significant deposition of halite and gypsum (Figure 4A and Figure 6). Liang and Han (2013) and Zhang et al. (2001) pointed out that the salt deposits in East Taijnar Salt Lake formed about ten thousand years ago. Since the samples of this study were from the east of East Taijnar Salt Lake, it can be concluded that the age obtained by the study was from a layer of clay in the middle of the lower halite.
The assimilation of the above discussions shows the prevalence of extremely dry climate during 27–4.6 ka, which is the period of salt formation in East Taijnar Salt Lake. However, the climate briefly changed around 10 ka. An increase in relative humidity around 10 ka led to the deposition of a thin layer of clay due to increased runoff. As lithology shows, a clast layer with an average detrital mineral content of 85.1% is deposited in the DT section since 4.6 ka. This layer was deposited by the Nalinggele River. It shows that the climate was relatively humid since 4.6 ka, and the hydrodynamic conditions in the Nalingele River became stronger, which led to the transport of abundant clastic minerals. Yang (2015) reported frequent catastrophic palaeofloods by the Nalinggele River during 4.7–3.5 ka, confirming our conclusion. Although the climate became relatively humid since 4.6 ka, dry conditions still existed, which is also shown by the existence of halite (average content 6.9%) and gypsum (average content 6.7%). The progress of salt formation did not stop since 4.6 ka. From 0.3 ka, the runoff volume of the Nalinggele River decreased and East Taijnar Salt Lake evolved into a landscape as it is today.
The XTW section in West Taijnar Salt Lake is 1.7 m thick. We extracted quartz from the bottom of the section for dating (Figure 4B). Dating results and frequent cases of halite deposition show that the climate was dry during 1.64–0.67 ka. Section XT2-1 in West Taijnar Salt Lake is dated using OSL and radiocarbon dating (AMS 14C) (Wang et al., 2019). As the results of their study show, West Taijnar Salt Lake deposited a large quantity of halite during 8.33–0.25 ka (Figure 4C), which is similar to the deposition in East Taijnar Salt Lake. However, West Taijnar Salt Lake has fewer clast minerals. East Taijnar Salt Lake receives a larger inflow from the Nalinggele River than West Taijnar Salt Lake, reducing the transport of clast to the latter. The depth of the section YLP in Yilingping Lake is approximately 2.7 m. Data on dating and large halite deposition show that Yiliping Salt Lake is dry since 38.09 ka.
To summarize, the period of salt formation in East Taijnar Salt Lake and West Taijnar Salt Lake began 27 ka ago. During this period, the climate was extremely dry. At the beginning of the period (27–4.6 ka), cold and dry climatic conditions were maintained. The humid climate occurred around 10 ka. In the later stages of the salt formation period (after 4.6 ka ago), the climate was relatively humid, but remained dry. The salt-forming age of Yiliping Salt Lake is 38.09 ka, which testifies to a dry climate since 38.09 ka.
Comparison With Climate in Arid Central Asia and Monsoon Asia
The Qaidam Basin lies close to the border between the Asian Summer Monsoon (ASM) and the mid-latitude westerlies (Chen et al., 2008). Sediments from the Three Lakes in the transition zone between arid Central Asia and monsoon Asia provide insight into the controlling influence of the summer monsoon and the westerlies on the climate in the central Qaidam Basin.
Figure 9 shows the synthesized Holocene effective-moisture evolution in arid central Asia (ACA) and monsoon Asia. ACA as a whole experienced synchronous and coherent changes in moisture during the Holocene, namely the dry early Holocene, the wetter (less dry) early to mid-Holocene, and the moderately wet late Holocene (Chen et al., 2008). As discussed in Comparison With Climate in Arid Central Asia and Monsoon Asia Section the dry climate in the early Holocene and relatively humid climate in the late Holocene in the Three Lakes area is similar to the effective moisture history in ACA. The effective-moisture history in monsoonal Asia is out-of-phase with that of the ACA, as shown in Figure 9. Thus, we can conclude that the Three Lakes area is mainly controlled by the westerlies in the Holocene.
