- 1The Department of Gas and Condensate Field Development, National University of Oil and Gas “Gubkin University”, Moscow, Russia
- 2Key Laboratory of Continental Shale Hydrocarbon Accumulation and Efficient Development (Northeast Petroleum University), Ministry of Education, Daqing, China
- 3Institute of Unconventional Oil and Gas, Northeast Petroleum University, Daqing, China
- 4The Ninth Oil Production Plant of Daqing Oilfield Co., Ltd., Daqing, China
- 5The Oil and Gas Production Engineering Service Center, Sinopec Zhongyuan Oilfield, Puyang, China
- 6China Petroleum Engineering and Construction Corporation South-West Branch, Chengdu, China
Residual oil distribution plays a critical role in understanding of the CO2 flooding processes, but its quantitative research for reservoirs with different permeability levels rarely has been comprehensively conducted in the laboratory. This article presents the results of an experimental study on the immiscible CO2 displacement efficiency in different permeability core samples and various oil distribution patterns prior to and after immiscible CO2 flooding. Experiments were conducted on four core samples extracted from the selected oil field with a permeability range from 0.210–66.077 mD. The experimental results show that the immiscible CO2 can mobilize oil in ultralow-permeability environment and achieve a reasonable displacement efficiency (40.98%). The contribution of different oil distribution patterns to displacement efficiency varies in reservoirs with different permeabilities. With the increase of core permeability, the contribution of cluster and intergranular pore oil distribution patterns to displacement efficiency increases. However, the oil displacement efficiency of corner and oil film patterns tends to increase with lower permeability. Therefore, immiscible CO2 flooding is recommended for ultralow-permeability case, especially for reservoirs with larger amount of oil in corner and oil film distribution patterns. The oil displacement efficiency calculated by immiscible CO2 flooding experiment results agrees reasonably well with the core frozen slices observation. The results of this study have practical significance that refers to the effective development of low-permeability reservoirs.
Introduction
The onshore proven undeveloped oil reserves in China are mainly low-permeability and ultralow-permeability reservoirs (Zhao et al., 2011; Duan et al., 2014). There are many researches in this field with different focuses (Li et al., 2017; Zhang et al., 2020; Wang et al., 2021). Field tests show that CO2 flooding is a potential option to improve the oil recovery of low-permeability sandstone reservoirs (Zekri et al., 2006; Bourgeois et al., 2011; Wei et al., 2020a), which is the future development direction of enhanced oil recovery (EOR) in these reservoirs (Su et al., 2011; Hao et al., 2016; Li et al., 2019; Wu et al., 2020).
Determination of residual oil distribution is critically important for developers to identify the potential sweet point, which provides a reasonable basis for the optimum oil recovery method selection. Although residual oil distribution plays a critical role in understanding of the processes which take place within a reservoir during and after CO2 flooding, and many laboratory studies have been performed to evaluate the residual oil distribution, very limited effort has been focused on its quantitative research at the core scale considering different permeability levels. Wang et al. (2013) studied the residual oil distribution in ultralow-permeability reservoir by using nuclear magnetic resonance (NMR) technique, and they considered wettability, pressure gradient, and different water cuts. Iglauer et al. (2014) imaged a sequence of surfactant EOR production steps with a microcomputed tomography, and they limited the study to microscopic sweep efficiency. Residual oil distribution on pore-scale during secondary and tertiary solvent injection was investigated by Shokrlu and Babadagli, (2015), and they studied the efficiency of a miscible-displacement process that is conducted in a cyclic form similar to water-alternating gas. Hu et al. (2017) conducted an experimental study on the mechanism of CO2-immiscible flooding and residual oil distribution in a water-drive reservoir. Al-Bayati et al. (2019) presented the results of an experimental study on immiscible CO2 flooding efficiency in sandstone porous media, but their research did not involve microscale investigation and they focused on the influence of permeability heterogeneity. Wei et al. (2020b) conducted an experimental study, in which the original oil–water distribution feature and that after fluid injection were revealed, and they only covered the case of tight oil reservoirs. Druetta and Picchioni (2020) developed a numerical model to investigate the mechanisms and effectiveness of polymer flooding on the residual oil after