- 1Department of Earth Sciences, Durham University, Durham, United Kingdom
- 2School of Earth and Ocean Sciences, Cardiff University, Cardiff, United Kingdom
- 3School of Geographical and Earth Sciences, University of Glasgow, Glasgow, United Kingdom
The chemical and isotopic compositions of volcanic arc lavas often show evidence for involvement of a sedimentary component during magma genesis. Determining where this sedimentary component is added to arc magmas is of vital importance for constraining the extent to which sediments and volatiles are recycled at subduction zones. Lavas from Martinique in the Lesser Antilles arc have wide ranging isotopic compositions extending to highly radiogenic values (e.g. 87/Sr/86Sr up to ∼0.710) that could, in principle, be explained by sediment addition to the mantle source or by crustal assimilation in the upper plate. We use Sr isotopic compositions of plagioclase from Martinique plutonic xenoliths to provide evidence supporting the crustal assimilation hypothesis. Plagioclase from plutonic xenoliths formed in the mid-crust (∼12 km) show a restricted range of unradiogenic Sr isotope ratios (87Sr/86Sr = 0.7041–0.7042) whereas plagioclase from upper crustal plutonic xenoliths (∼6 km) show greater intra-sample variation and more radiogenic Sr isotopic compositions up to 87Sr/86Sr = 0.7047. This trend is also observed in plutonic xenolith whole rock 87Sr/86Sr. Combined, these results indicate that the range of Sr isotope compositions becomes larger and more radiogenic in Martinique magmas as a result of sediment assimilation at shallow crustal levels. This is supported by Assimilation-Fractional Crystallization modeling, which shows that assimilation of chemically and isotopically heterogenous crustal sediments can produce the isotopic variation in Martinique plutonic xenoliths and lavas. Our results highlight the importance of constraining crustal contributions from the upper plate before using arc lava geochemistry to quantify sediment and volatile recycling at subduction zones and assessing potential heterogeneity of arc mantle sources.
Introduction
The chemical and isotopic compositions of arc magmas are generally considered to reflect contributions from the mantle wedge combined with fluids and/or melts derived from subducting oceanic crust and sediments (e.g. Kelemen et al., 2003 and references therein). Many arc magmas have trace element and isotopic compositions displaced from MORB toward crustal compositions (e.g. Davidson et al., 2005), which may be explained by input from the subducting slab, or assimilation of arc crust during magma ascent and storage. Geochemical constraints upon the relative importance of slab vs. upper plate contributions to arc magmas have clear implications for understanding sediment recycling at subduction zones. Trace element contents of lavas have been combined with estimates of the trace element input from subducting sediments to perform mass balance calculations to determine the extent of sediment recycling (e.g. Plank and Langmuir, 1993). However, modification of trace element concentrations in arc lavas by assimilation of crustal material can cause misleading estimates of the amount of sediment recycled into the mantle. Similar calculations have been used to assess recycling of volatiles e.g. CO2 at subduction zones (Bebout et al., 2014 and references therein). Assimilation of crustal sediments also modifies the volatile budget of arc magmas (e.g. Chadwick et al., 2007; Deegan et al., 2010), which in turn affects eruption explosivity, hence it is vital to constrain the relative contributions of the overlying vs. subducting plate to quantify recycling in arc systems.
Lavas from the intra-oceanic Lesser Antilles arc (LAA, Figure 1) are notable for their extreme range in radiogenic isotopic compositions, extending from MORB-like signatures to highly radiogenic, “crustal/sedimentary” values (Figure 2; Davidson, 1985; Davidson, 1986; Davidson et al., 1987; Davidson and Harmon, 1989; Van Soest, 2000; Labanieh et al., 2010; Bezard et al., 2014). Lavas show radiogenic isotopic variation both along the arc and at individual volcanic centers (Figure 3). Lavas from the island of Martinique span the whole Sr, Nd and Pb isotopic range of the arc (Figures 2, 3, Nd isotopes not shown), and therefore provide an ideal suite to investigate the cause(s) of these variations.
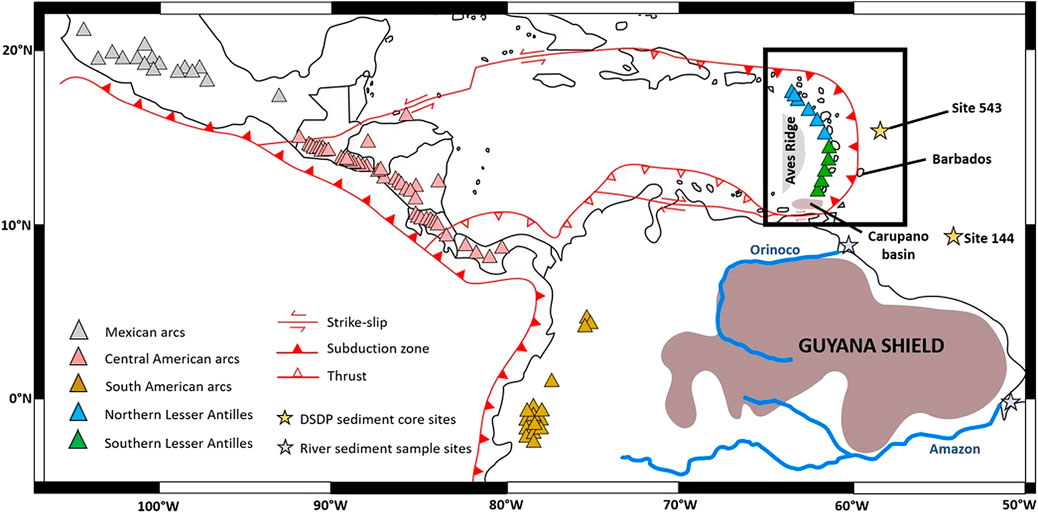
FIGURE 1. Tectonic setting of Lesser Antilles arc (outlined by black box) and nearby arcs in Central-South America. The sampling sites of sediments discussed in Potential Sediment Sources are labeled. Locations of Site 543 and Site 144 from Carpentier et al. (2008). Locations for Orinoco and Amazon samples from Allegre et al. (1996); Parra et al. (1997) and Rousseau et al. (2019).
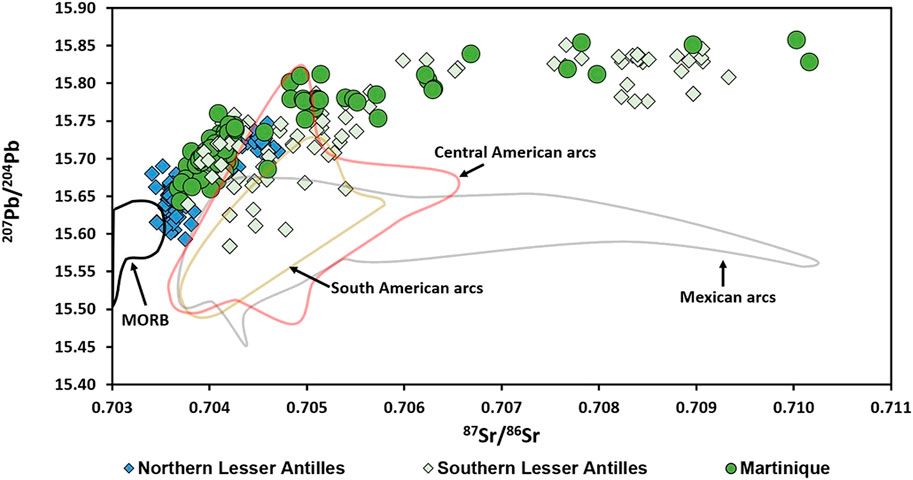
FIGURE 2. 87Sr/86Sr vs. 207Pb/204Pb of lavas from the Lesser Antilles arc, compared to nearby arcs from Central-South America shown in Figure 1. For all data points, uncertainty (2 SE) is smaller than symbol size. Northern Lesser Antilles = Saba to Dominica. Southern Lesser Antilles = Martinique to Grenada (Martinique data shown separately). Data sources: Northern Lesser Antilles—Van Soest et al. (2002); Lindsay et al. (2005); Toothill et al. (2007); DuFrane et al. (2009); Davidson and Wilson (2011). Southern Lesser Antilles–Thirlwall and Graham (1984); Davidson (1986); White and Dupre (1986); Smith et al. (1996); Thirlwall et al. (1996); Turner et al. (1996); Heath et al. (1998); Labanieh et al. (2010); Huang et al. (2011); Bezard et al. (2014). Southern American, Central American and Mexican arc data from the Georoc database (http://georoc.mpch-mainz.gwdg.de/georoc/). MORB data from the PetDB database (www.earthchem.org/petdb) for Mid-Atlantic Ridge basalts 30° either side of the equator. Labanieh et al. (2010) found that the difference between measured and age corrected isotope ratios for the oldest lavas in the arc (∼25 Ma, from Martinique) was negligible. Since majority of lavas in the LAA are much younger than 25 Ma, age correction is considered negligible by majority of authors and would not alter the trend shown.
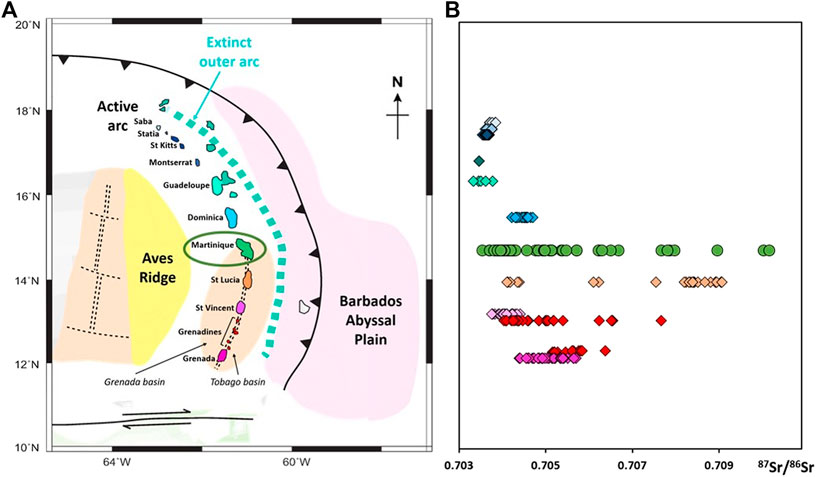
FIGURE 3. (A) Map of Lesser Antilles arc (region marked by black outlined box on Figure 1) modified from Allen et al. (2019). (B) Compilation of along arc variation in 87Sr/86Sr of lavas. For all data points, uncertainty (2 SE) is smaller than symbol size. Data sources are the same as Figure 2. Note that the same along arc trends are also observed in the Nd and Pb isotopic systems.
The large isotopic variation in the LAA, and in particular Martinique lavas, has been interpreted as the result of variable sediment addition (White and Dupre, 1986; Davidson et al., 1987; Carpentier et al., 2008). However, there exists significant debate as to whether the sedimentary component is 1) added to the mantle wedge from subducting sediments (White and Dupre, 1986; Carpentier et al., 2008; Labanieh et al., 2010; Labanieh et al., 2012; Hu et al., 2021), or 2) incorporated during magma ascent and storage in the upper plate, via assimilation of unsubducted crustal sediments (Davidson, 1985, Davidson, 1986; Davidson et al., 1987; Davidson and Harmon, 1989; Smith et al., 1996; Van Soest et al., 2002; Davidson and Wilson, 2011; Bezard et al., 2014).
The majority of previous studies have used whole rock radiogenic isotopic compositions of arc lavas to assess the origin of the crustal signature, but there are potential issues with this approach. Crystals formed in different parts of a magma plumbing system record the isotopic composition of the magmas from which they grew. Whole rock compositions average the isotopic signatures of individual components e.g. crystals and groundmass, hence evidence for changes in isotopic composition of magmas during storage in the crust (recorded by the crystals) may be lost. The use of lavas may also be problematic since late stage processes such as magma mixing and/or subaerial alteration have the potential to overprint processes occurring deeper in the crust (e.g. Davidson et al., 2007). In addition, the mid to lower crust of the upper plate is probably a key location for the differentiation of arc lavas (e.g. the “Deep Crustal Hot Zone” of Annen et al., 2006). Finally, arc lavas travel from their mantle source through the crust before they are erupted. Even when fresh arc lava chemistry unambiguously indicates a sedimentary component, it is extremely challenging to determine where that component was incorporated.
