- 1Centro de Informação e Vigilância Sismovulcânica dos Açores (CIVISA), Ponta Delgada, Portugal
- 2Instituto de Investigação em Vulcanologia e Avaliação de Riscos (IVAR), Universidade dos Açores, Ponta Delgada, Portugal
- 3Department of Earth and Planetary Science, University of California, Berkeley, CA, United States
- 4School of Geography, Geology and the Environment, Keele University, Keele, United Kingdom
Ignimbrites are relatively uncommon on ocean island volcanoes and yet they constitute a significant portion of the stratigraphy of Terceira Island (Azores). The Lajes-Angra Ignimbrite Formation (ca. 25 cal ka BP) contains the youngest ignimbrites on Terceira and records two ignimbrite-forming eruptions of Pico Alto volcano that occurred closely spaced in time. Here, we present the first detailed lithofacies analysis and architecture of the Angra and Lajes ignimbrites, complemented by petrographic, mineral chemical, whole rock and groundmass glass geochemical data. The two ignimbrites have the same comenditic trachyte composition, but show considerable variability in trace element and groundmass glass compositions, revealing complex petrogenetic processes in the Pico Alto magma reservoir prior to eruption. The Angra Ignimbrite has a high-aspect ratio and is massive throughout its thickness. It was formed by a small-volume but sustained pyroclastic density current (PDC) fed by a short-lived, low pyroclastic fountain. Overall, the PDC had high particle concentration, granular fluid-based flow conditions and was mostly channelled into a valley on the south part of Terceira. By contrast, the Lajes Ignimbrite has a low-aspect ratio and shows vertical and lateral lithofacies variations. It was formed by a sustained quasi-steady PDC generated from vigorous and prolonged pyroclastic fountaining. The ignimbrite architecture reveals that depositional conditions of the parent PDC evolved as the eruption waxed. The dilute front of the current rapidly changed to a high particle concentration, granular fluid-based PDC that extended to the north and south coasts, with limited capacity to surmount topographic highs. Contrary to what is commonly assumed, the low-aspect ratio of the Lajes Ignimbrite is interpreted to result from deposition of a relatively low velocity PDC over a generally flat topography. This work highlights that the geometry (aspect ratio) of ignimbrites does not necessarily reflect the kinetic energy of PDCs and thus should not be used as a proxy for PDC emplacement dynamics. Although the probability of an ignimbrite-forming eruption on Terceira is relatively low, such a scenario should not be underestimated, as a future event would have devastating consequences for the island’s 55,000 inhabitants.
Introduction
Ignimbrites are formed by sedimentation of hot mixtures of ash and vesiculated juvenile (pumiceous) clasts from pyroclastic density currents (PDCs) generated during explosive volcanic eruptions (Sparks et al., 1973; Cas and Wright, 1987; Branney and Kokelaar, 2002). Ignimbrite-forming events can result from a wide range of eruption styles, from caldera-forming eruptions to short-lived explosions, and occur in different geodynamic settings (e.g., Freundt and Schmincke, 1986; Lipman, 1997; Giordano, 1998; Giordano et al., 2002; Sulpizio et al., 2007; Sulpizio et al., 2010; Cas et al., 2011; Pensa et al., 2015; Pimentel et al., 2015; Scarpati et al., 2020).
Although relatively uncommon on ocean island volcanoes, ignimbrites are known to occur in Iceland (Jørgensen, 1980), the Canary Islands (Gran Canaria and Tenerife; Bogaard and Schmincke, 1998; Brown et al., 2003), Cape Verde (Santo Antão Island; Eisele et al., 2015), and have also been reported on the island of Hawaii (Shea and Owen, 2016). In the Azores archipelago, ignimbrites are found on at least four islands: São Miguel, Terceira, Graciosa, and Faial (Self, 1976; Gaspar, 1996; Duncan et al., 1999; Gertisser et al., 2010; Pensa et al., 2015; Pimentel et al., 2015). Ignimbrites on Terceira constitute a significant portion of the island’s geological record, including at least seven major formations containing peralkaline ignimbrites dated between ca. 86 ka and ca. 25 cal ka BP (Self, 1974; Gertisser et al., 2010; Jeffery et al., 2017).
The reconstruction of ignimbrite-forming eruptions on islands is challenging, as the deposits are often incompletely preserved on account of the small area of deposition, the typical rough topography, and the high erosion rates on volcanic islands. Small oceanic islands are particularly vulnerable to explosive eruptions and the risk is potentially aggravated by their geographical isolation and the weak socio-economic conditions of local communities. Therefore, detailed studies are needed to widen our understanding of ignimbrite-forming eruptions on oceanic islands and their potential impacts. Here, we focus on the youngest ignimbrite formation from Terceira, the Lajes-Angra Ignimbrite Formation (ca. 25 cal ka BP), hereafter abbreviated as LAI following Gertisser et al. (2010). The LAI is composed of two closely related ignimbrites (Lajes and Angra members) originating from Pico Alto volcano that together extend over nearly two-thirds of the island (Self, 1974; Self, 1976; Gertisser et al., 2010). Although closely related, the two ignimbrites contrast strongly in their spatial distribution, thickness and architecture.
