- 1College of Water Conservancy and Hydropower Engineering, Hohai University, Nanjing, China
- 2Key Laboratory of Comprehensive and Highly Efficient Utilization of Salt Lake Resources, Qinghai Provincial Key Laboratory of Geology and Environment of Salt Lakes, Qinghai Institute of Salt Lakes, Chinese Academy of Sciences, Xining, China
- 3State Key Laboratory of Paleobiology and Stratigraphy, Nanjing Institute of Geology and Paleontology, Chinese Academy of Sciences, Nanjing, China
- 4NAS Ukraine, Institute Geology & Geochemistry Combustible Minerals, Lvov, Ukraine
- 5University of Chinese Academy of Sciences, Beijing, China
Polyhalite occurrence in the Kunteyi Playa in the Qaidam Basin has been known for many years. However, the genetic mechanism of this deposit remains unclear. In this study, a typical section in the playa depocenter is selected to study the polyhalite mineralogy combined with the homogenization temperature and composition of halite fluid inclusions in shallow evaporitic strata. The results show that 1) the main evaporite minerals in the strata are halite and polyhalite; no common gypsum is found; 2) analyses of homogenization temperatures of halite fluid inclusions indicate that a higher temperature is needed for polyhalite generation compared with other saline minerals; and 3) the fluid inclusion chemical analysis shows that they are sulfate-type minerals with a shortage of Ca. Thus, it can be concluded that the formation of polyhalite is not related to gypsum replacement, and deep oilfield brines may provide a Ca source and a higher temperature for polyhalite formation, where the mixing and interaction occurred between K- and Mg-enriched sulfate brines and deep Ca-enriched brines under the control of climate and tectonics in the study area. While most polyhalite was generated natively, some formed during secondary generation, which was potentially related to replacement with carnallites or sylvites.
Introduction
Many complex chemical and physical processes occur during brine evolution in an actual salt lake system, and it remains difficult to explain certain specific mineral generation. These processes range from the dissolution to precipitation of various minerals, which depend on a variety of climate and other geological environmental conditions. Precipitation of polyhalite (Ca2MgK2(SO4)4·2H2O) is an important process that occurs during brine evaporation. Understanding polyhalite generation is critical for explaining the evolution of specific salt lakes in salt lake systems. As an important potassium-bearing mineral, polyhalite has been found in many evaporite basins worldwide (Warren, 2006). Most researchers believe that it is produced by the conversion of Ca-rich minerals that interact with a specific fluid at a later stage of brine evolution (Stewart, 1965; Pierre, 1985; Harville and Fritz, 1986; Li and Han, 1987; Wei, 1987; Liu et al., 2008; Liu et al., 2010; Ma et al., 2010; García-Veigas et al., 2013; Holt et al., 2014; Niu et al., 2015). Some have also considered that it can also be generated by multifluid interaction between Ca-rich brines and K–Mg-rich ones (Stewart, 1965; Perthuisot, 1971; Wang and Zheng, 2014). The transformation of gypsum to polyhalite requires brine with high K, Mg, and SO4, which has been used to decipher the origin of polyhalite in the Delaware Basin in the United States and the Zdrada Platform in Poland (Harville and Fritz, 1986; Peryt et al., 1998). In the Miocene marine Great kavir evaporite basin in central Iran, the inflow of CaCl2-rich brine accounts for the absence of primary polyhalite (Rahimpour-Bonab et al., 2007). Ultra-micro-analyses of halite fluid inclusions, developed by Petrichenko (1973), have been widely applied to study the evolution of ancient seawater and brine from halite fluid inclusions (Petrychenko et al., 2002; Kovalevych et al., 2006, 2009; Meng et al., 2014). The concentrations of K, Mg, Ca, and SO4 in a fluid inclusion (>20 μm) can be determined using this method (Kovalevuch et al., 2009). By analyzing the components of halite inclusions and saturation indices of the main evaporite minerals, García-Veigas et al. (2013) revealed that the Miocene polyhalite in the Granada Basin in Spain was mainly formed during the early stage of halogeneses. Polyhalite has also been extensively deposited in modern environments. In the Californian shore lagoon in the United States, after detailed investigation, the Holocene polyhalite is thought to have formed during the early stage of evaporite genesis, with CaSO4 being replaced (Hardie, 1968). Most polyhalite studies are based on marine environments, while polyhalite deposition in continental settings is rarely considered. The Kunteyi Playa, located in the northern Qaidam Basin in China, is known for its large-scale modern inland lacustrine polyhalite deposits. The geological reserves of polyhalite in the playa are approximately 200 million tons (Li et al., 1990). To the best of our knowledge, no work has systematically studied the formation mechanism of polyhalite deposits in this playa, except for some simple descriptions in earlier exploration works. Therefore, we selected a shallow section near the surface to conduct systematic studies to discuss the polyhalite genesis and better understand the evolution of this special playa. In this study, we demonstrate how the combined information from sedimentary characteristics, polyhalite mineralogy, and halite fluid inclusions support the evolution of brine and the polyhalite deposition processes in the Kunteyi Playa.
Geological Setting
The Qaidam Basin, located in the northern Qinghai–Tibet Plateau, has an area of 120,000 km2 and an average elevation of 3,000 m. It is confined to the tectonic belts of Qilian Mountain, Kunlun Mountain, and Altyn Mountain, which controlled the basin evolution. The Qaidam Basin was generated as a result of multistage strike-slip activities accompanied by strong uplift on the Altyn Fault belt since the Cenozoic (Yin et al., 2002; Li et al., 2006). There were at least three strong uplifts (∼30 Ma, ∼8 Ma, and ∼2.6 Ma) during the Cenozoic that affected and changed the tectonic development in the basin (Pan et al., 2015). Outcrops of Cenozoic strata occur along the northern and western basin edges. From bottom to top, these strata comprise seven formations: the Lulehe (E1-2), Lower Ganchaigou (E3), Upper Ganchaigou (N1), Lower Youshashan (N21), Upper Youshashan (N22), Shizigou (N23), and Qigequan formations (Q1-2) (Shen et al., 1993; Wang et al., 1997).
The Kunteyi Playa covers an area of 1,680 km2 in the northern Qaidam Basin (Figure 1). The playa is characterized by an extremely dry climate with an average precipitation of only 15.7 mm per year, while the average evaporation rate is as high as 3,095.9 mm per year (Zhang and Xuan, 1996). Although one seasonal river, the Qingshui River, recharges the border of this playa, there is no other surface water recharging it, which makes it a closed basin surrounded by the tectonic belts of the Eboliang to the west, Hulushan to the south, and Lenghu to the east (Wang et al., 1997). Zhang. (1987) concluded that the playa was separated from an ancient pan-lake in the Qaidam Basin during tectonic movement in the Late Pliocene and then formed as an isolated subbasin. During the Early and Middle Pleistocene, the basin sedimentary environment primarily alternated between a salt lake and saline–semisaline water. The salt lake environment was characterized by halite deposition, while gypsum deposition was a feature of the saline–semisaline water environment. It was affected by neotectonic movement during the Late Pleistocene when the western part of the Qaidam Basin continued to uplift, and the Kunteyi Playa was already isolated. At this time, the depth of Kunteyi Lake was shallower than during the Early and Middle Pleistocene and the sedimentary environment changed from a saline–semisaline water environment to a salt lake environment. Since the Late Pliocene and Quaternary, the uplift of the Kunlun Mountains and the Altyn Tagh Mountains caused the development of several secondary or subsidiary basins, and these basins ultimately evolved into playas with climatic desiccation (Chen and Bowler, 1986). The evaporite sediments occurred mainly from 0.97 Ma to 0.03 Ma, with halite and gypsum as the major evaporites, with some mirabilite and polyhalite minerals (Han et al., 1995; Wang et al., 1997; Huang and Han, 2007).