waterflooding, and they validated their results by comparing them with the experiments carried out by other authors. Although they conducted numerical simulation, it still provided some perspectives on the experimental investigation of residual oil distribution. Wang et al. (2020) conducted an investigation on the multiphase flow characteristics and EOR mechanism of water-alternating gas (WAG) injection after continuous CO2 injection at the microscale using CT scanning and microelectronic photolithography. They concluded that WAG injection after continuous CO2 injection increased oil recovery by 23.15%, which was dominated by the first and second WAG injection cycles. Wei et al. (2021) also applied the NMR technology to carry out experimental research, in which the microscopic oil displacement mechanism in low-permeability reservoirs was discussed, but they focused on five different EOR methods including CO2 flooding. To our knowledge, the displacement efficiency of immiscible CO2 flooding by considering the contribution of various oil distribution patterns to displacement efficiency in reservoirs with different permeabilities have not been investigated before. In this work, in order to represent different oil recovery scenarios, scales in the different permeability recovery process are considered: from the macro- to microscale systems. The core permeability range is from 0.210–66.077 mD. The efficiency of immiscible CO2 flooding is evaluated by the field core drainage experiments, combined with the frozen slice technology the displacement process of oil by immiscible CO2 is analyzed quantitatively. Thus, this research is dedicated to bridge the existing gap in our knowledge by following a systematic experimental approach and improve fundamental understanding with which it will be possible to develop better production techniques and improved CO2 EOR models.
Experimental Work and Methodology
Coreflooding Equipment, Core Samples, and Chemicals
In immiscible CO2 flooding experiments, we have followed a systematic approach. Figure 1 shows a schematic diagram of the core flooding apparatus used in this study, which consists of the injection system, the displacement system, the pressure control system, and the fluid collection system. The advanced frozen slice technology and the laser confocal scanning microscopy have been applied in order to visualize oil distribution. Experiments were carried out on four core samples from the Daqing Oilfield, China. In the experiments, kerosene and synthetic brine with a salinity of 6778 mg/L were used to simulate oil and formation water. Table 1 presents the results of measured properties of the sandstone core samples. The core sample G-2 with medium permeability was selected in order to be compared with other low-permeability cores.
Experimental Procedure
Specific experimental procedures are as follows: 1) prepare the formation water and oil as mentioned, 2) clean and dry the core sample, 3) put the core sample in the holder and maintain the confining pressure at 3 MPa; vacuum the core for 24 h, 4) inject formation water into the core by hand pump until the pressure at the end of the core remains at 0.5 MPa, 5) inject oil at a constant speed of 0.005 ml/min until no water flows out, 6) place the core in the 90°C thermotank for 48 h, 7) cut 1.5 cm core end to make a frozen slice, 8) set the temperature and the back pressure of the displacement system at 50°C and 6.5 MPa to ensure immiscible condition (Yang et al., 2015; Zhang et al., 2017; Dindoruk et al., 2020); flood the remaining core with a constant speed of 0.01 ml/min for three pore volume (PV) CO2, 9) record injection pressure, oil and gas production every certain time until no oil comes out, 10) cut 1.5 cm core end to make another frozen slice, and 11) use the laser confocal scanning microscopy to visualize residual oil distribution.
The same procedure was applied to all core samples with different permeabilities.
Results and Discussion
In this section, the flooding experiment results are analyzed in order to understand the macroscopic displacement efficiency relative to different permeabilities. The results of residual oil distribution visualization by laser confocal microscopy are discussed to shed some light on the microscale oil distribution and contribution of different distribution patterns to displacement efficiency with all mentioned permeability ranges.
Immiscible CO2 Flooding Experiments: Macroscopic Displacement Efficiency
The displacement efficiency of immiscible CO2 flooding as a function of PV number for core samples with different permeabilities was calculated and is presented in Figure 2. Figure 3 shows the change of percent displacement efficiency and oil saturation post and prior to CO2 flooding with permeability.
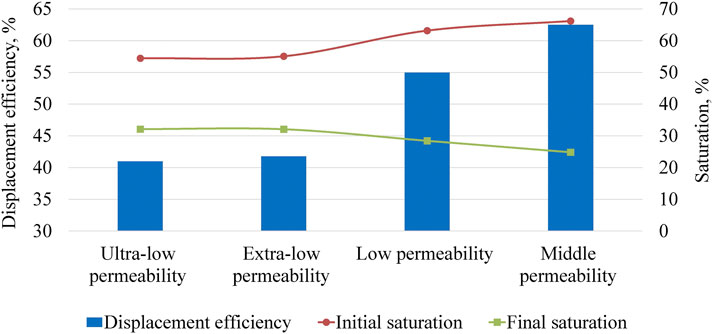
FIGURE 3. Displacement efficiency and oil saturation post and prior to CO2 flooding vs. permeabilities.
