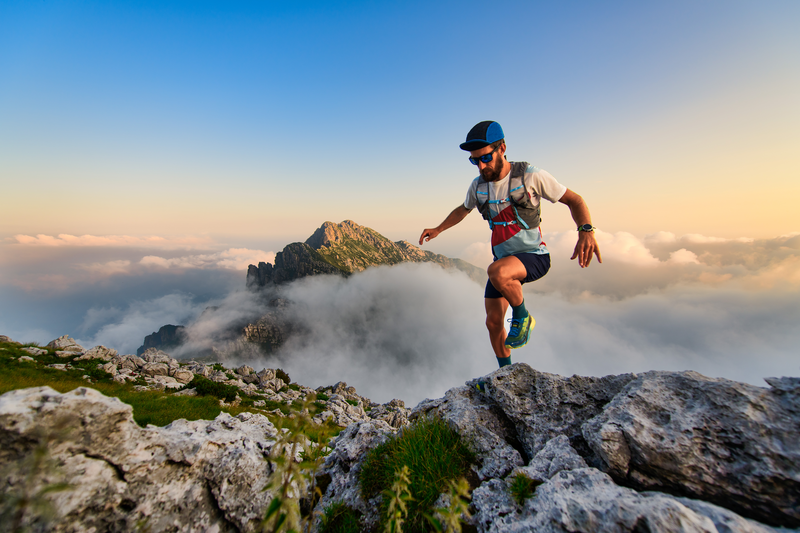
94% of researchers rate our articles as excellent or good
Learn more about the work of our research integrity team to safeguard the quality of each article we publish.
Find out more
ORIGINAL RESEARCH article
Front. Earth Sci. , 06 July 2021
Sec. Geochemistry
Volume 9 - 2021 | https://doi.org/10.3389/feart.2021.699365
This article is part of the Research Topic Hydrobiogeochemistry of Major Asian Rivers View all 11 articles
Arctic tundra wetlands may be an important source of dissolved organic carbon (DOC) in Arctic rivers and the Arctic Ocean under global warming. We investigated stable water isotopes and DOC concentration in wetlands, tributaries, and the mainstream at the lower reaches of the Indigirka River in northeastern Siberia during the summers of 2010–2014 to assess the complex hydrology and role of wetlands as sources of riverine DOC. The wetlands had higher values of δ18O and DOC concentration than the tributaries and mainstream of the Indigirka River. A relationship between the two parameters was observed in the wetlands, tributaries, and mainstream, suggesting the wetlands can be a source of DOC for the mainstream through the tributaries. The combined temporal variations in riverine δ18O and DOC concentration indicate the mainstream water flowed into the tributaries during relatively high river-level periods in summer, whereas high DOC water in the downstream wetlands could be discharged to the mainstream through the tributaries during the low river-level periods. A minor fraction (7–13%) of riverine and wetland DOC was degraded during 40 days of dark incubation. Overall, the downstream wetlands potentially provide relatively less biodegradable DOC to the Arctic river and costal ecosystem during the low river-level periods—from late summer to autumn.
The pedosphere of the Arctic has substantial stocks of organic carbon and plays an important role in the global carbon cycle as sources and sinks of CO2 and CH4, which are affected by global warming (McGuire et al., 2009; McGuire et al., 2010b). Arctic rivers transport large amounts of organic carbon, mostly in the form of dissolved organic carbon (DOC), from the Arctic pedosphere to the Arctic Ocean (Gordeev et al., 1996; Dittmar and Kattner, 2003; Rachold et al., 2004; Raymond et al., 2007; McClelland et al., 2012). Although the Arctic Ocean corresponds to only 1% of the global ocean volume, it receives more than 10% of both global river discharge and DOC, namely 25–36 TgCyr−1 (Lobbes, 2000; Lammers et al., 2001; Raymond et al., 2007; Holmes et al., 2012). In the future, riverine DOC concentration and flux are predicted to increase due to Arctic warming (Frey and Smith, 2005); yet, some proportion of the DOC in Arctic rivers is known to be degraded by microbes (e.g., Köhler et al., 2002; Holmes et al., 2012; Stanley et al., 2016). Thus, the transport of DOC from the Arctic pedosphere to the Arctic Ocean by rivers is possibly an important process controlling not only organic carbon stocks in soil but also marine ecosystems, in particular microbial loops.
The transport of DOC from the pedosphere to the river networks of Arctic watersheds is unique because of the presence of permafrost. The terrestrial runoff carries DOC derived from organic-rich surface soil and fresh vegetation in boreal forests (Finlay et al., 2006; Amon et al., 2012) to rivers during the spring because the frozen ground limits the infiltration of rain and snowmelt. Therefore, the river discharge and riverine DOC concentration generally reach their maxima during the snowmelt season in Arctic rivers (Cooper et al., 2008; Prokushkin et al., 2009, 2011; Holmes et al., 2008, 2012). After the peak of river discharge, the DOC concentration generally decreases correspondingly with the decrease in discharge (Cooper et al., 2008; Prokushkin et al., 2009, 2011; Holmes et al., 2008, 2012), a phenomenon which can be attributed to the longer flow path of the infiltration water, created by seasonal deepening of the active layer. This longer flow path allows DOC decomposition and/or adsorption onto mineral particles in the soil before leaching into the stream (MacLean et al., 1999; Striegl et al., 2005; Petrone et al., 2006; Prokushkin et al., 2007).
This mechanism of decrease in DOC concentration after snowmelt is possibly less effective in the downstream tundra wetlands, which have a shallower active layer than the upstream boreal forests. In fact, Amon et al. (2012) reported that the contribution of mosses and peat bogs to Arctic river DOC significantly increased after spring (i.e., after thawing of the active layer). It has also been pointed out that the degradation of permafrost in the subsurface of the downstream peatland probably releases DOC from the permafrost into rivers, thereby increasing the DOC concentration and flux in Arctic rivers (Frey and Smith, 2005; Frey and McClelland, 2009). Therefore, knowledge regarding DOC leaching from downstream tundra wetlands to rivers is crucial for a better estimation of the DOC flux in Arctic rivers under global warming. However, downstream tundra wetlands have not received considerable attention as a DOC source, because previous studies have focused mostly on major Arctic rivers (e.g., Kattner et al., 1999; Amon et al., 2012; Holmes et al., 2012; Kicklighter et al., 2013; Pokrovsky et al., 2015) for which the watersheds cover extensive regions of boreal forests.