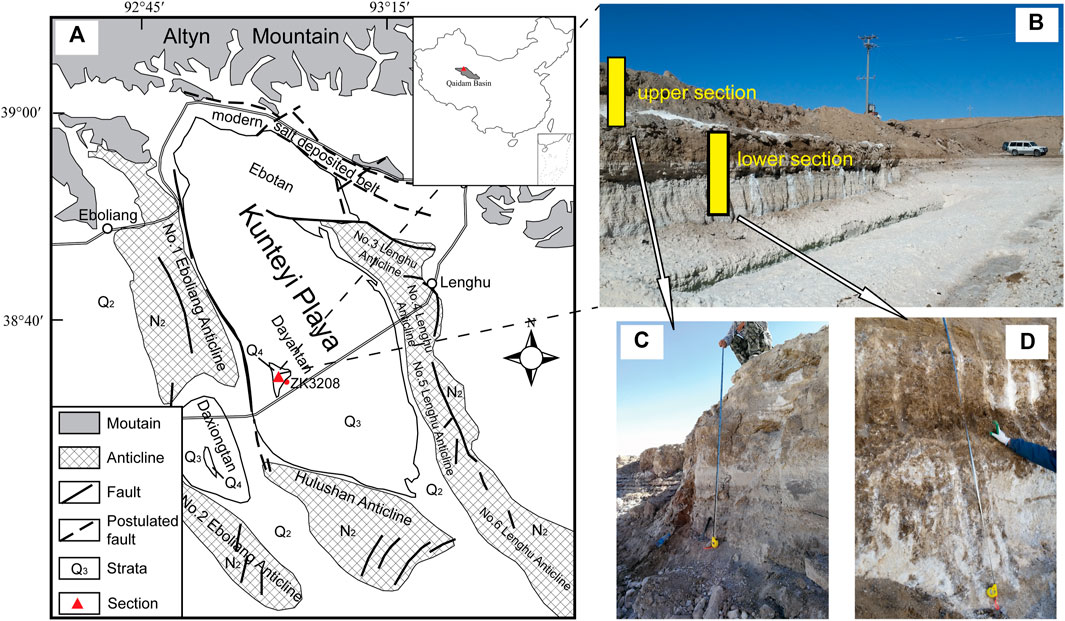
FIGURE 1. Sketch of the Kunteyi Basin and the section with polyhalite deposits we selected in this study. (A) the Kunteyi Basin and its surrounding geomorphology ((Altyn Mountain to the north, uplifted anticlines, mainly the Lenghu structure No. 3, No.4, No. 5, and No. 6) to the east and south, Hulushan anticline (No. 1 and No. 2), and Eboliang anticlines, respectively); (B) the selected geological section comprised of upper (C) and lower (D) section.
Polyhalite deposits are commonly found in brine aquifers and silt-rich aquitards in shallow strata, which are the most important solid K-containing minerals. However, polyhalite in the basin cannot be exploited directly because of its low dissolution rate and low permeability (Yuan et al., 2018). This type of mineral is mainly distributed in the Pleistocene stratum on the west side of the basin, and its deposition probably occurred during the middle to late stage of the Middle Pleistocene (Wei et al., 1993). The buried depth of polyhalite is about 6–65 m, characterized as lenses. The maximum thickness of a single layer of the ore bed is up to 12 m, and the minimum is 0.3 m. The maximum thickness of the bed is up to 18.1 m; results from chemical analysis suggested that the average content of K2SO4 is 3.95% (Liu et al., 2010).
Materials and Methods
An approximately 6-m thick section with polyhalite deposits near borehole ZK3208 in the Kunteyi Playa was investigated in this study (Figure 1). Seventeen samples (BD-01–17) were collected based on the lithologic characteristics of the sections. The sample BD-01 being collected in the depth of 0.20 m is dark brown silty clay with halite and trace gypsum. BD-02 (0.50 m in depth) is white fine-grained halite crust and brown silt. BD-03∼BD-05 (0.70 m, 1.20 m, 1.70 m) are brown clayey silt with fine-grained and semieuhedral halite. BD-06 and BD-07 (2.20 m, 2.70 m) are brown clayey silt with halite and fine sand. BD-08∼BD-10 (2.90 m, 3.50 m, 3.70 m) are pale yellow, loose fine sand with scattered halite and minor clay. BD-11 and BD-12 (3.90 m, 4.10 m) are grey medium-coarse grain halite with minor mud. Samples BD-13 and BD-14 (4.30 m, 4.50 m) are brown polyhalite with minor scattered halite and mud. BD-15∼BD-17 (4.80 m, 5.20 m, 5.70 m) are characterized as grey medium-coarse grain halite with minor polyhalite and mud (Figures 2, 3). To ensure sample representativeness, the exposed loose sediments on the surface of the section were removed before sampling.
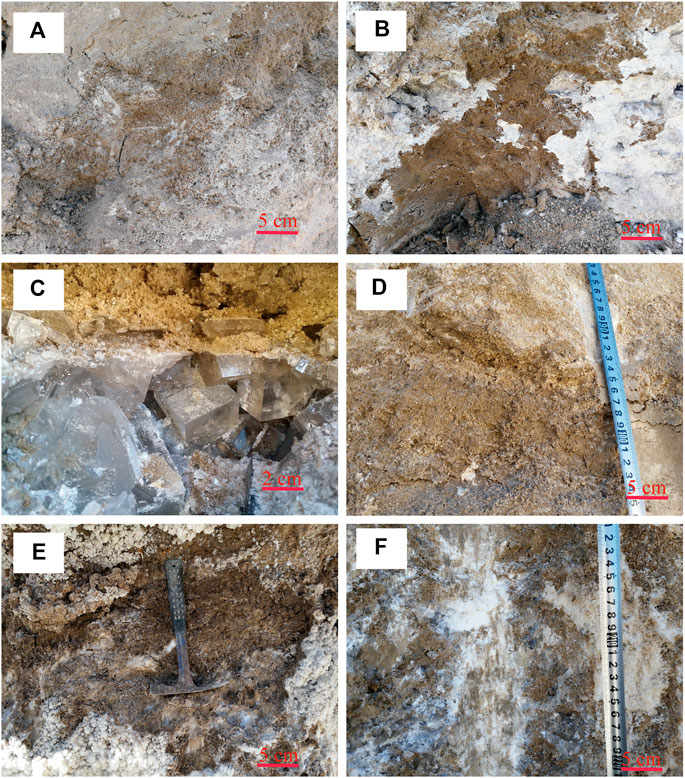
FIGURE 3. Lithological characteristics of the section in study area. The upper section ((A,B), 2.70 m in depth) is composed with brown to dark brown fine sand and halite, with a thinly mirabilite deposited on the section surface. The lower section (from 5.70 to 2.70 m upwards) is characterized as (C) a grey clay-bearing halite layer with large halite crystals (∼0.3–1.5 cm diameter); (D) a white to grey salt-containing sand layer, local white polyhalite lenses existed; (E,F) a horizontal polyhalite-enriched sublayer was the main polyhalite formation layer.
The mineralogical investigations consisted of three main parts. First, every 100 g representative sample was crushed to 60 mesh, and 3–5 g was weighed and ground continually to 200 mesh. The samples were then scanned by X-ray diffraction (XRD) (X’Pert Pro) continually with a working condition of 30 mA and 40 kV. Three samples (BD-10, BD-13, and BD-14) with a high proportion of polyhalite were selected to analyze the micromorphology and chemical components using scanning electron microscope—energy dispersive spectrometer (SEM-EDS) (JSM-5610LV/INCA series, Max Resolution HV3.5 nm, Min Resolution LV5.0 nm, Magnification: 35–30 million times). All experiments were performed at the Salt Lake Analysis Center at the Qinghai Institute of Salt Lakes, Chinese Academy of Sciences.