To overcome these ambiguities, this study explores plagioclase from plutonic xenoliths and their Sr isotopic compositions. The LAA is notable for its abundant plutonic xenoliths (e.g., Arculus and Wills, 1980; Melekhova et al., 2019), which represent deeper parts of the magmatic plumbing system. We focus on samples from Martinique due to its well-established variability in whole rock lava compositions and compare our findings with the more isotopically restricted island of St Vincent (Figure 3). These Martinique plutonic xenoliths record crystallization conditions at 21—6 km depth in the crust (Cooper et al., 2016; Melekhova et al., 2019) and, therefore, have the potential to record the isotopic composition of magmas throughout the crust. Previous studies have employed the 87Sr/86Sr isotopic composition of plagioclase phenocrysts in erupted lavas to assess crustal assimilation at arc volcanoes (e.g. Davidson et al., 2007; Bezard et al., 2014; Ginibre and Davidson, 2014; Waight and Tornqvist, 2018). Plagioclase is ubiquitous in Lesser Antilles plutonic xenoliths. Strontium concentrations in plagioclase are high (generally >250 μg/g, Cooper et al., 2016), thus precise 87Sr/86Sr measurements can be achieved on microgram sized pieces extracted from crystals (Charlier et al., 2006). Here, we determine the Sr isotopic composition of crystal domains within individual plagioclase crystals in plutonic xenoliths from a range of depths in the arc crust. We aim to test whether or not the plutonic roots of Martinique already display a radiogenic Sr isotopic composition. A radiogenic composition (similar to the higher 87Sr/86Sr lavas) is expected if subducted sediments are incorporated into the mantle source, whereas less radiogenic compositions would support a dominant role for crustal contamination within the upper plate.
Materials and Methods
Sample Suite
Plutonic xenoliths were collected between 2008 and 2017 during a series of field campaigns to the Lesser Antilles. Five plutonic xenoliths from Martinique (MQ1, MQ6, MQ35, MQ48, and MQ59) and one from St Vincent (VS36) were chosen as the focus of this study. These samples form part of a wider sample set characterized in terms of petrology, mineral chemistry and whole rock major and trace elements by Cooper et al. (2016) and Tollan et al. (2012), providing key context for plagioclase isotopic analyses. We also present whole rock Sr, Nd, and Pb isotope data for 15 of the characterized Martinique plutonic xenoliths to give further context to our plagioclase Sr isotope data. Samples were collected ex situ, mostly from river beds on the flanks of volcanic edifices. The Martinique xenoliths are inferred to be derived from eruptions of either Mt Pelee (0–126 ka) or Mt Conil (127–550 ka), the most recent phases of volcanic activity on the island (Germa et al., 2011; Cooper et al., 2016). The St Vincent xenolith is inferred to have been erupted at La Soufriere volcano (<3.6 ka, Heath et al., 1998; Tollan et al., 2012).
Samples show good textural equilibration (120° grain contacts, minor or no evidence for deformation). The relative equilibration depths of selected xenoliths were estimated by mineral geobarometry and comparison to experimental studies (Cooper et al., 2016; Melekhova et al., 2019). Calculated depths vary from 21 to 6 km, though large uncertainty exists on these estimates (typically ∼6 km). This suggests that the plutonic xenoliths represent portions of the magmatic plumbing system covering the middle to upper crust.
Plagioclase from Martinique lava sample M8321 (87Sr/86Sr = 0.706246; Davidson, 1986) were also analyzed for Sr isotopic composition. This is one of the most radiogenic Martinique lavas with plagioclase crystals large enough for isotopic analysis. The lava is from the emergent/subaerial phase of the Intermediate arc, dated at 9.2–8.4 Ma (Germa et al., 2011), in a period when the most radiogenic (highest 87Sr/86Sr) lavas in Martinique were erupted (Davidson, 1986; Labanieh et al., 2010).
Sampling and Analytical Techniques
Details of sample and target crystal selection, micro-milling and analytical procedures are outlined briefly below and are described in detail in Supplementary Files S1,S2. All laboratory procedures were carried out at Durham University, in the G.J. Russell Electron Microscopy Facility and the Arthur Holmes Isotope Geology Laboratory.
Plutonic Xenolith Sample Selection
A subset of the plutonic xenoliths characterized by Cooper et al. (2016), with a variety of mineral assemblages, were chosen for plagioclase isotopic analyses. Selected plagioclase crystals cover a range of anorthite contents (Supplementary File S1 and Table S1.1). The plutonic xenolith from St Vincent was included to compare a nearby volcanic center with restricted isotopic variation (Heath et al., 1998; Figure 3).
Optical microscopy of 100 µm thick sections was used to identify suitable plagioclase crystals for micro-milling and isotopic analysis. Potential target crystals were defined as containing minimal cracks and inclusions, and distinct core and rim zones if present. Electron backscatter imaging (BSE) and chemical mapping using a Hitachi SU70 SEM was used to confirm the presence/lack of chemical zoning (details in Supplementary File S1). BSE images and chemical maps were then assessed to identify the most suitable plagioclase domain(s) for microanalysis.
Micro-Milling
Material was recovered from plagioclase in situ, using a New Wave Micromill. The micro-milling and sample recovery procedure closely followed Charlier et al. (2006) except that to maximize sample recovery trenches were milled rather than a series of conical holes as in the former study. Sampling trenches were 60 µm deep and of sufficient length to yield ≥3 ng Sr for analysis, based on calculations of volume of material removed during milling and assuming a minimum Sr concentration of 250 μg/g (based on plagioclase Sr concentration data from Cooper et al., 2016). During micromilling, care was taken to avoid inclusions wherever possible.
Chemical Separation and Measurement
Milled material was digested using 150 µl Teflon distilled (TD) 29 M HF:50 µl TD 16 M HNO3 and refluxed overnight on a hotplate at 100°C. The solution was then evaporated to incipient dryness at 100°C and the residue taken up in 50 μl TD 16 M HNO3. This solution was returned to the hotplate for 90 min, evaporated, and re-dissolved in 50 μl TD 16 M HNO3. The solution was evaporated, taken up in 400 μl TD 3 M HNO3 and refluxed at 100°C prior to Sr separation.
Strontium separation closely follows the procedure outlined in Charlier et al. (2006). Briefly, Sr was separated and purified via two passes through pre-cleaned Eichrom Sr spec resin. Samples were loaded on to the resin in 400 μl TD 3 M HNO3. Matrix elements were eluted with 3 × 250 μl TD 3 M HNO3 followed by Sr elution with 400 μl Milli-Q water. The Sr solutions were subsequently evaporated at 100°C. All samples and standards were analyzed for Sr isotope ratios on a Thermo Fisher Scientific Triton Plus Thermal ionization Mass Spectrometer (TIMS). Full details of the measurement protocol are given in Supplementary File S2.
External precision and accuracy were assessed via multiple measurements of NIST Sr standard NBS 987 throughout the period of analyses, of varying load sizes from 1 to 10 ng (i.e. of similar size to the samples). The average 87Sr/86Sr = 0.710259 ± 0.000031 (2σ, n = 33) agrees well with the accepted value of 87Sr/86Sr = 0.710248 ± 0.000023 2σ (Thirlwall, 1991). Method accuracy was also assessed via seven repeat analyses of ∼10 ng Sr loads of USGS reference material BCR-2. To avoid potential heterogeneity with the BCR-2 powder a single large digestion was made from which repeat 10 µl aliquots were taken and diluted with 390 µl TD 3 M HNO3 prior to Sr separation. The average 87Sr/86Sr = 0.705041 ± 0.000063 (2σ, n = 7) agrees within uncertainty of the value of 87Sr/86Sr = 0.705013 ± 0.000010 2σ obtained with TIMS analyses of BCR-2 by Weis et al. (2006).
Total procedural blanks measured 5–20 pg (n = 6) and column blanks 3–50 pg (n = 9), with all but one below 25 pg. Concentration checks on aliquots of sample solutions taken after Sr separation indicated that majority of samples contained >3 ng Sr, therefore blanks are typically <1% of sample size and any blank correction would be negligible.
Whole Rock Sr-Nd-Pb Isotopes
The plutonic xenoliths analyzed for major and trace element compositions by Cooper et al. (2016) were previously powdered and whole rock Sr, Nd, and Pb isotopes were determined on these powders using standard HF dissolution techniques and established column separation and measurement techniques. A more detailed description, including standard data, is found in Supplementary File S2.
Results
Petrography
Petrography and Classification of Xenoliths
Plutonic xenoliths are identified as either “cumulates” or “non-cumulate gabbros” based on chemical and textural characteristics (Cooper et al., 2016). If the whole rock and mineral chemistry (and texture) are consistent with the xenolith originating as a subtractive assemblage it is identified as a cumulate. Otherwise, xenoliths are identified as non-cumulate gabbros (NCG), indicative of origin as solidified equivalents of potentially eruptible lavas, formed “without movement of crystals relative to host melts” (Cooper et al., 2016; Cooper et al., 2019). Based on these definitions, this study analyzed the Sr isotopic composition of plagioclase in four cumulates and two NCG’s. Mineral phases include plagioclase (Pl), clinopyroxene (CPX), orthopyroxene (OPX), amphibole (Hornblende, Amph/Hbl), olivine (Ol), and oxides (Ox), which is typical of Lesser Antilles plutonic xenolith assemblages (Arculus and Wills, 1980; Tollan et al., 2012; Stamper et al., 2014; Cooper et al., 2016, Cooper et al., 2019; Melekhova et al., 2019). Xenoliths are classified based on mineral assemblage using the scheme of Streckeisen (1976), and textural features. Those in which plagioclase were analyzed cover almost the full range of plutonic xenolith types from Martinique identified by Cooper et al. (2016). The single investigated lava is a two-pyroxene andesite typical of Martinique (e.g. intermediate subaerial lavas from Davidson, 1986).
A detailed petrographic description of each sample is given in Supplementary File S1. The classification and petrographic observations listed for each plutonic xenolith sample are summarized from Cooper et al. (2016) and Tollan et al. (2012), supplemented by additional details on the characteristics of plagioclase, including SEM images showing the crystals selected for isotopic analysis.
How Representative are Selected Plagioclase?
Plagioclase crystals were chosen primarily for their suitability for micromilling, and care was taken to ensure that selected crystals were representative of each sample. All crystals analyzed from cumulates are characterized by a lack of zonation (excluding minor overgrowth rim zones too narrow to be targeted by micro-milling). Examples of typical unzoned cumulate plagioclase are shown in Figure 4. Where multiple plagioclase size populations were observed, e.g. MQ48, crystals from both populations were targeted. In NCG’s, about half of the plagioclase crystals display normal zoning (examples shown in Figure 4), which provided an opportunity to assess changes in isotopic composition during their crystallization. All crystals selected from these samples display normal zoning, with both cores and rims analyzed. The three plagioclase crystals selected from lava M8321 display the three different zoning patterns (described in Supplementary File S1). In this sample cores, rims, and bulk groundmass were analyzed.
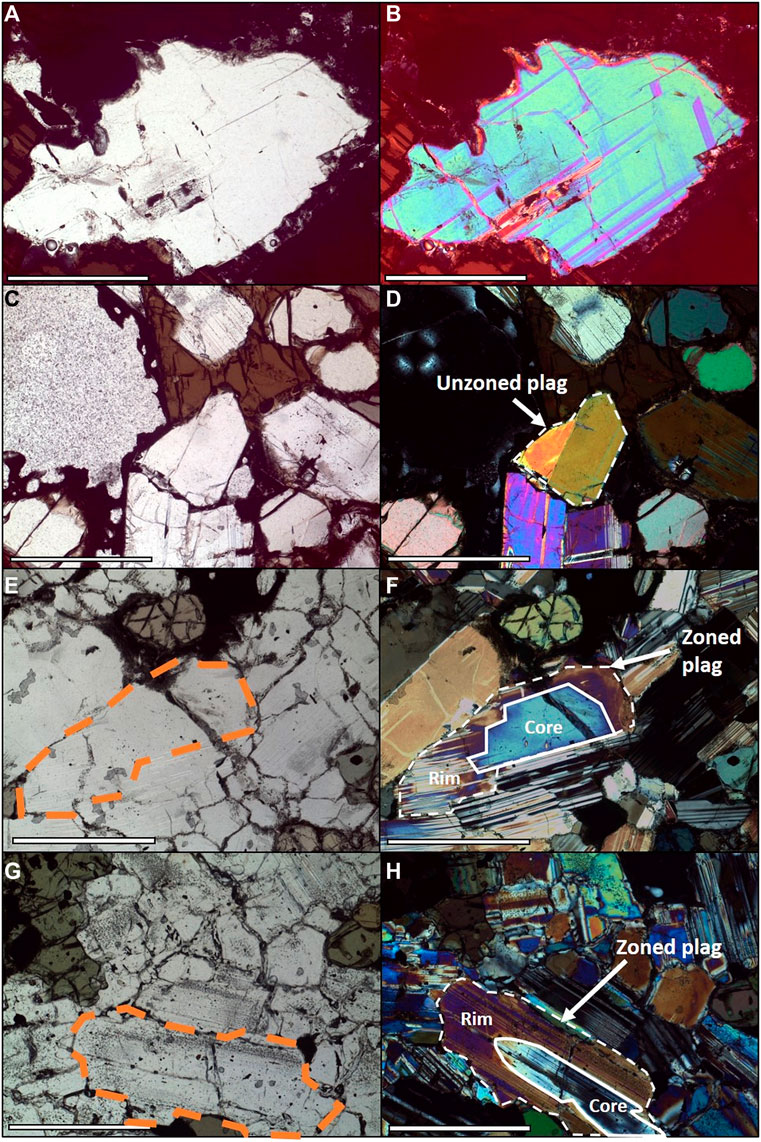
FIGURE 4. (A) and (B) Typical unzoned cumulate plagioclase from sample MQ59. (C) and (D) Typical unzoned cumulate plagioclase from sample MQ48. (E) and (F) Typical zoned plagioclase in NCG sample MQ35. (G) and (H) Typical zoned plagioclase in NCG sample MQ6. Orange outlines in (E) and (G) highlight labeled zoned plagioclase in (F) and (H). Images (A), (C), (E), and (G) in PPL, (B), (D,) (F), and (H) in XPL. White scale bar = 1 mm. Unusually high birefringence colors in XPL images are due to the 100 µm thickness of the sections.