In this study, we evaluated the DOC concentration in the mainstream, tributaries, and wetlands at the lower reaches of the Indigirka River in summer to assess the role of the wetlands as a source of riverine DOC. The Indigirka River basin, located in northeastern Siberia, is relatively small compared with other major Arctic river basins in the continuous permafrost zone, and tundra wetlands are widely distributed in the middle and lower reaches. Therefore, the study region is suitable for evaluating the potential of wetlands as DOC sources for Arctic rivers. In addition, we used stable isotopes of water to assess the complex hydrology in the study region (Woo, 2012), that is, the occupation of tributaries by water from the mainstream during snowmelt, as well as the contribution of water from downstream tundra wetlands to tributaries after the snowmelt.
The study area is located in the downstream region of the Indigirka River near Chokurdakh (70°37'N, 147°53′E), Republic of Sakha (Yakutia), Russia (Figure 1). The upper and middle reaches of the Indigirka River basin mostly consist of taiga forest, alpine desert, and mountain tundra landscapes with an altitude of up to 2,800 m, whereas the lower reaches consist of the forest tundra and tundra landscapes with a relatively low altitude (Geodesy and Cartography Bureau of Soviet Council of Ministers, 1989; Gusev et al., 2013; Tei et al., 2020).
FIGURE 1. Location of the study area. (A) Location of the study region in northeastern Siberia (ArcGIS ESRI, Redland, CA, United States) modified by Takano et al. (2019). The Indigirka River basin boundaries are shown with a black line. (B) Satellite image of the Indigirka River lowland around the village of Chokurdakh from Landsat 8 modified by Shingubara et al. (2019). Observation points of the mainstream (CKH, B, and V) and one tributary (K) of the Indigirka River are shown. Those of the other tributaries are shown by closed white circles.
Observation and sampling were conducted in four sites (V: Verkhny Khatistakha; K: Kodac; CKH: Chokurdakh; B: Boydom), which are adjacent to the Indigirka River or its tributary. The study area is located on the taiga−tundra boundary, and tree density decreases from site V to site B, which is relatively close to the tundra landscape. Trees/shrubs and wetlands are distributed in patches in the area. The only tree species present is Siberian larch (Larix cajanderi; syn L. gmelinii) and the dominant shrubs are dwarf birches (Betula nana), dwarf willows (Salix spp.), and dwarf alders (Alnus fruticosa; syn Deschekia fruticosa). The area where the trees/shrubs grow is covered by green-mosses (including Tomentypnum nitens, Hylocomium splendens, and Aulacomnium turgidum), and the vegetation of the wetlands includes sedges (Eriophorum spp. and Carex spp.) and sphagnum-mosses (including Sphagnum balticum, S. squarrosum, and S. angustifolium). The mainstream and tributaries are surrounded by bare-ground/shrub and wetland-dominant (>50%) land cover, respectively, in the Indigirka lowland (Morozumi et al., 2019a). More details of the vegetation and dominant species in the study area are described in Morozumi et al. (2019b).
The observation area is located in the Russian Arctic and underlain by continuous permafrost with the mean annual air temperature and precipitation of −13.9°C and 208 mm, respectively, for the period of 1950–2008 according to the Baseline Meteorological Data in Siberia (BMDS) Version 5.0 (Yabuki et al., 2011). Snowmelt and active layer thawing usually start in late May to early June. The growing season is from late June to early August, with a thaw depth of approximately 20–30 cm (Shingubara et al., 2019; Takano et al., 2019). The maximum thaw depth (more than 50 cm in depth) typically occurs in the first half of September, whereas the depth of the surface organic layer is approximately 30 cm (Takano et al., 2019). Active layer freezing usually starts in the second half of September to October and freezes completely from November to December.
Field observations and sample collection were conducted during 14–July 24, 2010, 9–July 28, 2011, 25 June−August 11, 2012, 17 June−July 25, 2013, 23–April 26, 2014, and 30 June−August 2, 2014. The observation team traveled to each sampling site by motorboat in summer and snowmobile in spring.
River water samples from the Indigirka mainstream and tributaries, wetland water, precipitation, and snow cover were mainly collected in the four observation sites (Figure 1). The sampling points of river water adjacent to sites B, K, V, and CKH are hereinafter referred to as points B, K, V, and CKH, respectively. Time-series observations of the mainstream and tributary water were conducted at intervals of 2 or 3 days for isotope analysis sampling and 1 week for DOC analysis sampling. Those of the tributary water were conducted at point K, and the sampling points of the other tributary waters are shown in Figure 1B. Wetland water was collected from the surface water of the wetlands, whereas bulk snow was obtained as a core of diameter 10 cm and placed in a plastic bag to melt. The water samples for isotope analysis were stored in 3- or 6-ml glass vials. For this study, the isotopic compositions of the Indigirka mainstream and tributaries in 2011 and 2012, summer precipitation in 2012–2014, and snow cover were obtained from Takano et al. (2019).
Samples for the DOC analysis were collected in a 60 ml plastic syringe after three consecutive rinses with the sample water in each sampling point and passed through polyvinylidene difluoride (PVDF) filters (Durapore, Millipore) of pore size 0.45 µm. After three consecutive rinses with the filtrates, the final filtrates were stored in 50 ml polyethylene bottles, which were wrapped with aluminum foil and maintained frozen in dark until analysis. The syringes and bottles were pre-washed by soaking in 0.1 mol l−1 HCl for 24 h and then rinsed with Milli-Q water.
