- 1School of Earth Science and Resources, China University of Geosciences (Beijing), Beijing, China
- 2State Key Laboratory of Biogeology and Environmental Geology, China University of Geosciences (Beijing), Beijing, China
- 3International Center for Isotope Effect Research, Nanjing University, Nanjing, China
- 4School of Earth Sciences and Engineering, Nanjing University, Nanjing, China
- 5School of Earth and Environmental Sciences, University of St Andrews, St Andrews, United Kingdom
The middle Ediacaran Period records one of the deepest negative carbonate carbon isotope (δ13Ccarb) excursions in Earth history (termed the Shuram excursion). This excursion is argued by many to represent a large perturbation of the global carbon cycle. If true, this event may also have induced significant changes in the nitrogen cycle, because carbon and nitrogen are intimately coupled in the global ocean. However, the response of the nitrogen cycle to the Shuram excursion remains ambiguous. Here, we reported high resolution bulk nitrogen isotope (δ15N) and organic carbon isotope (δ13Corg) data from the upper Doushantuo Formation in two well-preserved sections (Jiulongwan and Xiangerwan) in South China. The Shuram-equivalent excursion is well developed in both localities, and our results show a synchronous decrease in δ15N across the event. This observation is further supported by bootstrapping simulations taking into account all published δ15N data from the Doushantuo Formation. Isotopic mass balance calculations suggest that the decrease in δ15N during the Shuram excursion is best explained by the reduction of isotopic fractionation associated with water column denitrification (εwd) in response to feedbacks between carbon and nitrogen cycling, which were modulated by changes in primary productivity and recycled nutrient elements through remineralization of organic matter. The study presented here thus offers a new perspective for coupled variations in carbon and nitrogen cycles and sheds new light on this critical time in Earth history.
Introduction
Unraveling the global carbon cycle in deep time of Earth history mostly relies on the analyses of carbon isotope compositions in carbonate rocks (δ13Ccarb) and organic matter (δ13Corg) (Kump and Arthur, 1999). With the expansion of δ13Ccarb datasets over the past 2 decades (cf. Lyons et al., 2014), a general δ13Ccarb picture from the Archean to present has become evident. A major feature of this curve is that the magnitude of isotopic variation in the Precambrian is much larger than that of the Phanerozoic, as exemplified by the notable Shuram negative δ13Ccarb excursion documented in the middle Ediacaran (Burns and Matter, 1993; Fike et al., 2006; Le Guerroué et al., 2006a, 2006b). This excursion and its possible equivalents are widely distributed in Ediacaran successions all over the world and have been generally used as a tie-point for the stratigraphic correlations across different continents (Jiang et al., 2007; Zhu et al., 2007; Halverson et al., 2010; Grotzinger et al., 2011; Wang et al., 2012; Lu et al., 2013; Husson et al., 2015; Wang et al., 2016; Zhou et al., 2017), although whether they are truly synchronous remains to be tested independently by precise radiometric ages (Grotzinger et al., 2011; Zhou et al., 2017).
Among all negative δ13Ccarb excursions from the Archean to Phanerozoic, the Shuram excursion is remarkable for two reasons: first, it is one of the largest negative excursions in Earth history, with δ13Ccarb decreasing from +5‰ to as low as −12‰ (Grotzinger et al., 2011); second, it may have lasted for millions of years (∼5–50 Myr) before returning to positive values (Le Guerroué et al., 2006a, 2006b; Bowring et al., 2007; Jiang et al., 2007; Cui et al., 2015; Sui et al., 2019; Canfield et al., 2020; Gong and Li, 2020; Rooney et al., 2020). The anomalously low δ13Ccarb values (down to −12‰) during the Shuram excursion are below the mantle-derived carbon isotope value of −6‰ (Melezhik et al., 2005) and can therefore not be readily explained by the conventional steady-state mass balance model of carbon isotopes (Kump and Arthur, 1999). Several non-steady-state models link the Shuram excursion to the rise of oxygen in the atmosphere-ocean system, which may have resulted in the oxidation of an inferred large dissolved organic carbon (DOC) reservoir in deep ocean (Rothman et al., 2003; Fike et al., 2006; Jiang et al., 2007; McFadden et al., 2008) and/or other reduced carbon sources, including terrestrial organic matter (Kaufman et al., 2007; Shi et al., 2018), methane hydrates (Bjerrum and Canfield, 2011) and expelled hydrocarbons (Lee et al., 2015). These models imply that the Shuram excursion recorded the primary isotopic composition of seawater, which is further supported by a recent in situ carbon isotope study from the Wonoka Formation in Australia (Husson et al., 2020). These hypotheses have been challenged on the basis that the oxidant budget may have been insufficient if the Shuram excursion lasted for more than 5 Myr (Bristow and Kennedy, 2008); however, recent biogeochemical modeling results suggest that the oxidants required for the oxidiation of DOC could have derived from the weathering of sulfate evaporites (Shields et al., 2019). Alternatively, the Shuram excursion has been interpreted as reflecting secondary processes such as meteoric alteration (Knauth and Kennedy, 2009; Swart and Kennedy, 2012), burial diagenesis (Derry, 2010), or the contribution from authigenic carbonates (Schrag et al., 2013; Cui et al., 2017; Jiang et al., 2019). However, these diagenetic processes, which are essentially local phenomena, are difficult to reconcile with the global distribution of the Shuram excursion and its unique occurrence in the Ediacaran (Grotzinger et al., 2011). Additionally, Paulsen et al. (2017) argued that the Shuram excursion may be partially attributed to extensive release of mantle 12C-enriched carbon associated with carbonatite and alkaline magmatism during the Ediacaran period, but the extremely low δ13Ccarb values of the Shuram excursion still require additional input from surface processes.
The carbon cycle has intimate relationships with the nitrogen and oxygen cycles in the global ocean (Fennel et al., 2005). Nitrogen is one of the major nutrient elements required for all life. In the excess of bioavailable P, fixed N may become an important limiting nutrient in the ocean and thereby control the amount of carbon sequestered into sediments and the rate of oxygen production through photosynthesis (Falkowski, 1997; Tyrrell, 1999). Conversely, the concentration of fixed N is mainly determined by the balance between N2 fixation, the major pathway of N into aquatic ecosystems, and the reconversion of fixed nitrogen to N2 gas mainly via denitrification (stepwise reduction of nitrate to N2) and anammox (coupled oxidation of ammonium with reduction of nitrite) (Sigman et al., 2009; Devol, 2015), which in turn is largely dependent on the ocean redox structure (Quan et al., 2013; Ader et al., 2016; Stüeken et al., 2016). During denitrification, organic matter is an important electron donor (Sigman et al., 2009), although it can be replaced by ferrous Fe(II) or hydrogen sulfide (H2S) (Lam and Kuypers, 2011; Michiels et al., 2017). Coupled nitrate (NO3−) reduction and the oxidation of organic matter through denitrification would result in the transformation of 12C-enriched organic carbon to inorganic carbon, and the preferential release of light 14N to the atmosphere, thereby elevating the δ15N in the fixed N pool and lowering the δ13C of the dissolved inorganic carbon pool (Sigman et al., 2009; Kump et al., 2011). Further, the remineralization of organic matter in the oceans would release the organic-bound N into seawater with limited nitrogen isotopic fractionation (−1 to +2‰, Ader et al., 2016; Stüeken et al., 2016), which could serve as new nutrient N source to fuel productivity (Higgins et al., 2012; Xu et al., 2020).
The Shuram excursion, if recording a large perturbation in the carbon cycle, provides an excellent window into the feedback between carbon, oxygen and nitrogen cycles in deep time. However, the role of the nitrogen cycle in the Shuram excursion has not been systematically investigated. Kikumoto et al. (2014) reported nitrogen isotope data from the Ediacaran to early Cambrian in a drill core in the Yangtze Gorges area, South China, and found a coherent decrease in δ15N along with the Shuram excursion in the upper Doushantuo Formation (Locally named as N3, EN3 or DOUNCE, Jiang et al., 2007; Zhou and Xiao, 2007; Zhu et al., 2013). This negative δ15N excursion was interpreted as evidence for an increased nitrate pool which may have resulted in the partial assimilation of NO3− (Kikumoto et al., 2014; Nishizawa et al., 2019). Nitrogen isotope data have also been reported from the other Ediacaran sections in South China and other continents (e.g., Ader et al., 2014; Spangenberg et al., 2014; Wang et al., 2017; Chen et al., 2019; Lan et al., 2019; Nishizawa et al., 2019), but the Shuram excursion is poorly developed or missing in these sections. In this contribution, we report high resolution δ15N and δ13Corg data from the upper Doushantuo Formation in two sections (Jiulongwan and Xiangerwan) in the Yangtze Gorges area, South China, where the Shuram-EN3 excursion is well recorded (Jiang et al., 2007; An et al., 2015; Zhou et al., 2017). The new data presented here, along with previous ones, will shed new light on the origin of the Shuram excursion and the coevolution of carbon and nitrogen cycles during this critical interval.
Geological Setting and Study Sections
The Ediacaran Doushantuo Formation was deposited in an inferred passive continental margin setting along the southeast part of the South China Block, following the termination of the Marinoan glaciation (Wang and Li, 2003; Jiang et al., 2011). In the Yangtze Gorges area, western Hubei Province, the Doushantuo Formation in the stratotype (Jiulongwan) section can be divided into four lithological members: Member I is a 3–5 m thick cap carbonate, Member II is characterized by interlayered black shale and muddy limestone with abundant centimeter-scaled chert nodules, Member III is dominated by carbonate deposits, and Member IV consists mainly of black shales with meter-scale carbonate concretions (e.g., Jiang et al., 2011). Abundant microfossils and macrofossils have been reported from the Doushantuo Formation, providing a unique window into eukaryotic evolution leading up to the Cambrian Explosion, as well as a valuable tool for biostratigraphic subdivision (Liu et al., 2014; Xiao et al., 2016; Zhou et al., 2019 and references therein). Geochronologically, the depositional age of the Doushantuo Formation has previously been constrained to ca. 635–551 Ma based on U-Pb zircon dating (Condon et al., 2005; Zhang et al., 2005). Regional stratigraphic correlation suggest that the ca. 551 Ma tuffaceous layer within the Miaohe Member, which was traditionally correlated with Member IV, is likely located in the overlying Dengying Formation (An et al., 2015), implying that the upper boundary of the Doushantuo Formation is older than 551 Ma. However, a more detailed investigation of δ13Ccarb of multiple sections around the Huangling Anticline (Zhou et al., 2017) and the δ13Corg study from the Miaohe member (Xiao et al., 2017) argue against the correlation scheme proposed by An et al. (2015).