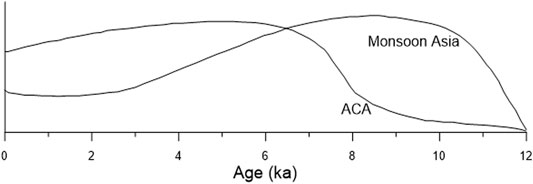
FIGURE 9. The synthesized Holocene effective-moisture evolution in arid Central Asia (ACA) and monsoon Asia (Chen et al., 2008).
Geomorphic Processes since the Late Pleistocene
In the early Late Pleistocene, the Three Lakes were located in the same secondary basin, as evidenced by the consistency of the bottom sediments of the late Pleistocene and the continuity of the stratigraphic distribution (Rong, 2002). Due to the uplift of the Bayanwuer anticline and the Taijnar anticline, as well as other anticlines after that, Yiliping Salt Lake changed into an independent basin and deposited the upper salt layer since the middle-late Pleistocene. This separation explains the differences in depositions between Yiliping Salt Lake, West Taijnar Salt Lake and East Taijnar Salt Lake since the late Pleistocene. Wang (2020) indicated the age of formation of the upper halite at 40 ka. Combining this with our dating results from the YLP section, we conclude that the upper halite began to form at 40 ka. Yiliping Salt Lake evolved into an independent basin at 40 ka. In contrast, East Taijnar Salt Lake and Wset Taijnar Salt Lake were a unitive basin fed by the Nalinggele River. Unitive deposition of East Taijnar Salt Lake and West Taijnar Salt Lake was halted by tectonic activity between the late Pleistocene and Holocene, leading to the division of the unitive basin into two lakes in the Holocene (Zhang, 1987; Zhu et al., 1989). The top layer of the late Pleistocene sediments is exposed to the east of East Taijnar Salt Lake (Figure 7B). This means that the central and thickest part of the late Pleistocene deposits is between East Taijnar Salt Lake and West Taijnar Salt Lake, which shows that these two lakes were not divided before the late Pleistocene. Liang and Han (2013) reported that the age of the top layer of the late Pleistocene sediments is 27 ka. Therefore, East Taijnar Salt Lake and West Taijnar Salt Lake partitioned at 27 ka, and the lower layer of salt was deposited until the Holocene. The climate in the Holocene evolved from dry to relatively humid, facilitating the transport and deposition of clastic sediments in East Taijnar Salt Lake and West Taijnar Salt Lake (Figure 4). Since 4 ka, East Taijnar Salt Lake and West Taijnar Salt Lake deposited the upper salt layer due to dry climate.
Conclusion
By combining the results of OSL dating of sections in the Three Lakes with previous studies related to other salt lakes, we conclude that salt crusts in the Qaidam basin have different thicknesses. The thickness of the salt crust in the Three Lakes is less than 0.2 m, which is thinner than the salt crust in the western Qaidam basins, such as Chahansilatu and Dalangtan salt lake. The salt crust in the salt lakes Chahansilatu, Dalangtan and Kunteyi was formed earlier than the salt crust of the Three Lakes.
The confined lithium deposits in East Taijnar Salt Lake were formed before 27–4.6 ka. In comparison, the age of the phreatic lithium deposits in East Taijnar Salt Lake is at most 4.6 ka. Given that the evolutionary processes of West Taijnar Salt Lake and East Taijnar Salt Lake were identical, it could be assumed that the age of the lithium deposits in the former could be 27 ka. The age of the lithium deposit in Yiliping Salt Lake is 40 ka. The brine lithium deposits in South America are older than the corresponding deposits in the Qaidam Basina and probably formed between the middle Pleistocene and late Pleistocene. The youngest lithium deposits in Tibet formed around 4 ka.
According to the OSL dating and the mineral composition analysis of the Three Lakes, the period of salt formation in East Taijnar Salt Lake and West Taijnar Salt Lake began 27 ka ago. The climate was extremely dry during this period. The climatic conditions in the beginning (27–4 ka) could be characterized as cold and dry. A brief, relatively humid event occurred around 10 ka. During the later stages in the period of salt formation (after 4 ka ago), the conditions were relatively humid in the two lakes. However, the climate was predominantly dry as suggested by the crystallization of halite and gypsum. The salt-forming age of Yiliping Salt Lake is 38.09 ka, which has had a dry climate since 38.09 ka. A comparison of the climate with the synthesized Holocene effective-moisture evolution in arid Central Asia and monsoon Asia shows that the Three Lakes area is mainly controlled by the westerlies in the Holocene.