To evaluate the biodegradability of DOC, bottle incubation experiments were performed as described by Yamashita et al. (2013). Water samples were passed through PVDF filter of pore size 0.45 µm and collected in 50 ml polyethylene bottles, which were wrapped with aluminum foil; then, the samples were incubated in dark at room temperature (16–25°C). After 40 days of incubation, the samples were stored frozen in dark until analysis without any filtration. The incubation experiment was carried out with a single sample.
Stable isotope ratios of water have long been used in studies on the water cycle (e.g., Craig, 1961; Dansgaard, 1964; Craig and Gordon, 1965). They are considered to be a useful tool for determining source water and assessing hydrological processes in Arctic rivers (Welp et al., 2005; Cooper et al., 2008; Yi et al., 2010, 2012; Sugimoto and Maximov, 2012). The stable isotopic compositions of water (oxygen and hydrogen) were determined using the CO2/H2/H2O equilibration method with a mass spectrometer (MAT 253; Thermo Fisher Scientific, United States, manufactured in Germany) with a gas bench (Thermo Fisher Scientific, United States, manufactured in Germany). The isotopic compositions are expressed in delta notation relative to Vienna Standard Mean Ocean Water (VSMOW), defined as follows:
where Rsample and RVSMOW are the isotopic ratios of water (18O/16O or D/H) of the samples and VSMOW, respectively. The precision of the analysis was within ±0.2‰ for δ18O and ±2‰ for
The concentration of DOC was determined by high-temperature catalytic oxidation using a total organic carbon analyzer (TOC-VCSH, Shimadzu, Japan). The DOC concentration was calculated from the standard curve with 0, 3.6, 7.2, and 12 mg C L−1 potassium hydrogen phthalate solution, which was determined daily.
Figure 2 shows the
FIGURE 2. δD−δ18O plot grouping Indigirka mainstream and tributaries, wetlands, precipitation in summer, and snow cover with different colors and showing distributions as boxplots on the top and side panels. The Global Meteoric Water Line (GMWL; δD = 8 × δ18O+ 10) is shown as a black solid line. These data were obtained during summer in 2010–2014, and the data of snow cover were obtained in April 2014. The isotopic compositions of Indigirka mainstream and tributaries in 2011 and 2012, summer precipitation in 2012–2014, and snow cover are cited from Takano et al. (2019).
The
FIGURE 3. Time series for δ18O grouped to the Indigirka mainstream and tributary during summer in 2011–2013. The mainstream samples were observed at sampling points CKH, B, and V, and the tributary samples were observed at point K.
Figure 4 shows the box plots of DOC concentration in the Indigirka mainstream, Indigirka tributaries, and wetlands. The DOC concentration in the tributaries tended to be higher, ranging from 4.42 to 23.46 mg C L−1 (median of 9.19 mg C L−1), than that in the mainstream, which ranged from 4.20 to 9.04 mg C L−1 (median of 6.84 mg C L−1). Overall, the DOC concentration range of the tributaries was wider than that of the mainstream. The wetlands had the highest DOC concentration with the range from 12.04 to 44.43 mg C L−1 (median of 18.60 mg C L−1).
FIGURE 4. Boxplots showing the distribution of DOC concentration during summer in the Indigirka mainstream and tributaries in 2010–2013, and wetlands in 2010–2012.
The DOC concentration in the mainstream did not largely change during the observation periods of 2011 and 2012, but it showed a gradual decrease from 8.63 to 4.89 mg C L−1 during 2013 (Figure 5). The DOC concentration in the three other mainstream points CKH, B, and V was similar. However, at point B, the value was 8.47 mg C L−1 on DOY 195, and it was higher than the value of mainstream predicted from the variation trend. In 2011, the DOC concentration in the tributary observed at point K was almost the same as that in the mainstream until DOY 203, whereas the tributary showed approximately 5 mg C L−1 higher value than the mainstream on DOY 212. The DOC concentration in the tributary, ranging from 9.06 to 12.50 mg C L−1, was consistently higher than that in the mainstream, at approximately 5 mg C L−1 in 2012. In 2013, the DOC concentration in the tributary was similar to that in the mainstream until DOY 183, after which it was 9.12 mg C L−1, which was approximately 4 mg C L−1 higher than that in the mainstream, on DOY 192. Subsequently, it decreased again to a value similar to that of the mainstream by DOY 207.
FIGURE 5. Time series of DOC concentration grouped to the Indigirka mainstream and one tributary for summer observation periods in 2011–2013. The mainstream samples were observed at sampling points CKH, B, and V, and the tributary samples were observed at point K.
The DOC loss (%) after 40 days of dark incubation of the water samples was compared with the initial concentration (Figure 6). The loss in the Indigirka mainstream, ranging from 7.1 to 8.6%, was slightly lower than that in the tributaries, ranging from 7.8 to 12.6%. A plot of tributaries showed the highest initial DOC concentration of 21.3 mg C L−1; nevertheless, the DOC loss (7.8%) was not significantly different from that in the other sites. The DOC loss in one wetland water sample with a high initial DOC concentration of 18.4 mg C L−1 was 9.7%. Based on these findings, there appears to be no relationship between DOC loss and initial DOC concentration.
FIGURE 6. DOC loss in the water of the Indigirka mainstream, tributaries, and wetlands observed in 2011 after 40 days of lab incubation at room temperature, compared with the initial DOC concentration.
Generally, the δ18O of Arctic rivers rapidly decrease in spring with the highest discharge due to the inflow of snowmelt water, followed by an increase in summer due to the recession of the snowmelt water and the contribution of runoff of summer precipitation (Welp et al., 2005; Cooper et al., 2008; Yi et al., 2010, 2012; Sugimoto and Maximov, 2012). Similarly, in the Indigirka River, the water level reaches the maximum during the snowmelt season, and then gradually decreases in summer (Figure 7). For this study area, snow cover and summer precipitation with the lowest (median of −29.9‰) and highest (median of −15.3‰)
FIGURE 7. Seasonal and interannual variations in the river water level of Indigirka mainstream at CKH during 2009–2017. The data are from Tei et al. (2020). The blue line denotes the temporal average of the river water level during 2009–2017, and the red, green, and purple lines show the river water level in 2011, 2012, and 2013, respectively.