In this paper, we use lithofacies analysis and ignimbrite architecture coupled with petrography, mineral chemistry, and whole rock and glass compositions to provide insights into the pre-eruptive magmatic system, and to infer the eruption style, transport and depositional processes of the parent PDCs of Angra and Lajes ignimbrites. We also reassess the use of aspect ratio as a proxy for emplacement dynamics of PDCs. The insights gained from this study are fundamental in improving our knowledge of the future eruptive behaviour of Pico Alto volcano and have significant implications for the assessment of volcanic hazard on Terceira and on other small islands prone to this type of volcanic activity.
Ignimbrite Geometry and Pyroclastic Density Current Emplacement Dynamics
Ignimbrite geometry, or aspect ratio (Walker et al., 1980; Walker, 1983), is one of the most common criteria used to classify ignimbrites. The aspect ratio is determined as the ratio of the average thickness of the deposit (H) to the diameter of a circle with an equal area to that covered by the ignimbrite (L). Accordingly, ignimbrites are subdivided into two endmembers: high-aspect ratio ignimbrites (HARIs) and low-aspect ratio ignimbrites (LARIs). The aspect ratio of ignimbrites has also been widely used as a proxy for PDC emplacement dynamics (i.e., as an estimate of kinetic energy, and hence velocity and competence).
HARIs are characteristically thick when compared to their horizontal extent (H/L 10–2–10–3) and are usually confined to valleys or topographic depressions. Well known examples include the 0.76 Ma Bishop Tuff Ignimbrite, California (Wilson and Hildreth, 1997), the AD 1912 Valley of Ten Thousand Smokes Ignimbrite, Alaska (Hildreth and Fierstein, 2012), and the ignimbrite of the AD 1991 eruption of Mt. Pinatubo, Philippines (Scott et al., 1996). HARIs are thought to result from deposition of low energy PDCs (e.g., Walker, 1983; Druitt, 1998; Cas et al., 2011).
By contrast, LARIs are relatively thin and widespread deposits (H/L 10–4–10–5) that mantle the landscape with a rather uniform thickness, including veneers on topographic highs. Well studied examples include the 2.08 Ma Cerro Galán Ignimbrite, Argentina (Cas et al., 2011), the 45 ka Green Tuff Ignimbrite, Pantelleria (Williams et al., 2014), and the AD 186 Taupo Ignimbrite, New Zealand (Wilson, 1985). LARIs are often interpreted to result from deposition by highly energetic PDCs that are able to surmount topographic barriers by momentum rather than mere inundation (e.g., Walker et al., 1980; Walker, 1983; Druitt, 1998; Dade, 2003; Cas et al., 2011).
However, the aspect ratio as a simple geometric relationship does not correlate with lithofacies variations observed in ignimbrites (Giordano and Doronzo, 2017), which mainly reflect flow-boundary zone conditions in PDCs (Branney and Kokelaar, 2002). Therefore, it can be misleading to directly derive fundamental differences in emplacement dynamics of PDCs from the geometry of the deposits. Study of ignimbrite lithofacies and architecture is thus of paramount importance for better understanding of the temporal and spatial evolution of PDCs.
Geological Setting and Evolution of Terceira Island
The Azores archipelago consists of nine volcanic islands spread over 600 km in the Atlantic Ocean that sit astride the triple junction of the North American, Eurasian and Nubian lithospheric plates (Figure 1 inset). Terceira Island comprises four overlapping central volcanoes: Cinco Picos, Guilherme Moniz, Pico Alto, and Santa Bárbara; as well as a fissure zone (Figure 1A; Self, 1974; Self, 1976; Madeira, 2005).