East Taijnar Salt Lake, West Taijnar Salt Lake, and Yiliping Salt Lake were situated in the same secondary basin during the late Pleistocene. By 40 ka, Yiliping Salt Lake evolved into an independent basin due to tectonic activity. The unitive deposition of East Taijnar Salt Lake and West Taijnar Salt Lake was completed gradually from the later period of the late Pleistocene. These two lakes partitioned completely at 27 ka, and then they deposited the lower layers of salt until the Holocene. The Nalinggele River brought abundant detrital minerals during the Holocene, especially in East Taijnar Salt Lake, which led to a brief desalination of the lake. The upper salt layer was deposited in East Taijnar Salt Lake and West Taijnar Salt Lake due to the extremely dry climate during the Holocene.
Data Availability Statement
The original contributions presented in the study are included in the article/Supplementary Material, further inquiries can be directed to the corresponding author.
Author Contributions
ZM designed the research. ZM, FH, TC, LY, XL, FC, XL, and WY performed the research. ZM and TC analyzed the data. ZM, FH, and TC wrote the manuscript.
Funding
This study was supported by the Natural Science Foundation of Qinghai Province (2019-ZJ-911) and the Natural Science Foundation of Qinghai Province (2020-ZJ-939Q).
Conflict of Interest
The authors declare that the research was conducted in the absence of any commercial or financial relationships that could be construed as a potential conflict of interest.
Acknowledgments
We thank reviewers for valuable suggestions and comments related to the manuscript. We also thank Li Han for help in the XRD analysis and Yixuan Wang for help in analyzing the OSL data.
Supplementary Material
The Supplementary Material for this article can be found online at: https://www.frontiersin.org/articles/10.3389/feart.2021.702223/full#supplementary-material
References
Abbott, M. B., Seltzer, G. O., Kelts, K. R., and Southon, J. (1997). Holocene Paleohydrology of the Tropical Andes from Lake Records. Quat. Res. 47 (1), 70–80. doi:10.1006/qres.1996.1874
An, F., Ma, H., Wei, H., and Lai, Z. (2012). Distinguishing Aeolian Signature from Lacustrine Sediments of the Qaidam Basin in Northeastern Qinghai-Tibetan Plateau and its Palaeoclimatic Implications. Aeolian Res. 4, 17–30. doi:10.1016/j.aeolia.2011.12.004
Baker, P. A., Rigsby, C. A., Seltzer, G. O., Fritz, S. C., Lowenstein, T. K., Bacher, N. P., et al. (2001a). Tropical Climate Changes at Millennial and Orbital Timescales on the Bolivian Altiplano. Nature 409 (6821), 698–701. doi:10.1038/35055524
Baker, P. A., Seltzer, P. G., Fritz, S. C., Dunbar, R. B., Grove, M., Tapia, P., et al. (2001b). The History of South American Tropical Precipitation for the Past 25,000 Years. Science 291 (5504), 640–643. doi:10.1126/science.291.5504.640
Bobst, A., Lowenstein, T. K., Jordan, T. E., Godfrey, L. V., Ku, T. L., and Luo, S. D. (2001). A 106 kapaleoclimate record from the Salar de Atacama, northern Chile. Palaeogeogr. Palaeoclimatol. Palaeoecol. 173 (1-2), 21–42. doi:10.1016/S0031-0182(01)00308-X
Chang, Q., Lai, Z., An, F., Wang, H., Lei, Y., and Han, F. (2017). Chronology for Terraces of the Nalinggele River in the north Qinghai-Tibet Plateau and Implications for Salt lake Resource Formation in the Qaidam Basin. Quat. Int. 430 (PT.B), 12–20. doi:10.1016/j.quaint.2016.02.022