In the permafrost zone, precipitation and snowmelt water are generally limited infiltrating to the subsurface by the frozen ground during spring (Sugimoto et al., 2003), whereas during summer, they flow into the river through the subsurface owing to deepening of the seasonal thawed active layer. Therefore, wetlands and soil water in summer are assumed to be mixtures of residual snowmelt water, summer precipitation, and soil water remaining from the previous year. In this study, the
The high
Flooding commonly occurs during the snowmelt season in Arctic river lowlands (Woo, 2012). The flooding of the Indigirka River mainstream probably extends and contributes to the tributaries, as the water level of the tributary has been observed to suddenly increase even though snow melting had not yet progressed sufficiently in the tributary basin (Morozumi et al., 2020). The same trend of variation in the
According to these results, it can be considered that the mainstream water flowed into the tributaries during both flooding period and relatively high river-level period in summer. On the contrary, water in the downstream wetlands can discharge to the tributaries and mainstream when the river level is lowered, thereby contributing as a source. Namely, the contribution of drainage from the wetlands to the river becomes the largest after summer.
Overall, the wetlands showed obviously higher DOC concentration than the rivers (Figure 4). The DOC concentration in wetlands was also higher than that of the six largest Arctic rivers (Ob’, Yenisey, Lena, Kolyma, Yukon and Mackenzie), which ranged from 4.39 to 10.14 mg C L−1 in summer (from 4.35 to 11.37 mg C L−1 annual average) (Cooper et al., 2008; Amon et al., 2012; Holmes et al., 2012). Furthermore, the organic layer of the surface soil is known to store large amounts of organic carbon in the Arctic region (Hugelius et al., 2014), and in this study area, the surface organic layer is relatively thick, with a depth of approximately 30 cm (Takano et al., 2019). Therefore, the high DOC concentration in the wetlands is considered to be the result of sufficient dissolution from the soil organic carbon stored in the organic layer.
The
FIGURE 8. Relationship of δ18O and DOC concentration in the Indigirka mainstream, one tributary, and the wetlands. The mainstream samples were observed at sampling points CKH, B, and V, and the tributary samples were observed at point K.
The DOC concentration in the mainstream tended to decrease during the summer observation period in 2013 (Figure 5). This decreasing trend was also observed for other Arctic rivers after the snowmelt season (Cooper et al., 2008; Prokushkin et al., 2009, Prokushkin er al., 2011; Holmes et al., 2008, Holmes et al., 2012). This was possibly caused by a longer flow path of the infiltration water created by seasonal deepening of the active layer in boreal forests; the longer flow path allows DOC decomposition and/or adsorption onto mineral particles in the soil before leaching into the stream (MacLean et al., 1999; Striegl et al., 2005; Petrone et al., 2006; Prokushkin et al., 2007). The subarctic taiga distributed in the upper and middle reaches of the Indigirka River basin could be considered to cause a decreasing trend in the DOC concentration in the mainstream. Additionally, the wetland water with a high DOC concentration in the downstream region could not drain to the tributaries and mainstream due to the relatively high river level (Figure 7), as described for the temporal variation in
From a previous study, Amon et al. (2012) reported that the proportion of DOC derived from wetlands in Arctic riverine DOC increased after the high-flow season. Yet, in 2012, the DOC concentration in the mainstream was relatively uniform during the entire observation period (Figure 5). During that period, both
In 2011, the DOC concentration in the mainstream was also relatively uniform at approximately 8 mg C L−1 during the observation period, except on DOY 208 with 6.26 mg C L−1 DOC (Figure 5). Although it is not certain because the amount of data was small and the period was short, the recovery of DOC concentration from the depression on DOY 208 might have been caused by the drainage from the downstream wetlands through the tributaries due to a decrease in the river level (Figure 7). On the basis of this finding, it can be considered that the drainage from the downstream wetlands likely increases with a decrease in the river level; therefore, the effect of the downstream wetland water on mainstream DOC concentration potentially increases from late summer (i.e., after the decrease in river level) to autumn.
The limitation of drainage due to high river levels not only disturbs DOC release but also maintains high soil moisture during the growing season. This may have several effects such as an increase in methane emissions (Shingubara et al., 2019; Murase et al., 2020), the withering of trees at the taiga-tundra boundary (Liang et al., 2014), and thickening of the seasonally thawed active layer (Takano et al., 2019). Such biogeochemical changes can affect the overall carbon cycle in the Arctic region (McGuire et al., 2010a). For example, the thickening of the active layer (i.e., thawing of surface permafrost), in which an estimated 1,000 ± 150 Pg of organic carbon could be stored at 0–3 m depth (Hugelius et al., 2014), potentially causes not only an increase in carbon emission to the atmosphere (Turetsky et al., 2020), but also an increase in the DOC concentration in soil water and wetlands (Fouché et al., 2020). This in turn causes an increase in the Arctic riverine DOC concentration in late summer to autumn (Frey and Smith, 2005; Frey and McClelland, 2009).