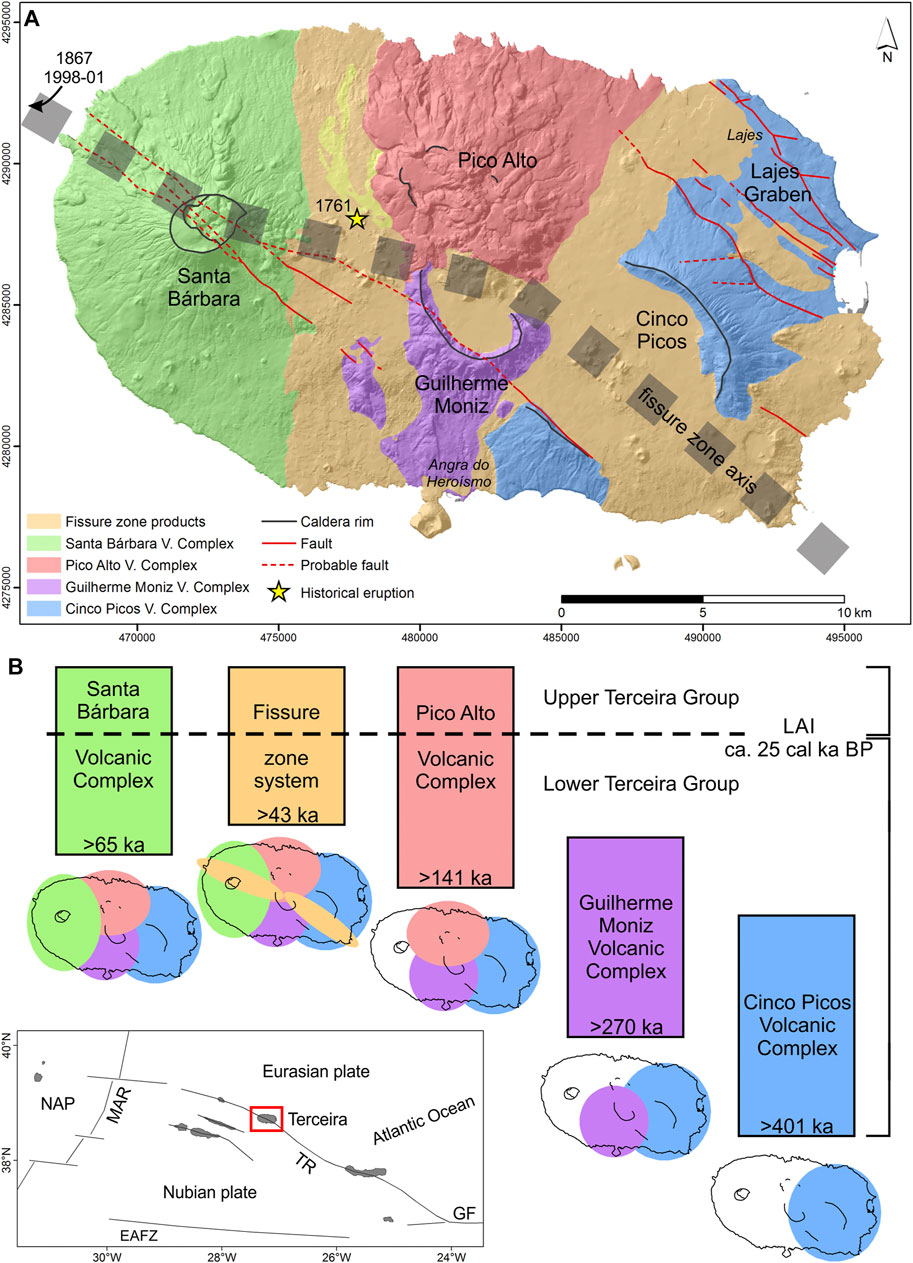
FIGURE 1. (A) Simplified geological map of Terceira Island (modified from Madeira, 2005); UTM coordinates, zone 26S. (B) Stratigraphic scheme and reconstruction of the different phases of evolution of Terceira Island (ages from Calvert et al., 2006; Gertisser et al., 2010; Hildenbrand et al., 2014); LAI—Lajes-Angra Ignimbrite Formation. Inset shows the geodynamic setting of the Azores archipelago and main structural features of the region (NAP—North American plate; MAR—Mid-Atlantic Ridge; TR—Terceira Rift; EAFZ—East Azores Fracture Zone; GF—Gloria Fault).
Cinco Picos volcano forms the eastern third of Terceira (Figure 1A) and is dominated by a 7 km-wide caldera that is strongly eroded and buried by products of neighbouring volcanoes. The caldera floor is covered by basaltic lavas and scoria cones of the fissure zone. The products of the Cinco Picos Volcanic Complex are mostly hawaiitic to mugearitic lavas, with minor exposures of comenditic trachyte lavas and pyroclastic deposits (Self, 1974; Self, 1976; Self and Gunn, 1976; Madeira, 2005; Gertisser et al., 2010). The history of this extinct volcano is as poorly known as it is exposed; Cinco Picos is the oldest edifice of the island (dated at 401 ± 6 ka; Hildenbrand et al., 2014), its eruptive activity started in the Pleistocene and the caldera was formed less than 280 ka ago (Féraud et al., 1980). The NE flank of Cinco Picos is deeply dissected by the NW-SE-trending fault scarps of the Lajes Graben (Figure 1A).
Guilherme Moniz volcano, in the centre of the island, is characterized by an elliptical caldera with a general NW-SE elongation (Figure 1A), marked by near-vertical walls in the south, while the northern sector is almost entirely buried by recent lava domes and coulées from Pico Alto (Self, 1974; Self, 1976; Madeira, 2005; Gertisser et al., 2010). The floor of the caldera is filled by basaltic lavas of the fissure zone. Guilherme Moniz Volcanic Complex shows a wide range of products from hawaiitic lavas to comenditic coulées and ignimbrites (Self, 1974; Self, 1976; Self and Gunn, 1976; Gertisser et al., 2010). The eruptive activity of this extinct volcano started more than 270 ka and ceased sometime after 111 ka (Calvert et al., 2006).