The cooling nucleation approach was used to measure the homogenization temperature of halite fluid inclusions (Lowenstein et al., 1998; Benison and Goldstein, 1999; Meng et al., 2011; Meng et al., 2013; Zhang et al., 2016). First, a 0.5 mm thin slice was removed from the selected halite crystal using a razor blade along a cleavage plane. Then, detailed petrographic observations on every segment were performed, and the pure liquid fluid inclusions with primary chevron shape characteristics were marked and documented clearly under the microscope. All the samples were rapidly cooled to 18°C and held. When nucleation vapor bubbles emerged, the homogenization temperature was measured on a cooling–heating stage (LINKAM THMSG600, measurement range: 196–600°C, error: ±1°C) coupled with a microscope (Axioskop 40 Pol, eyepiece lens ×10, objective lens ×20, 50×). After the set-up, the heating stage was warmed at a rate of 0.5°C/min up to 15°C. Finally, the temperature was lowered at a rate of 0.1°C/min until all artificially nucleated vapor bubbles disappeared (homogenized).
Ultra-micro-analysis of halite fluid inclusions was performed in this study as follows.
1) Fluid inclusion extraction. A series of well-preserved salt crystals was selected and cleaned before drying in an oven at warm temperatures. Then, like the criterion of homogenization temperature analysis, the characteristics of geometry and distribution of a series of 0.3–0.5 mm thin slices were removed from the selected halite crystals using a razor blade along a cleavage plane and were observed to determine the fluid inclusion origin. Furthermore, under the microscope, fluid from the inclusions was extracted using a conical microdrill with a capillary glass tube.
2) Measurement of major elements. Special reagents were added to interact with the extracted fluid from the inclusion, depending on the specific ions to be measured. (NH4)2C2O4 (5%) was added to determine the Ca, BaCl2 (30%) to determine SO4, Na3Co(NO2)·0.5H2O (3%) to determine K, C6H12N4 (30%) and K4[Fe(CN)6]·3H2O (15%) to determine lower concentrations of Mg (<6 g/L), and C6H12N4 (30%) and (NH4)2C2O4 (15%) to determine higher Mg concentrations (>6 g/L). Reaction stoichiometries are presented below.
The depleted reagent added depends on the reactions studied, which are visually controlled under a microscope. This always exceeds the amount of element to be precipitated. The capillary glass tube was sealed and centrifuged until the ultimate precipitation reactions were completed. The volumes of the precipitate and solution formed during this process were measured separately and then compared to those formed from a standard solution (Petrichenko, 1973). To minimize the measurement error, we obtained a series of analysis data for each component in the inclusions; the error rate decreased to 16–17% by two to three parallel analyses (Petrichenko, 1973). The minimum quantity of the studied ions needed for such an error rate is (in g/L) 0.8 for K, 1.0 for Mg, 0.9 for Ca, and 0.5 for SO4, and the smaller values are not very precise. These experimental analyses were performed at the State Key Laboratory of Paleobiology and Stratigraphy, Nanjing Institute of Geology and Paleontology, Chinese Academy of Sciences.
Results
Petrography
The whole section for the study included the upper and lower sections (Figure 1B). The sediments in the upper section (from 2.70 m to the surface) were mainly composed of brown to dark brown silt or fine sand with halite (Figures 3A,B). In this section, it was difficult to identify polyhalite minerals in the field. The lower section from the bottom (5.70 m) to approximately 2.80 m upwards was divided into four parts (Figure 2). The first part was a grey clay-bearing halite layer with large halite crystals characterized by well-developed intercrystallite voids (∼0.3–1.5 cm diameter) (Figure 3C). The second part was a white to grey salt-containing sand layer (Figure 3D), in which the detrital materials were more abundant than in the other layers. Local white polyhalite-containing stripes could also be found. The third part was brown and grey-white salt and polyhalite, 50 cm thick, with a horizontal polyhalite-enriched sublayer that could be easily identified and was the main polyhalite formation layer (Figure 3E). The polyhalite distributions were much more heterogeneous, as they contained a higher level in white matrix than in grey-yellow ones. The fourth part was a grey or grey-white, middle to coarse granular halite (Figure 3F), where recrystallization phenomena were significant. Polyhalite was mixed within the grey silt in this layer. According to the description above, compared to salt layers, polyhalite minerals were mainly deposited in the silt/clay between two significant salt layers.
Mineralogy
Observation under a microscope and XRD results showed that the saline minerals mainly consisted of fine- to coarse-grained halite, grey massive polyhalite, and gypsum. Moreover, a few sylvites and syngenites were found locally in the upper part (Table 1). Clastic sediments coexisted with saline minerals. Halite was the most common saline mineral and was present in each layer. In some layers, the halite was nearly pure, which is consistent with our field observations. Polyhalite was another main saline mineral found in most layers, characterized as fine-grained structure, 0.01 to 0.05 mm in length; the types of single crystal are leaf-like, needle-like, and fibrous (Figure 4). The percentage of polyhalite in most layers was no more than 20%, and only a few samples contained more than 40%. It is interesting that gypsum minerals were only found in the upper layer (from 2.7 m to the top) and were not detected in the lower part (from 5.7 to 2.7 m). In fact, we repeatedly tested all samples to avoid measurement errors. However, the second result was almost the same as that of the first. Therefore, the lower part did not contain gypsum. In addition, quartz was the main mineral in clastic sediments, and other clastic minerals detected by XRD included albite, chamosite, and muscovite.
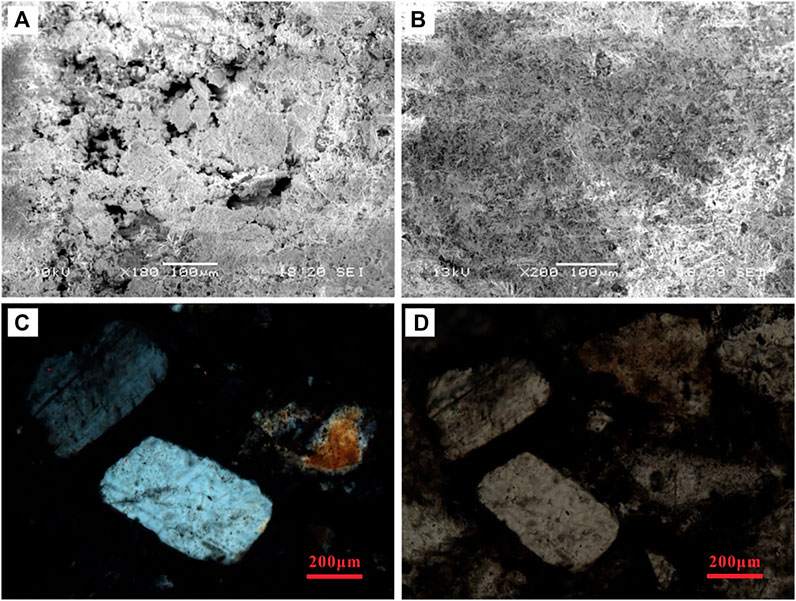
FIGURE 4. Photos of microscope of morphology of polyhalite. (A) and (B) indicate the polyhalite in transition layer between layers of halite and clastic; (C) and (D) refer to the morphology of polyhalite in halite layer.