Chen, A. D., Zheng, M. P., Shi, L. F., Wang, H. L., Xu, J. M., and Yuan, W. H. (2017). Gypsum 230Th Dating of the 15YZK01 Drilling Core in the Qaidam Basin: Salt Deposits and Their Link to Quaternary Glaciation and Tectonic Movement. Acta Geosci. Sin. 38 (4), 494–504. (in Chinese with English abstract). doi:10.3975/cagsb.2017.04.06
Chen, F., Yu, Z., Yang, M., Ito, E., Wang, S., Madsen, D. B., et al. (2008). Holocene Moisture Evolution in Arid central Asia and its Out-of-phase Relationship with Asian Monsoon History. Quat. Sci. Rev. 27 (3-4), 351–364. doi:10.1016/j.quascirev.2007.10.017
Chung, F. H. (1974). Quantitative Interpretation of X-ray Diffraction Patterns of Mixtures. II. Adiabatic Principle of X-ray Diffraction Analysis of Mixtures. J. Appl. Cryst. 7 (6), 526–531. doi:10.1107/S0021889874010387
Diederich, J. L., Wennrich, V., Bao, R., Büttner, C., Bolten, A., Brill, D., et al. (2020). A 68 Ka Precipitation Record from the Hyperarid Core of the Atacama Desert in Northern Chile. Glob. Planet. Change 184, 103054. doi:10.1016/j.gloplacha.2019.103054
Duller, G. A. T. (2003). Distinguishing Quartz and Feldspar in Single Grain Luminescence Measurements. Radiat. Measurements 37 (2), 161–165. doi:10.1016/S1350-4487(02)00170-1
Ericksen, G. E., Vine, J. D., and Raul Ballón, A. (1978). Chemical composition and distribution of lithium-rich brines in salar de Uyuni and nearby salars in southwestern Bolivia. Energy 3 (3), 355–363. doi:10.1016/0360-5442(78)90032-4
Fan, Q., Lai, Z., Long, H., Sun, Y., and Liu, X. (2010). OSL Chronology for Lacustrine Sediments Recording High Stands of Gahai Lake in Qaidam Basin, Northeastern Qinghai-Tibetan Plateau. Quat. Geochronol. 5 (2-3), 223–227. doi:10.1016/j.quageo.2009.02.012
Fan, Q., Ma, H., Ma, Z., Wei, H., and Han, F. (2013). An Assessment and Comparison of 230Th and AMS 14C Ages for Lacustrine Sediments from Qarhan Salt Lake Area in Arid Western China. Environ. Earth Sci. 71, 1227–1237. doi:10.1007/s12665-013-2526-5
Fornari, M., Risacher, F., and Féraud, G. (2001). Dating of Paleolakes in the central Altiplano of Bolivia. Palaeogeogr. Palaeoclimatol. Palaeoecol. 172 (3-4), 269–282. doi:10.1016/S0031-0182(01)00301-7
Garrett, D. E. (2004). Handbook of Lithium and Natural Calcium Chloride: Their Deposits, Processing, Uses and Properties. Oxford, England: Elsevier Academic Press. doi:10.1016/B978-0-12-276152-2.X5035-X
Gruber, P. W., Medina, P. A., Keoleian, G. A., Kesler, S. E., Everson, M. P., and Wallington, T. J. (2011). Global Lithium Availability. J. Ind. Ecol. 15, 760–775. doi:10.1111/j.1530-9290.2011.00359.x
Han, W., Ma, Z., Lai, Z., Appel, E., Fang, X., and Yu, L. (2014). Wind Erosion on the north-eastern Tibetan Plateau: Constraints from OSL and U-Th Dating of Playa Salt Crust in the Qaidam Basin. Earth Surf. Process. Landforms 39, 779–789. doi:10.1002/esp.3483
Huang, Q., and Han, F. Q. (2007). Evolution of Salt Lakes and Palaeoclimate Fluctuation in Qaidam Basin. Beijing: Science Press. in Chinese with English abstract
Kesler, S. E., Gruber, P. W., Medina, P. A., Keoleian, G. A., Everson, M. P., and Wallington, T. J. (2012). Global Lithium Resources: Relative Importance of Pegmatite, Brine and Other Deposits. Ore Geology. Rev. 48, 55–69. doi:10.1016/j.oregeorev.2012.05.006
Klein, A. G., Seltzer, G. O., and Isacks, B. L. (1999). Modern and Last Local Glacial Maximum Snowlines in the Central Andes of Peru, Bolivia, and Northern Chile. Quat. Sci. Rev. 18 (1), 63–84. doi:10.1016/S0277-3791(98)00095-X