Pico Alto volcano, located north of Guilherme Moniz, is characterized by a cluster of lava domes and coulées that completely fill and overflow a caldera (Figure 1A). The Pico Alto Volcanic Complex comprises thick pyroclastic formations, including comenditic trachyte ignimbrites, comenditic-pantelleritic trachyte lavas and pumice fall deposits (Self, 1974; Self, 1976; Self and Gunn, 1976; Mungall and Martin, 1995; Pimentel, 2006; Gertisser et al., 2010). The eruptive history of Pico Alto started more than 141 ka ago (Gertisser et al., 2010) and shows evidence of several violent eruptions. The last major ignimbrite-forming period is dated at ca. 25 cal ka BP and is recorded by the LAI. Since then, the volcano has produced many domes and coulées, often preceded by pumice fall deposits (Self, 1974; Self, 1976; Calvert et al., 2006; Pimentel, 2006).
Santa Bárbara volcano occupies the western third of the island (Figure 1A) and is a conical-shape edifice truncated by two small overlapping calderas. The 2 km-wide inner caldera is filled by lava domes and is nested within an older caldera. The slopes of the volcano show several alignments of lava domes and coulées (Self, 1974; Self, 1976; Madeira, 2005; Pimentel, 2006). The Santa Bárbara Volcanic Complex includes a broad range of products, from basaltic-hawaiitic lavas and pyroclastic deposits to comenditic lava domes and coulees with associated pumice fall deposits (Self, 1974; Self, 1976; Self and Gunn, 1976; Mungall and Martin, 1995; Pimentel, 2006). Santa Bárbara is the youngest central volcano on Terceira (dated at 65 ± 13 ka; Hildenbrand et al., 2014); its eruptive activity started during the Late Pleistocene and has continued until the present (Self, 1974; Self, 1976; Calvert et al., 2006).
The fissure zone intersects Terceira along a general WNW-ESE to NW-SE orientation (Figure 1A). This volcanic system is characterized by a 2 km-wide diffuse alignment of vents (scoria cones, spatter cones and eruptive fissures) best seen in the central part of the island, which has produced basaltic-hawaiitic lava flows (Self, 1974; Self, 1976; Self and Gunn, 1976; Mungall and Martin, 1995; Zanon and Pimentel, 2015; Pimentel et al., 2016). Fissure volcanism extends offshore for several kilometres forming submarine ridges along the flanks of the island (Chiocci et al., 2013; Casalbore et al., 2015). The age of the fissure zone is poorly constrained to more than 43 ka (Calvert et al., 2006) in the SE segment but it is probably as old as the island itself, spanning from the Pleistocene to present. Three historical eruptions are known to have occurred: one paired subaerial eruption in the centre of the island in 1761 (Pimentel et al., 2016) and two submarine eruptions along the Serreta Ridge in 1867 and 1998–2001 (Weston, 1964; Gaspar et al., 2003; Casas et al., 2018).
The stratigraphy of Terceira is divided into two main groups (Figure 1B), according to the scheme of Self (1974): the Lower Terceira Group (LTG) and the Upper Terceira Group (UTG). These are separated by the LAI which forms a widespread lithostratigraphic horizon across much of the island. The UTG is formed by the products of at least 116 intercalated eruptions of Pico Alto, Santa Bárbara, and the fissure zone (Self, 1974; Self, 1976).
Overview of the Lajes-Angra Ignimbrite Formation
The LAI is the youngest of at least seven ignimbrite-bearing formations that fall into a relatively narrow period of activity between ca. 86 ka and ca. 25 cal ka BP ago (Figure 2). It is thought that most of the ignimbrite-forming events were associated with caldera-forming (or caldera-enlarging) phases of Pico Alto and, possibly, Guilherme Moniz volcanoes (Gertisser et al., 2010).
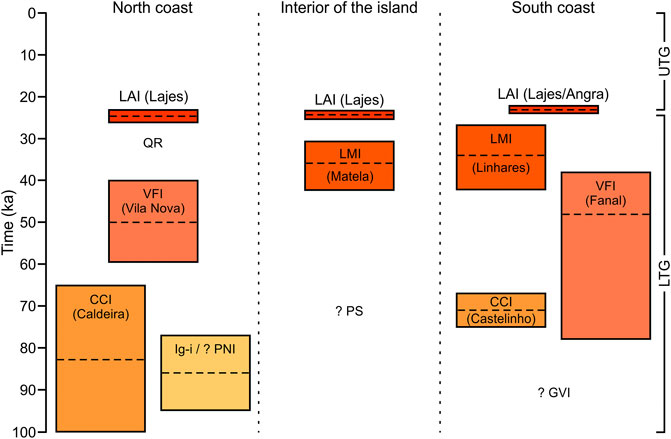
FIGURE 2. Correlation of ignimbrite formations on Terceira, showing average ages and maximum errors for formations found on the north and south coasts and in the interior of the island. Ignimbrite members are given in parenthesis. Abbreviations: LAI—Lajes-Angra Ignimbrite; LMI—Linhares- Matela Ignimbrite; VFI—Vila Nova-Fanal Ignimbrite; CCI—Caldeira-Castelinho Ignimbrite; GVI—Grota do Vale Ignimbrite; PS—Posto Santo spatter-flow deposit; QR—Quatro Ribeiras pyroclastic flow deposit; Ig-i—Ignimbrite i; PNI—Pedras Negras Ignimbrite (adapted from Gertisser et al., 2010).