Cauliflower-like polyhalite surrounded by medium-coarse-grained halite crystals and clay mineral particles was observed under SEM (Figure 5A). The EDS results showed that the polyhalite aggregates were mainly composed of polyhalite with siliceous clasts and halite facies. The EDS results suggest that the aggregation or mixture is mainly composed of polyhalite, but some siliceous clastic material and halite coexist with it. In Figure 5B, the contents of K and Mg were obviously higher than those of S(SO4) and Ca; however, the Cl content was also very high. This implies that, except for polyhalite, some potash minerals, such as sylvite (KCl) and carnallite (KMgCl3·H2O), probably were present in the sample. The evidence indicates that when polyhalite was deposited, the brine evolved into a K- and Mg-enriched stage. In fact, the coexistence of polyhalite with K-bearing chlorides was also found in the Qarhan salt lake (Yang et al., 1993; Yuan et al., 1995). In Figure 5C, the chemical component of the sample is more complicated than that of the former sample, which suggests a complex of minerals. Therefore, although some chloride minerals were not detected by XRD, they probably existed, indicating that the brine was highly concentrated and enriched in K and Mg.
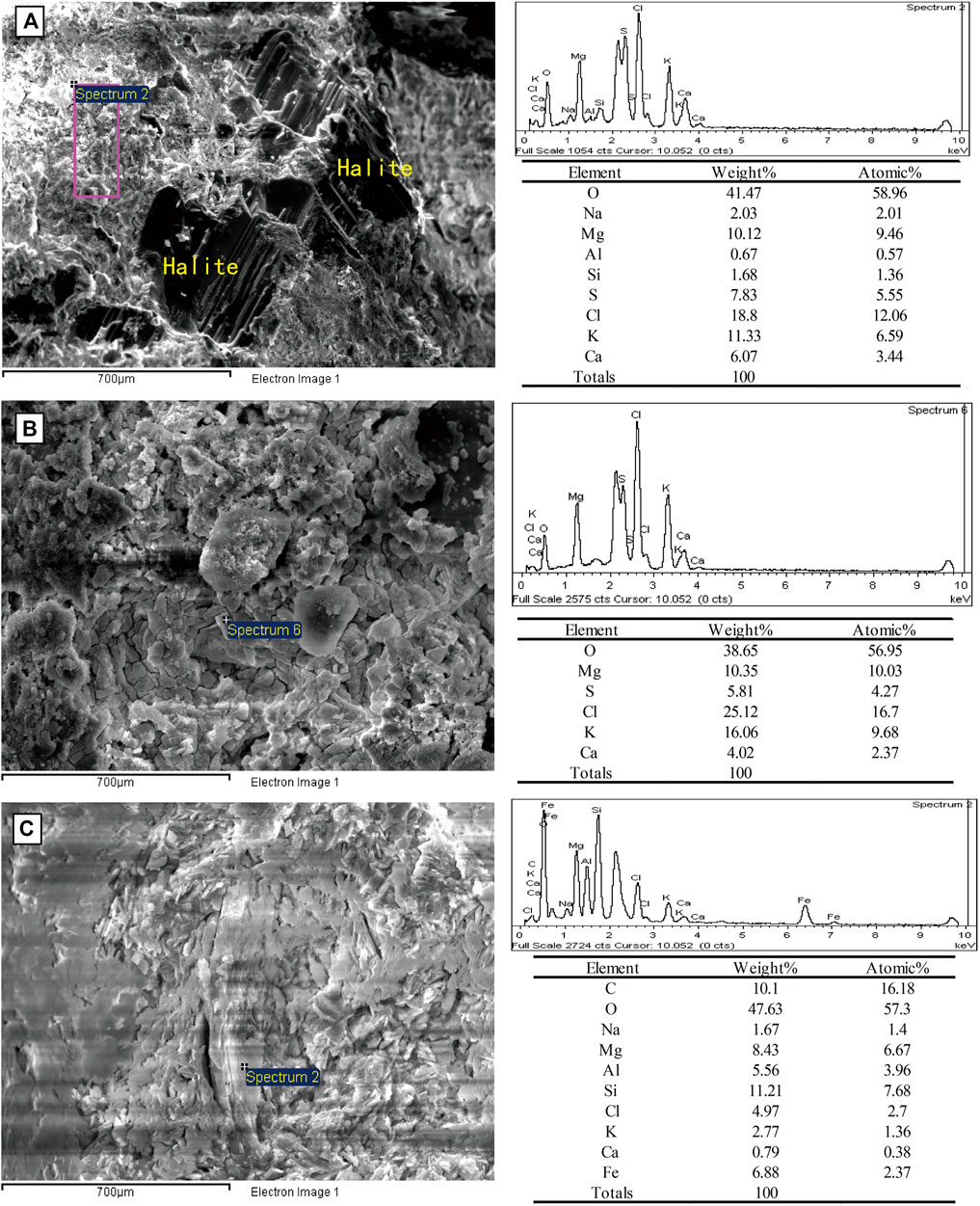
FIGURE 5. Images of minerals from SEM (left) and relatively the results of mineral spectrums from EDS (right). In image (A) (from sample BD-10), the scanning area purple rectangle is an aggregation of some minerals. In image (B) (from sample BD-13), the scanning point is a polyhalite piece. In image (C) (from sample BD-14), on a larger scale (10 μm), it shows a more complicated component.
Homogenization Temperatures of Halite Fluid Inclusions
The cooling nucleation approach was used to measure the homogenization temperature of halite fluid inclusions. The results show that the fluid inclusion temperature gradually decreased from the bottom to the top of the section (Table 2). A possible explanation might be related to the effect of the solar pool event due to brine delamination or extreme surface heat from the inland desert (Zambito and Benison, 2013; Wang and Lowenstein, 2017).
Many fluid inclusions were found in chevron-type halite crystals (Figure 6), which can form at the bottom of salt lakes that are less than 60 cm in depth as a result of competitive growth (Handford, 1990; Meng et al., 2013). The maximum homogenization temperature can reach 80.2°C, which records the bottom water temperatures of that time.
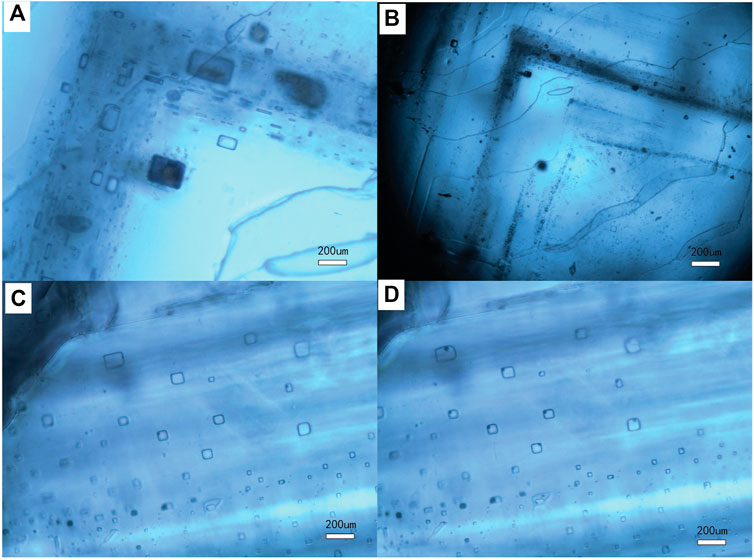
FIGURE 6. Photos of halite fluid inclusions. (A,B) indicate the primary chevron halite, sampled from BD-12. (C) is the photo of inclusion before freezing. (D) shows that vapor bubbles emerged after freezing, sampled from BD-17.