Regarding the loss of riverine DOC from the river system, such a loss may be caused by photochemical and microbial degradation within the system during transport (Wiegner and Seitzinger, 2001; Yang et al., 2021). For example, in the Alaskan and Kolyma Rivers, a substantial fraction of DOC in spring freshet water (20–40%) has been found to be biodegradable, whereas only a minor fraction (less than 10%) has been found to be degraded during summer by microbes (Holmes et al., 2008; Mann et al., 2012). The high DOC lability of spring freshet water has been attributed to less degraded recently formed (<5–10 years) DOC (Neff et al., 2006; Raymond et al., 2007), derived from the organic-rich surface soil and fresh vegetation (Finlay et al., 2006; Amon et al., 2012). Additionally, a limitation of microbial processing due to cold temperatures and a relatively short residence time in the flow path may contribute to a less degraded DOC. Contrarily, the lower lability of summer riverine DOC is considered to be due to the component of a relatively old DOC (Neff et al., 2006; Raymond et al., 2007), which originates from the deeper part of the active layer where DOC decomposition and/or adsorption onto mineral particles occur during the thawing season of active layer (MacLean et al., 1999; Striegl et al., 2005; Petrone et al., 2006; Prokushkin et al., 2007). In this study, a minor fraction (7–13%) of DOC was degraded during the 40 days of dark incubation of riverine and wetland DOC observed in summer (Figure 6). This is comparable with the lability of Arctic river DOC in summer (Holmes et al., 2008; Mann et al., 2012), implying that downstream wetlands supply relatively less biodegradable DOC. Meanwhile, the predicted release of highly biodegradable DOC due to permafrost thawing has been generally established (Abbott et al., 2014; Fouché et al., 2020). Furthermore, the photochemical degradation of DOC has known to be the other important degradation process of Arctic terrestrial DOC, which in turn affects biodegradation (Mann et al., 2012; Cory et al., 2014; Ward and Cory, 2016; Ward et al., 2017). As riverine DOC affects Arctic coastal ecosystems, such as primary production, food webs, and elemental cycles (Dittmar and Kattner, 2003; Dunton et al., 2006), changes in the chemical characteristics and bio- and photo-reactivity of DOC from downstream wetlands with ongoing climatic change need to be further clarified.
We investigated the DOC concentration and stable isotopes of water in the lower reaches of the Indigirka River in northeastern Siberia during summer to assess the complex hydrology and role of wetlands as a source of Arctic riverine DOC. The
The original contributions presented in the study are included in the article/Supplementary Material, further inquiries can be directed to the corresponding author.
Conceptualization: STa, YY Data curation: STa, STe, ML, RS, and TM Formal analysis: STa Funding acquisition: AS Investigation: STa, YY, ST, ML, RS, TM, and AS Methodology: STa, YY Project administration: TM, AS Resources: TM, AS Supervision: TM, AS Validation: STa, TM, and AS Visualization: STa. Writing—original draft: STa, YY Writing—review and editing: STa, YY, STe, ML, RS, TM, TM, and AS.
This research was supported by a Grantin-Aid from the Global Center of Excellence Program “Establishment of Center for Integrated Field Environmental Science” (IFES-GCOE) funded by the Ministry of Education, Culture, Sports, Science and Technology–Japan (MEXT), Japan Science and Technology Agency (JST, Strategic International Collaborative Research Program: SICORP) EU cooperative research project “Dynamics of permafrost and methane emission in Arctic terrestrial ecosystem in Eastern Siberia,” the Green Network of Excellence (GRENE) program funded by MEXT, the COPERA (C budget of Ecosystems, Cities and Villages on Permafrost in Eastern Russian Arctic) project funded by the Belmont Forum through JST, and KAKENHI JP19H04249 by the Japan Society for the Promotion of Science.
The authors declare that the research was conducted in the absence of any commercial or financial relationships that could be construed as a potential conflict of interest.
We sincerely thank Alexander Kononov, Roman Petrov, Egor Starostin, Alexandra Alexeeva, and other members of the Institute for Biological Problems of Cryolithozone Siberian Branch of the Russian Academy of Science, Tatiana Stryukova, Sergey Ianygin, and other staff at the Allikhovsky Ulus Inspectorate of Nature Protection for supporting our fieldwork in the vicinity of Chokurdakh. We also acknowledge the help of Yumi Hoshino, Satori Nunohashi, Kanako Tanaka, Kayoko Saito, Hanae Kudo, Ruslan Shakhmatov, Aleksandr Nogovitcyn, Yuka Sazuka, and Shuji Goto in our research group at Hokkaido University. We would like to thank Editage (www.editage.com) for English language editing.
Abbott, B. W., Larouche, J. R., Jones, J. B., Bowden, W. B., and Balser, A. W. (2014). Elevated Dissolved Organic Carbon Biodegradability from Thawing and Collapsing Permafrost. J. Geophys. Res. Biogeosci. 119 (10), 2049–2063. doi:10.1002/2014jg002678
Amon, R. M. W., Rinehart, A. J., Duan, S., Louchouarn, P., Prokushkin, A., Guggenberger, G., et al. (2012). Dissolved Organic Matter Sources in Large Arctic Rivers. Geochim. Cosmochi. Acta 94, 217–237. doi:10.1016/j.gca.2012.07.015
Cooper, L. W., McClelland, J. W., Holmes, R. M., Raymond, P. A., Gibson, J. J., Guay, C. K., et al. (2008). Flow-weighted Values of Runoff Tracers (δ18O, DOC, Ba, Alkalinity) from the Six Largest Arctic Rivers. Geophys. Res. Lett. 35 (18). Article L18606. doi:10.1029/2008gl035007
Cory, R. M., Ward, C. P., Crump, B. C., and Kling, G. W. (2014). Sunlight Controls Water Column Processing of Carbon in Arctic Fresh Waters. Science 345, 925–928. doi:10.1126/science.1253119
Craig, H., and Gordon, L. I. (1965). “Deuterium and Oxygen 18 Variations in the Ocean and the marine Atmosphere,” in Proceedings of a Conference on Stable Isotopes in Oceanographic Studies and Paleotemperatures, Lischi and Figli; Pisa, Italy. Editor E. Tongiorgi (Pisa: Laboratory of Geology and Nuclear Science), 9–130.