As expected, in all runs, displacement efficiency increased with the increasing of pore volume of CO2 injected. At the initial stage of immiscible CO2 flooding, the oil displacement efficiency of cores with different permeability levels did not change significantly, and the ultralow and extra low-permeability cores had even faster growth rate than that of the PV number. Injecting CO2 as an immiscible agent at experimental conditions resulted in final oil displacement efficiency of 40.98 and 41.76% for ultralow and extra low permeability, respectively. There was only a slight change in displacement efficiency when the permeability increased from ultralow to extra low, but immiscible CO2 flooding performance improved significantly at higher permeability. The following oil displacement efficiency was obtained: 54.98 and 62.49% corresponding to low and medium permeability, respectively. At the higher permeability, the injected CO2 succeeded to contact more oil in place and managed to displace and/or extract much more oil than in the lower permeability case. The displacement efficiency of immiscible CO2 flooding in medium permeability core was increased by 52.49, 49.64, and 13.66% compared with ultralow-, extra low-, and low-permeability core samples, respectively.
The increase of displacement efficiency began to slow down after about 0.35 PV of CO2 had been injected for ultralow and extra low-permeability cores, and 0.9 PV for higher permeability cores. Moreover, in the case of medium permeability, higher oil saturation prior to CO2 flooding and lower oil saturation post flooding were observed. Nevertheless, experimental results show that the immiscible CO2 can mobilize oil in ultralow-permeability environment with a reasonable recovery.
Quantitative Observation of Core-Frozen Slices: Microscopic Displacement Efficiency
We performed a series of experiments as mentioned above for quantitative observation of different residual oil distribution patterns on core frozen slices with different permeabilities. The frozen slices of ultralow-permeability core prior to and post-immiscible CO2 flooding are shown in Figure 4. Three observation points were selected evenly on each of the slices. In order to determine the oil distribution patterns and analyze their contribution to displacement efficiency, microphoto images of oil saturation were taken prior to and after CO2 flood, which are shown in Figure 5. Figures 5A–C show oil distribution of the three observation points before the CO2 flood, and Figures 5D–F reveal three observation points oil distribution after CO2 flood. We can see a significant decrease of oil saturation after CO2 floods.
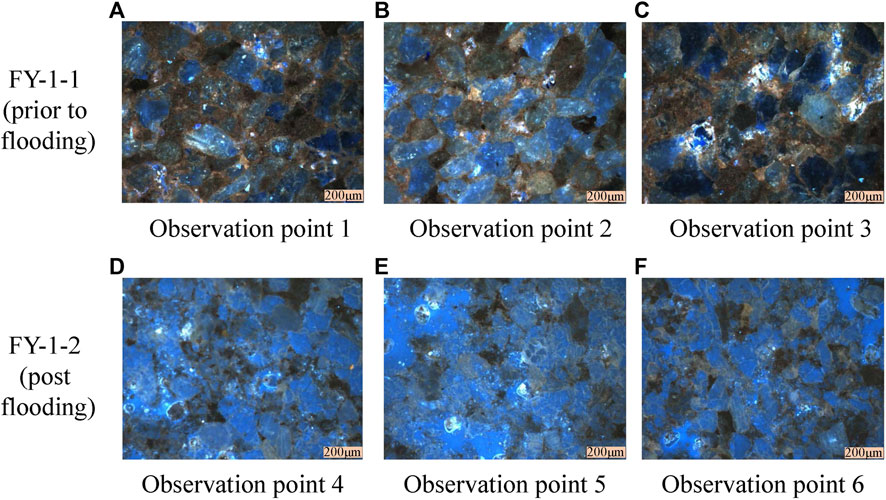
FIGURE 5. Oil saturation prior to and post CO2 floods in reservoir core with ultralow permeability. (A–C: observation points before the CO2 flood, D–F: observation points after CO2 flood).