Chemical Components of Halite Fluid Inclusions
The results for the chemical components and types of halite fluid inclusions are shown in Table 3. The concentrations of K, Mg, and SO4 were much higher than those of modern brines in this area, and Ca was not observed in the fluid inclusions. Compared with K in secondary fluid inclusions (2 g/L) in the bottom salt layers (BD-16, BD-17), the value in primary fluid inclusions was up to 30 g/L. In the upper salt layers, the concentration values of K in both the primary and secondary fluid inclusions were similar (∼30 g/L). However, the values of Mg and SO4 changed significantly and were disorderly. We can conclude that there might be a syngenetic or parasyngenetic origin of these fluid
Discussion
Mineral Assemblages and Their Sedimentological Significance
Traditionally, polyhalite is a type of secondary mineral, as it is considered to be a substitute for gypsum and usually coexists with Ca-SO4 minerals (Hardie, 1968; García-Veigas et al., 2013; Holt et al., 2014). It is important to identify the types of sedimentological environment and genesis of polyhalite minerals. However, in this study, gypsum was not detected in the lower part of the clay sediments. The replacement of gypsum by polyhalite does not occur in these clastic sediments, and these polyhalites are probably primary minerals, similar to the coexistence of these minerals with salts. Many polyhalite crystals coexist with clastic sediments, which can even be over 50%. The simulation results using the EVP/EQL program show that when concentrated K- and Mg-enriched brines mix with Ca-enriched deep brine (also called oilfield brine), a nearly pure polyhalite was formed (Ai et al., 2018). In fact, some ions are more enriched in fine-grained clastic sediments (e.g., peat, clay, etc.) than in salt lake brines. A comparison of lake brine and mud brine from Xiao Chaidan Lake in the Qaidam Basin shows that the main chemical components are obviously more enriched in mud brine than those in lake brine (Du et al., 2016). Therefore, it is postulated that the polyhalite in clastic sediments is precipitated after highly concentrated underground mud brines enriched with K and Mg mixed with the later intruded Ca-enriched deep brine.
The section is divided into two parts from bottom to top: the lower part (polyhalite in salts without gypsum) and the upper part (polyhalite in clastics with gypsum) (Figure 7). In the lower part (II), there were more salts and less clastic sediments. The obvious characteristic is the absence of gypsum, whether in salt or clastic sediments. For polyhalite in halite and without gypsum, these minerals are usually considered to be primary (Wei et al., 1993). Recently, studies on Triassic polyhalite deposits in the Sichuan Basin have shown that polyhalite coexisting with halite is considered to be primary and precipitated after seawater enriched in Ca mixed with seawater enriched in K and Mg (Zhong et al., 2020). Ca ions were not detected in the primary or secondary fluid inclusions in halite (Table 3), which indicates that these polyhalites precipitated after halite formation. Considering that the brine evolved into the stage of potash deposition (Figure 8), the brine should be highly enriched in K and Mg. It is quite possible that these polyhalites formed after the mixing of K- and Mg-enriched brines with deep Ca-enriched fluids (Han et al., 1982).
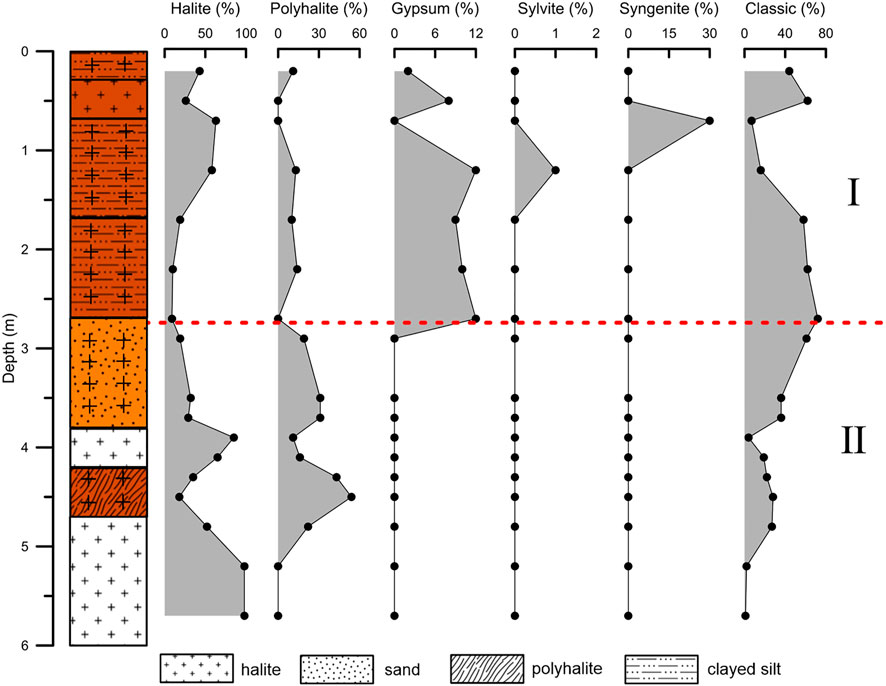
FIGURE 7. Changes in main minerals with depth in section. The upper part (Ⅰ) is characterized by polyhalite coexisting with gypsum, and the lower part (II) is characterized by polyhalite coexisting without gypsum.
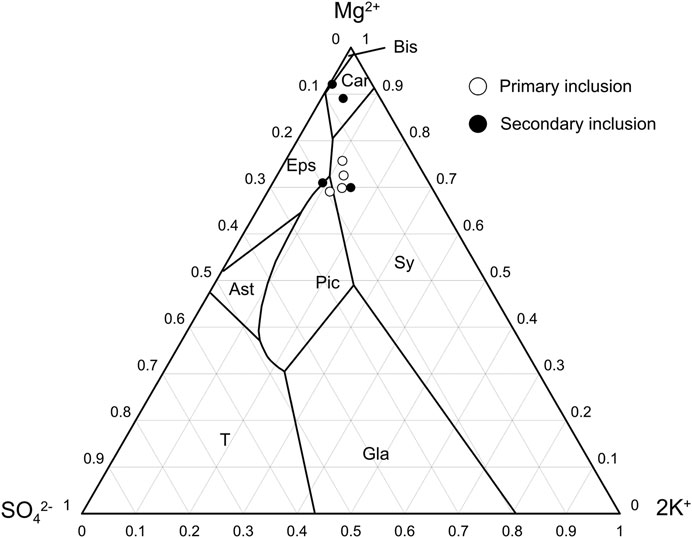
FIGURE 8. Projection points of fluid composition of halite inclusions in metastable phase diagram of K–Na–Mg–SO4–Cl system (after Meng et al., 2013). Car—KCl·MgCl2·6H2O, Eps—MgSO4·7H2O, Sy—KCl, Pic—K2SO4·MgSO4·6H2O, Ast—Na2SO4·MgSO4·4H2O, T—Na2SO4, Gla—Na2SO4·3K2SO4.
The precipitation in the upper part (Ⅰ) of the sediments may have experienced a drier environment and the concentration of brine was higher because of the occurrence of some potassium-bearing salt minerals. The type of polyhalite coexisting with gypsum or other CaSO4 minerals (syngenite) in this part is less than 15%, which indicates that the polyhalite in the upper part was derived from gypsum replacement. The same conclusion was drawn by Wei et al. (1993).
Furthermore, a five-member system mesostable phase diagram of the ore-forming fluid (K–Na–Mg–SO4–Cl) is shown in Figure 8. All observed fluids reached the point of K–Mg-containing mineral precipitation. Primary fluid inclusions in the bottom salt layers (BD-16, BD-17) were spotted in the sylvite phase zone, which suggested that the sylvite precipitation stage occurred when salt began to precipitate. However, the projection points of secondary inclusions from the same samples were found in the carnallite phase zone. This can be explained by the formation of secondary halite after the brine evolved to sylvite precipitation coupled with further evaporation processes. This also suggests that the brine entered the stage of sylvite precipitation when halite formed, and the concentration of this brine increased during the later halite formation. The projection points of both primary and secondary inclusions in sample BD-11 from the upper layer were located approximately in the phase zone of sylvite or picromerite, which also suggested that the halite and sylvite precipitation stages were coincident. During the late period of enhancement of the secondary mineralization and recrystallization process for halite, these inclusion features changed a little in the closed environment.