Our samples were collected from the upper part of the Doushantuo Formation in the Jiulongwan and Xiangerwan sections in the Yangtze Gorges area (Figure 1). These two sections were chosen for nitrogen isotope analyses due to the excellent preservation of the Shuram-EN3 carbon isotope excursion (Jiang et al., 2007; An et al., 2015; Zhou et al., 2017). Paleogeographically, the two sections were both deposited in proximal settings of an intrashelf basin (Jiang et al., 2011; An et al., 2015). The Jiulongwan section, located in the southern part of the Huangling Anticline (Figure 1), has been extensively studied to characterize the ocean redox structure (e.g., Jiang et al., 2007; McFadden et al., 2008; Li et al., 2010; Zhou et al., 2012; Ling et al., 2013; Wei et al., 2018; Fan et al., 2020, 2021). The upper Doushantuo Formation in the Jiulongwan section is dominated by cherty dolostone, muddy limestone/dolostone and black shales (Figure 2). The Shuram-EN3 excursion begins in the middle part of Member III, continued upwards through the rest of the Doushantuo Formation until return to positive δ13Ccarb values at the Doushantuo/Dengying boundary (Figure 2). The Xiangerwan section is located in the northwestern part of the Huangling Anticline (Figure 1), about 60 km away from the Jiulongwan section. The upper Doushantuo Formation in this section is mainly composed of medium-thick bedded dolostone, pink dolostone and thinly bedded limestone with subordinate black shales (Figure 3). The pink dolostone was interpreted as the precipitate of a primary marine red bed enabled by ocean oxygenation (Song et al., 2017). The pattern of the Shuram-EN3 excursion in the Xiangerwan section is similar to that of the Jiulongwan section (Figure 3).
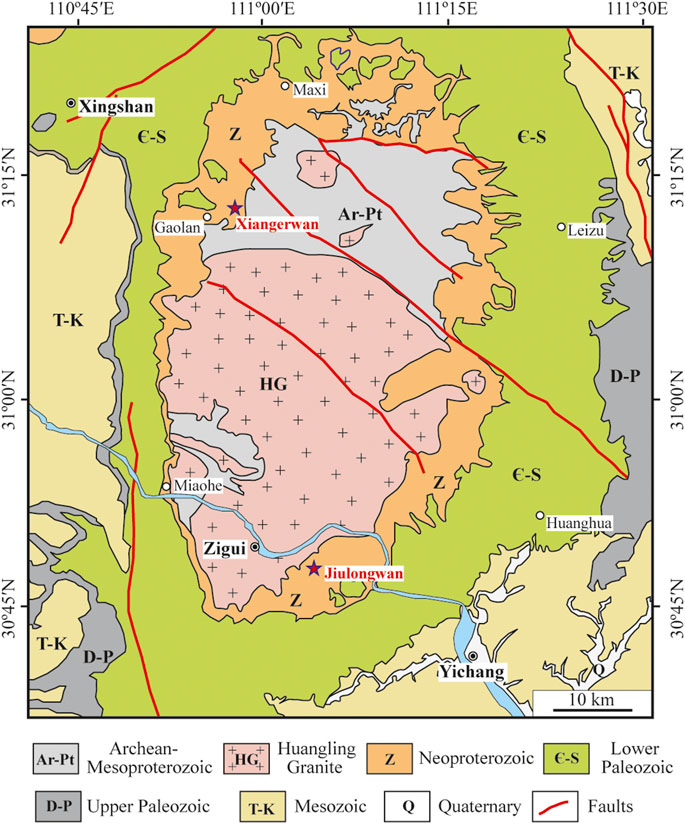
FIGURE 1. Simplified geological map in the Yangtze Gorges area (modified from An et al., 2015). Red stars show the locations of two study sections.
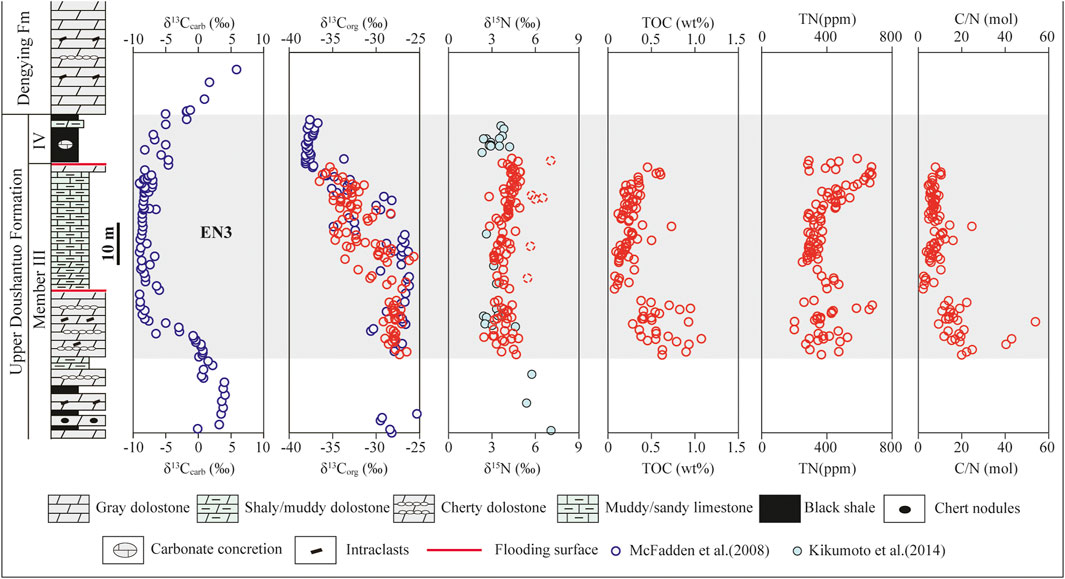
FIGURE 2. The geochemical profiles of δ13Ccarb, δ13Corg, δ15N, TOC, TN and MC/N spanning the Shuram-EN3 excursion in the Jiulongwan section. The data points with dashed outline fall away from the general trend.
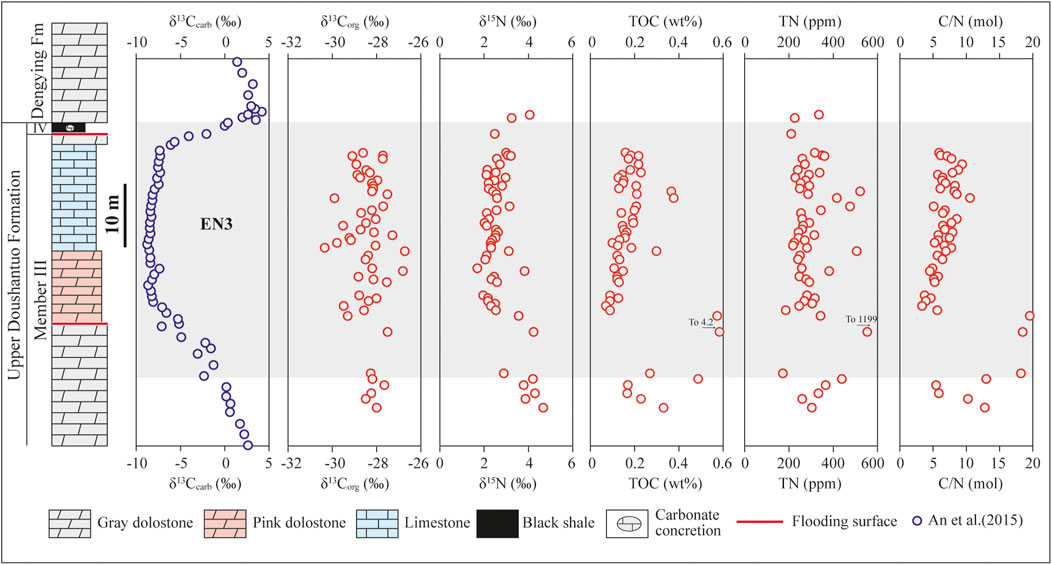
FIGURE 3. The geochemical profiles of δ13Ccarb, δ13Corg, δ15N, TOC, TN and MC/N spanning the Shuram-EN3 excursion in the Xiangerwan section.
Analytical Method
We performed measurements of δ15N and δ13Corg, total nitrogen (TN) contents, and total organic carbon (TOC) contents for 162 samples. Fresh sample chips without any weathering surfaces or visible veins were ground into powders below 200 mesh in an agate mortar. About 10 g of powders from each sample were treated with 2 M excess HCl to ensure the complete removal of carbonate. The carbonate-free samples were then rinsed with deionized water multiple times until a near-neutral pH value was reached. After centrifuging, the decarbonated sample residue was dried at 70°C in an oven before analysis. The isotopic and elemental compositions were measured in the Oxy-Anion Stable Isotope Consortium (OASIC) at Louisiana State University (LSU), using a Vario Microcube Elemental Analyzer (EA) connected to an Isoprime 100 isotope ratio mass spectrometer (IRMS). Because most of our samples are TOC-lean carbonate rocks, approximately 50–100 mg sample powders (based on TOC estimation) were wrapped in tin capsules and combusted in the EA to enhance the N signal. The organic carbon isotope compositions are reported as δ values with reference to the Vienna Pee Dee Belemnite standard (VPDB). The nitrogen isotope compositions are reported in standard δ notation in per mil (‰) deviations from atmospheric N2 (0‰, Air). Reference standard acetanilide-OASIC (δ13C = −27.62‰, δ15N = +1.61‰) was used to calibrate the analytical results. Measurements of C and N concentrations of blanks were below detection limits, suggesting that contamination from capsules did not contribute much to our results. A few samples (n = 10) with N peaks much lower than that of the reference standard were excluded from the dataset and from the discussion. The reproducibility monitored by the reference material was better than 0.1‰ for δ13Corg and 0.3‰ for δ15N.
Results
The analytical results of δ15N, δ13Corg, TN, TOC and C/N are shown in Figures 2, 3 and Supplementary Tables S1, S2. The δ13Corg variations in the Jiulongwan section are well matched with previously published data by McFadden et al. (2008) (Figure 2). In the prelude and lower part of the Shuram-EN3 excursion, δ13Corg is relatively high and centers around −28‰. It becomes more variable but shows an overall decreasing trend in the rest of Member III. Low and stable δ13Corg values cluster around -38‰ in the Member IV black shale. The δ15N data of our samples in the Jiulongwan section largely overlap with those reported from the equivalent interval in the nearby (within 3 km) Wuhe drillcore (Figure 2; Kikumoto et al., 2014). Before the Shuram-EN3 excursion, a few data points show relatively high δ15N values from +5.4‰ to +6.7‰. During the main phase of the Shuram-EN3 excursion, most of δ15N values fall in the range of +3‰ to +5.5‰, except for a few outliers, with an average of +3.8 ± 0.8‰ (n = 123) (Figure 2; this study; Kikumoto et al., 2014). The variability in δ15N is independent of lithological changes.