All ignimbrite-bearing formations pre-dating the LAI are part of the LTG. Most are named according to the type locations of the members found on the north and south coasts of the island (Figure 2). These formations are bounded by major unconformities and have been interpreted to result from single eruptions or, more often, from a few events closely spaced in time (Gertisser et al., 2010). Most of the older ignimbrites on Terceira share similarities with the LAI, including mildly peralkaline compositions, lithofacies, and internal architecture, suggesting that the magmatic and eruptive processes involved in ignimbrite-forming eruptions have been recurrent over the last ca. 100 kyr (Gertisser et al., 2010; Jeffery et al., 2017).
The LAI presently crops out along the north and south coasts, and in a few localities in the interior of the island, but originally may have covered nearly two-thirds of Terceira (Self, 1974; Self, 1976; Gertisser et al., 2010). The LAI consists of two members: the Angra Ignimbrite is named after the city of Angra do Heroísmo (in the south of the island, see Figure 1 for location) where thick ignimbrite exposures are found; the Lajes Ignimbrite is named after the village of Lajes (in the NE sector of the island, see Figure 1 for location) where it is best represented and was initially described. The Lajes Ignimbrite was one of the first examples of low-aspect ratio ignimbrites described in the literature (Self, 1971, followed by Walker et al., 1980).
The two ignimbrites were initially interpreted by Self (1974), Self (1976) as resulting from two eruptions of Pico Alto volcano that occurred approximately 4 kyr apart. This interpretation was based on three radiocarbon ages (Shotton and Williams, 1973; Shotton et al., 1974) and a quarry outcrop in the centre of the island, where the Lajes Ignimbrite was interpreted possibly overlying the Angra Ignimbrite (Self, 1971). However, in the light of present-day knowledge of Terceira ignimbrite stratigraphy we now think that the lower unit of this outcrop was not the Angra Ignimbrite but an older ignimbrite (possibly the Linhares-Matela Ignimbrite; Gertisser et al., 2010). Unfortunately, this key exposure has been removed by subsequent quarrying.
Radiocarbon dates from recent studies (Calvert et al., 2006; Gertisser et al., 2010), calibrated with the IntCal20 calibration curve (Reimer et al., 2020), closed the age gap between the Angra and Lajes ignimbrites: Angra Ignimbrite ages range from 25.3–25.8 cal ka BP (2σ) to 24.9–28.1 cal ka BP (2σ) (Gertisser et al., 2010); Lajes Ignimbrite ages range from 23.0–25.3 cal ka BP (2σ) to 26.0–28.8 cal ka BP (2σ) (Gertisser et al., 2010). High precision dating provided ages of 25.2–25.7 cal ka BP (2σ) and 25.5–25.9 cal ka BP (2σ) for the Lajes Ignimbrite (Calvert et al., 2006). As these ages overlap within uncertainty, we infer that both ignimbrites were erupted approximately at the same time ca. 25 cal ka BP ago. Available age data for the Angra and Lajes ignimbrites are provided in Supplementary Material S1.
Field and Laboratory Methods
The LAI was studied in detail during field surveys, which combined geological mapping and lithofacies analysis. In total 83 stratigraphic sections were reconstructed throughout the island; each stratigraphic section was thoroughly described, measured and photographed. Maximum clast size (juveniles and lithics) was determined by averaging the length of the longest axis of the three largest clasts. The presence of major unconformities, including palaeosols and erosion surfaces, was used to delimit the deposits.
Lithofacies were described by non-genetic terms, based upon the lithological characteristics of the deposits (e.g., internal structures, grain size, sorting, component variations and intensity of welding). Nomenclature and abbreviations of lithofacies (Table 1) followed the schemes of Branney and Kokelaar (2002) and Sulpizio et al. (2007). Terminology used for the description of bed thickness, grain size, and sorting was adopted from Sohn and Chough (1989).
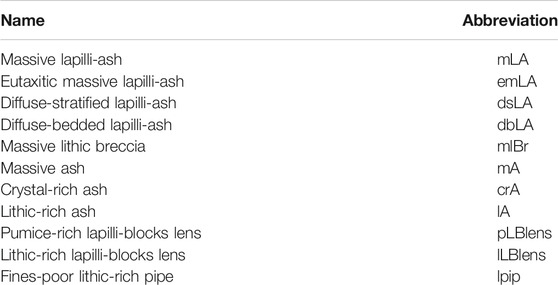
TABLE 1. Lithofacies nomenclature and abbreviation (modified from Branney and Kokelaar 2002; Sulpizio et al., 2007).
Fifteen samples of fresh juvenile clasts were collected for petrographic, whole rock, glass and mineral chemistry analyses. Petrographic descriptions were performed by thin-section observation under an Olympus BX51 binocular microscope. Modal counting was carried out with a CPX-Solutions MicroStepper ID818 point-counter stage, counting at least 1,000 points on each thin-section.