Geochemical Results
Plagioclase Sr isotopic data is presented in Table 1 and plutonic xenolith whole rock Sr, Nd, and Pb isotopic data in Table 2. Rb/Sr ratios are very low (<0.01) in plutonic xenolith whole rock and plagioclase, and the maximum sample age is estimated at 25 Ma, hence age correction is negligible, with the exception of the M8321 groundmass data (see Supplementary File S2 for age correction details).
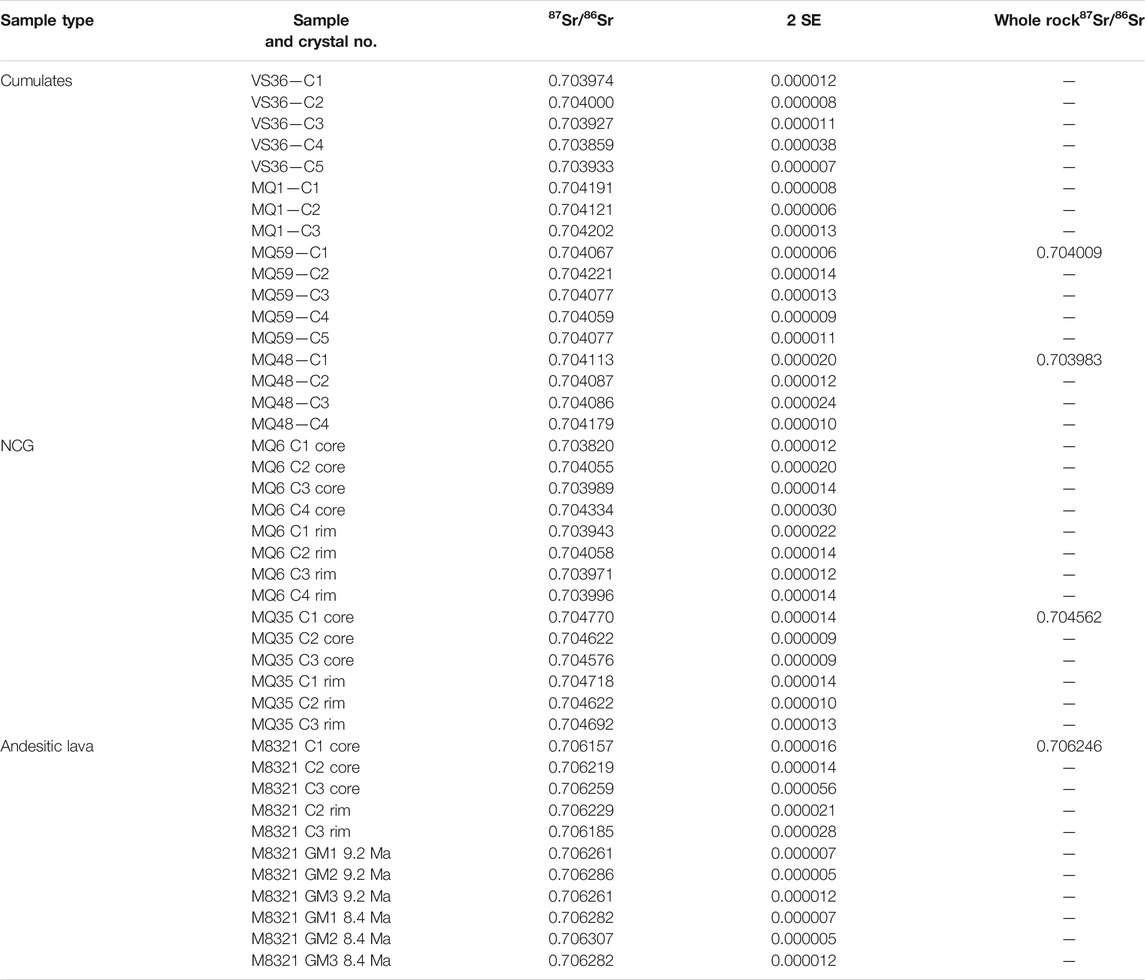
TABLE 1. 87Sr/86Sr of individual plagioclase in cumulates, and core and rim zones of plagioclase in NCG’s and lava. Whole rock87Sr/86Sr also shown if available. Lava whole rock value from Davidson, (1986). SE = standard error.
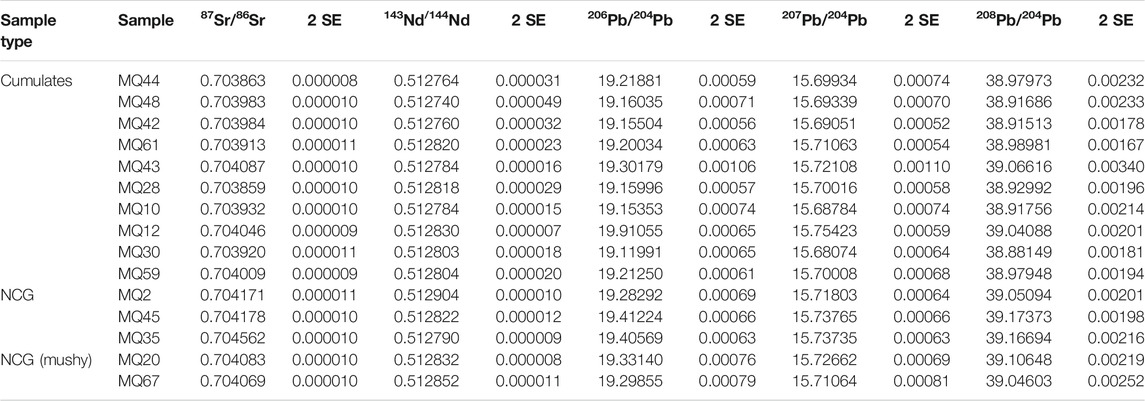
TABLE 2. Whole rock87Sr/86Sr, 143Nd/144Nd, 206Pb/204Pb, 207Pb/204Pb, and208Pb/204Pb of cumulates and NCG’s. SE = standard error.
Martinique Cumulate Plagioclase
Unzoned plagioclase from Martinique cumulates show a restricted range of unradiogenic Sr isotope ratios across all three samples (87Sr/86Sr = 0.704059–0.704221) (Figures 5, 6). Cumulate plagioclase 87Sr/86Sr slightly exceeds whole rock values (Figure 6).
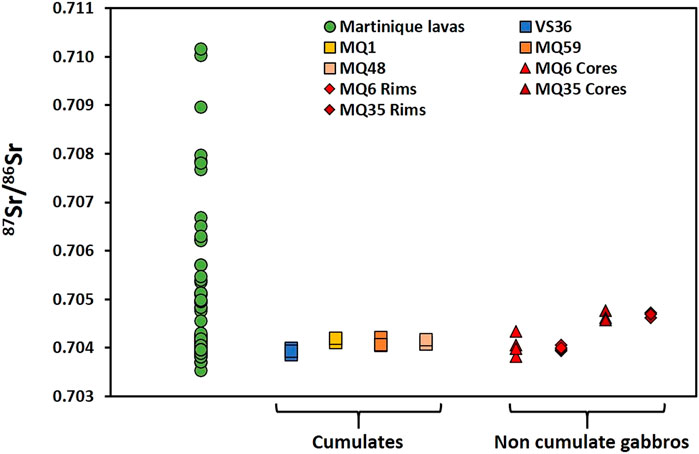
FIGURE 5. 87Sr/86Sr of individual plagioclase in cumulates, and core and rim zones of plagioclase in NCG’s, compared with the whole rock 87Sr/86Sr range of Martinique lavas. Lava data from Davidson (1986) and Labanieh et al. (2010). For all data points, uncertainty (2 SE) is smaller than symbol size.
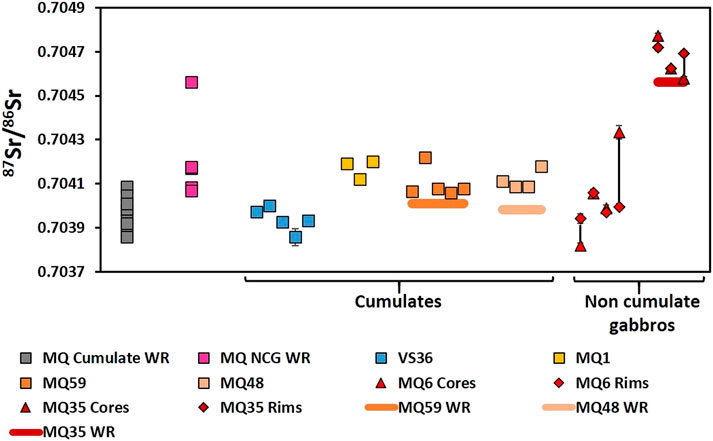
FIGURE 6. Details of 87Sr/86Sr variation in cumulate and NCG plagioclase. The whole rock 87Sr/86Sr range for Martinique cumulates and NCG’s is plotted for comparison. Whole rock data for individual samples shown as colored bars (bar width covers uncertainty). Where visible, black bars show uncertainty (2 SE), otherwise uncertainty is smaller than symbol size. Core-rim pairs are joined by tie lines.
Martinique Non-Cumulate Gabbro Plagioclase
Plagioclase cores from both NCG samples show greater intra-sample Sr isotopic variation than those of unzoned plagioclase in cumulates (Figures 5, 6). MQ6 cores show a wider range in 87Sr/86Sr (0.703820–0.704334) than MQ35 cores (0.704576–0.704770). Within samples, plagioclase rim compositions show a more limited range in 87Sr/86Sr than cores (MQ6 = 0.703943–0.704058, MQ35 = 0.704622–0.704718) (Figures 5, 6). No systematic variation in 87Sr/86Sr between core and rim is present in either sample. Notably, plagioclase in MQ35 is more radiogenic than that of cumulates. In MQ35 87Sr/86Sr in plagioclase is equivalent to or exceeds the whole rock value (Figure 6).
Martinique Andesitic Lava Plagioclase
Cores and rims in plagioclase from the andesitic lava (M8321) show a narrow range in 87Sr/86Sr (cores = 0.706157–0.706259, rims = 0.706185–0.706229), close to or within uncertainty of the whole rock value (Figure 7). Core-rim pairs have indistinguishable Sr isotopic compositions within uncertainty. Groundmass 87Sr/86Sr defines a very narrow range (0.706282–0.706307 corrected to 8.4 Ma, 0.706261–0.706286 corrected to 9.2 Ma).
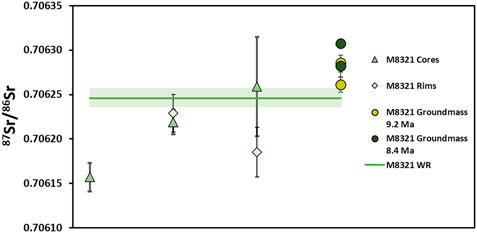
FIGURE 7. Details of 87Sr/86Sr variation in lava sample M8321. The whole rock value from Davidson (1986) is plotted for comparison. Black and gray bars (and green box around the WR value) show uncertainty (2 SE).
St Vincent Cumulate Plagioclase
Unzoned plagioclase crystals from a single St Vincent cumulate (VS36) have restricted and unradiogenic Sr isotope ratios (87Sr/86Sr = 0.703859–0.704000), with slightly less radiogenic compositions than Martinique cumulate plagioclase (Figure 6).
Martinique Plutonic Xenolith Whole Rock Data
Martinique cumulates show a narrow range in whole rock 87Sr/86Sr and unradiogenic compositions (87Sr/86Sr = 0.703859–0.704087, Figures 6, 8). NCG’s show a wider range in whole rock 87Sr/86Sr and extend to slightly more radiogenic compositions (87Sr/86Sr = 0.704069–0.704562, Figures 6, 8).