In the Xiangerwan section, δ13Corg does not show any clear stratigraphic trend across the Shuram-EN3 excursion. It varies between −30.3‰ and −26.7‰, with an average of −28.4 ± 0.7‰ (n = 49) (Figure 3). Variations in δ15N from this section generally mirror the trend of δ13Ccarb reported by An et al. (2015), although the magnitude of change is small (Figure 3). Prior to the Shuram-EN3 excursion, δ15N varies from +3.0‰ to +4.7‰ and the mean value is +4.0 ± 0.6‰ (n = 6). During the main stage of excursion, most samples have δ15N values between +2.0‰ and +3.8‰. The average value (+2.5 ± 0.4‰, n = 43) is about 1.5‰ lower than that of the pre-excursion interval. An increase of δ15N relative to pre-excursion values is observed in the upper Doushantuo Formation, coincident with the rising branch of the Shuram-EN3 excursion. Overall, the δ15N values from the Xiangerwan section are 1–2‰ lower than those from the Jiulongwan section.
Discussion
Evaluation of the Preservation of Primary Isotopic Signals
Whether δ15N and δ13Corg in sedimentary rocks record primary autochthonous signatures is dependent on several factors, including paleogeographic setting, diagenesis, metamorphism, and potential contributions of allochthonous materials (Ader et al., 2009; Ader et al., 2016; Robinson et al., 2012; Stüeken et al., 2016). In modern oceans, sedimentary δ15N of organic matter may show large offsets compared to sinking organic particles at sites located off continental margins due to extended remineralization of organic matter in the water column and upper sediment. In contrast, on continental shelves with high sediment accumulation rates and/or organic matter content, sedimentary organic matter generally displays δ15N values similar to that of sinking particles (Robinson et al., 2012). The two study sections were deposited in shallow shelf environments (Jiang et al., 2011; An et al., 2015), minimizing this effect on sedimentary δ15N as well as δ13Corg. However subsequent biological decomposition and thermal maturation of sedimentary organic matter during early and burial diagenesis can still perturb the original δ15N and δ13Corg values (Ader et al., 2009; Ader et al., 2016; Stüeken et al., 2016). Several lines of evidence, however, argue against these processes as major controls on our data. First, the isotopic alteration by biological decomposition during early diagenesis is generally small for δ13Corg (Ader et al., 2009 and references therein). Although this effect could be large for δ15N (up to 4‰) when bottom water was oxic (Altabet et al., 1999; Freudenthal et al., 2001; Lehmann et al., 2002; Prokopenko et al., 2006), Fe speciation data, I/(Ca + Mg) ratios and Ce anomales (Ce/Ce*) from the Jiulongwan and Xiangerwan sections indicate oxygen-depleted bottom water conditions (Li et al., 2010; Zhou et al., 2012; Ling et al., 2013; Wei et al., 2019). Second, Raman spectra and equivalent vitrinite reflectance data suggested that the peak heating temperature of the Doushantuo Formation was <300°C (Chang et al., 2020), and at such low metamorphic grade thermal alterations of isotopic composition would be limited for both δ15N and δ13Corg (Ader et al., 2009; Ader et al., 2016; Stüeken et al., 2016; Stüeken et al., 2017). Lastly, if the original δ13Corg and δ15N were largely modified by the preferential loss of light 12C and/or 14N during early and burial diagenesis, one would expect correlation between δ15N, TN, C/N, and δ13Corg as well as δ13Corg and TOC. No significant correlations, however, are observed between these parameters (Figure 4). A subset of samples show a negative correlation between δ13Corg and TOC commonly seen in Precambrian rocks, but the reason of this is unknown based on current research and warrants further investigation.
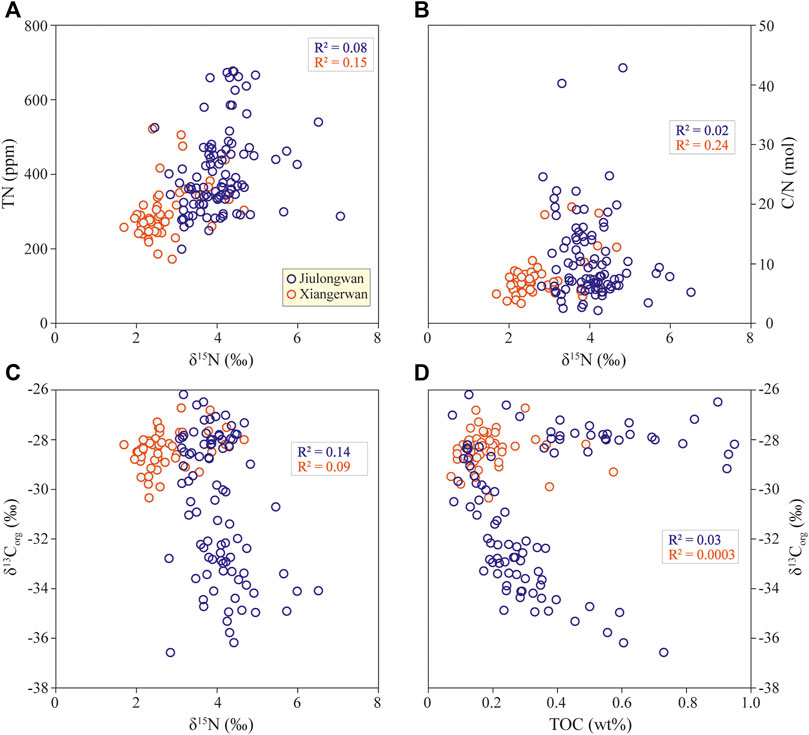
FIGURE 4. Cross plots of TN vs. δ15N (A), C/N vs. δ15N (B), δ13Corg vs. δ15N (C), and δ13Corg vs. TOC (D) in study sections.
The addition of material from allochthonous sources is another factor that can affect sedimentary δ13Corg and δ15N. These allochthonous sources include hydrothermal fluids and detrital input. Hydrothermal fluids are unlikely to increase the organic carbon to sedimentary rocks, but they could contain some inorganic N that can be trapped by sedimentary clay when moving along the fractures, thereby affecting the bulk δ15N (e.g., Zerkle et al., 2017; Luo et al., 2018). However, no clear correlation is observed between potassium abundance (Wang, 2019) and δ15N and in the Xiangerwan section (Figure 5A), suggesting that exchange with hydrothermal fluids was limited and did not severely bias our δ15N values. For sedimentary rocks with low authigenic TOC contents, detrital organic matter could be a major component and overprint the primary δ13Corg (Jiang et al., 2010). It is difficult to quantify the contribution of detrital organic matter and detrital clay-bound N to our samples; however, δ15N, δ13Corg and TOC do not show correlations with Al content in the Xiangerwan section (Wang, 2019)─an indicator of detrital input (Figures 5B–D–D). Therefore, we suggest that detrital input did not significantly affect our data. Nevertheless, this question can be further clarified in a future dedicated study.
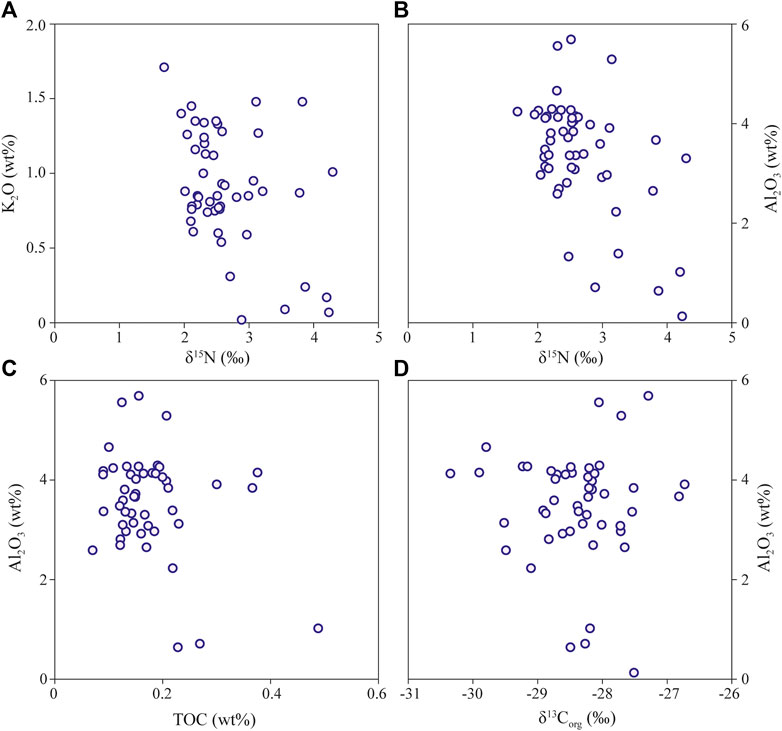
FIGURE 5. Cross plots of K2O vs. δ15N (A), Al2O3 vs. δ15N (B), Al2O3 vs. TOC (C), and Al2O3 vs. δ13Corg(D) in the Xiangerwan section. The Al2O3 and K2O data are from Wang (2019).
Variations of δ15N-δ13Ccarb Pattern in the Upper Doushantuo Formation
The upper Doushantuo Formation in South China is characterized by a prominent negative δ13Ccarb excursion (N3, EN3 or DOUNCE) that is widely accepted to be equivalent to the Shuram excursion (Jiang et al., 2007; Zhou and Xiao, 2007; Zhu et al., 2007; Zhu et al., 2013; McFadden et al., 2008; Wang et al., 2012; Lu et al., 2013; An et al., 2015; Wang et al., 2016; Li et al., 2017; Zhou et al., 2017; Wei et al., 2019). Although the Shuram-EN3 excursion has a wide paleogeographic distribution from the inner shelf to upper slope setting, it shows large spatial variations in its pattern, magnitude and stratigraphic coverage (Wang et al., 2016; Zhou et al., 2017). In some sections, this excursion is completely absent (Zhou et al., 2017). The discrepancy of the Shuram-EN3 excursion between different sections has been attributed to facies change, stratigraphic truncation, the diachronous nature of the Doushantuo/Dengying boundary and/or diagenetic overprinting of primary isotope signatures (Cui et al., 2015; Wang et al., 2016; Zhou et al., 2017).
δ15N data for the Ediacaran Doushantuo Formation were first reported from the Wuhe drillcore in Yangtze Gorges area by Kikumoto et al. (2014). They found a synchronous decrease in δ15N from ca. +6‰ to ca. +3.2‰ along with the Shuram-EN3 excursion (Figure 6B; Kikumoto et al., 2014). Our new δ15N data from the upper Doushantuo Formation in the Jiulongwan section, which is <3 km away from the Wuhe drillcore, are well consistent with the trend reported by Kikumoto et al. (2014) when excluding a few outliers (Figure 6B). In the inner shelf Xiangerwan section about 60 km north of the Jiulongwan section, the Shuram-EN3 excursion is well developed and shows striking similarity with that in the Jiulongwan section (Figure 6A; An et al., 2015). The δ15N data also mirror the trend observed in the Wuhe drillcore, although the absolute values are overall 1–2‰ lower than those of the Wuhe drillcore both before and during the Shuram-EN3 excursion. The δ15N-δ13Ccarb pattern from the upper Doushantuo Formation in the outer shelf Wangjiapeng drillcore section shows some differences compared to the Wuhe (Jiulongwan) and Xiangerwan sections (Figure 6C; Lan et al., 2019). The Shuram-EN3 excursion in this section is marked by a sharp decrease of δ13Ccarb to a minimum value of −5.4‰, followed by a quick return to positive values, while the associated δ15N in this interval shows an opposite (increasing) trend. It should be noted that δ15N in the Wangjiapeng section shows a small negative excursion in the ‘uppermost’ Doushantuo Formation, clearly postdating the Shuram-EN3 excursion (Lan et al., 2019). Whether this negative δ15N excursion can be correlated with that in the Wuhe and Xiangerwan sections is questionable and requires further investigation.