Whole rock major and trace element analyses were undertaken at Actlabs (Activation Laboratories Ltd, Canada), using inductively coupled plasma optical emission spectroscopy (ICP-OES) and inductively coupled plasma mass spectrometry (ICP-MS). Major element compositions of groundmass glass and mineral phases were determined at the Dipartimento di Scienze della Terra “Ardito Desio”, Università degli Studi di Milano (Italy) using a JEOL JXA 8200 Superprobe equipped with five wavelength-dispersive spectrometers, an energy-dispersive detector and cathodoluminescence system. Further details on analytical methods are presented in Supplementary Material S2. Full whole rock and groundmass glass data are given in Supplementary Material S3.
Ignimbrite Distribution
The spatial distribution of the Angra and Lajes ignimbrites was reconstructed combining direct field measurements with geological interpretation, accounting for the inferred palaeotopography and distance from source (following the method of Wilson, 1991). The Angra Ignimbrite crops out only in the southern part of the island, north of Angra do Heroísmo, where it reaches up to 14 m thickness, and along the coastal cliffs east of the city. Its distribution is constrained to one valley on the southern flank of Guilherme Moniz volcano (Figure 3) and the reconstructed extent occupies an area of approximately 10 km2.
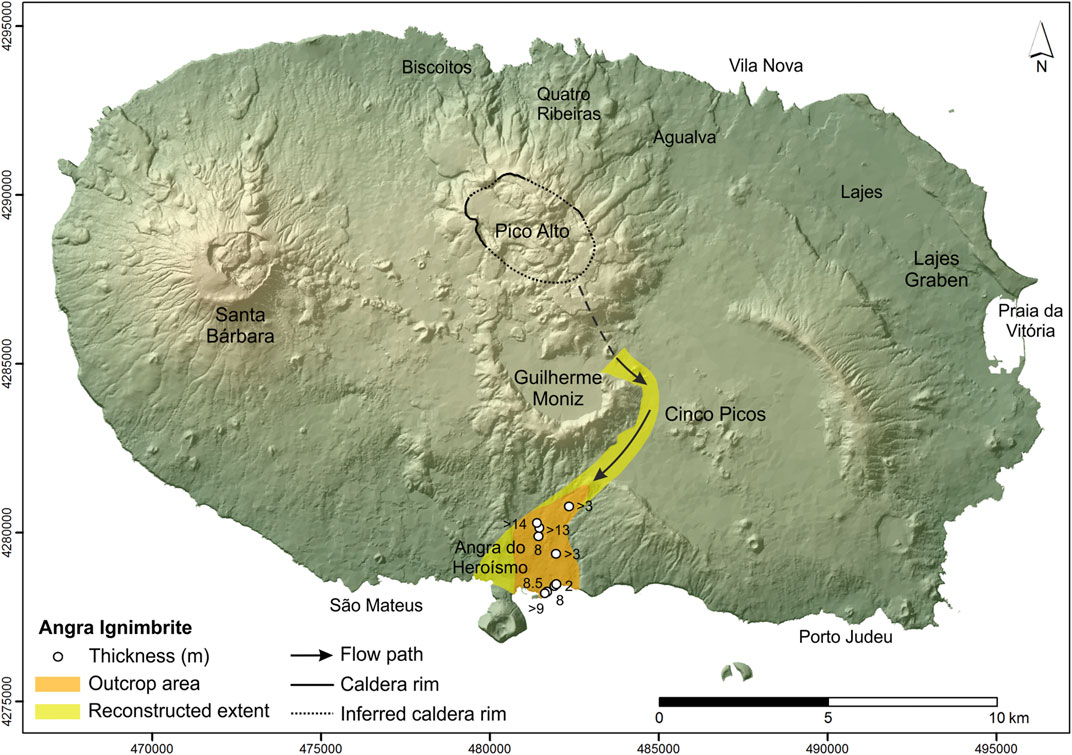
FIGURE 3. Distribution map of the Angra Ignimbrite, including documented stratigraphic sections (white circles). Arrows indicate main flow paths; Locality names referred throughout the text are shown; UTM coordinates, zone 26S.
The Lajes Ignimbrite crops out on the northern part of the island, between Biscoitos and Lajes villages, and on the southern part at São Mateus and Porto Judeu villages (Figure 4). It is best exposed on low-lying areas between Agualva and Lajes villages, where thick ignimbrite (up to 10 m in thickness) is ubiquitously found along the coastal cliffs. In the NE sector of the island, the floor of the Lajes Graben is largely filled by the Lajes Ignimbrite. North of Porto Judeu the outcrops extend into Cinco Picos caldera following a stream valley. Other minor patches of the ignimbrite crop out in the middle of the island in active or abandoned quarries. The reconstructed extent of the Lajes Ignimbrite occupies an area of at least 168 km2.