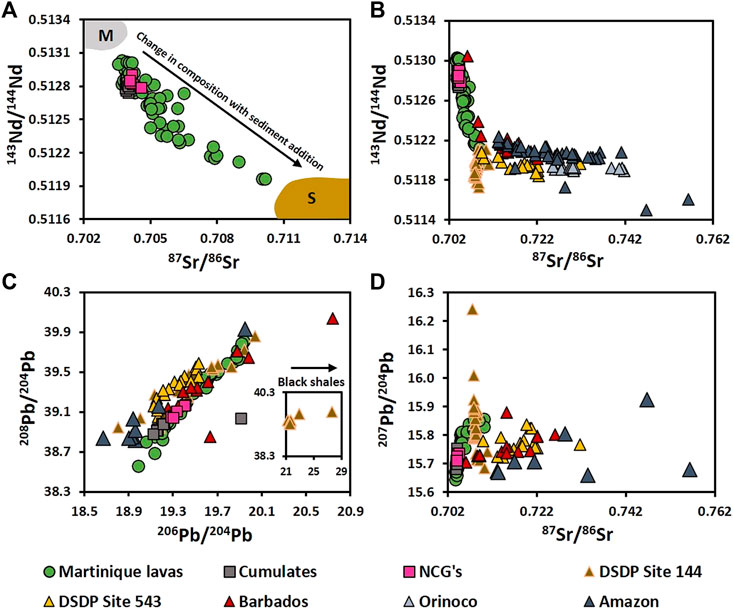
FIGURE 8. (A) Whole rock 87Sr/86Sr vs. 143Nd/144Nd for Martinique lavas and plutonic xenoliths (this study), with schematic fields for mantle (M) and sediment (S) end members. As shown, a suitable sediment end member is likely to have more radiogenic Sr and less radiogenic Nd than the lavas. (B), (C), and (D)87Sr/86Sr vs. 143Nd/144Nd, 208Pb/204Pb vs. 206Pb/204Pb, and 87Sr/86Sr vs. 207Pb/204Pb, respectively, for Martinique lavas and plutonic xenoliths (this study), potential equivalents to subducted sediments (Site 543, Site 144, Barbados) and sediments from the Orinoco and Amazon rivers. Inset in C) shows data for black shale unit of Site 144. For all data points, uncertainty (2 SE) is smaller than symbol size. Data sources: Martinique lavas—Davidson (1986), Labanieh et al. (2010). Site 543, Site 144 and Barbados—Carpentier et al. (2008); Carpentier et al. (2009). Amazon—Allegre et al. (1996); Parra et al. (1997); Viers et al. (2008); Rousseau et al. (2019). Orinoco—Parra et al. (1997); Rousseau et al. (2019).
Cumulates also show a restricted whole rock Nd isotopic range (143Nd/144Nd = 0.512740–0.512830) which extends to slightly more unradiogenic compositions than NCG’s (143Nd/144Nd = 0.512790–0.512904) (Figure 8). Cumulate whole rock Pb isotopic compositions vary from 206Pb/204Pb = 19.11991–19.30179, 207Pb/204Pb = 15.68074–15.72108, and 208Pb/204Pb = 38.88149–39.06616 (Figure 8), excluding sample MQ12, which represents a clear outlier with anomalously high 206Pb/204Pb (19.91055) and 207Pb/204Pb (15.75423). NCG’s show a slightly more radiogenic range in whole rock Pb isotopic compositions (Figure 8), with 206Pb/204Pb = 19.28292–19.41226, 207Pb/204Pb = 15.71064–15.73765, and 208Pb/204Pb = 39.04603–39.17373.
Discussion
The new Sr isotopic data from plutonic xenoliths is used to test whether the crustal/sedimentary component in Martinique lavas is introduced from the subducting slab or upper plate crust. We first discuss the chemical characteristics of potential sediment sources on the subducting plate and in the arc crust. We then explain how the data supports the crustal sediment assimilation hypothesis. Assimilation-Fractional Crystallization modeling is used to attempt to quantify the amount of sediment addition and provide constraints on the type of sediments assimilated. Finally, the wider implications of these findings for magma genesis and subduction zone recycling processes in the LAA and other arcs are discussed.
Potential Sediment Sources
If a sediment component is responsible for the range of isotopic compositions measured in Martinique lavas, then it must have more radiogenic Sr and Pb and less radiogenic Nd isotope compositions than the most extreme Martinique lava (Figure 8A). The lithological and chemical characteristics of the two main potential sediment sources—the slab and the arc crust—are discussed below.
Subducting Slab
Potential analogues to sediments subducting below the Lesser Antilles arc (LAA) come from Deep Sea Drilling Program (DSDP) Sites 543 and 144 in the Atlantic Ocean, and Barbados (Carpentier et al., 2008; Labanieh et al., 2010; Figure 1). These sediments are probably similar to those subducted based on their location and age relative to the age of oceanic crust at the trench (Carpentier et al., 2008). Sediments at DSDP Site 543 (<16–82 Ma) are mostly carbonate-free pelagic clays, in contrast to DSDP Site 144 sediments (29–104 Ma) which are mostly calcareous and include a distinctive black shale unit (Carpentier et al., 2008). Sediments from both sites contain a component of South American continental material delivered by the Amazon and Orinoco rivers (Figure 1; Carpentier et al., 2008). The variation in radiogenic isotopic composition of Site 543 and Site 144 sediments with depth is presented and compared with the isotopic range of Martinique lavas in Figure 9. Both Site 543 and Site 144 contain sediments with sufficiently radiogenic Sr and unradiogenic Nd isotopic compositions to represent a viable end member for Martinique lavas, while Site 144 sediments also have adequately radiogenic Pb isotopic compositions.
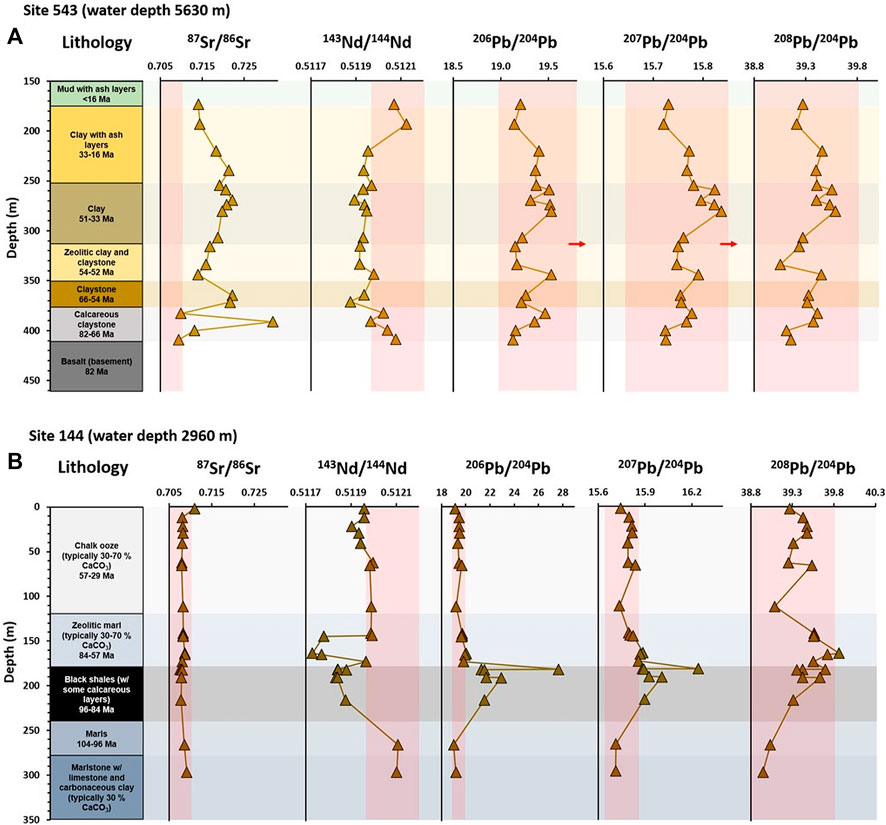
FIGURE 9. (A): Radiogenic isotopic compositions of DSDP Site 543 sediments, modified from Carpentier et al. (2008). (B): Radiogenic isotopic compositions of DSDP Site 144 sediments, modified from Carpentier et al. (2008). Pink bar indicates isotopic range of Martinique lavas (data sources as per Figure 8). For all data points, uncertainty (2 SE) is smaller than symbol size. Red arrows in (A) indicate that range of lavas exceeds the scale shown on the plot.
Sediments from Barbados include carbonate poor terrigenous claystones and sandstones, marls and chalks, with ages between 57 and 15 Ma (Carpentier et al., 2008). They are mixtures of detrital material from South America and volcanigenic material from the LAA and surrounding arcs (Carpentier et al., 2008; Carpentier et al., 2009). The radiogenic isotopic compositions of Barbados sediments are compared with Martinique lavas in Figure 8. Carpentier et al. (2008) noted that a mixture of the most radiogenic Barbados and Site 543 compositions represents a suitable sediment end member for Martinique (and Lesser Antilles) lavas.
Arc Crust
Recent tectonic models based on seismic and magnetic data show that the arc crust below the southern LAA (Martinique to Grenada) consists of oceanic crust and sedimentary cover of the formerly contiguous Grenada and Tobago basins (Figure 3) (Aitken et al., 2011; Allen et al., 2019). Fragments of dismembered Jurassic-Palaeocene age oceanic island arc terranes may also be present in the LAA basement (Neill et al., 2013). Tectonic reconstructions suggest that the region currently occupied by the southern LAA has been a site of sediment deposition since the Eocene (Escalona and Mann, 2011), supporting the idea that the arc, active since 25 Ma (Germa et al., 2011), was built through Grenada-Tobago basin sediments. These sediments comprise the upper few km of crust, thickening away from the centers of arc magmatism, reaching total thicknesses of 13–14 km (Aitken et al., 2011).
To the best of our knowledge, sediment samples from the Grenada and Tobago basins are not available, hence the type and chemical characteristics of the sediments cannot be directly constrained. However, Aitken et al. (2011) used seismic data to identify sedimentary lithologies in the two basins via correlation with well logs in the Carupano Basin (Figure 1), to the south, and uplifted sediments on the Grenadine Islands considered equivalent to deep Grenada basin sediments (Speed et al., 1993). Lithologies include turbidites, pelagic siltstones and marls, limestones, and shales (Aitken et al., 2011). Similar lithologies, along with a greater shale component and minor sandstone, are present in the Carupano Basin (Ysaccis, 1997). These studies suggest that sediments in the southern LAA crust are likely to be highly heterogeneous.
Data from modern sediments derived from the same sources as Grenada-Tobago basin sediments can provide constraints on the isotopic composition of sediments in LAA crust. From the Eocene to mid-Miocene (49–11.2 Ma), Grenada-Tobago basin sediments were sourced from the South American continent (Aitken et al., 2011). Sediment derived from uplifted Andean terranes and the Guyana shield was supplied by the proto-Maracaibo and Orinoco rivers (Xie et al., 2010; Escalona and Mann, 2011; Rojas-Agramonte et al., 2017). The present-day Orinoco and Amazon rivers also drain the Andes and Guyana shield (Rojas-Agramonte et al., 2017; Rousseau et al., 2019), hence the isotopic composition of sediments from these rivers is likely to be analogous to the sediments in the LAA crust.
The Amazon and Orinoco rivers drain old cratonic material with suspended sediment and clay typically having highly radiogenic 87Sr/86Sr (0.7132–0.7564) and unradiogenic 143Nd/144Nd (0.5122–0.5115) (Allègre et al., 1996; Parra et al., 1997; Viers et al., 2008; Rousseau et al., 2019) (Figure 8B). The limited Pb isotope data are typically less radiogenic than most Martinique lavas, though highly radiogenic samples (206Pb/204Pb = 19.95, 207Pb/204Pb = 15.924, 208Pb/204Pb = 39.93) have been reported (Allègre et al., 1996) (Figures 8C,D). Based on these data, it is plausible that sediments within LAA crust have the highly radiogenic Sr and Pb, and unradiogenic Nd isotopic compositions required to produce the isotopic variation in Martinique lavas. Since sediments at Sites 543 and 144 also include material from the Amazon and Orinoco (Carpentier et al., 2008), sediments of similar lithologies and isotopic compositions to those of the fore-arc are expected in the arc crust. Overall, the wide range of isotopic compositions in potential equivalents to sediments in the arc crust combined with a range of potential lithologies suggests that the arc crust is chemically and isotopically heterogenous. Both recycled subducted and unsubducted crustal sediments provide viable isotopic components in Martinique lavas specifically and lavas of the Lesser Antilles more generally. Thus, chemical characteristics of lavas alone cannot be used to distinguish whether the sediment component is incorporated by recycling into the mantle source or crustal assimilation of sediments.