The image processing software developed by our team is used for quantitative analysis of oil distribution in different pore structures. In this study, we found five dominant oil distribution patterns, namely, cluster, pore-throat, corner, intergranular pore, and oil film. Cluster distribution pattern refers to the oil trapped in the large pores connected by small pores and narrow throats. In the pore-throat distribution pattern, oil is snapped off at the neck (narrow throat) and may become residual under the current displacement conditions. In corner distribution pattern, oil is trapped in the end of the pores (the dead corner) due to poor connectivity. In the intergranular pore distribution, oil is in the dispersed phase due to the existence of fine particles in pores. Oil film distribution pattern refers to the oil located on the particle surface of the channel wall in the form of thin layer.
Figure 6 presents the software processing result of the residual oil distribution in ultralow-permeability reservoirs. It can be seen that all the five oil distribution patterns (Figure 6A—cluster, pore-throat, and corner; Figure 6B—intergranular pore; Figure 6C—oil film) exist in ultralow-permeability reservoirs but with different proportions. Similarly, we can calculate the oil distribution pattern of other cores before and after displacement. Figure 7 shows the proportions of different oil distribution patterns to total pore volume prior to and post CO2 floods, then we can determine the production contribution from every oil distribution pattern.
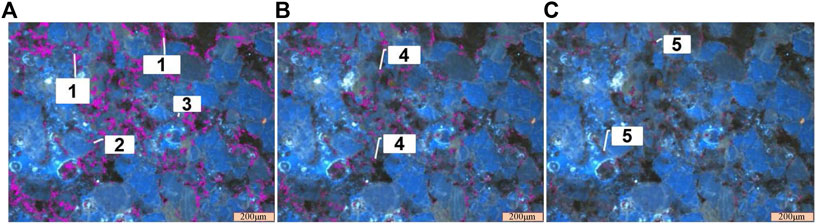
FIGURE 6. Visualization of different residual oil distribution patterns of core sample FY-1 (A: 1—cluster; 2—pore-throat; 3—corner; B: 4—intergranular pore; C: 5—oil film).
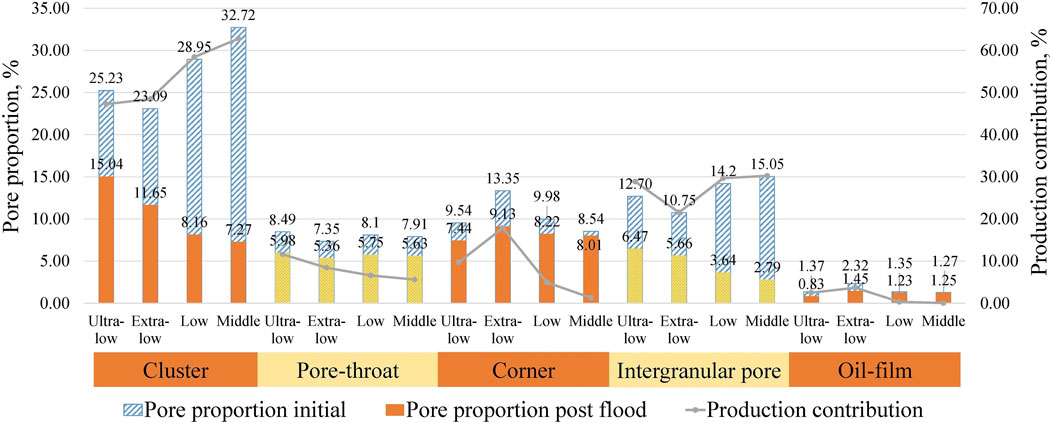
FIGURE 7. Proportions of oil distribution patterns to total pore volume before and after CO2 floods, their production contribution.
From Figures 6, 7, we can learn that various oil distribution patterns had different contributions to displacement efficiency in reservoirs with different permeabilities. The most common oil distribution pattern for all core samples was cluster, which had the biggest contribution to oil production. The distribution pattern of intergranular pore also had a higher production contribution. Oil did not tend to be stored in oil film pattern, which was mainly affected by the pore structure. Generally speaking, the larger the permeability was, the greater the contribution of cluster and intergranular distribution patterns to the oil production and the higher the ultimate oil displacement efficiency. The production contribution of the cluster distribution pattern in medium permeability core was increased by 32.89, 29.56, and 7.44% when compared with ultralow-, extra low-, and low-permeability core samples, respectively.