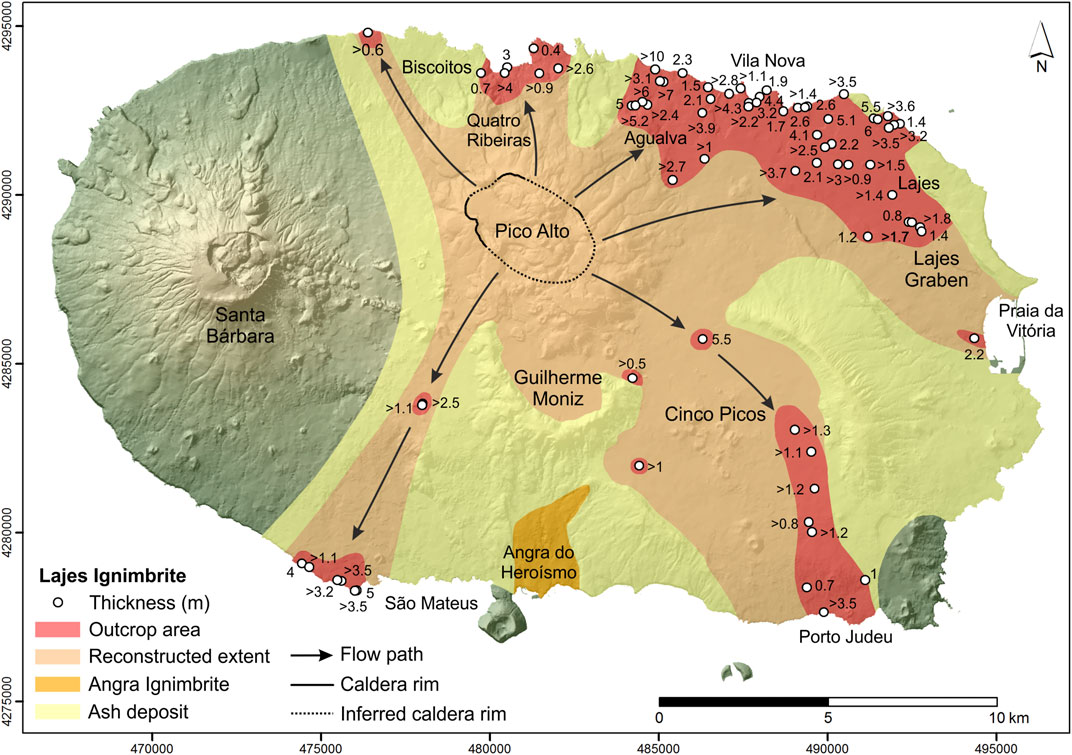
FIGURE 4. Distribution map of the Lajes Ignimbrite, including documented stratigraphic sections (white circles). Arrows indicate the main flow paths; Locality names referred throughout the text are shown; UTM coordinates, zone 26S.
Remnants of a fine ash deposit, commonly weathered into orange-brown soil, crop out over large areas of Terceira (Figure 4) at the same stratigraphic position of the Angra and Lajes ignimbrites. In places, the top of the ignimbrites can be traced to this ash deposit. The ash deposit occupies a much wider area than that of the ignimbrites and can be found covering topographic highs with a relatively uniform thickness (<50 cm). Where the ignimbrites are not present, this ash deposit forms a valuable lithostratigraphic marker horizon.
Overall, the LAI, including the associated ash deposit, extends radially outward from Pico Alto caldera over most of the island, except for the western third (Figure 4). It is estimated that the deposits of this ignimbrite formation occupy a minimum area on land of 284 km2 (i.e., >70% of the surface area of Terceira).
Ignimbrite Lithofacies
Massive Lapilli-Ash
Description: Massive lapilli-ash (mLA) is the most common lithofacies and typically constitutes the main part of the Angra and Lajes ignimbrites. It is matrix-supported, ranging from well sorted to very poorly sorted (Angra 1.5 < σϕ < 4.1 vs. Lajes 2.7 < σϕ < 5.0; Self, 1974), with variable proportions of juvenile and lithic clasts in a light grey to dark grey matrix of vitric ash and abundant feldspar crystals (up to 3 mm in length) (Figures 5A,B). Juvenile clasts are rounded to subrounded, ranging from light grey pumice to dark grey/black scoria; black dense clasts are only found in the Lajes Ignimbrite. The juvenile clasts are usually coarsely porphyritic with feldspar crystals up to 5 mm in length (see detailed description of juvenile clasts in section “Petrography and mineral chemistry”). Lapilli-sized clasts are predominant but maximum clast sizes of 20–25 cm are common and locally blocks can reach up to 80 cm. Lithic clasts are subordinate, angular to subrounded and comprise basaltic and trachytic lavas, hydrothermally altered clasts, and syenite xenoliths, in decreasing order of abundance. The lithics are typically <10 cm in size but in places blocks reach up 65 cm. Massive lapilli-ash deposits lack internal stratification, although locally framework-supported pumice-rich or lithic-rich lapilli-blocks lenses (pLBlens, lLBlens) are recognized; fines-poor lithic-rich pipes (lpip) are rare. This lithofacies is non-welded, but is commonly lithified, and contains abundant carbonized wood fragments.