Cumulate Isotopic Compositions Support Crustal Assimilation of Sediments
Plutonic xenoliths from Martinique are considered to represent portions of an open crystal mush system (Cooper et al., 2016), which consists predominantly of cumulates in the mid-crust and NCG’s in the upper crust. If the Sr isotopic variation observed in Martinique lavas is produced by varying sediment input to the mantle source (e.g., Carpentier et al., 2008; Labanieh et al., 2010), then a similar isotopic range would also be expected in mid-crustal magmas. To a first order, the unradiogenic and extremely restricted Sr isotopic range determined for individual cumulate plagioclase crystals (Figure 6) indicates that mid-crustal magmas beneath Martinique have not yet been modified significantly by sediment addition. The same logic can be applied to the wider cumulate whole rock 87Sr/86Sr data. The plagioclase and whole rock data support the hypothesis that isotopic heterogeneity and extreme radiogenic compositions of Martinique lavas are produced by assimilation of sediments at shallower crustal levels.
For comparison, plagioclase from a St Vincent cumulate also show unradiogenic Sr isotopic compositions close to those of Martinique cumulates (Figures 5, 6). Tollan et al. (2012), used oxygen isotopes to show that St Vincent cumulates experienced negligible crustal contamination, suggesting that their isotopic composition should reflect the mantle source. St Vincent cumulate plagioclase have slightly lower 87Sr/86Sr than those from Martinique, suggesting that the two islands are fed by mantle sources with similar, but not identical, compositions. This conclusion was also reached by Davidson and Wilson (2011) and Bezard et al. (2015) in studies of primitive Lesser Antilles lavas. Unlike Martinique, lavas on St Vincent do not extend to highly radiogenic isotopic compositions (Figure 3) and the impact of crustal assimilation is considered minimal (Heath et al., 1998). Since there are only subtle differences in the isotopic compositions inferred for the mantle sources of the two islands, the wider isotopic range of Martinique lavas is better explained by assimilation of crustal sediments.
Inferences About Assimilation From Isotopic Heterogeneity of Shallow Non-Cumulate Gabbros and Lavas
Non-cumulate gabbros are considered plutonic equivalents of potentially eruptible lavas that crystallized within the 27 km thick Martinique crust at shallower levels than the cumulates (Cooper et al., 2016). Evidence for upper crustal crystallization comes from comparing natural mineral assemblages and compositions to those from experimental studies. These comparisons suggest crystallization of NCGs between 2 and 4 kbar (Martel et al., 1999; Cooper et al., 2016; Melekhova et al., 2019), corresponding to depths of approximately 6–12 km (vs. ∼12 km or greater for cumulates).
If the isotopic composition of a magma is inherited from its mantle source and subsequent evolution of that magma remains a closed system, crystals within the same rock should show minimal radiogenic isotopic variation. In contrast, intra-sample isotopic variation among crystals is characteristic of an open system, involving, for example, crustal assimilation (e.g., Baker et al., 2000). The isotopic diversity recorded by NCG plagioclase cores (particularly MQ6, Figure 6) may therefore indicate sediment assimilation during crystallization. In NCG sample MQ35, the presence of both cores and rims with significantly more radiogenic 87Sr/86Sr than cumulates again suggests that magmas can acquire more radiogenic compositions during crystal growth at shallow crustal levels.
In both NCG samples, more evolved plagioclase rims show less isotopic variation than cores and have Sr isotopic compositions within the range defined by the associated cores. We propose the following processes to explain this trend. Emplacement of new magma into the upper crust, in contact with crustal sediments, leads to sediment assimilation and crystallization of plagioclase cores. Assimilation of variable amounts of sediment, or sediments with different isotopic compositions, could produce an isotopically heterogenous magma resulting in crystallization of isotopically heterogenous plagioclase. Alternatively, isotopic variation among plagioclase cores may reflect crystallization at different times during progressive contamination of the newly emplaced magma.
Plagioclase rims may have crystallized from a more evolved, more isotopically homogenous melt that interacted with the pre-existing plagioclase cores. Other Martinique plutonic xenoliths contain evidence for percolating evolved melts (Cooper et al., 2016) and plutonic xenoliths from Statia in the northern LAA contain melt inclusions ranging in composition from basalt to rhyolite (Cooper et al., 2019). These lines of evidence suggest that it is plausible for plagioclase formed during an initial phase of crystallization (accompanied by assimilation) to interact with more evolved melts in the Martinique magma plumbing system. The fact that NCG plagioclase rims have Sr isotopic compositions within the range defined by the cores from the same sample suggests that in this scenario, the rim-forming melts were contaminated with crust to a similar extent to the core forming melts, hence were likely sourced from nearby regions of the crystal mush in the upper crust.
The most radiogenic Sr isotopic signatures in the LAA are observed in Martinique lavas. Sample M8321 represents one of the more radiogenic lavas, with a whole rock isotopic composition of 87Sr/86Sr = 0.706246. Plagioclase crystals from M8321 have 87Sr/86Sr close to the whole rock value (Figure 7) and are significantly more radiogenic than NCG plagioclase (Figure 6). This suggests that the magma from which they are derived had assimilated more sediment, or a more radiogenic sediment, than the magmas that crystallized the NCGs. M8321 plagioclase crystals show either normal, reverse, or both normal and reverse zoning, a feature commonly observed in Martinique lavas and interpreted as evidence for mixing shortly before eruption (Coulon et al., 1984; Bourdier et al., 1985; Gorgaud et al., 1989). The indistinguishable Sr isotopic composition of analyzed core-rim pairs (Figure 7) suggests that all components involved in this mixing probably had similar Sr isotopic compositions. Plagioclase crystals show only very minor isotopic disequilibrium with the bulk groundmass and have isotopic compositions close to or within uncertainty of the whole rock value, consistent with an origin as true phenocrysts grown in the final erupted melt (or mixed melts).
If the minimal isotopic variation between plagioclase cores, rims and groundmass in M8321 was considered in isolation, then it could be argued that the radiogenic isotopic composition of this lava was inherited from the mantle source. However, by combining this observation with the isotopic data from plutonic xenoliths, we argue that shallow level assimilation is the dominant process controlling isotopic variation in Martinique lavas. Lava M8321 is quite evolved (approx. 58 wt% SiO2) and increasing 87Sr/86Sr generally correlates with increasing SiO2 in Martinique lavas (Davidson, 1986; Davidson and Wilson, 2011). As discussed by Bezard et al. (2014), this would require a fortuitous process linking the extent of source contamination and magmatic differentiation for isotopic variation to be produced by subducted sediment addition alone.
Quantifying the Addition of Sedimentary Components
Previous estimates of the amount of sediment addition required to generate the radiogenic isotopic compositions of Martinique and Lesser Antilles lavas are based on Sr, Nd, Pb and oxygen isotope data and calculated using binary mixing models. These studies estimate that bulk addition of up to 20–40% sediment in either the mantle source (Carpentier et al., 2008; Labanieh et al., 2010) or within the crust (Davidson and Harmon, 1989; Bezard et al., 2014) explains the most extreme Lesser Antilles lava compositions. Here we use Assimilation-Fractional Crystallization (AFC) modeling to assess whether crustal sediment assimilation can produce the Sr-Nd-Pb isotopic variation in Martinique lavas and estimate the amount of sediment assimilation required.
Assimilation-Fractional Crystallization Modeling End Members and Parameters
Mid-crustal cumulates from Martinique crystallize from magmas that have undergone prior olivine fractionation at depth (Cooper et al., 2016). This suggests that parental magmas to NCGs and lavas, ascending to the upper crust from the mid-crust or deeper, are likely to have higher Sr concentrations than primary mantle melts, because Sr is incompatible in olivine. Therefore, we model sediment addition to a parental magma rather than a primary mantle melt composition. The Ile a Ramiers basalt, the least evolved lava erupted in Martinique (∼12% MgO, Labanieh et al., 2010), is a reasonable approximation for parental magmas ascending to the upper crust. Its Sr isotopic composition of 87Sr/86Sr ∼ 0.7038 is consistent with the isotopic composition of Martinique primitive magmas calculated by Davidson and Wilson (2011), and is close to the least radiogenic value measured in this study (NCG sample MQ6). Hence the composition of the Ile a Ramiers basalt is used here to represent magmas prior to upper crustal sediment assimilation, hereon referred to as “parental magmas.”
End member compositions used in AFC models are given in Table 3. In Arc Crust, we argue that LAA crustal sediments are likely to be both lithologically and chemically heterogenous, based on the best available constraints. This heterogeneity is emphasized by the wide range of potential crustal sediment compositions shown in Figure 8. To represent a lithologically and chemically heterogenous crust, eight different potential sediment end members were considered. These include the average Sr-Nd-Pb isotopic compositions of sediments from DSDP Sites 543 and 144, and from Barbados. We also include the 50:50 mixture of bulk Site 543 and bulk Site 144 sediments that Bezard et al. (2015) suggested were involved in St Lucia magmatism. Potential highly radiogenic crustal end members are represented by the average composition of sediments from the Orinoco (from Parra et al., 1997; Rousseau et al., 2019) and the most extreme composition from Barbados (Carpentier et al., 2008; Carpentier et al., 2009). Amazon sediments show a wide range of very radiogenic compositions (e.g. 87Sr/86Sr = 0.7132–0.7564, Allègre et al., 1996). The minimum and maximum isotopic compositions were used as additional potential crustal end members, with concentrations equal to the average of Amazon sediments [Sr and Pb from Allègre et al. (1996); Nd from Rousseau et al. (2019)]. The end member compositions in Table 3 are plotted relative to Martinique lavas and the isotopic range of sediments potentially equivalent to those in the arc crust in Figure 10.
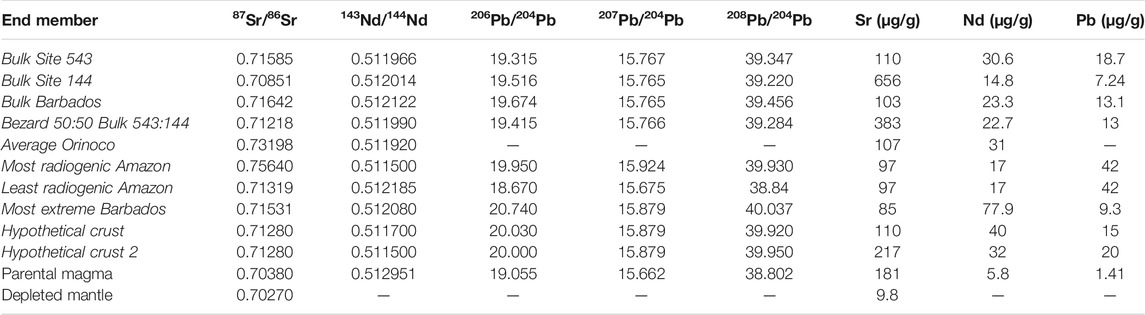
TABLE 3. Sr-Nd-Pb isotopic compositions and concentrations of end members used in AFC modeling. Data sources: Bulk Site 543, Bulk Site 144, Bulk Barbados, Most extreme Barbados—Carpentier et al. (2008); Carpentier et al, (2009). Bezard 50:50 Bulk 543:144—Bezard et al. (2015). Average Orinoco—average of samples from Parra et al. (1997) and Rousseau et al. (2019) (n = 15). Most and least radiogenic Amazon—Allegre et al. (1996), Rousseau et al. (2019). Primitive magma—Ile a Ramiers high Mg basalt from Labanieh et al. (2010), (2012). Depleted mantle—Salters and Stracke, (2004). End members in italics represent sediment/crustal end members.
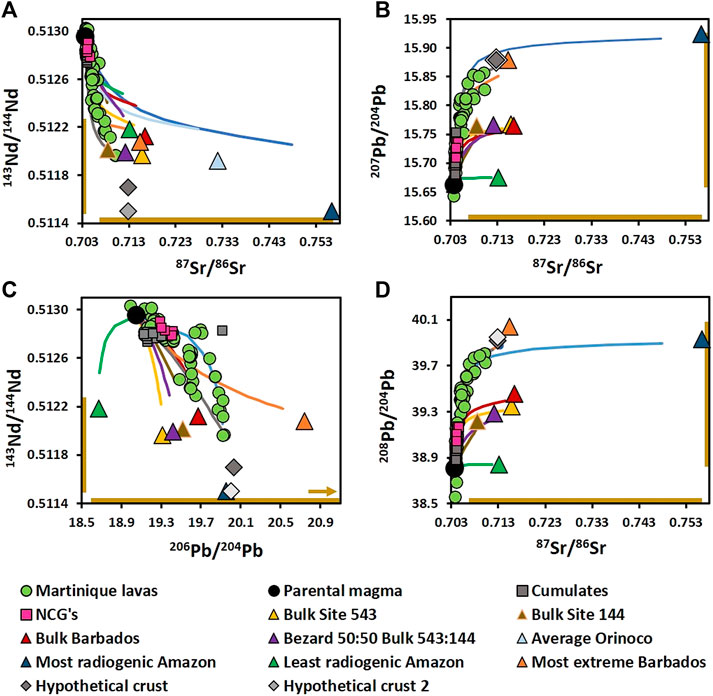
FIGURE 10. (A)87Sr/86Sr vs. 143Nd/144Nd, (B)87Sr/86Sr vs. 207Pb/204Pb, (C)206Pb/204Pb vs. 143Nd/144Nd and (D)87Sr/86Sr vs. 208Pb/204Pb, for Martinique lavas, plutonic xenoliths (this study) and end members used in AFC modeling from Table 3. Data sources as per Table 3 and Figure 8. For all data points, uncertainty (2 SE) is smaller than symbol size. Brown bars along axes show range of isotopic compositions of sediments potentially equivalent to those in the arc crust from Figure 8. Colored curves correspond to AFC models for each crustal end member, shown in detail in Figure 11.