Compared with other core samples, the in situ oil saturation of core sample with extra low permeability was more concentrated in the corner and oil film distribution patterns, resulting in relatively low oil mobility. Therefore, although its permeability was much higher than that of ultralow-permeability core, only slight increase in displacement efficiency was observed, and this phenomenon should be studied on case-by-case basis using the formation fluids and rocks under reservoir conditions. In general, oil displacement efficiency of corner and oil film patterns tends to decrease with higher permeability. In core samples with ultralow permeability, CO2 can enter into smaller pores, expand the sweep area, and improve the displacement efficiency of oil in pore-throat, corner, and oil film distribution pattern. There was no big change between cores with different permeabilities in the contribution of pore-throat oil distribution pattern to displacement efficiency.
The displacement efficiencies of immiscible CO2 flooding in reservoir core samples with different permeabilities by microscopic oil distribution were 37.62% (ultralow), 41.52% (extra low), 56.86% (low), and 61.90% (medium). The average relative error of oil displacement efficiency calculated by immiscible CO2 flooding experiment results and by frozen slices observation was 3.28%.
Conclusion
The experiments described in this article represent immiscible CO2 floods on core samples with different permeabilities. From the experimental studies presented above, we can draw the following conclusions:
1. Immiscible CO2 flooding performance on reservoir cores improves with the increase of permeability. Compared with ultralow-, extra low-, and low-permeability core samples from the target oilfield, the displacement efficiency of immiscible CO2 flooding on medium permeability core was increased by 52.49, 49.64, and 13.66%, respectively.
2. In all core frozen slices, five oil distribution patterns with different proportions were observed. The experimental results showed that the production contribution of different oil distribution patterns varies in reservoirs with different permeability levels. Compared with other oil distribution patterns, there was no big change between cores with different permeabilities in the production contribution of pore-throat distribution pattern.
3. The contribution of cluster and intergranular pore-oil distribution patterns to displacement efficiency was more pronounced in cases with higher permeability. The production contribution of cluster distribution pattern in medium permeability core sample during immiscible CO2 flooding was increased by 32.89, 29.56, and 7.44% when compared with ultralow-, extra low-, and low-permeability cores, respectively. Therefore, the pore proportion of cluster and intergranular pore oil distribution patterns is an important indicator for efficient development of higher permeability reservoirs.
4. The oil displacement efficiency of corner and oil film distribution patterns tends to increase with lower permeability, where CO2 can enter the smaller pore structures and expand the sweep area. In ultralow-permeability reservoirs, the immiscible CO2 can mobilize oil in all five distribution patterns and achieve reasonable displacement efficiency (40.98%). Therefore, immiscible CO2 flooding is recommended for ultralow-permeability case, especially for reservoirs with larger amount of oil in corner and oil film distribution patterns.
Data Availability Statement
The original contributions presented in the study are included in the article/Supplementary Material, further inquiries can be directed to the corresponding authors.
Author Contributions
YY proposed the research. X-FZ, C-XL and XK prepared figures and tables, and interpreted the structural data. YY, A-LW and J-G W developed the main ideas. L-YS participated in the field work. X-FZ and A-LW participated in the lab work. YY, X-FZ, A-LW and J-G W contributed to writing the original manuscript. L-YS, C-XL and XK contributed to revising the manuscript. All co-authors actively contributed to the manuscript with comments, ideas, and suggestions.
Funding
This work was funded by the Youth Science Foundation of Northeast Petroleum University (Grant No2019QNL-22).
Conflict of Interest
Author L-YS was employed by the company The Ninth Oil Production Plant of Daqing Oilfield Co., Ltd; author C-XL was employed by the company The Oil and Gas Production Engineering Service Center, Sinopec Zhongyuan Oilfield; author XK was employed by the company China Petroleum Engineering and Construction Corporation South-West Branch.
The remaining authors declare that the research was conducted in the absence of any commercial or financial relationships that could be construed as a potential conflict of interest.