Craig, H. (1961). ISOTOPIC VARIATIONS IN METEORIC WATERS. Science 133 (346), 1702–1703. doi:10.1126/science.133.3465.1702
Dansgaard, W. (1964). STABLE ISOTOPES IN PRECIPITATION. Tellus 16 (4), 436–468. doi:10.3402/tellusa.v16i4.8993
Dittmar, T., and Kattner, G. (2003). The Biogeochemistry of the River and Shelf Ecosystem of the Arctic Ocean: a Review. Mar. Chem. 83 (3-4), 103–120. doi:10.1016/s0304-4203(03)00105-1
Dunton, K. H., Weingartner, T., and Carmack, E. C. (2006). The Nearshore Western Beaufort Sea Ecosystem: Circulation and Importance of Terrestrial Carbon in Arctic Coastal Food Webs. Prog. Oceanography 71 (2-4), 362–378. doi:10.1016/j.pocean.2006.09.011
Finlay, J., Neff, J., Zimov, S., Davydova, A., and Davydov, S. (2006). Snowmelt Dominance of Dissolved Organic Carbon in High-Latitude Watersheds: Implications for Characterization and Flux of River DOC. Geophys. Res. Lett. 33 (10). Article L10401. doi:10.1029/2006gl025754
Fouché, J., Christiansen, C. T., Lafrenière, M. J., Grogan, P., and Lamoureux, S. F. (2020). Canadian Permafrost Stores Large Pools of Ammonium and Optically Distinct Dissolved Organic Matter. Nat. Commun. 11 (1). Article 4500. doi:10.1038/s41467-020-18331-w
Frey, K. E., and McClelland, J. W. (2009). Impacts of Permafrost Degradation on Arctic River Biogeochemistry. Hydrol. Process. 23 (1), 169–182. doi:10.1002/hyp.7196
Frey, K. E., and Smith, L. C. (2005). Amplified Carbon Release from Vast West Siberian Peatlands by 2100. Geophys. Res. Lett. 32 (9). doi:10.1029/2004gl022025), Article L09401
Geodesy and Cartography Bureau of Soviet Council of Ministers (1989). Atlas of Yakutia. Moscow: Geodesy and Cartography Bureau of Soviet Council of Ministers.
Gordeev, V. V., Martin, J. M., Sidorov, I. S., and Sidorova, M. V. (1996). A Reassessment of the Eurasian River Input of Water, Sediment, Major Elements, and Nutrients to the Arctic Ocean. Am. J. Sci. 296 (6), 664–691. doi:10.2475/ajs.296.6.664
Gusev, E. M., Nasonova, O. N., Dzhogan, L. Y., and Aizel’, G. V. (2013). Modeling Streamflow of the Olenek and Indigirka Rivers Using Land Surface Model SWAP. Water Resour. 40 (5), 535–543. doi:10.1134/S0097807813030056
Holmes, R. M., McClelland, J. W., Peterson, B. J., Tank, S. E., Bulygina, E., Eglinton, T. I., et al. (2012). Seasonal and Annual Fluxes of Nutrients and Organic Matter from Large Rivers to the Arctic Ocean and Surrounding Seas. Estuaries Coasts 35 (2), 369–382. doi:10.1007/s12237-011-9386-6
Holmes, R. M., McClelland, J. W., Raymond, P. A., Frazer, B. B., Peterson, B. J., and Stieglitz, M. (2008). Lability of DOC Transported by Alaskan Rivers to the Arctic Ocean. Geophys. Res. Lett. 35 (3). Article L03402 doi:10.1029/2007gl032837
Hugelius, G., Strauss, J., Zubrzycki, S., Harden, J. W., Schuur, E. A. G., Ping, C.-L., et al. (2014). Estimated Stocks of Circumpolar Permafrost Carbon with Quantified Uncertainty Ranges and Identified Data Gaps. Biogeosciences 11 (23), 6573–6593. doi:10.5194/bg-11-6573-2014
Kattner, G., Lobbes, J. M., Fitznar, H. P., Engbrodt, R., Nothig, E. M., and Lara, R. J. (1999). Tracing Dissolved Organic Substances and Nutrients from the Lena River through Laptev Sea (Arctic). Mar. Chem. 65 (1-2), 25–39. doi:10.1016/s0304-4203(99)00008-0
Kicklighter, D. W., Hayes, D. J., McClelland, J. W., Peterson, B. J., McGuire, A. D., and Melillo, J. M. (2013). Insights and Issues with Simulating Terrestrial DOC Loading of Arctic River Networks. Ecol. Appl. 23 (8), 1817–1836. doi:10.1890/11-1050.1
Köhler, S., Buffam, I., Jonsson, A., and Bishop, K. (2002). Photochemical and Microbial Processing of Stream and Soil Water Dissolved Organic Matter in a Boreal Forested Catchment in Northern Sweden. Aquat. Sci. 64 (3), 269–281. doi:10.1007/s00027-002-8071-z
Lammers, R. B., Shiklomanov, A. I., Vörösmarty, C. J., Fekete, B. M., and Peterson, B. J. (2001). Assessment of Contemporary Arctic River Runoff Based on Observational Discharge Records. J. Geophys. Res. 106 (D4), 3321–3334. doi:10.1029/2000jd900444
Liang, M., Sugimoto, A., Tei, S., Bragin, I. V., Takano, S., Morozumi, T., et al. (2014). Importance of Soil Moisture and N Availability to Larch Growth and Distribution in the Arctic Taiga-Tundra Boundary Ecosystem, Northeastern Siberia. Polar Sci. 8 (4), 327–341. doi:10.1016/j.polar.2014.07.008
Lobbes, J. M., Fitznar, H. P., and Kattner, G. (2000). Biogeochemical Characteristics of Dissolved and Particulate Organic Matter in Russian Rivers Entering the Arctic Ocean. Geochimica Et Cosmochimica Acta 64 (17), 2973–2983. doi:10.1016/s0016-7037(00)00409-9
Mann, P. J., Davydova, A., Zimov, N., Spencer, R. G. M., Davydov, S., Bulygina, E., et al. (2012). Controls on the Composition and Lability of Dissolved Organic Matter in Siberia's Kolyma River basin. J. Geophys. Res. 117. Article G01028. doi:10.1029/2011jg001798