The fractionating assemblage used in the AFC models was that of NCG sample MQ6 (Supplementary File S1), which closely resembles the phenocryst assemblage of the lavas (e.g. Coulon et al., 1984; Davidson and Wilson, 2011). Bulk partition coefficients were calculated for this modal mineralogy using the mineral-melt partition coefficients listed in Supplementary File S3.
Modeling and Quantifying Crustal Assimilation
Results of AFC models, calculated using the equations of De Paolo (1981), are plotted in Figures 10, 11, with details of the calculations given in Supplementary File S3. The value of r (rate of assimilation/rate of crystal fractionation) was set at 0.3, similar to previous AFC models for LAA lavas (e.g., Smith et al., 1996; Labanieh et al., 2010). An additional sediment end member (“Hypothetical crust” in Table 3) was also modeled in order to reproduce the most extreme (most radiogenic Sr and Pb, most unradiogenic Nd) lava compositions. This end member (plotted on Figure 10) lies within the range of potential arc crust sediment isotopic compositions identified in Arc Crust (Figures 8, 10) and hence represents a reasonable approximation for an assimilant. Combined, the AFC models for the various crustal end members cover most of the observed isotopic variation in Martinique lavas (Figure 11). This suggests that Martinique lava isotopic compositions can be explained by assimilation of crustal sediments with a range of isotopic and chemical compositions, with individual lavas having assimilated different crustal sediments.
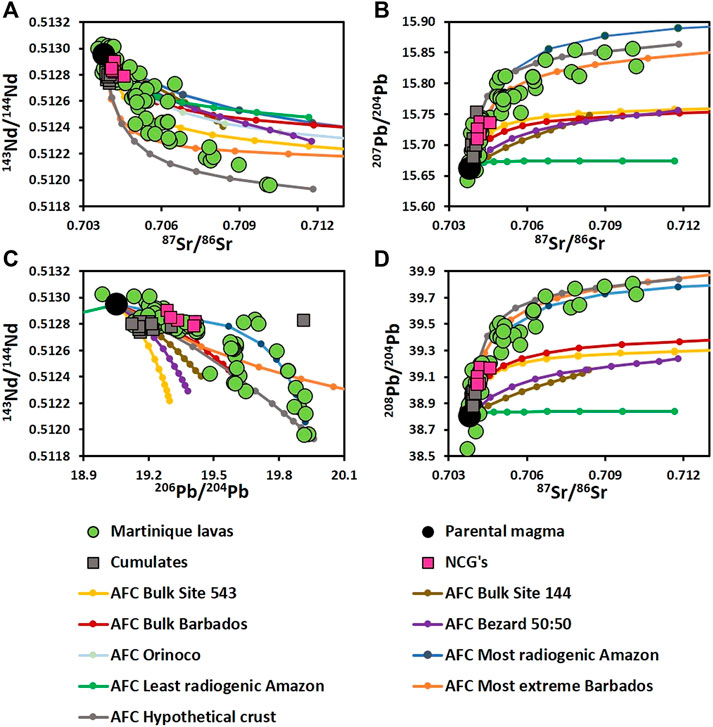
FIGURE 11. (A)87Sr/86Sr vs. 143Nd/144Nd, (B)87Sr/86Sr vs. 207Pb/204Pb, (C)206Pb/204Pb vs. 143Nd/144Nd and (D)87Sr/86Sr vs. 208Pb/204Pb, for Martinique lavas and plutonic xenoliths (this study). Data sources as per Table 3 and Figure 8. For all data points, uncertainty (2 SE) is smaller than symbol size. Each colored curve corresponds to an AFC model using a different crustal end member from Table 3 as the assimilant composition (see legend). Each point along the curves represents an increment of 10% fractional crystallization, up to a maximum of 90%. Details of AFC model calculations are given in Supplementary File S3.
The sediment end members considered represent only a few possible assimilants, which theoretically could lie anywhere within the range of sediment compositions outlined in Figures 8, 10. Since the LAA crust has yet to be directly sampled, its actual isotopic composition remains to be determined but it is possible that sediments with more extreme isotopic compositions than those considered here could also exist within the arc crust.
To quantify the amount of crustal assimilation required to produce the isotopic compositions of Martinique lavas, we employed the method of Aitcheson and Forrest (1994). This method solves the AFC equations of De Paolo (1981) to determine the crust/magma ratio ρ (mass of crust assimilated/mass of original magma), which can be converted into a proportion of crust assimilated. If the isotopic and elemental compositions of the original magma and assimilant are defined, then the crust/magma ratio can be calculated for a lava of a given isotopic composition. We calculated the crust/magma ratio for the Martinique lava with the most extreme isotopic composition to determine the maximum amount of crustal sediment assimilated by Martinique lavas (details of the calculation given in Supplementary File S3). Assimilation of 28% sediment with the composition of the “Hypothetical crust” end member is required to produce the most extreme Martinique lava composition. To illustrate the effect of a chemically and isotopically heterogenous crust, we generated an alternative sediment end member (“Hypothetical crust two” in Table 3) which also reproduces the isotopic composition of the most extreme Martinique lava with only 23% assimilation (Supplementary File S3). This end member also has isotopic compositions within the potential range for crustal sediments shown on Figures 8, 10. These results show that the amount of crustal assimilation required varies depending on the composition of crust being assimilated.
Previous estimates of the amount of sediment addition (whether to the mantle source or via crustal assimilation) required to produce the most extreme Martinique lava composition are similar to our calculated values of 23–28%. Given the wide range of potential assimilant compositions, the proportion of crustal sediment assimilated by Martinique lavas cannot be accurately quantified. However, 28% should be treated as a maximum amount, with most lavas likely experiencing significantly less assimilation. Our new AFC modeling, combined with the constraints from plutonic xenoliths on where assimilation takes place, clearly demonstrates that isotopic variation in Martinique lavas is best explained by assimilation of isotopically and chemically heterogenous sediments in the upper crust.
Sediment Addition to the Mantle Source?
Although the evidence presented supports crustal assimilation at shallow crustal levels as the dominant process responsible for the large isotopic range and radiogenic compositions of Martinique lavas, this does not preclude the involvement of subducted sediment in generating the compositions of the parental magmas and cumulates, which are more radiogenic in terms of Sr isotopes than typical depleted mantle. As such, the amount of subducted sediment addition to the mantle source required to produce parental magma and cumulate plagioclase Sr isotopic compositions was calculated. A binary mixing approach was used for simplicity. The average depleted mantle composition of Salters and Stracke (2004) was used as the mantle end member. Potential subducted sediment end members were represented by average compositions of DSDP Site 543, DSDP Site 144 and Barbados sediments (Table 3).
The Sr isotopic composition of Martinique parental magmas (87Sr/86Sr = 0.7038) allows a maximum of ∼0.8% bulk sediment addition to the mantle source (Figure 12). This value agrees well with the <1% subducted sediment contribution to parental magmas at Mt Pelee, Martinique estimated by Davidson and Wilson (2011). Cumulate plagioclase compositions allow a maximum of 1–1.2% bulk sediment addition to the mantle source (Figure 12). The slightly higher 87Sr/86Sr of the cumulates relative to parental magma could be explained by minor variations in sediment input to the mantle source. Alternatively, the parental and cumulate forming magmas may have been affected by minor assimilation, either of deep sediments or oceanic crustal material, which underlies Grenada-Tobago basin sediments (Aitken et al., 2011; Allen et al., 2019). Since the maximum sediment contribution to the parental magma is <1% (regardless of where sediment addition occurs), this composition can still be considered a reasonable proxy for Martinique magmas prior to upper crustal assimilation. It should be noted that the Nd and Pb isotopic compositions of the parental magma also require <1% sediment contribution (when modeled as above for Sr isotopic composition).
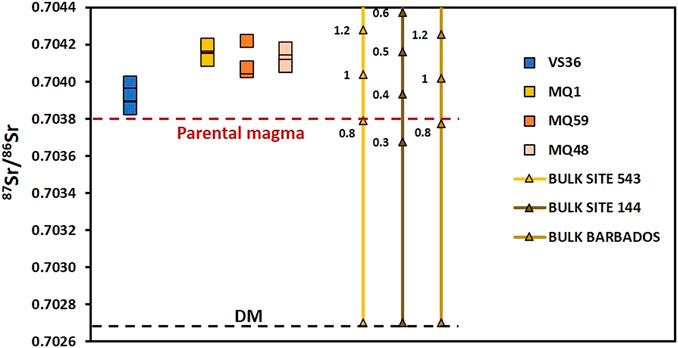
FIGURE 12. 87Sr/86Sr of cumulate plagioclase compared with single component mixing lines between depleted mantle and potential subducted sediment end members. The subducted sediment end member from Table 3 corresponding to each mixing line is shown in the legend. Labeled tickmarks represent percentage sediment addition to mantle source. Data sources as per Table 3. Dashed line labeled DM = depleted mantle [87Sr/86Sr = 0.70270, from Salters and Stracke (2004)]. Red dashed line shows Martinique parental magma composition (87Sr/86Sr = 0.70380). Where visible, black bars show uncertainty (2 SE), otherwise uncertainty is smaller than symbol size.
Wider Significance
Our study of Martinique lavas in conjunction with plutonic xenoliths places important spatial constraints on the location where the crustal/sedimentary isotopic signatures of Martinique lavas are introduced, which simply cannot be derived from studies of lavas alone. We have shown that the crustal/sedimentary signatures are almost entirely inherited from AFC processes taking place in the upper crust, with only a very minor role for subducted sediment in the mantle source. Application of the approach applied here may reveal a greater role for crustal assimilation than previously considered in other arc systems where lava geochemistry is currently interpreted to reflect source processes. If crustal contributions are underestimated, relative contributions from the subducting slab and mantle will be overestimated, which has major implications for quantifying element and sediment recycling at subduction zones. Improved constraints on crustal contributions could also be utilized to re-assess the composition and heterogeneity of arc mantle source regions, by correcting lava compositions for the effects of crustal assimilation.
The latest tectonic model for the evolution of the LAA (Allen et al., 2019) corroborates evidence for crustal sediment assimilation in Martinique. The model suggests that an outer arc was active east of the current arc from 40 to 25 Ma. A back-arc basin formed during this period, extending from the present-day location of Dominica south to Grenada. Around 22 Ma, the arc migrated west and the southern volcanic centers of the current Lesser Antilles arc (Martinique to Grenada) were subsequently built through the back-arc basin crust (oceanic crust and sediments), separating it into the Grenada and Tobago basins (Figure 3). Evidence for crustal contamination in Martinique (and other southern LAA islands) demonstrates that magma interacted with the thick sediment pile of the Grenada-Tobago basins. In other arc settings where the tectonic evolution is less well constrained, the methods employed in this study could be used to identify shallow crustal sediment assimilation and thereby indicate the presence of sediments within the arc crust. This information could be used to infer the past locations of sedimentary basins/depocenters, which could be incorporated into tectonic models for arc development and may also indicate potential locations of hydrocarbon bearing sediments.
In an alternative scenario, isotopic studies of plutonic xenoliths from other arcs may prove a less effective means of recognizing crustal assimilation than in the LAA. The proximity of the LAA to the South American continental margin has resulted in a component of the arc crust composed of sediments which, based on available constraints, can be assumed to have highly radiogenic isotopic compositions. The contrast in isotopic compositions between crustal sediments and primitive magmas makes sediment assimilation easily detected in the LAA and may be unique among arcs. Upper plate crustal sediments elsewhere may not have such highly radiogenic or diverse compositions resulting in a less obvious geochemical signature of assimilation.