References
Al-Bayati, D., Saeedi, A., Myers, M., White, C., and Xie, Q. (2019). An Experimental Investigation of Immiscible-CO2-Flooding Efficiency in Sandstone Reservoirs: Influence of Permeability Heterogeneity. SPE Reservoir Eval. Eng. 22 (3), 990–997. doi:10.2118/190876-PA
Bourgeois, M. J., Thibeau, S., and Guo, J. (2011). Modelling Residual Oil Saturation in Miscible and Immiscible Gas Floods by Use of Alpha-Factors. Vienna, Austria: Paper presented at the SPE EUROPEC/EAGE Annual Conference and Exhibition. doi:10.2118/143379-MS
Dindoruk, B., Johns, R., and Orr, F. M. (2020). Measurement of Minimum Miscibility Pressure: A State of the Art Review. Virtual: Paper presented at the SPE Improved Oil Recovery Conference. doi:10.2118/200462-MS
Druetta, P., and Picchioni, F. (2020). Influence of Physical and Rheological Properties of Sweeping Fluids on the Residual Oil Saturation at the Micro- and Macroscale. J. Non-Newtonian Fluid Mech. 286, 104444. doi:10.1016/j.jnnfm.2020.104444
Duan, B., Yin, Y., Hu, Y., Yao, W., Zhu, Z., and Nie, C. (2014). Study on the Fluid's Flowing Law in the Different-Level Pores of Low Permeability Reservoir. Sci. Technol. Eng. 14, 26–30. doi:10.3969/j.issn.1671-1815.2014.10.006 (in Chinese with English abstract).
Hao, H., Hou, J., Zhao, F., Hou, L., Wang, Z., and Song, Z. (2016). Experiments of Gas Channeling Control during CO2 Immiscible Flooding in Low Permeability Reservoirs with Heterogeneity. Pet. Geology. Recovery Efficiency 23, 95–100. doi:10.3969/j.issn.1009-9603.2016.03.017 (in Chinese with English abstract).
Hu, W., Lu, C., Wang, R., Yang, Y., and Wang, X. (2017). Mechanism of CO2 Immiscible Flooding and Distribution of Remaining Oil in Water Drive Oil Reservoir. Pet. Geology. Recovery Efficiency 24, 99–105. doi:10.13673/j.cnki.cn37-1359/te.2017.05.015 (in Chinese with English abstract).
Iglauer, S., Sarmadivaleh, M., Geng, C., and Lebedev, M. (2014). In-situ Residual Oil Saturation and Cluster Size Distribution in Sandstones after Surfactant Flooding Imaged with X-ray Micro-computed Tomography. Doha, Qatar: Paper presented at the International Petroleum Technology Conference. doi:10.2523/IPTC-17312-MS
Li, R., Liao, X., Zou, J., Zhang, H., Zhou, X., Mu, L., et al. (2019). Experimental and Numerical Studies on Asphaltene Deposition Distribution during CO2 Flooding in Ultra-low Permeability Reservoirs. Houston, Texas, USA: Paper presented at the Carbon Management Technology Conference. doi:10.7122/CMTC-552770-MS
Li, Y., Jia, D., Rui, Z., Peng, J., Fu, C., and Zhang, J.(2017). Evaluation Method of Rock Brittleness Based on Statistical Constitutive Relations for Rock Damage. J. Pet. Sci. Eng.. 153, 123–132. doi:10.1016/j.petrol.2017.03.041
Shokrlu, Y. H., and Babadagli, T. (2015). Pore-scale Investigation of Phase Distribution and Residual-Oil Development during Secondary and Tertiary Solvent Injection. SPE Reservoir Eval. Eng. 18, 39–52. doi:10.2118/173180-PA
Su, Y., Wu, C., Zhang, Q., and Wu, X. (2011). Characteristics of Immiscible CO2 Displacement in Extremely Low Permeability Reservoir. J. Chongqing Univ. 34, 53–57. doi:10.11835/j.issn.1000-582X.2011.04.010 (in Chinese with English abstract).
Wang, L., He, Y., Wang, Q., Liu, M., and Jin, X. (2020). Multiphase Flow Characteristics and EOR Mechanism of Immiscible CO2 Water-Alternating-Gas Injection after Continuous CO2 Injection: A Micro-scale Visual Investigation. Fuel. 282, 118689. doi:10.1016/j.fuel.2020.118689
Wang, X., Yang, Z., Li, H., and Li, Z. (2013). Microscopic Distribution of Remaining Oil of Ultra-low Permeability Reservoir by Using NMR Technique. J. Basic Sci. Eng. 21, 702–709. doi:10.3969/j.issn.1005-0930.2013.04.012 (in Chinese with English abstract).