McClelland, J. W., Holmes, R. M., Dunton, K. H., and Macdonald, R. W. (2012). The Arctic Ocean Estuary. Estuaries Coasts 35 (2), 353–368. doi:10.1007/s12237-010-9357-3
McGuire, A. D., Anderson, L. G., Christensen, T. R., Dallimore, S., Guo, L., Hayes, D. J., et al. (2009). Sensitivity of the Carbon Cycle in the Arctic to Climate Change. Ecol. Monogr. 79 (4), 523–555. doi:10.1890/08-2025.1
McGuire, A. D., Hayes, D. J., Kicklighter, D. W., Manizza, M., Zhuang, Q., Chen, M., et al. (2010a). An Analysis of the Carbon Balance of the Arctic Basin from 1997 to 2006. Tellus B: Chem. Phys. Meteorology 62 (5), 455–474. doi:10.1111/j.1600-0889.2010.00497.x
McGuire, A. D., Macdonald, R. W., Schuur, E. A., Harden, J. W., Kuhry, P., Hayes, D. J., et al. (2010b). The Carbon Budget of the Northern Cryosphere Region. Curr. Opin. Environ. Sustainability 2 (4), 231–236. doi:10.1016/j.cosust.2010.05.003
Morozumi, T., Shingubara, R., Murase, J., Nagai, S., Kobayashi, H., Takano, S., et al. (2019a). Usability of Water Surface Reflectance for the Determination of Riverine Dissolved Methane during Extreme Flooding in Northeastern Siberia. Polar Sci. 21, 186–194. doi:10.1016/j.polar.2019.01.005
Morozumi, T., Shingubara, R., Suzuki, R., Kobayashi, H., Tei, S., Takano, S., et al. (2019b). Estimating Methane Emissions Using Vegetation Mapping in the Taiga-Tundra Boundary of a north-eastern Siberian lowland. Tellus B: Chem. Phys. Meteorol. 71. Article 1581004. doi:10.1080/16000889.2019.1581004
Morozumi, T., Sugimoto, A., Suzuki, R., Nagai, S., Kobayashi, H., Tei, S., et al. (2020). Photographic Records of Plant Phenology and spring River Flush Timing in a River lowland Ecosystem at the Taiga-Tundra Boundary, Northeastern Siberia. Ecol. Res. 35 (5), 717–723. doi:10.1111/1440-1703.12107
Murase, J., Sugimoto, A., Shingubara, R., Liang, M., Morozumi, T., Takano, S., et al. (2020). Methane Oxidation Potential of the Arctic Wetland Soils of a Taiga-Tundra Ecotone in Northeastern Siberia. Soil Sci. Plant Nutr. 66 (4), 645–652. doi:10.1080/00380768.2020.1786343
Neff, J. C., Finlay, J. C., Zimov, S. A., Davydov, S. P., Carrasco, J. J., Schuur, E. A. G., et al. (2006). Seasonal Changes in the Age and Structure of Dissolved Organic Carbon in Siberian Rivers and Streams. Geophys. Res. Lett. 33 (23). Article L23401. doi:10.1029/2006gl028222
Pokrovsky, O. S., Manasypov, R. M., Loiko, S., Shirokova, L. S., Krickov, I. A., Pokrovsky, B. G., et al. (2015). Permafrost Coverage, Watershed Area and Season Control of Dissolved Carbon and Major Elements in Western Siberian Rivers. Biogeosciences 12 (21), 6301–6320. doi:10.5194/bg-12-6301-2015
Prokushkin, A. S., Kawahigashi, M., and Tokareva, I. V. (2009). “Global Warming and Dissolved Organic Carbon Release from Permafrost Soils,” in Permafrost Soils. Series Soil Biology 16. Editor R. Margesin (Berlin, Germany: Springer-Verlag), 237–250.
Prokushkin, A. S., Pokrovsky, O. S., Shirokova, L. S., Korets, M. A., Viers, J., Prokushkin, S. G., et al. (2011). Sources and the Flux Pattern of Dissolved Carbon in Rivers of the Yenisey basin Draining the Central Siberian Plateau. Environ. Res. Lett. 6 (4), 045212. doi:10.1088/1748-9326/6/4/045212
Rachold, V., Eicken, H., Gordeev, V. V., Grigoriev, M. N., Hubberten, H.-W., Lisitzin, A. P., et al. (2004). “Modern Terrigenous Organic Carbon Input to the Arctic Ocean,” in The Organic Carbon Cycle in the Arctic Ocean. Editors R. S. Stein, and R. W. Macdonald (New York: Springer), 33–55. doi:10.1007/978-3-642-18912-8_2
Raymond, P. A., McClelland, J. W., Holmes, R. M., Zhulidov, A. V., Mull, K., Peterson, B. J., et al. (2007). Flux and Age of Dissolved Organic Carbon Exported to the Arctic Ocean: A Carbon Isotopic Study of the Five Largest Arctic Rivers. Glob. Biogeochem. Cycles 21 (4). Article Gb4011. doi:10.1029/2007gb002934
Shingubara, R., Sugimoto, A., Murase, J., Iwahana, G., Tei, S., Liang, M., et al. (2019). Multi-year Effect of Wetting on CH4 Flux at Taiga-Tundra Boundary in Northeastern Siberia Deduced from Stable Isotope Ratios of CH4. Biogeosciences 16 (3), 755–768. doi:10.5194/bg-16-755-2019
Stanley, E. H., Casson, N. J., Christel, S. T., Crawford, J. T., Loken, L. C., and Oliver, S. K. (2016). The Ecology of Methane in Streams and Rivers: Patterns, Controls, and Global Significance. Ecol. Monogr. 86 (2), 146–171. doi:10.1890/15-1027
Sugimoto, A., and Maximov, T. C. (2012). “Study on Hydrological Processes in Lena River Basin Using Stable Isotope Ratios of River Water (IAEA-TECDOC−1673),” in Monitoring Isotopes in Rivers: Creation of the Global Network of Isotopes in Rivers, Vienna (Vienna: IAEA), 41–49. Available at: https://www.osti.gov/etdeweb/biblio/21570990.