In the Yangjiaping section on the shelf margin, Ediacaran δ13Ccarb data were reported by several research groups (Zhu et al., 2007; Ader et al., 2009; Kunimitsu et al., 2011; Cui et al., 2015). Here the Shuram-EN3 excursion spans the upper Doushantuo Formation and the lower part of the overlying Dengying Formation, but the data show large sample-to-sample variations (Wang et al., 2016). A few δ15N data points from this interval show a decreasing trend from ∼ +4.3‰ to ∼ +3.0‰ (Figure 6D; Ader et al., 2014). More data are needed to confirm this variation. Chen et al. (2019) reported δ15N data from the Doushantuo Formation in an upper slope drill core section (zk 2012) in the Daotuo area, northeastern Guizhou Province. A conspicuous decline in δ15N was observed in the upper Doushantuo Formation (Figure 6E; Chen et al., 2019). Although no δ13Ccarb data were reported in zk 2012, the δ13Ccarb profile from an adjacent drill core verifies the presence of the Shuram-EN3 excursion in the upper Doushantuo Formation (Wei et al., 2019). Coupled δ15N-δ13Ccarb data have also been reported from the Lantian Formation in southern Anhui, which is equivalent to the Doushantuo Formation (Wang et al., 2017). The δ13Ccarb from this unit is dominated by negative values and show a negative excursion in the upper Lantian Formation that is considered to be correlative to the Shuram excursion. However, the δ15N data from this interval do not show a clear stratigraphic trend (Figure 6F; Wang et al., 2017). In the basinal Fentan section, the Shruam-EN3 excursion is documented in the upper Doushantuo Formation (Figure 6G; Lu et al., 2013; Furuyama et al., 2017). In the same interval, the δ15N also show a clear decreasing trend from ∼ +4.5‰ to nadir +0.7‰ (Figure 6G; Nishizawa et al., 2019). Recently, a δ15N study was performed for the Doushantuo Formation in the proximal E-Shan section (Peng et al., 2020), However, this section is not included in our correlation (Figure 6) because the Dousantuo Formation is dominated by siliciclastic rocks and do not contain the Shuram excursion.
To better characterize the overall δ15N trend during this critical time interval, we simulate the δ15N variations before and during the Shuram excursion for each section using a bootstrap resampling method. Out of seven sections, six sections are characterized by a bimodal δ15N distribution (Figure 7). Notably, although the δ15N data from the Lantian section do not show a clear stratigraphic trend (Wang et al., 2017), the model result indicates that δ15N during the Shuram excursion are statistically 0.6‰ lower than prior to the excursion (Figure 7E). In the Wangjiapeng section, the mean δ15N distributions obtained from bootstrap resampling largely overlap for pre- and syn-excursion strata (Figure 7C). This is likely due to a prominent negative δ15N excursion in the lower part of the Doushantuo Formation (Lan et al., 2019). The bimodal δ15N distribution becomes even more evident when the bootstrap method is applied to all data from the seven sections (Figure 7H), providing evidence for a synchronous decrease of δ15N associated with the Shuram excursion. This covariation pattern could be attributed to a feedback between nitrogen and carbon cycles that will be discussed in the following section.
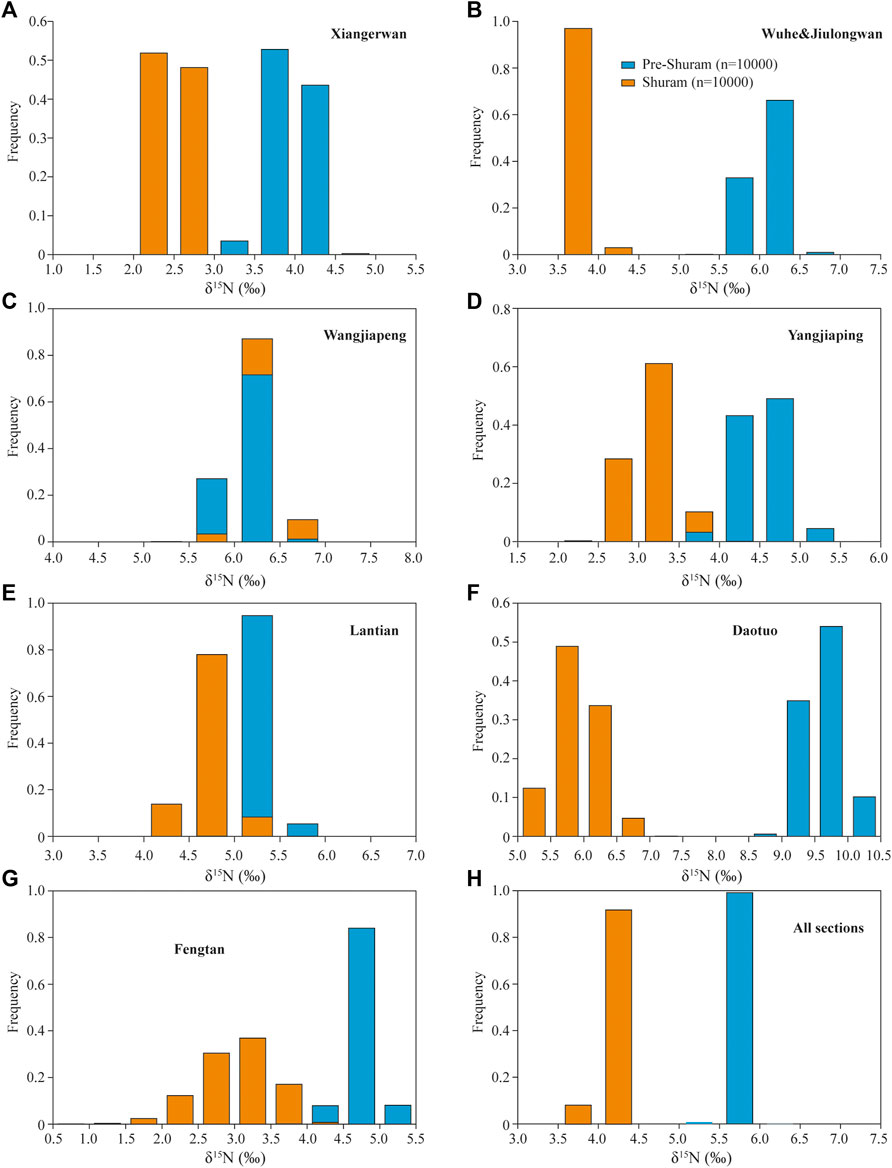
FIGURE 7. Bootstrap distribution of δ15N before and during the Shuram-EN3 excursion in the Xiangerean section (A), Jiulongwan section (B), Wangjiapeng drill core (C), Yangjiaping section (D), Lantian drill core (E), Daotuo drill core (F), Fengtan section (G), and all sections (H).
Feedback Between Nitrogen and Carbon Cycles
Modeled results indicate that δ15N data from the Ediacaran Doushantuo Formation show contrasting distributions before and during the Shuram excursion (Figure 7). High δ15N values from the lower-middle Doushantuo Formation prior to the Shuram excursion have been attributed to incomplete denitrification that preferentially removed light 14N from the ocean (Kikumoto et al., 2014; Ader et al., 2014; Wang et al., 2017, 2018; Chen et al., 2019; Lan et al., 2019; Xu et al., 2020). The decrease of δ15N associated with the Shuram excursion was previously interpreted as partial assimilation of NO3− from an expanding nitrate pool in response to a rise of oxygen levels in the ocean-atmosphere system, implying that dissolved P rather than N was the limiting nutrient during the excursion interval (Kikumoto et al., 2014; Nishizawa et al., 2019). We challenge this explanation for the following reasons. First, if the decrease in δ15N was caused by partial assimilation of NO3−, the 15N-enriched residual NO3− would be quantitatively utilized in other parts of the basin. One should expect a positive δ15N excursion in the upper Doushantuo Formation in some sections, which, however, has not been observed in sections studied to date (Figure 7); Second, the upper Doushantuo Formation contains abundant phosphatized fossils and phosphorite deposits (Xiao et al., 1998; Xiao et al., 2014; Liu et al., 2014; She et al., 2014; Yin et al., 2015; Zhang Y. et al., 2019), possibly indicating high dissolved P level in coeval seawater (e.g., Laakso et al., 2020). Thus, it is unlikely that P was severely limited during the Shuram excursion.
Alternatively, the isotopic shift could have been caused by a shift in the location of denitrification changes in the relative proportions of water column denitrification vs. sedimentary denitrification can affect the seawater δ15N and accordingly sedimentary δ15N (Sigman et al., 2009; Algeo et al., 2014; Stüeken et al., 2016). In the modern oceans, water column denitrification accounts for 25–32% of total denitrification and is generally accompanied by large isotope fractionation (ε ≈ δ15Nreactant - δ15Nproduct). The remaining 68–75% of denitrification occurs within sedimentary porewaters with small or negligible isotope fractionation. The balance between these two processes and N2 fixation determines the isotope composition of modern seawater (average ca. +5‰) (Sigman et al., 2009; Algeo et al., 2014; Stüeken et al., 2016). Assuming a steady isotope scenario, a high fraction of water column denitrification would thus increase the δ15N of seawater nitrate, and vice versa (Sigman et al., 2009; Algeo et al., 2014; Stüeken et al., 2016). Hence, the decrease of δ15N coupled with the Shuram excursion on the Yangtze Platform could have resulted from the decrease of the fraction of water column denitrification. This requires a more widely oxygenated ocean than today, i.e. a complete absence of oxygen minimum zones, which is not supported by any current geochemical and geological evidence. However, the nitrogen isotope mass balance can potentially also be affected by sea level. Algeo et al. (2014) compiled δ15N data from the Cryogenian to present and found a long-term decrease in δ15N from the Cryogenian to the Cambrian, which they attributed to a first-order climate-driven sea level change. According to this model, high eustatic sea level could have resulted in relatively low δ15N if the dominant locus of denitrification shifted to sediments (Algeo et al., 2014). However, this model is difficult to reconcile with the relatively stable positive δ15N values from ca. 750 Ma to 570 Ma, spanning from the Tonian through the Cryogenian to the middle Ediacaran (Ader et al., 2014). Particularly, after the deglaciation of the Marinoan Snowball Earth, which represented a global sea level rise, δ15N remains high for at least 60 Myr or even longer until the onset of the Shuram excursion (Xiang et al., 2018; Chen et al., 2019; Xu et al., 2020). Further, from the Ediacaran to early Cambrian the ocean was characterized by predominately anoxic environments with multiple short-term oxygenation events (Sahoo et al., 2016; Li et al., 2018). Under such conditions, the fraction of water column denitrification should always have been much higher than it is in more oxygenated oceans like today, even during intervals of high eustatic sea level. Therefore, changes in proportion of water column denitrification alone cannot readily explain the decrease in δ15N during the Shuram excursion.