Recent studies have demonstrated that volatile fluxes from arc volcanoes (e.g. He and CO2) contain contributions from crustal sediments (e.g. Troll et al., 2012; Yu et al., 2016; Aiuppa et al., 2017; Mason et al., 2017). Therefore, it is likely that volatile contents and compositions of magmas in volcanic arcs are modified by crustal sediment assimilation. This highlights the importance of considering and accurately quantifying volatile contributions from upper plate crust in models of volatile recycling at subduction zones. If volatiles added in the crust are mistakenly attributed to the subducted component, the contribution from the downgoing slab to arc volatile output could be significantly overestimated, resulting in misleadingly elevated volatile recycling efficiency estimates. Finally, addition of volatiles to magmas at shallow crustal levels may influence the typically explosive eruptive behavior of LAA volcanoes, as has been suggested for other arc volcanoes e.g. Merapi (Deegan et al., 2010).
Conclusion
The Sr isotopic composition of plagioclase from plutonic xenoliths has been used to evaluate the origin of the wide isotopic range and highly radiogenic compositions of Martinique lavas. Through this approach it has been possible to distinguish between competing hypotheses where the compositions of the lavas result from variations in subducted sediment addition to the mantle source vs. assimilation of unsubducted, shallow, crustal sediments. Unzoned plagioclase crystals from mid-crustal cumulate xenoliths show restricted ranges of unradiogenic Sr isotope ratios. In contrast, cores of zoned plagioclase crystals from upper-crustal NCG xenoliths are characterized by wider intra-sample Sr isotopic ranges than cumulate plagioclase. Some NCG plagioclase crystals have both core and rim compositions with more radiogenic 87Sr/86Sr than mid-crustal cumulates. Whole rock Sr isotopic compositions follow the same pattern, with cumulates showing restricted, unradiogenic 87Sr/86Sr and NCG’s extending to slightly more radiogenic values. Combined, these results support the hypothesis that Martinique magmas acquire more radiogenic compositions and isotopic diversity via assimilation of in situ sediment in the upper crust. Consistent with the best available constraints for crustal sediment composition, AFC modeling demonstrates that the isotopic variation in Martinique lavas can be produced by assimilation of chemically and isotopically heterogenous crust. The novel approach used here could be applied to other arc systems to assess the relative roles of subducted sediment and crustal contributions which in turn would have significant implications for understanding arc mantle source compositions and quantifying sediment and volatile recycling at subduction zones.
Data Availability Statement
The original contributions presented in the study are included in the article/Supplementary Material, further inquiries can be directed to the corresponding author.
Author Contributions
JP, GC, CM, and IN devised the project. JB and GC performed petrographic analyses, JB performed SEM analyses. JB, GC and GN carried out sample preparation and isotopic analyses. All authors contributed to interpretation of the data and writing of the manuscript.
Funding
This research was funded by a NERC IAPETUS DTP PhD scholarship to JB and the NERC VoiLA project (Grant Number NE/K010824/1 from the Natural Environment Research Council) (www.voila.ac.uk).
Conflict of Interest
The authors declare that the research was conducted in the absence of any commercial or financial relationships that could be construed as a potential conflict of interest.
Acknowledgments
The authors would like to thank all members of the NERC VoiLA consortium and project partners for discussions during the course of this project both in the United Kingdom and during the 2019 consortium workshop in the Lesser Antilles. E. Melekhova is thanked in particular for initial discussions of the existing plutonic xenolith suite. B. Rogers is thanked for her contribution to testing and refining low concentration TIMS Sr isotopic analyses. Ian Chaplin and Sophie Edwards are thanked for thin section preparation. We are grateful to Leon Bowen for his support with SEM analyses.
Supplementary Material
The Supplementary Material for this article can be found online at: https://www.frontiersin.org/articles/10.3389/feart.2021.682583/full#supplementary-material
References
Aitcheson, S. J., and Forrest, A. H. (1994). Quantification of Crustal Contamination in Open Magmatic Systems. J. Petrol. 35 (2), 461–488. doi:10.1093/petrology/35.2.461
Aitken, T., Mann, P., Escalona, A., and Christeson, G. L. (2011). Evolution of the Grenada and Tobago Basins and Implications for Arc Migration. Mar. Pet. Geology. 28 (1), 235–258. doi:10.1016/j.marpetgeo.2009.10.003
Aiuppa, A., Fischer, T. P., Plank, T., Robidoux, P., and Di Napoli, R. (2017). Along-arc, Inter-arc and Arc-To-Arc Variations in Volcanic Gas CO 2/S T Ratios Reveal Dual Source of Carbon in Arc Volcanism. Earth-Science Rev. 168, 24–47. doi:10.1016/j.earscirev.2017.03.005
Allègre, C. J., Dupré, B., Négrel, P., and Gaillardet, J. (1996). Sr-Nd-Pb Isotope Systematics in Amazon and Congo River Systems: Constraints about Erosion Processes. Chem. Geology. 131 (1-4), 93–112. doi:10.1016/0009-2541(96)00028-9
Allen, R. W., Collier, J. S., Stewart, A. G., Henstock, T., Goes, S., and Rietbrock, A.VoiLA Team (2019). The Role of Arc Migration in the Development of the Lesser Antilles: A New Tectonic Model for the Cenozoic Evolution of the Eastern Caribbean. Geology 47 (9), 891–895. doi:10.1130/G46708.1
Annen, C., Blundy, J. D., and Sparks, R. S. J. (2006). The Genesis of Intermediate and Silicic Magmas in Deep Crustal Hot Zones. J. Petrol. 47 (3), 505–539. doi:10.1093/petrology/egi084
Arculus, R. J., and Wills, K. J. A. (1980). The Petrology of Plutonic Blocks and Inclusions from the Lesser Antilles Island Arc. J. Petrol. 21 (4), 743–799. doi:10.1093/petrology/21.4.743
Baker, J. A., Macpherson, C. G., Menzies, M. A., Thirlwall, M. F., Al-Kadasi, M., and Mattey, D. P. (2000). Resolving Crustal and Mantle Contributions to continental Flood Volcanism, Yemen; Constraints from mineral Oxygen Isotope Data. J. Petrol. 41 (12), 1805–1820. doi:10.1093/petrology/41.12.1805
Bebout, G. E. (2014). “Chemical and Isotopic Cycling in Subduction Zones,” in Treatise on Geochemistry. Second Edition. Elsevier, 703–747. doi:10.1016/B978-0-08-095975-7.00322-3
Bezard, R., Davidson, J. P., Turner, S., Macpherson, C. G., Lindsay, J. M., and Boyce, A. J. (2014). Assimilation of Sediments Embedded in the Oceanic Arc Crust: Myth or Reality?. Earth Planet. Sci. Lett. 395, 51–60. doi:10.1016/j.epsl.2014.03.038
Bezard, R., Turner, S., Davidson, J. P., Macpherson, C. G., and Lindsay, J. M. (2015). Seeing through the Effects of Crustal Assimilation to Assess the Source Composition beneath the Southern Lesser Antilles Arc. J. Petrol. 56 (4), 815–844. doi:10.1093/petrology/egv018
Bourdier, J. L., Gourgaud, A., and Vincent, P. M. (1985). Magma Mixing in a Main Stage of Formation of Montagne Pelée: the Saint Vincent-type Scoria Flow Sequence (Martinique, FWI). J. volcanology geothermal Res. 25 (3-4), 309–332. doi:10.1016/0377-0273(85)90019-8
Carpentier, M., Chauvel, C., and Mattielli, N. (2008). Pb-Nd Isotopic Constraints on Sedimentary Input into the Lesser Antilles Arc System. Earth Planet. Sci. Lett. 272 (1–2), 199–211. doi:10.1016/j.epsl.2008.04.036
Carpentier, M., Chauvel, C., Maury, R. C., and Mattielli, N. (2009). The “Zircon Effect” as Recorded by the Chemical and Hf Isotopic Compositions of Lesser Antilles Forearc Sediments. Earth Planet. Sci. Lett. 287 (1–2), 86–99. doi:10.1016/j.epsl.2009.07.043
Chadwick, J. P., Troll, V. R., Ginibre, C., Morgan, D., Gertisser, R., Waight, T. E., et al. (2007). Carbonate Assimilation at Merapi Volcano, Java, Indonesia: Insights from crystal Isotope Stratigraphy. J. Petrol. 48 (9), 1793–1812. doi:10.1093/petrology/egm038
Charlier, B. L. A., Ginibre, C., Morgan, D., Nowell, G. M., Pearson, D. G., Davidson, J. P., et al. (2006). Methods for the Microsampling and High-Precision Analysis of Strontium and Rubidium Isotopes at Single crystal Scale for Petrological and Geochronological Applications. Chem. Geology. 232 (3-4), 114–133. doi:10.1016/j.chemgeo.2006.02.015
Cooper, G. F., Blundy, J. D., Macpherson, C. G., Humphreys, M. C. S., and Davidson, J. P. (2019). Evidence from Plutonic Xenoliths for Magma Differentiation, Mixing and Storage in a Volatile-Rich crystal Mush beneath St. Eustatius, Lesser Antilles. Contrib. Mineralogy Petrol. 174 (5). doi:10.1007/Fs00410-019-1576-410.1007/s00410-019-1576-4
Cooper, G. F., Davidson, J. P., and Blundy, J. D. (2016). Plutonic Xenoliths from Martinique, Lesser Antilles: Evidence for Open System Processes and Reactive Melt Flow in Island Arc Crust. Contrib. Mineral. Petrol. 171, 87. doi:10.1007/s00410-016-1299-8
Coulon, C., Clocchiatti, R., Maury, R. C., and Westercamp, D. (1984). Petrology of Basaltic Xenoliths in Andesitic to Dacitic Host Lavas from Martinique (Lesser Antilles): Evidence for Magma Mixing. Bull. Volcanol 47 (4), 705–734. doi:10.1007/BF01952340
Davidson, J. (1985). Mechanisms of Contamination in Lesser Antilles Island Arc Magmas from Radiogenic and Oxygen Isotope Relationships. Earth Planet. Sci. Lett. 72 (2–3), 163–174. doi:10.1016/0012-821X(85)90003-2
Davidson, J. P. (1987). Crustal Contamination versus Subduction Zone Enrichment: Examples from the Lesser Antilles and Implications for Mantle Source Compositions of Island Arc Volcanic Rocks. Geochimica et Cosmochimica Acta 51, 2185–2198. doi:10.1016/0016-7037(87)90268-7
Davidson, J. P., and Harmon, R. S. (1989). Oxygen Isotope Constraints on the Petrogenesis of Volcanic Arc Magmas from Martinique, Lesser Antilles. Earth Planet. Sci. Lett. 95 (3–4), 255–270. doi:10.1016/0012-821X(89)90101-5
Davidson, J. P., Hora, J. M., Garrison, J. M., and Dungan, M. A. (2005). Crustal Forensics in Arc Magmas. J. Volcanology Geothermal Res. 140 (1-3), 157–170. doi:10.1016/j.jvolgeores.2004.07.019
Davidson, J. P. (1986). Isotopic and Trace Element Constraints on the Petrogenesis of Subduction-Related Lavas from Martinique, Lesser Antilles. J. Geophys. Res. 91 (B6), 5943. doi:10.1029/JB091iB06p05943
Davidson, J. P., Morgan, D. J., Charlier, B. L. A., Harlou, R., and Hora, J. M. (2007). Microsampling and Isotopic Analysis of Igneous Rocks: Implications for the Study of Magmatic Systems. Annu. Rev. Earth Planet. Sci. 35 (1), 273–311. doi:10.1146/annurev.earth.35.031306.140211
Davidson, J., and Wilson, M. (2011). Differentiation and Source Processes at Mt Pelée and the Quill; Active Volcanoes in the Lesser Antilles Arc. J. Petrol. 52 (7–8), 1493–1531. doi:10.1093/petrology/egq095
Deegan, F. M., Troll, V. R., Freda, C., Misiti, V., Chadwick, J. P., McLeod, C. L., et al. (2010). Magma-Carbonate Interaction Processes and Associated CO2 Release at Merapi Volcano, Indonesia: Insights from Experimental Petrology. J. Petrol. 51 (5), 1027–1051. doi:10.1093/petrology/egq010