Wang, Z., Zeng, L., Yu, J., Zhang, Z., Yang, S., and Liu, Y. (2021). Key Geological Factors Controlling Oil Displacement Efficiency of CO2 Injection in Low-Permeability Reservoirs. Energy Explor. Exploit. 39 (3), 993–1009. doi:10.1177/0144598721995609
Wei, J., Li, J., Zhou, X., and Zhang, X. (2020a). Effect of Pressure and CO2 Content on the Asphaltene Precipitation in the Light Crude Oil. Pet. Sci. Technol. 38 (2), 116–123. doi:10.1080/10916466.2019.1684947
Wei, J., Zhou, X., Zhou, J., Li, J., and Wang, A. (2020b). Recovery Efficiency of Tight Oil Reservoirs with Different Injection Fluids: An Experimental Investigation of Oil-Water Distribution Feature. J. Pet. Sci. Eng. 195, 107678. doi:10.1016/j.petrol.2020.107678
Wei, J., Zhou, J., Li, J., Zhou, X., Dong, W., and Cheng, Z., (2021). Experimental Study on Oil Recovery Mechanism of CO2 Associated Enhancing Oil Recovery Methods in Low Permeability Reservoirs. J. Pet. Sci. Eng. 197, 108047. doi:10.1016/j.petrol.2020.108047
Wu, S., Fan, T., Zhao, L., Peng, H., Wang, B., and Ma, X., (2020). Miscible CO2 Flooding Simulation with a Compositional Model in Middle East Carbonate Reservoir. Virtual: Paper presented at the SPE Asia Pacific Oil & Gas Conference and Exhibition. doi:10.2118/202259-MS
Yang, S., Lian, L., Yang, Y., Li, S., Tang, J., Ji, Z., et al. (2015). Molecular Optimization Design and Evaluation of Miscible Processing Aids Applied to CO2 Flooding. Xinjiang Pet. Geology. 36, 555–559. doi:10.7657/XJPG20150510 (in Chinese with English abstract).
Zekri, A. Y., Almehaideb, R. A., and Shedid, S. A. (2006). Displacement Efficiency of Supercritical CO2 Flooding in Tight Carbonate Rocks under Immiscible Conditions. Vienna, Austria: Paper presented at the SPE Europec/EAGE Annual Conference and Exhibition. doi:10.2118/98911-MS
Zhang, F., An, M., Zhang, L., Fang, Y., and Elsworth, D. (2020). Effect of Mineralogy on Friction-Dilation Relationships for Simulated Faults: Implications for Permeability Evolution in Caprock Faults. Geosci. Front. 11 (02), 439–450. doi:10.1016/j.gsf.2019.05.014
Zhang, X., Chen, H., Li, B., Mei, Y., Yang, G., and Tang, H. (2017). Discussion on Controlling Conditions of Optimal Near-Miscible Flooding by Impure CO2 in Low Permeability Reservoir. China Offshore Oil and Gas 29, 75–78. doi:10.11935/j.issn.1673-1506.2017.06.009 (in Chinese with English abstract).
Keywords: residual oil distribution, immiscible CO2 flooding, displacement efficiency, frozen slice technology, ultralow permeability
Citation: Yang Y, Zhou X-F, Sun L-Y, Wang A-L, Wei J-g, Li C-X and Ke X (2021) Immiscible CO2 Flooding Efficiency in Low-Permeability Reservoirs: An Experimental Investigation of Residual Oil Distribution. Front. Earth Sci. 9:693960. doi: 10.3389/feart.2021.693960
Received: 12 April 2021; Accepted: 10 May 2021;
Published: 28 May 2021.
Edited by:
Yuwei Li, Liaoning University, ChinaReviewed by:
Zhaojie Song, China University of Petroleum, ChinaPengliang Yu, The University of Auckland, New Zealand
Copyright © 2021 Yang, Zhou, Sun, Wang, Wei, Li and Ke. This is an open-access article distributed under the terms of the Creative Commons Attribution License (CC BY). The use, distribution or reproduction in other forums is permitted, provided the original author(s) and the copyright owner(s) are credited and that the original publication in this journal is cited, in accordance with accepted academic practice. No use, distribution or reproduction is permitted which does not comply with these terms.
*Correspondence: An-Lun Wang, 969659914@qq.com