Variation in the isotope fractionation associated with water column denitrification is another factor that can modulate seawater δ15N. According to the mass balance model for the nitrogen cycle (Sigman et al., 2009; Algeo et al., 2014; Stüeken et al., 2016), the seawater nitrate δ15N is determined by the isotopic balance between input and output processes, which can be described in the following formulation:
where εfix refers to isotopic fractionation of N2 fixation, and εden represents net isotope fractionation of total denitrification which can be expressed as:
where the subscripts wd and sd refer to water column denitrification and sedimentary denitrification, respectively, and fwd represents the fraction of water column denitrification. In modern oceans, the εwd can vary from 0‰ for quantitative denitrification to 30‰ (Sigman et al., 2009 and references therein). To model seawater nitrate δ15N as a function of εwd, we set εfix to be +1‰ and εsd to be 0‰ (Sigman et al., 2009 and references therein). If the fraction of water column denitrification was 25% during the Ediacaran as generally suggested for today (Sigman et al., 2009), the mean δ15N of pre-Shuram samples corresponds to εwd of 26–28%, and the decrease of δ15N during the Shuram excursion would require a decrease of εwd to 19–21% (Figure 8). The εwd could have been smaller if fwd increased (Figure 8). For example, εwd for the mean δ15N of the Shuram interval would be 12–13‰, if fwd increased to 40%, and it would decrease to ca. 6‰ when increasing fwd to 80%. A high fraction of fwd was possible for the Ediacaran when anoxic marine environments were more extensive than today. Modifying εsd to 3‰ as observed in some modern sediment (Kessler et al., 2014) would require a lower εwd to produce the same δ15N. This difference would be large when fwd was low but small as fwd increased (Figure 8).
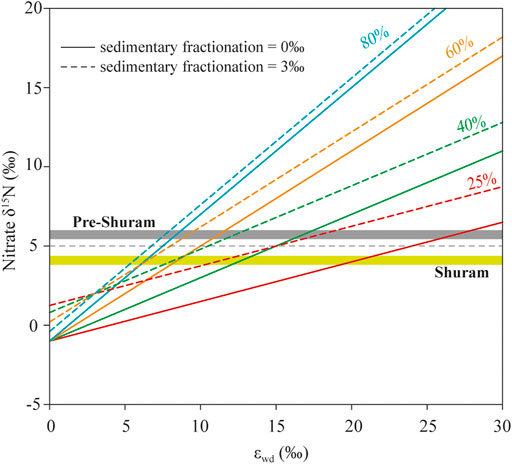
FIGURE 8. Mass balance model showing seawater nitrate δ15N as function of εwd. Colored oblique lines represent the fraction of water column denitrification (fwd) from 25% (red line) to 80% (blue line). Horizontal dash line indicates the average δ15N and modern seawater. Shaded zones show the bootstrap δ15N range (same as in Figure 7H) before and during the Shuram excursion.
As discussed above, the decrease of δ15N associated with the Shuram excursion can be well explained by the decrease of εwd. We argue that this change may have been caused by a feedback between the nitrogen and carbon cycles at that time. Enhanced continental weathering before the Shuram excursion, as evidenced by the increase of 87Sr/86Sr (Sawaki et al., 2010; Wang et al., 2014; Cui et al., 2015; Xiao et al., 2016; Lan et al., 2019), would have delivered substantial amounts of nutrients to the ocean, promoting primary productivity (Williams et al., 2019). High primary productivity would have resulted in an expansion of anoxic bottom waters on productive continental shelves. These anoxic waters would have been capped by oxic surface waters, leading to extensive aerobic respiration or organic matter along the redox interface. An ensuing consequence of this combined effect would have been the significant consumption of nitrate through enhanced denitrification. Stoichiometric relationships indicate that remineralization of 1 mol of organic carbon through denitrification would consume 85 mol or even more nitrate (Altabet, 2006). The shrinkage of the nitrate pool may have reduced εwd due to reservoir effects, as has been documented from modern microbial cultures, where εwd decreased when nitrate levels dropped to a few µM (compared to ∼30 µM in the modern open ocean) (Kritee et al., 2012). Additionally, the decrease of nitrate levels would inevitably shift the nitrogen cycle towards N2 fixation and ammonium assimilation, which may also partially contribute to the lower δ15N during the Shuram excursion, as evidenced by a near zero value observed in the Fengtan section (Nishizawa et al., 2019). In the long time scale, an increase in organic burial in the context of high primary productivity would have led to rising O2 levels (e.g., Alcott et al., 2019). This inference is consistent with the increase of I/(Ca + Mg) ratios (Hardisty et al., 2017; Wei et al., 2019), a large positive δ238U excursion (Zhang F. et al., 2019; Cao et al., 2020), and a negative excursion of thallium isotope composition (ε205Tl) (Fan et al., 2020) during the Shuram interval. The rise of oxygen would have resulted in partial oxidation of dissolved organic carbon stored in the anoxic deep ocean (Rothman et al., 2003) or of other forms of reduced carbon (e.g., Bjerrum and Canfield, 2011), lowering the δ13C of inorganic carbon in seawater, as documented in the Shuram excursion. Further, the recycled N and P from the oxidation of organic matter could have been upwelled to the photic zone, providing new nutrient input for organisms and further stimulating primary productivity. Although we cannot completely rule out the possibility of diagenetic overprint over the Shuram signal in individual cases, the coupled variations of δ13Ccarb and δ15N in multiple sections across the Yangtze Platform suggest that they may partially record changes in primary seawater signals in response to the complex feedback between carbon and nitrogen cyclings during this critical period.
Conclusion
High resolution δ15N and δ13Corg data are reported from the upper part of the Ediacaran Doushantuo Formation in two well-preserved sections in the Yangtze Gorges area, South China. These data, coupled with previously published δ13Ccarb in the same sections, are used to elucidate the inherent relationship between carbon and nitrogen cycling during the Shuram-EN3 excursion─the deepest negative δ13Ccarb excursion in Earth history. The δ15N data in the studied sections show concurrent variations with δ13Ccarb, although the magnitude of change is much smaller. Bootstrapping simulations further demonstrate a clear decrease of δ15N associated with the Shuram-EN3 excursion. We argue that the decrease in δ15N during the Shuram-EN3 excursion can be reasonably explained by the reduction of isotopic fractionation associated with water column denitrification rather than the partial assimilation of nitrate. The parallel changes in δ13Ccarb and δ15N may have resulted from feedbacks between carbon and nitrogen cycles.
Data Availability Statement
The original contributions presented in the study are included in the article/Supplementary Material, further inquiries can be directed to the corresponding author.
Author Contributions
XW and XS designed research. DX and XW collected samples. DX, XW, and YP performed lab analyses. XW, DX, XS, YP, and ES wrote the paper.
Funding
This research is supported by the National Natural Science Foundation of China (41872032, 41830215, 41930320) and the Chinese ‘111’ project (B20011).
Conflict of Interest
The authors declare that the research was conducted in the absence of any commercial or financial relationships that could be construed as a potential conflict of interest.
Acknowledgments
Haoming Wei and Lijing Wang participated part of field trip and sample collection. We are indebted to Hao Yan for the kind help with the carbon and nitrogen isotope analyses. Thanks are given to two reviewers for valuable comments that improve the quality of this paper.
Supplementary Material
The Supplementary Material for this article can be found online at: https://www.frontiersin.org/articles/10.3389/feart.2021.678149/full#supplementary-material
References
Ader, M., Macouin, M., Trindade, R. I. F., Hadrien, M.-H., Yang, Z., Sun, Z., et al. (2009). A Multilayered Water Column in the Ediacaran Yangtze Platform? Insights from Carbonate and Organic Matter Paired δ13C. Earth Planet. Sci. Lett. 288, 213–227. doi:10.1016/j.epsl.2009.09.024
Ader, M., Sansjofre, P., Halverson, G. P., Busigny, V., Trindade, R. I. F., Kunzmann, M., et al. (2014). Ocean Redox Structure across the Late Neoproterozoic Oxygenation Event: A Nitrogen Isotope Perspective. Earth Planet. Sci. Lett. 396, 1–13. doi:10.1016/j.epsl.2014.03.042
Ader, M., Thomazo, C., Sansjofre, P., Busigny, V., Papineau, D., Laffont, R., et al. (2016). Interpretation of the Nitrogen Isotopic Composition of Precambrian Sedimentary Rocks: Assumptions and Perspectives. Chem. Geol. 429, 93–110. doi:10.1016/j.chemgeo.2016.02.010
Alcott, L. J., Mills, B. J. W., and Poulton, S. W. (2019). Stepwise Earth Oxygenation Is an Inherent Property of Global Biogeochemical Cycling. Science 366, 1333–1337. doi:10.1126/science.aax6459
Algeo, T. J., Meyers, P. A., Robinson, R. S., Rowe, H., and Jiang, G. Q. (2014). Icehouse-greenhouse Variations in marine Denitrification. Biogeosciences 11, 1273–1295. doi:10.5194/bg-11-1273-2014
Altabet, M. A. (2006). “Isotopic Tracers of the Marine Nitrogen Cycle: Present and Past,” in Marine Organic Matter: Biomarkers, Isotopes and DNA. The Handbook of Environmental Chemistry, Vol 2N. Editor J.K. Volkman (Berlin, Heidelberg: Springer), 251–293. doi:10.1007/698_2_008
Altabet, M. A., Pilskaln, C., Thunell, R., Pride, C., Sigman, D., Chavez, F., et al. (1999). The Nitrogen Isotope Biogeochemistry of Sinking Particles from the Margin of the Eastern North Pacific. Deep Sea Res. Oceanographic Res. Pap. 46, 655–679. doi:10.1016/S0967-0637(98)00084-3
An, Z., Jiang, G., Tong, J., Tian, L., Ye, Q., Song, H., et al. (2015). Stratigraphic Position of the Ediacaran Miaohe Biota and its Constrains on the Age of the Upper Doushantuo δ13C Anomaly in the Yangtze Gorges Area, South China. Precambrian Res. 271, 243–253. doi:10.1016/j.precamres.2015.10.007
Bjerrum, C. J., and Canfield, D. E. (2011). Towards a Quantitative Understanding of the Late Neoproterozoic Carbon Cycle. Proc. Natl. Acad. Sci. 108, 5542–5547. doi:10.1073/pnas.1101755108
Bowring, S. A., Grotzinger, J. P., Condon, D. J., Ramezani, J., Newall, M. J., and Allen, P. A. (2007). Geochronologic Constraints on the Chronostratigraphic Framework of the Neoproterozoic Huqf Supergroup, Sultanate of Oman. Am. J. Sci. 307, 1097–1145. doi:10.2475/10.2007.01
Bristow, T. F., and Kennedy, M. J. (2008). Carbon Isotope Excursions and the Oxidant Budget of the Ediacaran Atmosphere and Ocean. Geol 36, 863–866. doi:10.1130/g24968a.1
Burns, S. J., and Matter, A. (1993). Carbon Isotopic Record of the Latest Proterozoic from Oman. Eclogae Geologicae Helv. 86, 595–607.