Sugimoto, A., Naito, D., Yanagisawa, N., Ichiyanagi, K., Kurita, N., Kubota, J., et al. (2003). Characteristics of Soil Moisture in Permafrost Observed in East Siberian Taiga with Stable Isotopes of Water. Hydrol. Process. 17 (6), 1073–1092. doi:10.1002/hyp.1180
Takano, S., Sugimoto, A., Tei, S., Liang, M., Shingubara, R., Morozumi, T., et al. (2019). Isotopic Compositions of Ground Ice in Near-Surface Permafrost in Relation to Vegetation and Microtopography at the Taiga-Tundra Boundary in the Indigirka River Lowlands, Northeastern Siberia. Plos One 14 (10). Article0223720. doi:10.1371/journal.pone.0223720
Tei, S., Morozumi, T., Nagai, S., Takano, S., Sugimoto, A., Shingubara, R., et al. (2020). An Extreme Flood Caused by a Heavy Snowfall over the Indigirka River basin in Northeastern Siberia. Hydrological Process. 34 (3), 522–537. doi:10.1002/hyp.13601
Turetsky, M. R., Abbott, B. W., Jones, M. C., Anthony, K. W., Olefeldt, D., Schuur, E. A. G., et al. (2020). Carbon Release through Abrupt Permafrost Thaw. Nat. Geosci. 13(2), 138, 143. doi:10.1038/s41561-019-0526-0
Ward, C. P., and Cory, R. M. (2016). Complete and Partial Photo-Oxidation of Dissolved Organic Matter Draining Permafrost Soils. Environ. Sci. Technol. 50 (7), 3545–3553. doi:10.1021/acs.est.5b05354
Ward, C. P., Nalven, S. G., Crump, B. C., Kling, G. W., and Cory, R. M. (2017). Photochemical Alteration of Organic Carbon Draining Permafrost Soils Shifts Microbial Metabolic Pathways and Stimulates Respiration. Nat. Commun. 8 (1), 1–7. doi:10.1038/s41467-017-00759-2
Welp, L. R., Randerson, J. T., Finlay, J. C., Davydov, S. P., Zimova, G. M., Davydova, A. I., et al. (2005). A High-Resolution Time Series of Oxygen Isotopes from the Kolyma River: Implications for the Seasonal Dynamics of Discharge and basin-scale Water Use. Geophys. Res. Lett. 32 (14). Article L14401. doi:10.1029/2005gl022857
Wiegner, T., and Seitzinger, S. (2001). Photochemical and Microbial Degradation of External Dissolved Organic Matter Inputs to Rivers. Aquat. Microb. Ecol. 24 (1), 27–40. doi:10.3354/ame024027
Yabuki, H., Park, H., Kawamoto, H., Suzuki, R., Razuvaev, V. N., Bulygina, O. N., et al. (2011). Baseline Meteorological Data in Siberia (BMDS) Version 5.0. RIGC, JAMSTEC, Yokosuka, Japan Distributed by CrDAP, Digital Media. Available at: https://ads.nipr.ac.jp/dataset/A20131107-002. (Accessed June 2020).
Yamashita, Y., Nosaka, Y., Suzuki, K., Ogawa, H., Takahashi, K., and Saito, H. (2013). Photobleaching as a Factor Controlling Spectral Characteristics of Chromophoric Dissolved Organic Matter in Open Ocean. Biogeosciences 10 (11), 7207–7217. doi:10.5194/bg-10-7207-2013
Yang, X., Yuan, J., Yue, F.-J., Li, S.-L., Wang, B., Mohinuzzaman, M., et al. (2021). New Insights into Mechanisms of Sunlight- and Dark-Mediated High-Temperature Accelerated Diurnal Production-Degradation of Fluorescent DOM in lake Waters. Sci. Total Environ. 760, 143377. doi:10.1016/j.scitotenv.2020.143377
Yi, Y., Gibson, J. J., Cooper, L. W., Hélie, J.-F., Birks, S. J., McClelland, J. W., et al. (2012). Isotopic Signals (18O,2H,3H) of Six Major Rivers Draining the Pan-Arctic Watershed. Glob. Biogeochem. Cycles 26. Article Gb1027. doi:10.1029/2011gb004159
Keywords: Arctic tundra wetland, riverine dissolved organic carbon, wetland hydrology, stable water isotopes, Northeastern siberia
Citation: Takano S, Yamashita Y, Tei S, Liang M, Shingubara R, Morozumi T, Maximov TC and Sugimoto A (2021) Stable Water Isotope Assessment of Tundra Wetland Hydrology as a Potential Source of Arctic Riverine Dissolved Organic Carbon in the Indigirka River Lowland, Northeastern Siberia. Front. Earth Sci. 9:699365. doi: 10.3389/feart.2021.699365
Received: 23 April 2021; Accepted: 21 June 2021;
Published: 06 July 2021.
Edited by:
Shafi Mohammad Tareq, Jahangirnagar University, BangladeshReviewed by:
Mohammad Mohinuzzaman, Noakhali Science and Technology University, BangladeshCopyright © 2021 Takano, Yamashita, Tei, Liang, Shingubara, Morozumi, Maximov and Sugimoto. This is an open-access article distributed under the terms of the Creative Commons Attribution License (CC BY). The use, distribution or reproduction in other forums is permitted, provided the original author(s) and the copyright owner(s) are credited and that the original publication in this journal is cited, in accordance with accepted academic practice. No use, distribution or reproduction is permitted which does not comply with these terms.
*Correspondence: Shinya Takano, aGF3ay1maWVsZEBlZXMuaG9rdWRhaS5hYy5qcA==
Disclaimer: All claims expressed in this article are solely those of the authors and do not necessarily represent those of their affiliated organizations, or those of the publisher, the editors and the reviewers. Any product that may be evaluated in this article or claim that may be made by its manufacturer is not guaranteed or endorsed by the publisher.
Research integrity at Frontiers
Learn more about the work of our research integrity team to safeguard the quality of each article we publish.