Formation Condition and Ore-Formed Fluid Evolution of Polyhalite Deposits
The lithology of the observed strata of the Kunteyi Playa shows alternating precipitation of evaporites and clastic sediments. Evaporites started to precipitate 730 ka, and the salt lake encountered eight significant fluctuation exchanges between dry hot and wet cold climate conditions (Han et al., 1995; Liu et al., 1998). After 240 ka, the paleoclimate tended to be drier, which intensified the role of evaporation in the area. Furthermore, water recharge was also reduced. Therefore, it was characterized as a gradually drier condition for the deposition of environmental sediments (Liu et al., 1998). The brine was concentrated via evaporation gradually, with enrichment in K and Mg; thus, it provided the necessary materials for polyhalite formation.
Apparently, the fluid inclusions in these salt crystals are characterized by different high temperatures. The majority of nonferrous metals derive ultimately from igneous processes associated with magmatism (Blundy et al., 2021). This conclusion suggested that geothermal fluids provided both a circumstance of high temperature and Ca-rich brine for polyhalite formation. Zhao et al. (2010) reported that halite fluid inclusions from Qarhan salt lakes have a homogeneous temperature as high as 200°C, which is thought to be related to the effect of solar pool events. Regardless of the reason for the former scenario, the homogenization temperature data from fluid inclusions suggests that there was a high temperature environment for the formation of evaporites such as halite and polyhalite. In fact, in geological history, a high temperature (about 73°C) recorded in the Permian Nippewalla Group (North America) ephemeral lake halite showed that an extremely high temperature event could occur in salt lakes (Zambito and Benison, 2013).
During the evolution of polyhalite precipitation from brine, the Ca ion was always low in the brine, which could be impossible for the precipitation of large amounts of polyhalite only with this type of brine itself. Near the Kunteyi Playa, it has been reported that deep oilfield water is rich in Ca (Li et al., 2016; Shi et al., 2016). Combined with the homogenization temperature results shown in Homogenization Temperatures of Halite Fluid Inclusions, we suggest that the deep oilfield Ca-rich water is the major source of Ca for polyhalite formation. Homogenization temperatures results showed that the minimum of the brine temperature was 22.9°C, and the range of phase zone for polyhalite precipitation increased as the temperature increased. This condition was favorable for polyhalite formation, even on a large scale. According to the inclusion temperature results, the halite formation temperature is more than 20°C, and it increases up to 40–60°C, where polyhalite is enriched (Table 2). On a large scale, we conclude that brine with a higher temperature is an important factor for polyhalite formation. Tectonic conditions, as another important factor, provided a necessary conduit for deep Ca-rich water to the playa for polyhalite formation. To summarize, the complex conditions for polyhalite formation are the result of multi-impacts of rigorous climate, tectonic movements, and original materials.
The results of this study showed that brine evaporation evolution and polyhalite formation underwent complex physical and chemical processes, three important stages of which were included. Initially, the sulfate type of brine evolved to the step of K-containing mineral precipitation (such as sylvite and picromerite), which is a significant characteristic of K- and Mg-rich brine. Subsequently, polyhalite was formed as a result of high temperature deep Ca-rich brine injected into the evaporative basin. Because the polyhalite-rich formation occurred at the sylvite or carnallite precipitation stage, parts of these polyhalites may have formed as a result of metasomatism of these earlier formed K-bearing chlorides. Finally, an extremely dry climate and very limited supply of surface water caused restricted sedimentation in the basin; thus, the polyhalite was preserved. To date, no further corresponding petrographic evidence has been found to prove whether the interaction of these two types of brines underwent a process that produced a Ca-SO4 mineral, which was then quickly metasomatized by polyhalite.
Conclusion
In this study, in order to obtain the evidence of the formation of polyhalite in Kunteyi Playa, a multiapproach of sedimentology, mineralogy, and halite fluid inclusion analyses is utilized. The following conclusions were drawn from the analysis of the results.
1) Halite, gypsum, and polyhalite are commonly the major saline minerals but gypsum is not found in the lower part of the profile, which suggests that polyhalite formation in the lower part is not necessarily connected with the replacement by CaSO4-containing minerals. The polyhalite coexisting with CaSO4-containing minerals (gypsum or syngenite) in upper part of the section should be a secondary mineral occurrence. The polyhalite coexisting without CaSO4-containing minerals, whether in salts or in clastic, is probably primary.
2) During salination, the temperature of original brine had exceeded 20°C or even much higher, and the formation of polyhalite ranged from 40°C to 60°C; it provided a vital condition for large-scale polyhalite formation. Because polyhalite is a kind of Ca–Mg–K-rich mineral, the primary brines for its formation should contain K–Mg-rich mother brine and Ca-rich water. From this analysis, it is reasonable to infer that the formation of polyhalite occurred in the late stage of salt lake evolution.
3) During the mixing action between K–Mg-rich sulfate brine and deep Ca-rich oilfield brine, it underwent multicoupled physical and geochemical processes to form polyhalite, most of which was deposited as primary mineralization.
Data Availability Statement
The raw data supporting the conclusions of this article will be made available by the authors, without undue reservation.
Author Contributions
XY contributed to conception and design of the study. XZ, FM, JS, and AG organized the database. HC, YL, WM, WL, QT, JL, and YD contributed to the collection of samples and performed the statistical analysis. All authors contributed to manuscript revision and read and approved the submitted version.
Funding
The author is grateful to the National Natural Science Foundation of China (Grant nos. 41807216, 41672087, and 41402082), to the National Key Research & Development Program (Grant no. 2018YFC0406605), and to the Light of West Talent Program of Chinese Academy of Sciences (grant to YD) for support of this research.
Conflict of Interest
The authors declare that the research was conducted in the absence of any commercial or financial relationships that could be construed as a potential conflict of interest.
Publisher’s Note
All claims expressed in this article are solely those of the authors and do not necessarily represent those of their affiliated organizations, or those of the publisher, the editors and the reviewers. Any product that may be evaluated in this article, or claim that may be made by its manufacturer, is not guaranteed or endorsed by the publisher.
Acknowledgments
The authors are grateful for the anonymous reviewers for their useful comments to improve the content. Help from Wan Weihan and Huang Linwei of Lenghu Bindi Potash Company is greatly appreciated.
References
Ai, Z., Li, Y., Tang, Q., Cheng, H., Li, J., Zhou, T., et al. (2018). Preliminary Study on the Source of Formation-Ore Fluids of Polyhalite in Kunteyi Playa Based on Hydrogeochemical Simulation. J. Salt Lake Res. 26 (4), 44–50. in Chinese with English abstract. doi:10.12119/j.yhyj.201804006
Benison, K. C., and Goldstein, R. H. (1999). Permian Paleoclimate Data From Fluid Inclusions in Halite. Chem. Geol. 154, 113–132. doi:10.1016/S0009-2541(98)00127-2
Blundy, J., Afanasyev, A., Tattitch, B., Sparks, S., Melnik, O., Utkin, I., et al. (2021). The Economic Potential of Metalliferous Sub-volcanic Brines. R. Soc. Open Sci. 8, 202192. doi:10.1098/rsos.202192
Chen, K., and Bowler, J. M. (1986). Late Pleistocene Evolution of Salt Lakes in the Qaidam Basin, Qinghai Province, China. Palaeogeogr. Palaeoclimatol. Palaeoecol. 54, 87–104.