Canfield, D. E., Knoll, A. H., Poulton, S. W., Narbonne, G. M., and Dunning, G. R. (2020). Carbon Isotopes in Clastic Rocks and the Neoproterozoic Carbon Cycle. Am. J. Sci. 320, 97–124. doi:10.2475/02.2020.01
Cao, M., Daines, S. J., Lenton, T. M., Cui, H., Algeo, T. J., Dahl, T. W., et al. (2020). Comparison of Ediacaran platform and slope δ238U records in South China: Implications for global-ocean oxygenation and the origin of the Shuram Excursion. Geochimica et Cosmochimica Acta 287, 111–124. doi:10.1016/j.gca.2020.04.035
Chang, B., Li, C., Liu, D., Foster, I., Tripati, A., Lloyd, M. K., et al. (2020). Massive Formation of Early Diagenetic Dolomite in the Ediacaran Ocean: Constraints on the “Dolomite Problem”. Proc. Natl. Acad. Sci. USA 117, 14005–14014. doi:10.1073/pnas.1916673117
Chen, Y., Diamond, C. W., Stüeken, E. E., Cai, C., Gill, B. C., Zhang, F., et al. (2019). Coupled Evolution of Nitrogen Cycling and Redoxcline Dynamics on the Yangtze Block across the Ediacaran-Cambrian Transition. Geochimica et Cosmochimica Acta 257, 243–265. doi:10.1016/j.gca.2019.05.017
Condon, D., Zhu, M. Y., Bowring, S., Wang, W., Yang, A. H., and Jin, Y. G. (2005). U-pb Ages from the Neoproterozoic Doushantuo Formation, China. Science 308, 95–98. doi:10.1126/science.1107765
Cui, H., Kaufman, A. J., Xiao, S., Zhou, C., and Liu, X.-M. (2017). Was the Ediacaran Shuram Excursion a Globally Synchronized Early Diagenetic Event? Insights from Methane-Derived Authigenic Carbonates in the Uppermost Doushantuo Formation, South China. Chem. Geol. 450, 59–80. doi:10.1016/j.chemgeo.2016.12.010
Cui, H., Kaufman, A. J., Xiao, S., Zhu, M., Zhou, C., and Liu, X.-M. (2015). Redox Architecture of an Ediacaran Ocean Margin: Integrated Chemostratigraphic (δ13C-Δ34S-87Sr/86Sr-Ce/Ce*) Correlation of the Doushantuo Formation, South China. Chem. Geol. 405, 48–62. doi:10.1016/j.chemgeo.2015.04.009
Derry, L. A. (2010). A Burial Diagenesis Origin for the Ediacaran Shuram-Wonoka Carbon Isotope Anomaly. Earth Planet. Sci. Lett. 294, 152–162. doi:10.1016/j.epsl.2010.03.022
Devol, A. H. (2015). Denitrification, Anammox, and N2Production in Marine Sediments. Annu. Rev. Mar. Sci. 7, 403–423. doi:10.1146/annurev-marine-010213-135040
Falkowski, P. G. (1997). Evolution of the Nitrogen Cycle and its Influence on the Biological Sequestration of CO2 in the Ocean. Nature 387, 272–275. doi:10.1038/387272a0
Fan, H., Fu, X., Ward, J. F., Yin, R., Wen, H., and Feng, X. (2021). Mercury Isotopes Track the Cause of Carbon Perturbations in the Ediacaran Ocean. Geol. 49, 248–252. doi:10.1130/g48266.1
Fan, H., Nielsen, S. G., Owens, J. D., Auro, M., Shu, Y., Hardisty, D. S., et al. (2020). Constraining Oceanic Oxygenation during the Shuram Excursion in South China Using Thallium Isotopes. Geobiol. 18, 348–365. doi:10.1111/gbi.12379
Fennel, K., Follows, M., and Falkowski, P. G. (2005). The Co-evolution of the Nitrogen, Carbon and Oxygen Cycles in the Proterozoic Ocean. Am. J. Sci. 305, 526–545. doi:10.2475/ajs.305.6-8.526
Fike, D. A., Grotzinger, J. P., Pratt, L. M., and Summons, R. E. (2006). Oxidation of the Ediacaran Ocean. Nature 444, 744–747. doi:10.1038/nature05345
Freudenthal, T., Wagner, T., Wenzhöfer, F., Zabel, M., and Wefer, G. (2001). Early Diagenesis of Organic Matter from Sediments of the Eastern Subtropical Atlantic: Evidence from Stable Nitrogen and Carbon Isotopes. Geochimica et Cosmochimica Acta 65, 1795–1808. doi:10.1016/S0016-7037(01)00554-3
Furuyama, S., Kano, A., Kunimitsu, Y., Ishikawa, T., Wang, W., and Liu, X. (2017). Chemostratigraphy of the Ediacaran Basinal Setting on the Yangtze Platform, South China: Oceanographic and Diagenetic Aspects of the Carbon Isotopic Depth Gradient. Isl. Arc 26, e12196. doi:10.1111/iar.12196
Gong, Z., and Li, M. (2020). Astrochronology of the Ediacaran Shuram Carbon Isotope Excursion, Oman. Earth Planet. Sci. Lett. 547, 116462. doi:10.1016/j.epsl.2020.116462
Grotzinger, J. P., Fike, D. A., and Fischer, W. W. (2011). Enigmatic Origin of the Largest-Known Carbon Isotope Excursion in Earth's History. Nat. Geosci 4, 285–292. doi:10.1038/ngeo1138
Halverson, G. P., Wade, B. P., Hurtgen, M. T., and Barovich, K. M. (2010). Neoproterozoic Chemostratigraphy. Precambrian Res. 182, 337–350. doi:10.1016/j.precamres.2010.04.007
Hardisty, D. S., Lu, Z., Bekker, A., Diamond, C. W., Gill, B. C., Jiang, G., et al. (2017). Perspectives on Proterozoic Surface Ocean Redox from Iodine Contents in Ancient and Recent Carbonate. Earth Planet. Sci. Lett. 463, 159–170. doi:10.1016/j.epsl.2017.01.032
Higgins, M. B., Robinson, R. S., Husson, J. M., Carter, S. J., and Pearson, A. (2012). Dominant Eukaryotic export Production during Ocean Anoxic Events Reflects the Importance of Recycled NH4+. Proc. Natl. Acad. Sci. 109, 2269–2274. doi:10.1073/pnas.1104313109
Husson, J. M., Linzmeier, B. J., Kitajima, K., Ishida, A., Maloof, A. C., Schoene, B., et al. (2020). Large Isotopic Variability at the Micron-Scale in 'Shuram' Excursion Carbonates from South Australia. Earth Planet. Sci. Lett. 538, 116211. doi:10.1016/j.epsl.2020.116211
Husson, J. M., Maloof, A. C., Schoene, B., Chen, C. Y., and Higgins, J. A. (2015). Stratigraphic Expression of Earth's Deepest δ13C Excursion in the Wonoka Formation of South Australia. Am. J. Sci. 315, 1–45. doi:10.2475/01.2015.01
Jiang, G., Kaufman, A. J., Christie-Blick, N., Zhang, S., and Wu, H. (2007). Carbon Isotope Variability across the Ediacaran Yangtze Platform in South China: Implications for a Large Surface-To-Deep Ocean δ13C Gradient. Earth Planet. Sci. Lett. 261, 303–320. doi:10.1016/j.epsl.2007.07.009
Jiang, G., Shi, X., Zhang, S., Wang, Y., and Xiao, S. (2011). Stratigraphy and Paleogeography of the Ediacaran Doushantuo Formation (Ca. 635-551Ma) in South China. Gondwana Res. 19, 831–849. doi:10.1016/j.gr.2011.01.006
Jiang, G., Wang, X., Shi, X., Zhang, S., Xiao, S., and Dong, J. (2010). Organic Carbon Isotope Constraints on the Dissolved Organic Carbon (DOC) Reservoir at the Cryogenian-Ediacaran Transition. Earth Planet. Sci. Lett. 299, 159–168. doi:10.1016/j.epsl.2010.08.031
Jiang, L., Planavsky, N., Zhao, M., Liu, W., and Wang, X. (2019). Authigenic Origin for a Massive Negative Carbon Isotope Excursion. Geol. 47, 115–118. doi:10.1130/g45709.1
Kaufman, A. J., Corsetti, F. A., and Varni, M. A. (2007). The Effect of Rising Atmospheric Oxygen on Carbon and Sulfur Isotope Anomalies in the Neoproterozoic Johnnie Formation, Death Valley, USA. Chem. Geol. 237, 47–63. doi:10.1016/j.chemgeo.2006.06.023
Kessler, A. J., Bristow, L. A., Cardenas, M. B., Glud, R. N., Thamdrup, B., and Cook, P. L. M. (2014). The Isotope Effect of Denitrification in Permeable Sediments. Geochimica et Cosmochimica Acta 133, 156–167. doi:10.1016/j.gca.2014.02.029
Kikumoto, R., Tahata, M., Nishizawa, M., Sawaki, Y., Maruyama, S., Shu, D., et al. (2014). Nitrogen Isotope Chemostratigraphy of the Ediacaran and Early Cambrian Platform Sequence at Three Gorges, South China. Gondwana Res. 25, 1057–1069. doi:10.1016/j.gr.2013.06.002
Knauth, L. P., and Kennedy, M. J. (2009). The Late Precambrian Greening of the Earth. Nature 460, 728–732. doi:10.1038/nature08213
Kritee, K., Sigman, D. M., Granger, J., Ward, B. B., Jayakumar, A., and Deutsch, C. (2012). Reduced Isotope Fractionation by Denitrification under Conditions Relevant to the Ocean. Geochimica et Cosmochimica Acta 92, 243–259. doi:10.1016/j.gca.2012.05.020
Kump, L. R., and Arthur, M. A. (1999). Interpreting Carbon-Isotope Excursions: Carbonates and Organic Matter. Chem. Geol. 161, 181–198. doi:10.1016/S0009-2541(99)00086-8
Kump, L. R., Junium, C., Arthur, M. A., Brasier, A., Fallick, A., Melezhik, V., et al. (2011). Isotopic Evidence for Massive Oxidation of Organic Matter Following the Great Oxidation Event. Science 334, 1694–1696. doi:10.1126/science.1213999
Kunimitsu, Y., Setsuda, Y., Furuyama, S., Wang, W., Kano, A., et al. (2011). Ediacaran chemostratigraphy and paleoceanography at a shallow marine setting in northwestern Hunan Province, South China. Precambrian Res. 191, 194–208. doi:10.1016/j.precamres.2011.09.006
Laakso, T. A., Sperling, E. A., Johnston, D. T., and Knoll, A. H. (2020). Ediacaran Reorganization of the marine Phosphorus Cycle. Proc. Natl. Acad. Sci. USA 117, 11961–11967. doi:10.1073/pnas.1916738117
Lam, P., and Kuypers, M. M. M. (2011). Microbial Nitrogen Cycling Processes in Oxygen Minimum Zones. Annu. Rev. Mar. Sci. 3, 317–345. doi:10.1146/annurev-marine-120709-142814