Lai, Z., and Brückner, H. (2008). Effects of Feldspar Contamination on Equivalent Dose and the Shape of Growth Curve for OSL of Silt-Sized Quartz Extracted from Chinese Loess. Geochronometria 30 (1), 49–53. doi:10.2478/v10003-008-0010-0
Lai, Z., Mischke, S., and Madsen, D. (2014). Paleoenvironmental Implications of New OSL Dates on the Formation of the "Shell Bar" in the Qaidam Basin, Northeastern Qinghai-Tibetan Plateau. J. Paleolimnol. 51 (2), 197–210. doi:10.1007/s10933-013-9710-1
Lai, Z. P., Zöller, L., Fuchs, M., and Brückner, H. (2008). Alpha Efficiency Determination for OSL of Quartz Extracted from Chinese Loess. Radiat. Measurements 43, 767–770. doi:10.1016/j.radmeas.2008.01.022
Lai, Z. (2006). Testing the Use of an OSL Standardised Growth Curve (SGC) for Determination on Quartz from the Chinese Loess Plateau. Radiat. Measurements 41, 9–16. doi:10.1016/j.radmeas.2005.06.031
Liang, Q. S., and Han, F. Q. (2013). Geological Characteristics and Lithium Distribution of East Taijinar Salt Lake in Qaidam Basin. J. Salt Lake Res. 21 (3), 1–9. (in Chinese with English abstract)
Liang, Q. S., and Huang, Q. (1995). Salt-forming Ages of the Dabusun and Bieletan Regions in Qarhan Playa, Qinghai. Acta Sedimentol. Sin. 13 (3), 126–131. (in Chinese with English abstract). doi:10.14027/j.cnki.cjxb.1995.03.015
Liu, X. F., and Zheng, M. P. (1999). Geological Setting and Mineralizing Evolution of Super Large Li, B deposit, Zabuye, Tibet. Geol. Chem. Miner. 21 (2), 65–68. (in Chinese with English abstract)
Ma, N. N., Zheng, M. P., Ma, Z. B., Chen, W. X., Kong, F., and Shi, L. F. (2011). Forming Age of Surface Mirabilite in Dalangtan, Qaidam Basin and its Environmental Significance. Acta Geol. Sin. 85 (3), 433–444. (in Chinese with English abstract). doi:10.3724/SP.J.1011.2011.00415
Murray, A. S., and Wintle, A. G. (2000). Luminescence Dating of Quartz Using an Improved Single-Aliquot Regenerative-Dose Protocol. Radiat. Measurements 32 (1), 57–73. doi:10.1016/S1350-4487(99)00253-X
Owen, L. A., Finkel, R. C., Haizhou, M., and Barnard, P. L. (2006). Late Quaternary Landscape Evolution in the Kunlun Mountains and Qaidam Basin, Northern Tibet: A Framework for Examining the Links between Glaciation, lake Level Changes and Alluvial Fan Formation. Quat. Int. 154-155, 73–86. doi:10.1016/j.quaint.2006.02.008
Pfeiffer, M., Latorre, C., Santoro, C. M., Gayó, E. M., Rojas, R., Carrevedo, M. L., et al. (2018). Chronology, Stratigraphy and Hydrological Modelling of Extensive Wetlands and Paleolakes in the Hyperarid Core of the Atacama Desert during the Late Quaternary. Quat. Sci. Rev. 197, 224–245. doi:10.1016/j.quascirev.2018.08.001
Prescott, J. R., and Hutton, J. T. (1994). Cosmic ray Contributions to Dose Rates for Luminescence and ESR Dating: Large Depths and Long-Term Time Variations. Radiat. Measurements 23, 497–500. doi:10.1016/1350-4487(94)90086-8
Ree-Jones, J. (1995). Optical Dating of Young Sediments Using Fine-Grain Quartz. Anc.TL. 13, 9–13. doi:10.1108/02632779510104021
Roberts, H. M., and Duller, G. A. T. (2004). Standardised Growth Curves for Optical Dating of Sediment Using Multiple-Grain Aliquots. Radiat. Measurements 38 (2), 241–252. doi:10.1016/j.radmeas.2003.10.001