Du, Y., Ma, T., Xiao, C., and Chen, L. (2016). Analysis on Hydrochemistry Characteristic of Mud Water in Xiao Qaidam Lake. Arid Zone Res. 33 (1), 94–100. in Chinese with English abstract. doi:10.13866/j.azr.2016.01.11
García-Veigas, J., Cendón, D. I., Rosell, L., Ortí, F., Torres Ruiz, J., Martín, J. M., et al. (2013). Salt Deposition and Brine Evolution in the Granada Basin (Late Tortonian, SE Spain). Palaeogeogr. Palaeoclimatol. Palaeoecol. 369, 452–465. doi:10.1016/j.palaeo.2012.11.010
Han, W., Gu, S., and Cai, K. (1982). On the Formative Conditions of Polyhalite in the Six-Component System K, Na, Mg, Ca/Cl, SO4-H2o. Chin. Sci. Bull. 27 (12), 1319–1324.
Han, F., Huang, Q., Wang, K., Wang, H., and Yuan, L. (1995). Study of Geochemical Evolution and Palaeoclimatic Fluctuation of Kunteyi Salt lake in the Qaidam Basin, Qinghai. Oceanologia et Limnol. Sin. 26 (5), 502–508. in Chinese with English abstract.
Handford, C. R. (1990). Halite Depositional Facies in a Solar Salt Pond: a Key to Interpreting Physical Energy and Water Depth in Ancient Deposits? Geol 18, 691–694. doi:10.1130/0091-7613(1990)018<0691:hdfias>2.3.co;2
Hardie, L. A. (1968). The Origin of the Recent Non-Marine Evaporite Deposit of Saline Valley, Inyo County, California Geochim. Cosmochim. Acta 32, 1279–1301. doi:10.1016/0016-7037(68)90029-X
Harville, D. G., and Fritz, S. J. (1986). Modes of Diagenesis Responsible for Observed Succession of Potash Evaporites in the Salado Formation, Delaware Basin, New Mexico. J. Sediment. Petrol. 56 (5), 648–656. doi:10.1306/212f89fe-2b24-11d7-8648000102c1865d
Holt, N. M., García-Veigas, J., Lowenstein, T. K., Giles, P. S., and Williams-Stroud, S. (2014). The Major-Ion Composition of Carboniferous Seawater. Geochim. Cosmochim. Acta 134, 317–334. doi:10.1016/j.gca.2014.03.009
Huang, Q., and Han, F. (2007). Evolution of Salt Lakes and Palaeoclimate Fluctuation in Qaidam Basin. Beijing: Science Press. in Chinese with English abstract.
Kovalevych, V. M., Marshall, T., Peryt, T. M., Petrychenko, O. Y., and Zhukova, S. A. (2006). Chemical Composition of Seawater in Neoproterozoic: Results of Fluid Inclusion Study of Halite from Salt Range (Pakistan) and Amadeus Basin (Australia). Precambrian Res. 144 (1-2), 39–51. doi:10.1016/j.precamres.2005.10.004
Kovalevych, V., Paul, J., and Peryt, T. M. (2009). Fluid Inclusions in Halite from the Röt (Lower Triassic) Salt deposit in central Germany: Evidence for Seawater Chemistry and Conditions of Salt Deposition and Recrystallization. Carbonates Evaporites 24 (1), 45–57. doi:10.1007/bf03228056
Li, Y., and Han, W. (1987). An Experimental Study on the Formation Conditions of Polyhalite in Triassic System in Sichuan Basin. Geoscience 1 (3-4), 400–411. in Chinese with English abstract.
Li, C., Li, B., and Li, Z. (1990). Census Report of the Potash deposit in Kunteyi, Lenghu Town, Qinghai Province. Delingha: The Qinghai Qiandam comprehensive geological survey unit. in Chinese.
Li, H., Yang, J., Xu, Z., Sun, Z., Tapponnier, P., Van Der Woerd, J., et al. (2006). The Constraint of the Altyn Tagh Fault System to the Growth and Rise of the Northern Tibetan Plateau. Earth Sci. Front. 13 (4), 59–79. in Chinese with English abstract. doi:10.3321/j.issn:1005-2321.2006.04.006
Li, W., Zhang, X., Miao, W., Li, Y., Tang, Q., Wang, B., et al. (2016). Hydrochemical Characteristics of Oilfield Waters in Lenghu No.3 Structure Area of north Edge of Qaidam Basin. J. salt lake Res. 24 (2), 13–18. in Chinese with English abstract.
Liu, W., Xiao, Y., Han, F., and Peng, Z. (1998). Characteristics of Chlorine Isotopes in Salt Lakes of Kunteyi and Their Significance of Paleoclimate. Oceanologia et Limnol. Sin. 29 (4), 431–435. in Chinese with English abstract.
Liu, C., Wang, M., Jiao, P., Fan, W., Chen, Y., Yang, Z., et al. (2008). Sedimentary Characteristics and Origin of Polyhalite in Lop Nur Salt lake, Xinjiang. Mineral. Deposits 27 (6), 705–713. in Chinese with English abstract. doi:10.16111/j,0258-7106.2008.06.011
Liu, C., Ma, L., Jiao, P., Sun, X., and Chen, Y. (2010). Chemical Sedimentary Sequence of Lop Nur Salt lake in Xinjiang and its Controlling Factors. Mineral. Deposits 29 (4), 625–630. in Chinese with English abstract. doi:10.16111/j.0258-7106.2010.04.009
Lowenstein, T. K., Li, J., and Brown, C. B. (1998). Paleotemperatures From Fluid Inclusions in Halite: Method Verification and a 100,000 Year Paleotemperature Record, Death Valley, CA. Chem. Geol. 150, 223–245. doi:10.1016/S0009-2541(98)00061-8
Ma, L., Lowenstein, T. K., Li, B., Jiang, P., Liu, C., Zhong, J., et al. (2010). Hydrochemical Characteristics and Brine Evolution Paths of Lop Nor Basin, Xinjiang Province, Western China. Appl. Geochem. 25, 1770–1782. doi:10.1016/j.apgeochem.2010.09.005
Meng, F., Ni, P., Schiffbauer, J. D., Yuan, X., Zhou, C., Wang, Y., et al. (2011). Ediacaran Seawater Temperature: Evidence from Inclusions of Sinian Halite. Precambrian Res. 184 (1-4), 63–69. doi:10.1016/j.precamres.2010.10.004
Meng, F.-W., Ni, P., Yuan, X.-L., Zhou, C.-M., Yang, C.-H., and Li, Y.-P. (2013). Choosing the Best Ancient Analogue for Projected Future Temperatures: A Case Using Data from Fluid Inclusions of Middle-Late Eocene Halites. J. Asian Earth Sci. 67-68, 46–50. doi:10.1016/j.jseaes.2013.02.008
Meng, F.-W., Galamay, A. R., Ni, P., Yang, C.-H., Li, Y.-P., and Zhuo, Q.-G. (2014). The Major Composition of a Middle-Late Eocene Salt lake in the Yunying Depression of Jianghan Basin of Middle China Based on Analyses of Fluid Inclusions in Halite. J. Asian Earth Sci. 85, 97–105. doi:10.1016/j.jseaes.2014.01.024
Niu, X., Jiao, P., Cao, Y., Zhao, Y., and Liu, B. (2015). The Origin of Polyhalite and its Indicating Significance for the Potash Formation in the Bieletan Area of the Qarhan Salt lake, Qinghai. Acta Geol. Sin. 89 (11), 2087–2095. in Chinese with English abstract. doi:10.1111/1755-6724.12308_42
Pan, J., Li, H., Sun, Z., Liu, D., Wu, C., and Yu, C. (2015). Tectonic Response in the Qaidam basin Induced by Cenozoic Activities of the Altyn Tagh Fault. Acta Petrol. Sin. 31 (12), 3701–3712. in Chinese with English abstract. doi:10.00-0569/2015/031(12)-3701-12
Perthuisot, J.-P. (1971). Recent Polyhalite from Sebkha El Melah (Tunisia). Nat. Phys. Sci. 232, 186–187. doi:10.1038/physci232186a0
Peryt, , Pierre, , and Gryniv, (1998). Origin of Polyhalite Deposits in the Zechstein (Upper Permian) Zdrada Platform (Northern Poland). Sedimentology 45, 565–578. doi:10.1046/j.1365-3091.1998.00156.x
Petrichenko, O. I. (1973). Metody doslidzhennya vkluchen’v mineralakh galogennykh porid. Kiev, Ukraine: Naukova Dumka, 92.