Lan, Z., Sano, Y., Yahagi, T., Tanaka, K., Shirai, K., Papineau, D., et al. (2019). An Integrated Chemostratigraphic (δ13C-Δ18o-87Sr/86Sr-Δ15n) Study of the Doushantuo Formation in Western Hubei Province, South China. Precambrian Res. 320, 232–252. doi:10.1016/j.precamres.2018.10.018
Le Guerroué, E., Allen, P. A., and Cozzi, A. (2006a). Chemostratigraphic and Sedimentological Framework of the Largest Negative Carbon Isotopic Excursion in Earth History: The Neoproterozoic Shuram Formation (Nafun Group, Oman). Precambrian Res. 146, 68–92. doi:10.1016/j.precamres.2006.01.007
Le Guerroué, E., Allen, P. A., Cozzi, A., Etienne, J. L., and Fanning, M. (2006b). 50 Myr Recovery from the Largest negativeδ13C Excursion in the Ediacaran Ocean. Terra Nova 18, 147–153. doi:10.1111/j.1365-3121.2006.00674.x
Lee, C., Love, G. D., Fischer, W. W., Grotzinger, J. P., and Halverson, G. P. (2015). Marine Organic Matter Cycling during the Ediacaran Shuram Excursion. Geol. 43, 1106. doi:10.1130/g37236.1
Lehmann, M. F., Bernasconi, S. M., Barbieri, A., and McKenzie, J. A. (2002). Preservation of Organic Matter and Alteration of its Carbon and Nitrogen Isotope Composition during Simulated and In Situ Early Sedimentary Diagenesis. Geochimica et Cosmochimica Acta 66, 3573–3584. doi:10.1016/S0016-7037(02)00968-7
Li, C., Cheng, M., Zhu, M., and Lyons, T. W. (2018). Heterogeneous and Dynamic marine Shelf Oxygenation and Coupled Early Animal Evolution. Emerging Top. Life Sci. 2, 279–288. doi:10.1042/etls20170157
Li, C., Hardisty, D. S., Luo, G., Huang, J., Algeo, T. J., Cheng, M., et al. (2017). Uncovering the Spatial Heterogeneity of Ediacaran Carbon Cycling. Geobiol. 15, 211–224. doi:10.1111/gbi.12222
Li, C., Love, G. D., Lyons, T. W., Fike, D. A., Sessions, A. L., and Chu, X. (2010). A Stratified Redox Model for the Ediacaran Ocean. Science 328, 80–83. doi:10.1126/science.1182369
Ling, H.-F., Chen, X., Li, D., Wang, D., Shields-Zhou, G. A., and Zhu, M. (2013). Cerium Anomaly Variations in Ediacaran-Earliest Cambrian Carbonates from the Yangtze Gorges Area, South China: Implications for Oxygenation of Coeval Shallow Seawater. Precambrian Res. 225, 110–127. doi:10.1016/j.precamres.2011.10.011
Liu, P., Xiao, S., Yin, C., Chen, S., Zhou, C., and Li, M. (2014). Ediacaran Acanthomorphic Acritarchs and Other Microfossils from Chert Nodules of the Upper Doushantuo Formation in the Yangtze Gorges Area, South China. J. Paleontol. 88, 1–139. doi:10.1666/13-009
Lu, M., Zhu, M., Zhang, J., Shields-Zhou, G., Li, G., Zhao, F., et al. (2013). The DOUNCE Event at the Top of the Ediacaran Doushantuo Formation, South China: Broad Stratigraphic Occurrence and Non-diagenetic Origin. Precambrian Res. 225, 86–109. doi:10.1016/j.precamres.2011.10.018
Luo, G., Junium, C. K., Izon, G., Ono, S., Beukes, N. J., Algeo, T. J., et al. (2018). Nitrogen Fixation Sustained Productivity in the Wake of the Palaeoproterozoic Great Oxygenation Event. Nat. Commun. 9, 978. doi:10.1038/s41467-018-03361-2
Lyons, T. W., Reinhard, C. T., and Planavsky, N. J. (2014). The Rise of Oxygen in Earth's Early Ocean and Atmosphere. Nature 506, 307–315. doi:10.1038/nature13068
McFadden, K. A., Huang, J., Chu, X., Jiang, G., Kaufman, A. J., Zhou, C., et al. (2008). Pulsed Oxidation and Biological Evolution in the Ediacaran Doushantuo Formation. Proc. Natl. Acad. Sci. 105, 3197–3202. doi:10.1073/pnas.0708336105
Melezhik, V., Fallick, A., Pokrovsky, B. G., and Pokrovsky, B. (2005). Enigmatic Nature of Thick Sedimentary Carbonates Depleted in C beyond the Canonical Mantle Value: The Challenges to Our Understanding of the Terrestrial Carbon Cycle. Precambrian Res. 137, 131–165. doi:10.1016/j.precamres.2005.03.010
Michiels, C. C., Darchambeau, F., Roland, F. A. E., Morana, C., Llirós, M., García-Armisen, T., et al. (2017). Iron-dependent Nitrogen Cycling in a Ferruginous lake and the Nutrient Status of Proterozoic Oceans. Nat. Geosci 10, 217–221. doi:10.1038/ngeo2886
Nishizawa, M., Tsuchiya, Y., Du, W., Sawaki, Y., Matsui, Y., Wang, Y., et al. (2019). Shift in Limiting Nutrients in the Late Ediacaran-Early Cambrian marine Systems of South China. Palaeogeogr. Palaeoclimatol. Palaeoecol. 530, 281–299. doi:10.1016/j.palaeo.2019.05.036
Paulsen, T., Deering, C., Sliwinski, J., Bachmann, O., and Guillong, M. (2017). Evidence for a Spike in Mantle Carbon Outgassing during the Ediacaran Period. Nat. Geosci 10, 930–934. doi:10.1038/s41561-017-0011-6
Peng, Y., Dong, L., Ma, H., Wang, R., Lang, X., Peng, Y., et al. (2020). Surface Ocean Nitrate-Limitation in the Aftermath of Marinoan Snowball Earth: Evidence from the Ediacaran Doushantuo Formation in the Western Margin of the Yangtze Block, South China. Precambrian Res. 347, 105846. doi:10.1016/j.precamres.2020.105846
Prokopenko, M., Hammond, D., Berelson, W., Bernhard, J., Stott, L., and Douglas, R. (2006). Nitrogen Cycling in the Sediments of Santa Barbara basin and Eastern Subtropical North Pacific: Nitrogen Isotopes, Diagenesis and Possible Chemosymbiosis between Two Lithotrophs (Thioploca and Anammox)-"riding on a Glider". Earth Planet. Sci. Lett. 242, 186–204. doi:10.1016/j.epsl.2005.11.044
Quan, T. M., Wright, J. D., and Falkowski, P. G. (2013). Co-variation of Nitrogen Isotopes and Redox States through Glacial-Interglacial Cycles in the Black Sea. Geochimica Et Cosmochimica Acta 112, 305–320. doi:10.1016/j.gca.2013.02.029
Robinson, R. S., Kienast, M., Luiza Albuquerque, A., Altabet, M., Contreras, S., De Pol Holz, R., et al. (2012). A Review of Nitrogen Isotopic Alteration in marine Sediments. Paleoceanography 27, PA4203. doi:10.1029/2012pa002321
Rooney, A. D., Cantine, M. D., Bergmann, K. D., Gómez-Pérez, I., Al Baloushi, B., Boag, T. H., et al. (2020). Calibrating the Coevolution of Ediacaran Life and Environment. Proc. Natl. Acad. Sci. USA 117, 16824–16830. doi:10.1073/pnas.2002918117
Rothman, D. H., Hayes, J. M., and Summons, R. E. (2003). Dynamics of the Neoproterozoic Carbon Cycle. Pnas 100, 8124–8129. doi:10.1073/pnas.0832439100
Sahoo, S. K., Planavsky, N. J., Jiang, G., Kendall, B., Owens, J. D., Wang, X., et al. (2016). Oceanic Oxygenation Events in the Anoxic Ediacaran Ocean. Geobiol. 14, 457–468. doi:10.1111/gbi.12182
Sawaki, Y., Ohno, T., Tahata, M., Komiya, T., Hirata, T., Maruyama, S., et al. (2010). The Ediacaran Radiogenic Sr Isotope Excursion in the Doushantuo Formation in the Three Gorges Area, South China. Precambrian Res. 176, 46–64. doi:10.1016/j.precamres.2009.10.006
Schrag, D. P., Higgins, J. A., Macdonald, F. A., and Johnston, D. T. (2013). Authigenic Carbonate and the History of the Global Carbon Cycle. Science 339, 540–543. doi:10.1126/science.1229578
She, Z.-B., Strother, P., and Papineau, D. (2014). Terminal Proterozoic Cyanobacterial Blooms and Phosphogenesis Documented by the Doushantuo Granular Phosphorites II: Microbial Diversity and C Isotopes. Precambrian Res. 251, 62–79. doi:10.1016/j.precamres.2014.06.004
Shi, W., Li, C., Luo, G., Huang, J., Algeo, T. J., Jin, C., et al. (2018). Sulfur Isotope Evidence for Transient marine-shelf Oxidation during the Ediacaran Shuram Excursion. Geol. 46, 267–270. doi:10.1130/G39663.1
Shields, G. A., Mills, B. J. W., Zhu, M., Raub, T. D., Daines, S. J., and Lenton, T. M. (2019). Unique Neoproterozoic Carbon Isotope Excursions Sustained by Coupled Evaporite Dissolution and Pyrite Burial. Nat. Geosci. 12, 823–827. doi:10.1038/s41561-019-0434-3
Sigman, D. M., Casciotti, K. L., and Casciotti, K. L. (2001). “Nitrogen Isotopes in the Ocean,” in Encyclopedia of Ocean Science (Amsterdam: Elsevier), 1884–1894. doi:10.1006/rwos.2001.0172
Song, H., Jiang, G., Poulton, S. W., Wignall, P. B., Tong, J., Song, H., et al. (2017). The Onset of Widespread marine Red Beds and the Evolution of Ferruginous Oceans. Nat. Commun. 8, 399. doi:10.1038/s41467-017-00502-x
Spangenberg, J. E., Bagnoud-Velásquez, M., Boggiani, P. C., and Gaucher, C. (2014). Redox Variations and Bioproductivity in the Ediacaran: Evidence from Inorganic and Organic Geochemistry of the Corumbá Group, Brazil. Gondwana Res. 26, 1186–1207. doi:10.1016/j.gr.2013.08.014
Stüeken, E. E., Kipp, M. A., Koehler, M. C., and Buick, R. (2016). The Evolution of Earth's Biogeochemical Nitrogen Cycle. Earth-Science Rev. 160, 220–239. doi:10.1016/j.earscirev.2016.07.007
Stüeken, E. E., Zaloumis, J., Meixnerová, J., and Buick, R. (2017). Differential Metamorphic Effects on Nitrogen Isotopes in Kerogen Extracts and Bulk Rocks. Geochimica et Cosmochimica Acta 217, 80–94. doi:10.1016/j.gca.2017.08.019