Rodbell, D. T., Seltzer, G. O., Anderson, D. M., Abbott, M. B., Enfield, D. B., and Newman, J. H. (1999). An 15,000-Year Record of El Niño-Driven Alluviation in Southwestern Ecuador. Science 283 (5401), 516–520. doi:10.1126/science.283.5401.516
Shen, Z. S., Cheng, G., Yue, C. S., Liu, S. Q., Zhang, F. S., Wang, Q., et al. (1993). The Division and Sedimentary Environment of Quaternary Salt-Bearing Strata in Qaidam Basin. Beijing: Geological Publishing House. in Chinese with English abstract
Sun, Y., Lai, Z., Long, H., Liu, X., and Fan, Q. (2010). Quartz OSL Dating of Archaeological Sites in Xiao Qaidam Lake of the NE Qinghai-Tibetan Plateau and its Implications for Palaeoenvironmental Changes. Quat. Geochronol. 5 (2-3), 360–364. doi:10.1016/j.quageo.2009.02.013
Thompson, L. G., Davis, M. E., Mosley-Thompson, E., Sowers, T. A., Henderson, K. A., Zagorodnov, V., et al. (1998). A 25,000-Year Tropical Climate History from Bolivian Ice Cores. Science 282 (5398), 1858–1864. doi:10.1126/science.282.5395.1858
Walk, J., Stauch, G., Reyers, M., Vásquez, P., Sepúlveda, F. A., Bartz, M., et al. (2020). Gradients in Climate, Geology, and Topography Affecting Coastal Alluvial Fan Morphodynamics in Hyperarid Regions - the Atacama Perspective. Glob. Planet. Change 185, 102994. doi:10.1016/j.gloplacha.2019.102994
Wang, M. Q. (2020). Origin of Lithium-Rich Brine in Yiliping Salt lake, Qaidam Basin. PhD Thesis ((Beijing: China University of Geosciences). in Chinese with English abstract
Wang, Q. S., Qiu, J. Z., Shao, H. N., and Xu, H. (2015). Analysis on Metallogenic Characteristic and Resource Potential of Salt lake Brine Lithium Deposits in the Global. China Mining Mag. 24 (11), 82–88. (in Chinese with English abstract). doi:10.3969/j.issn.1004-4051.2015.11.018
Wang, Y. X., Chen, T. Y., Wu, C., Lai, Z. P., Guo, S. D., and Cong, L. (2019). Formation and Evolution of the Xitaijinair Salt Lake in Qaidam Basin Revealed by Chronology. Arid land Geogr. 42 (4), 876–884. (in Chinese with English abstract)
Yang, H. P., Liu, L., and Ding, G. F. (2019). Present Situation and Development Trend of Lithium Resources in the World. Conservation Utilization Mineral. Resour. 5, 26–40. (in Chinese with English abstract). doi:10.13779/j.cnki.issn1001-0076.2019.05.004
Yang, X. Y. (2015). Modern Flood Process and the OSL Dating and Grain-Size Characteristic on Palaeoflood Deposits of Nalinggele Watershed, Northern of the Qinghai-Tibet Plateau. Master thesis. (Xining (China): Qinghai Normal University. in Chinese with English abstract
Yu, J., Gao, C., Cheng, A., Liu, Y., Zhang, L., and He, X. (2013). Geomorphic, Hydroclimatic and Hydrothermal Controls on the Formation of Lithium Brine Deposits in the Qaidam Basin, Northern Tibetan Plateau, China. Ore Geology. Rev. 50, 171–183. doi:10.1016/j.oregeorev.2012.11.001
Yu, L., and Lai, Z. (2012). OSL Chronology and Palaeoclimatic Implications of Aeolian Sediments in the Eastern Qaidam Basin of the Northeastern Qinghai-Tibetan Plateau. Palaeogeogr. Palaeoclimatol. Palaeoecol. 337-338, 120–129. doi:10.1016/j.palaeo.2012.04.004
Yu, L. P., and Lai, Z. P. (2014). Holocene Climate Change Inferred from Stratigraphy and OSL Chronology of Aeolian Sediments in the Qaidam Basin, Northeastern Qinghai–Tibetan Plateau. Quat. Res. 81 (3), 488–499. doi:10.1016/j.yqres.2013.09.006
Yuan, J. Q., Huo, C. Y., and Cai, K. Q. (1983). The High Mountain-Deep basin saline Environment – A New Cenetic Model of Salt Deposits. Geol. Rev 29 (2), 159–165. (in Chinese with English abstract).