DePaolo, D. J. (1981). Trace Element and Isotopic Effects of Combined Wallrock Assimilation and Fractional Crystallization. Earth Planet. Sci. Lett. 53 (2), 189–202. doi:10.1016/0012-821X(81)90153-9
DuFrane, S. A., Turner, S., Dosseto, A., and van Soest, M. (2009). Reappraisal of Fluid and Sediment Contributions to Lesser Antilles Magmas. Chem. Geology. 265 (3–4), 272–278. doi:10.1016/j.chemgeo.2009.03.030
Escalona, A., and Mann, P. (2011). Tectonics, basin Subsidence Mechanisms, and Paleogeography of the Caribbean-South American Plate Boundary Zone. Mar. Pet. Geology. 28 (1), 8–39. doi:10.1016/j.marpetgeo.2010.01.016
Germa, A., Quidelleur, X., Labanieh, S., Chauvel, C., and Lahitte, P. (2011). The Volcanic Evolution of Martinique Island: Insights from K-Ar Dating into the Lesser Antilles Arc Migration since the Oligocene. J. Volcanology Geothermal Res. 208 (3-4), 122–135. doi:10.1016/j.jvolgeores.2011.09.007
Ginibre, C., and Davidson, J. P. (2014). Sr Isotope Zoning in Plagioclase from Parinacota Volcano (Northern Chile): Quantifying Magma Mixing and Crustal Contamination. J. Petrol. 55 (6), 1203–1238. doi:10.1093/petrology/egu023
Gourgaud, A., Fichaut, M., and Joron, J. L. (1989). Magmatology of Mt. Pelée (Martinique, FWI). I: Magma Mixing and Triggering of the 1902 and 1929 Pelean Nuées Ardentes. J. Volcanology Geothermal Res. 38 (1-2), 143–169. doi:10.1016/0377-0273(89)90035-8
Heath, E., Macdonald, R., Belkin, H., Hawkesworth, C., and Sigurdsson, H. (1998). Magmagenesis at Soufriere Volcano, St Vincent, Lesser Antilles Arc. J. Petrol. 39 (10), 1721–1764. doi:10.1093/petroj/39.10.1721
Hu, Y., Teng, F.-Z., and Chauvel, C. (2021). Potassium Isotopic Evidence for Sedimentary Input to the Mantle Source of Lesser Antilles Lavas. Geochimica et Cosmochimica Acta 295, 98–111. doi:10.1016/j.gca.2020.12.013
Huang, F., Lundstrom, C. C., Sigurdsson, H., and Zhang, Z. (2011). U-series Disequilibria in Kick'em Jenny Submarine Volcano Lavas: A New View of Time-Scales of Magmatism in Convergent Margins. Geochimica et Cosmochimica Acta 75 (1), 195–212. doi:10.1016/j.gca.2010.05.036
Kelemen, P. B., Hanghøj, K., and Greene, A. R. (2007). One View of the Geochemistry of Subduction-Related Magmatic Arcs, with an Emphasis on Primitive Andesite and Lower Crust. TrGeo 3, 1–70. doi:10.1016/B0-08-043751-6/03035-8
Labanieh, S., Chauvel, C., Germa, A., Quidelleur, X., and Lewin, E. (2010). Isotopic Hyperbolas Constrain Sources and Processes under the Lesser Antilles Arc. Earth Planet. Sci. Lett. 298 (1–2), 35–46. doi:10.1016/j.epsl.2010.07.018
Labanieh, S., Chauvel, C., Germa, A., and Quidelleur, X. (2012). Martinique: A clear Case for Sediment Melting and Slab Dehydration as a Function of Distance to the Trench. J. Petrol. 53 (12), 2441–2464. doi:10.1093/petrology/egs055
Lindsay, J. M., Trumbull, R. B., and Siebel, W. (2005). Geochemistry and Petrogenesis of Late Pleistocene to Recent Volcanism in Southern Dominica, Lesser Antilles. J. Volcanology Geothermal Res. 148 (3-4), 253–294. doi:10.1016/j.jvolgeores.2005.04.018
Martel, C., Pichavant, M., Holtz, F., Scaillet, B., Bourdier, J.-L., and Traineau, H. (1999). Effects offO2and H2O on Andesite Phase Relations between 2 and 4 Kbar. J. Geophys. Res. 104 (B12), 29453–29470. doi:10.1029/1999JB900191
Mason, E., Edmonds, M., and Turchyn, A. V. (2017). Remobilization of Crustal Carbon May Dominate Volcanic Arc Emissions. Science 357 (6348), 290–294. doi:10.1126/science.aan5049
Melekhova, E., Schlaphorst, D., Blundy, J., Kendall, J.-M., Connolly, C., McCarthy, A., et al. (2019). Lateral Variation in Crustal Structure along the Lesser Antilles Arc from Petrology of Crustal Xenoliths and Seismic Receiver Functions. Earth Planet. Sci. Lett. 516, 12–24. doi:10.1016/j.epsl.2019.03.030
Neill, I., Kerr, A. C., Hastie, A. R., Pindell, J. L., and Millar, I. L. (2013). The Albian-Turonian Island Arc Rocks of Tobago, West Indies: Geochemistry, Petrogenesis, and Caribbean Plate Tectonics. J. Petrol. 54 (8), 1607–1639. doi:10.1093/petrology/egt025
Parra, M., Faugères, J. C., Grousset, F., and Pujol, C. (1997). Sr-Nd Isotopes as Tracers of fine-grained Detrital Sediments: the South-Barbados Accretionary Prism during the Last 150 Kyr. Mar. Geology. 136 (3-4), 225–243. doi:10.1016/S0025-3227(96)00066-7
Plank, T., and Langmuir, C. H. (1993). Tracing Trace Elements from Sediment Input to Volcanic Output at Subduction Zones. Nature 362 (6422), 739–743. doi:10.1038/362739a0
Rojas-Agramonte, Y., Williams, I. S., Arculus, R., Kröner, A., García-Casco, A., Lázaro, C., et al. (2017). Ancient Xenocrystic Zircon in Young Volcanic Rocks of the Southern Lesser Antilles Island Arc. Lithos 290-291, 228–252. doi:10.1016/j.lithos.2017.08.002
Rousseau, T. C. C., Roddaz, M., Moquet, J.-S., Handt Delgado, H., Calves, G., and Bayon, G. (2019). Controls on the Geochemistry of Suspended Sediments from Large Tropical South American Rivers (Amazon, Orinoco and Maroni). Chem. Geology. 522, 38–54. doi:10.1016/j.chemgeo.2019.05.027
Salters, V. J. M., and Stracke, A. (2004). Composition of the Depleted Mantle. Geochem. Geophys. Geosyst. 5 (5), a–n. doi:10.1029/2003GC000597
Smith, T. E., Thirlwall, M. F., and Macpherson, C. (1996). Trace Element and Isotope Geochemistry of the Volcanic Rocks of Bequia, Grenadine Islands, Lesser Antilles Arc: A Study of Subduction Enrichment and Intra-crustal Contamination. J. Petrol. 37 (1), 117–143. doi:10.1093/petrology/37.1.117
Speed, R. C., Smith-Horowitz, P. L., Perch-Nielsen, K. V. S., and Sanfilippo, A. B. (1993). Southern Lesser Antilles Arc Platform: Pre-late Miocene Stratigraphy, Structure, and Tectonic Evolution, 277. Geological Society of America.
Stamper, C. C., Blundy, J. D., Arculus, R. J., and Melekhova, E. (2014). Petrology of Plutonic Xenoliths and Volcanic Rocks from Grenada, Lesser Antilles. J. Petrol. 55 (7), 1353–1387. doi:10.1093/petrology/egu027
Streckeisen, A. (1976). To Each Plutonic Rock its Proper Name. Earth-science Rev. 12 (1), 1–33. doi:10.1016/0012-8252(76)90052-0
Thirlwall, M. F., Graham, A. M., Arculus, R. J., Harmon, R. S., and Macpherson, C. G. (1996). Resolution of the Effects of Crustal Assimilation, Sediment Subduction, and Fluid Transport in Island Arc Magmas: PbSrNdO Isotope Geochemistry of Grenada, Lesser Antilles. Geochimica et Cosmochimica Acta 60 (23), 4785–4810. doi:10.1016/S0016-7037(96)00272-4
Thirlwall, M. F., and Graham, A. M. (1984). Evolution of High-Ca, High-Sr C-Series Basalts from Grenada, Lesser Antilles: the Effects of Intra-crustal Contamination. J. Geol. Soc. 141 (3), 427–445. doi:10.1144/gsjgs.141.3.0427
Thirlwall, M. F. (1991). Long-term Reproducibility of Multicollector Sr and Nd Isotope Ratio Analysis. Chem. Geology. Isotope Geosci. section 94 (2), 85–104. doi:10.1016/0168-9622(91)90002-E
Tollan, P. M. E., Bindeman, I., and Blundy, J. D. (2012). Cumulate Xenoliths from St. Vincent, Lesser Antilles Island Arc: A window into upper crustal differentiation of mantle-derived basalts. Contrib Mineral Petrol. 163 (2), 189–208. doi:10.1007/s00410-011-0665-9
Toothill, J., Williams, C. A., MacDonald, R., Turner, S. P., Rogers, N. W., Hawkesworth, C. J., et al. (2007). A complex petrogenesis for an arc magmatic suite, St Kitts, Lesser Antilles. Journal of Petrol. 48 (1), 3–42. doi:10.1093/petrology/egl052
Troll, V. R., Hilton, D. R., Jolis, E. M., Chadwick, J. P., Blythe, L. S., Deegan, F. M., et al. (2012). Crustal CO2liberation during the 2006 eruption and earthquake events at Merapi volcano, Indonesia. Geophys. Res. Lett. 39 11302. doi:10.1029/2012GL051307
Turner, S., Hawkesworth, C., van Calsteren, P., Heath, E., Macdonald, R., and Black, S. (1996). U-series isotopes and destructive plate margin magma genesis in the Lesser Antilles. Earth and Planetary Science Letters 142 (1–2), 191–207. doi:10.1016/0012-821X(96)00078-7
Van Soest, M. C., Hilton, D. R., Macpherson, C. G., and Mattey, D. P. (2002). Resolving Sediment Subduction and Crustal Contamination in the Lesser Antilles Island Arc: A Combined He-O-Sr Isotope Approach. J. Petrol. 43 (1), 143–170. doi:10.1093/petrology/43.1.143
Van Soest, M. C. (2000). Sediment Subduction and Crustal Contamination in the Lesser Antilles Island Arc: The Geochemical and Isotopic Imprints on Recent Lavas and Geothermal Fluids. PhD Thesis. Amsterdam (Netherlands): Vriej Universiteit Amsterdam.
Viers, J., Roddaz, M., Filizola, N., Guyot, J.-L., Sondag, F., Brunet, P., et al. (2008). Seasonal and Provenance Controls on Nd-Sr Isotopic Compositions of Amazon Rivers Suspended Sediments and Implications for Nd and Sr Fluxes Exported to the Atlantic Ocean. Earth Planet. Sci. Lett. 274 (3-4), 511–523. doi:10.1016/j.epsl.2008.08.011
Waight, T. E., and Tørnqvist, J. B. (2018). Sr Isotope Zoning in Plagioclase from Andesites at Cabo De Gata, Spain: Evidence for Shallow and Deep Contamination. Lithos 308-309, 159–167. doi:10.1016/j.lithos.2018.03.007
Weis, D., Kieffer, B., Maerschalk, C., Barling, J., De Jong, J., Williams, G. A., et al. (2006). High-precision Isotopic Characterization of USGS Reference Materials by TIMS and MC-ICP-MS. Geochem. Geophys. Geosyst. 7 (8). doi:10.1029/2006GC001283
White, W. M., and Dupré, B. (1986). Sediment subduction and magma genesis in the Lesser Antilles: Isotopic and trace element constraints. J. Geophys. Res. 91 (B6), 5927. doi:10.1029/JB091iB06p05927
Xie, X., Mann, P., and Escalona, A. (2010). Regional Provenance Study of Eocene Clastic Sedimentary Rocks within the South America-Caribbean Plate Boundary Zone Using Detrital Zircon Geochronology. Earth Planet. Sci. Lett. 291 (1-4), 159–171. doi:10.1016/j.epsl.2010.01.009
Ysaccis, R. (1997). Tertiary Evolution of the Northeastern Venezuela Offshore. PhD Thesis. Houston, Texas (USA): Rice University.
Keywords: crustal sediment assimilation, plutonic xenoliths, strontium isotopes, lesser antilles arc, plagioclase, martinique
Citation: Brown JR, Cooper GF, Nowell GM, Macpherson CG, Neill I and Prytulak J (2021) Isotopic Compositions of Plagioclase From Plutonic Xenoliths Reveal Crustal Assimilation Below Martinique, Lesser Antilles Arc. Front. Earth Sci. 9:682583. doi: 10.3389/feart.2021.682583
Received: 18 March 2021; Accepted: 11 May 2021;
Published: 25 May 2021.
Edited by:
Michel Grégoire, Center National de la Recherche Scientifique (CNRS), FranceCopyright © 2021 Brown, Cooper, Nowell, Macpherson, Neill and Prytulak. This is an open-access article distributed under the terms of the Creative Commons Attribution License (CC BY). The use, distribution or reproduction in other forums is permitted, provided the original author(s) and the copyright owner(s) are credited and that the original publication in this journal is cited, in accordance with accepted academic practice. No use, distribution or reproduction is permitted which does not comply with these terms.
*Correspondence: J. R. Brown, am9zaHVhLnIuYnJvd25AZHVyaGFtLmFjLnVr