Sui, Y., Huang, C., Zhang, R., Wang, Z., and Ogg, J. (2019). Astronomical Time Scale for the Middle-Upper Doushantuo Formation of Ediacaran in South China: Implications for the Duration of the Shuram/Wonoka Negative δ13C Excursion. Palaeogeogr. Palaeoclimatol. Palaeoecol. 532, 109273. doi:10.1016/j.palaeo.2019.109273
Swart, P. K., and Kennedy, M. J. (2012). Does the Global Stratigraphic Reproducibility of δ13C in Neoproterozoic Carbonates Require a marine Origin? A Pliocene-Pleistocene Comparison. Geol. 40, 87–90. doi:10.1130/g32538.1
Tyrrell, T. (1999). The Relative Influences of Nitrogen and Phosphorus on Oceanic Primary Production. Nature 400, 525–531. doi:10.1038/22941
Wang, J., and Li, Z.-X. (2003). History of Neoproterozoic Rift Basins in South China: Implications for Rodinia Break-Up. Precambrian Res. 122, 141–158. doi:10.1016/S0301-9268(02)00209-7
Wang, L. (2019). Variations of Trace Elements in the Upper Part of the Ediacaran Doushantuo Formation and Environmental Implication. Beijing: China University of Geosciences. Master Thesis (in Chinese with English abstract). doi:10.1130/abs/2019am-336558
Wang, W., Guan, C., Zhou, C., Peng, Y., Pratt, L. M., Chen, X., et al. (2017). Integrated Carbon, Sulfur, and Nitrogen Isotope Chemostratigraphy of the Ediacaran Lantian Formation in South China: Spatial Gradient, Ocean Redox Oscillation, and Fossil Distribution. Geobiol. 15, 552–571. doi:10.1111/gbi.12226
Wang, W., Zhou, C., Guan, C., Yuan, X., Chen, Z., and Wan, B. (2014). An Integrated Carbon, Oxygen, and Strontium Isotopic Studies of the Lantian Formation in South China with Implications for the Shuram Anomaly. Chem. Geol. 373, 10–26. doi:10.1016/j.chemgeo.2014.02.023
Wang, W., Zhou, C., Yuan, X., Chen, Z., and Xiao, S. (2012). A Pronounced Negative δ13C Excursion in an Ediacaran Succession of Western Yangtze Platform: A Possible Equivalent to the Shuram Event and its Implication for Chemostratigraphic Correlation in South China. Gondwana Res. 22, 1091–1101. doi:10.1016/j.gr.2012.02.017
Wang, X., Jiang, G., Shi, X., Peng, Y., and Morales, D. C. (2018). Nitrogen Isotope Constraints on the Early Ediacaran Ocean Redox Structure. Geochimica et Cosmochimica Acta 240, 220–235. doi:10.1016/j.gca.2018.08.034
Wang, X., Jiang, G., Shi, X., and Xiao, S. (2016). Paired Carbonate and Organic Carbon Isotope Variations of the Ediacaran Doushantuo Formation from an Upper Slope Section at Siduping, South China. Precambrian Res. 273, 53–66. doi:10.1016/j.precamres.2015.12.010
Wei, H., Wang, X., Shi, X., Jiang, G., Tang, D., Wang, L., et al. (2019). Iodine Content of the Carbonates from the Doushantuo Formation and Shallow Ocean Redox Change on the Ediacaran Yangtze Platform, South China. Precambrian Res. 322, 160–169. doi:10.1016/j.precamres.2019.01.007
Wei, W., Frei, R., Gilleaudeau, G. J., Li, D., Wei, G.-Y., Chen, X., et al. (2018). Oxygenation Variations in the Atmosphere and Shallow Seawaters of the Yangtze Platform during the Ediacaran Period: Clues from Cr-Isotope and Ce-Anomaly in Carbonates. Precambrian Res. 313, 78–90. doi:10.1016/j.precamres.2018.05.009
Williams, J. J., Mills, B. J. W., and Lenton, T. M. (2019). A Tectonically Driven Ediacaran Oxygenation Event. Nat. Commun. 10, 2690. doi:10.1038/s41467-019-10286-x
Xiang, L., Schoepfer, S. D., Zhang, H., Cao, C.-q., and Shen, S.-z. (2018). Evolution of Primary Producers and Productivity across the Ediacaran-Cambrian Transition. Precambrian Res. 313, 68–77. doi:10.1016/j.precamres.2018.05.023
Xiao, S., Bykova, N., Kovalick, A., and Gill, B. C. (2017). Stable Carbon Isotopes of Sedimentary Kerogens and Carbonaceous Macrofossils from the Ediacaran Miaohe Member in South China: Implications for Stratigraphic Correlation and Sources of Sedimentary Organic Carbon. Precambrian Res. 302, 171–179. doi:10.1016/j.precamres.2017.10.006
Xiao, S., Narbonne, G. M., Zhou, C., Laflamme, M., Grazhdankin, D. V., Moczydlowska-Vidal, M., et al. (2016). Towards an Ediacaran Time Scale: Problems, Protocols, and Prospects. Episodes 39, 540–555. doi:10.18814/epiiugs/2016/v39i4/103886
Xiao, S., Zhang, Y., and Knoll, A. H. (1998). Three-dimensional Preservation of Algae and Animal Embryos in a Neoproterozoic Phosphorite. Nature 391, 553–558. doi:10.1038/35318
Xiao, S., Zhou, C., Liu, P., Wang, D., and Yuan, X. (2014). Phosphatized Acanthomorphic Acritarchs and Related Microfossils from the Ediacaran Doushantuo Formation at Weng'an (South China) and Their Implications for Biostratigraphic Correlation. J. Paleontol. 88, 1–67. doi:10.1666/12-157r
Xu, D., Wang, X., Shi, X., Tang, D., Zhao, X., Feng, L., et al. (2020). Nitrogen Cycle Perturbations Linked to Metazoan Diversification during the Early Cambrian. Palaeogeogr. Palaeoclimatol. Palaeoecol. 538, 109392. doi:10.1016/j.palaeo.2019.109392
Yin, Z., Zhu, M., Davidson, E. H., Bottjer, D. J., Zhao, F., and Tafforeau, P. (2015). Sponge Grade Body Fossil with Cellular Resolution Dating 60 Myr before the Cambrian. Proc. Natl. Acad. Sci. USA 112, E1453–E1460. doi:10.1073/pnas.1414577112
Zerkle, A. L., Poulton, S. W., Newton, R. J., Mettam, C., Claire, M. W., Bekker, A., et al. (2017). Onset of the Aerobic Nitrogen Cycle during the Great Oxidation Event. Nature 542, 465–467. doi:10.1038/nature20826
Zhang, F., Xiao, S., Romaniello, S. J., Hardisty, D., Li, C., Melezhik, V., et al. (2019a). Global marine Redox Changes Drove the Rise and Fall of the Ediacara Biota. Geobiol. 17, 594–610. doi:10.1111/gbi.12359
Zhang, S., Jiang, G., Zhang, J., Song, B., Kennedy, M. J., and Christie-Blick, N. (2005). U-pb Sensitive High-Resolution Ion Microprobe Ages from the Doushantuo Formation in south China: Constraints on Late Neoproterozoic Glaciations. Geol 33, 473–476. doi:10.1130/g21418.1
Zhang, Y., Pufahl, P. K., Du, Y., Chen, G., Liu, J., Chen, Q., et al. (2019b). Economic Phosphorite from the Ediacaran Doushantuo Formation, South China, and the Neoproterozoic-Cambrian Phosphogenic Event. Sediment. Geol. 388, 1–19. doi:10.1016/j.sedgeo.2019.05.004
Zhou, C., Jiang, S., Xiao, S., Chen, Z., and Yuan, X. (2012). Rare Earth Elements and Carbon Isotope Geochemistry of the Doushantuo Formation in South China: Implication for Middle Ediacaran Shallow marine Redox Conditions. Chin. Sci. Bull. 57, 1998–2006. doi:10.1007/s11434-012-5082-6
Zhou, C., and Xiao, S. (2007). Ediacaran δ13C Chemostratigraphy of South China. Chem. Geol. 237, 89–108. doi:10.1016/j.chemgeo.2006.06.021
Zhou, C., Xiao, S., Wang, W., Guan, C., Ouyang, Q., and Chen, Z. (2017). The Stratigraphic Complexity of the Middle Ediacaran Carbon Isotopic Record in the Yangtze Gorges Area, South China, and its Implications for the Age and Chemostratigraphic Significance of the Shuram Excursion. Precambrian Res. 288, 23–38. doi:10.1016/j.precamres.2016.11.007
Zhou, C., Yuan, X., Xiao, S., Chen, Z., and Hua, H. (2019). Ediacaran Integrative Stratigraphy and Timescale of China. Sci. China Earth Sci. 62, 7–24. doi:10.1007/s11430-017-9216-2
Zhu, M., Lu, M., Zhang, J., Zhao, F., Li, G., Aihua, Y., et al. (2013). Carbon Isotope Chemostratigraphy and Sedimentary Facies Evolution of the Ediacaran Doushantuo Formation in Western Hubei, South China. Precambrian Res. 225, 7–28. doi:10.1016/j.precamres.2011.07.019
Keywords: Ediacaran, South China, Doushantuo Formation, Shuram excursion, nitrogen isotopes
Citation: Xu D, Wang X, Shi X, Peng Y and Stüeken EE (2021) Feedback Between Carbon and Nitrogen Cycles During the Ediacaran Shuram Excursion. Front. Earth Sci. 9:678149. doi: 10.3389/feart.2021.678149
Received: 09 March 2021; Accepted: 11 May 2021;
Published: 25 May 2021.
Edited by:
Jon Telling, Newcastle University, United KingdomReviewed by:
Qing Li, Qingdao Institute of Marine Geology (QIMG), ChinaChristophe Thomazo, Université de Bourgogne, France
Copyright © 2021 Xu, Wang, Shi, Peng and Stüeken. This is an open-access article distributed under the terms of the Creative Commons Attribution License (CC BY). The use, distribution or reproduction in other forums is permitted, provided the original author(s) and the copyright owner(s) are credited and that the original publication in this journal is cited, in accordance with accepted academic practice. No use, distribution or reproduction is permitted which does not comply with these terms.
*Correspondence: Xinqiang Wang, d3hxaWFuZzMwN0AxMjYuY29t