Zeng, F., and Xiang, S. (2017). Geochronology and mineral Composition of the Pleistocene Sediments in Xitaijinair Salt Lake Region, Qaidam Basin: Preliminary Results. J. Earth Sci. 28 (4), 622–627. doi:10.1007/s12583-016-0712-6
Zhang, H. C., Lei, G. L., and Chang, F. Q. (2007). Age Determination of Shell Bar Section in Salt Lake Qarhan, Qaidam Basin. Quat. Sci. 27 (4), 511–521. (in Chinese with English abstract). doi:10.3321/j.issn:1001-7410.2007.04.006
Zhang, H., Han, F. Q., and Liang, Q. S. (2001). Preliminary Studies on Deposition Characteristics and Ages of the Salt Sediments of Dongtai Salt Lake in Qinghai Province. Geol. Chem. Miner. 23 (2), 83–85. (in Chinese with English abstract). doi:10.3969/j.issn.1006-5296.2001.02.003
Zhang, M., and Liu, X. (2020). Climate Changes in the Qaidam Basin in NW China over the Past 40 Kyr. Palaeogeogr. Palaeoclimatol. Palaeoecol. 551, 109679. doi:10.1016/j.palaeo.2020.109679
Zhang, P. X. (1987). The Salt Lakes of the Qaidam Basin. Beijing: Science Press. in Chinese with English abstract
Zhang, S. J., Cui, L. W., Kong, L. H., Jiang, A. L., and Li, J. B. (2020). Summarize on the mineral Resources and Their Distribution at home and Abroad. Noferrous Met. Eng. 10 (10), 95–104. (in Chinese with English abstract). doi:10.3390/met10060767
Keywords: qaidam basin, optically stimulated luminescence, lithium deposits, evolution of lake, paleoclimate
Citation: Ma Z, Han F, Chen T, Yi L, Lu X, Chen F, Liu X and Yuan W (2021) The Forming Age and the Evolution Process of the Brine Lithium Deposits in the Qaidam Basin Based on Geochronology and Mineral Composition. Front. Earth Sci. 9:702223. doi: 10.3389/feart.2021.702223
Received: 29 April 2021; Accepted: 21 June 2021;
Published: 12 July 2021.
Edited by:
Xiangzhong Li, Yunnan University, ChinaReviewed by:
Shugang Kang, State Key Laboratory of Loess and Quaternary Geology, Institute of Earth Environment (CAS), ChinaGuoqiang Li, Lanzhou University, China
Copyright © 2021 Ma, Han, Chen, Yi, Lu, Chen, Liu and Yuan. This is an open-access article distributed under the terms of the Creative Commons Attribution License (CC BY). The use, distribution or reproduction in other forums is permitted, provided the original author(s) and the copyright owner(s) are credited and that the original publication in this journal is cited, in accordance with accepted academic practice. No use, distribution or reproduction is permitted which does not comply with these terms.
*Correspondence: Fengqing Han, aGFuZnFAaXNsLmFjLmNu; Tianyuan Chen, Y2hlbi50aWFueXVhbkBmb3htYWlsLmNvbQ==