Petrychenko, O. I., Peryt, T. M., and Roulston, B. (2002). Seawater Composition during Deposition of Viséan Evaporites in the Moncton Subbasin of New Brunswick as Inferred from the Fluid Inclusion Study of Halite. Can. J. Earth Sci. 39, 157–167. doi:10.1139/e01-066
Pierre, C. (1985). “Polyhalite replacement after gypsum at Ojo de Liebre lagoon (Baja Califormia Mexico): An early diagenesis by mixing of marine brines and continental waters,” in Sixth International Symposium on Salt. Editors B. C Schreiber, and L Harner, 1, 257–265.
Rahimpour-Bonab, H., Shariatinia, Z., and Siemann, M. G. (2007). Origin and Geochemistry of Miocene Marine Evaporites Associated with Red Beds: Great Kavir Basin. Central Iran. Geol. J. 42, 37–54. doi:10.1002/gj.1069
Shen, Z., Cheng, G., Le, C., and Liu, S. (1993). The Division and Sedimentary Environment of Quaternary Salt Bearing Strata in Qaidam Basin. Beijing: Geological Publishing House. in Chinese with English abstract.
Shi, G., Zhang, X., Li, Y., Tang, Q., Miao, W., Li, W., et al. (2016). Hydrochemical Components and Their Distribution Characteristics of Oilfield Waters in No.4 Structure of Lenghu, Qaidam Basin. J. salt lake Res. 24 (2), 19–25. in Chinese with English abstract.
Stewart, F. H. (1965). The Mineralogy of the British Permian Evaporites. Mineral. Mag. J. Mineral. Soc. 34, 460–470. doi:10.1180/minmag.1965.034.268.40
Wang, J., and Lowenstein, T. K. (2017). Anomalously High Cretaceous Paleobrine Temperatures: Hothouse, Hydrothermal or Solar Heating? Minerals 7, 245. doi:10.3390/min7120245
Wang, S., and Zheng, M. (2014). Discovery of Triassic Polyhalite in Changshou Area of East Sichuan Basin and its Genetic Study. Mineral. Deposits 33 (5), 1045–1056. in Chinese with English abstract. doi:10.16111/j.0258-7106.2014.05.013
Wang, M., Yang, Z., Liu, C., Xie, Z., Jiao, P., and Li, C. (1997). Potash Deposits and Their Exploitation Prospects of saline Lakes of the north Qaidam Basin. Beijing: Geological Publishing House. in Chinese with English abstract.
Wei, X., Shao, C., Wang, M., Zhao, D., Cai, K., Jiang, J., et al. (1993). Material Constituents, Depositional Features and Formation Conditions of Potassium-Rich Salt Lakes in Western Qaidam Basin. Beijing: Geological Publishing House. in Chinese with English abstract.
Wei, H. (1987). On Syngenesis, Diagenesis, Anadiagenesis and Supergene Alteration of Triassic gypsum Salt in Quxian, Sichuan. Acat Sedimentol. Sin. 5 (4), 56–65. in Chinese with English abstract.
Yang, Q., Wu, B., Wang, S., Cai, K., and Qian, Z. (1993). Study on Potash deposit of the Qarhan Salt lake. Beijing: Geological Publishing House. in Chinese with English abstract.
Yin, A., Rumelhart, P. E., Butler, R., Cowgill, E., Harrison, T. M., Foster, D. A., et al. (2002). Tectonic History of the Altyn Tagh Fault System in Northern Tibet Inferred from Cenozoic Sedimentation. Geol. Soc. America Bull. 114 (10), 1257–1295. doi:10.1130/0016-7606(2002)114<1257:thotat>2.0.co;2
Yuan, J., Yang, Q., Sun, D., Huo, C., Cai, K., Wang, W., et al. (1995). The Formation Conditions of the Potash Deposits in Qarhan saline lake, Qaidam Basin. China. Beijing: Geological Publishing House. in Chinese with English abstract.
Yuan, X., Zhang, X., Sheng, J., Gao, D., Li, B., Du, Y., et al. (2018). Restriction of Temperature on the Chemical Composition of Brine and Permeability of Aquifer in the saline lake. Acta Geol. Sin. 92 (8), 1724–1732. in Chinese with English abstract. doi:10.1111/acgs.2018.92.issue-4
Zambito, J. J., and Benison, K. C. (2013). Extremely High Temperatures and Paleoclimate Trends Recorded in Permian Ephemeral lake Halite. Geology 41, 587–591. doi:10.1130/g34078.1
Zhang, Y., and Xuan, Z. (1996). Economic Evaluation of Potassium and Magnesium Solid deposit in Kunteyi and Mahai Salt Lake of Qinghai Province. J. Salt Lake Sci. 4 (1), 36–45. in Chinese with English abstract.
Zhang, X.-Y., Meng, F.-W., Li, W.-X., Tang, Q.-L., and Ni, P. (2016). Reconstruction of Late Cretaceous Coastal Paleotemperature from Halite Deposits of the Late Cretaceous Nongbok Formation (Khorat Plateau, Laos). Palaeoworld 25, 425–430. doi:10.1016/j.palwor.2015.11.004
Zhang, P. (1987). Salt Lakes in Qaidam Basin. Beijing: Science Press. in Chinese with English abstract.
Keywords: Kunteyi Playa, Qaidam Basin, polyhalite, sedimentology, mineralogy, halite fluid inclusion, water-salt interaction
Citation: Yuan X, Meng F, Zhang X, Sheng J, Galamay AR, Cheng H, Li Y, Miao W, Li W, Tang Q, Li J and Du Y (2021) Ore-Forming Fluid Evolution of Shallow Polyhalite Deposits in the Kunteyi Playa in the North Qaidam Basin. Front. Earth Sci. 9:698347. doi: 10.3389/feart.2021.698347
Received: 28 April 2021; Accepted: 09 August 2021;
Published: 08 September 2021.
Edited by:
Zhongping Lai, Shantou University, ChinaReviewed by:
Licheng Wang, Institute of Tibetan Plateau Research (CAS), ChinaYangtong Cao, Chinese Academy of Geological Sciences, China
Copyright © 2021 Yuan, Meng, Zhang, Sheng, Galamay, Cheng, Li, Miao, Li, Tang, Li and Du. This is an open-access article distributed under the terms of the Creative Commons Attribution License (CC BY). The use, distribution or reproduction in other forums is permitted, provided the original author(s) and the copyright owner(s) are credited and that the original publication in this journal is cited, in accordance with accepted academic practice. No use, distribution or reproduction is permitted which does not comply with these terms.
*Correspondence: Xiying Zhang, eHl6aGNoaW5hQGlzbC5hYy5jbg==; Jinchang Sheng, c2g5MDFAc2luYS5jb20=