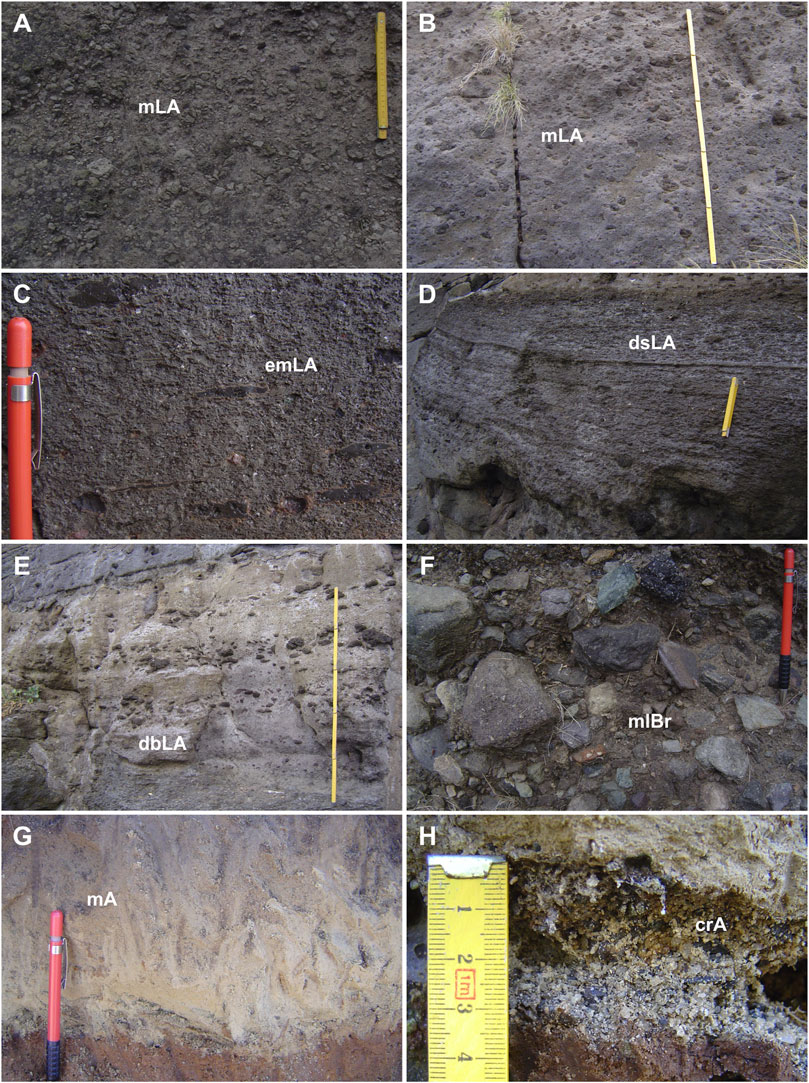
FIGURE 5. Lithofacies of the LAI. (A) Massive lapilli-ash (mLA) from the Angra Ignimbrite (TERL63 in Figure 7; 20 cm scale). (B) Lithified massive lapilli-ash (mLA) from the Lajes Ignimbrite (TERL2 in Figure 8; 1 m scale). (C) Eutaxitic massive lapilli-ash (emLA) from the Lajes Ignimbrite (TERL61 in Figure 7; pen for scale). (D) Diffuse-stratified lapilli-ash (dsLA) and (E) diffuse-bedded lapilli-ash (dbLA) from the Lajes Ignimbrite (both from TERL59 in Figure 7; D scale is 20 cm; E scale is 1 m); (F) Massive lithic breccia (mlBr) from the Lajes Ignimbrite (TERL39 in Figure 8; pen for scale); (G) Massive ash (mA) from the Lajes Ignimbrite (TERL20 in Figure 8; pen for scale). (H) Crystal-rich ash (crA) from the Lajes Ignimbrite (TERL18 in Figure 8; scale in cm).
Interpretation: The massive nature, poor sorting and absence of stratification suggest rapid progressive aggradation from a sustained high particle concentration, granular fluid-based PDC with a fluid escape-dominated flow-boundary zone, although the presence of clast lenses suggests the development of a granular flow-dominated flow-boundary zone (Druitt, 1998; Branney and Kokelaar, 2002; Scarpati et al., 2020). In a fluid escape-dominated flow-boundary zone clasts are supported by the upward flux of interstitial fluid escaping the matrix as a result of hindered settling, while in a granular flow-dominated flow-boundary zone clast interaction is the main support mechanism due to the high particle concentration and granular shear (Branney and Kokelaar, 2002; Sulpizio and Dellino, 2008).
Eutaxitic Massive Lapilli-Ash
Description: Eutaxitic massive lapilli-ash (emLA) is commonly seen in the lower part of the Lajes Ignimbrite but in places can represent more than half of its thickness. In the Angra Ignimbrite, this lithofacies is only found at one location on the costal cliff. The eutaxitic massive lapilli-ash lithofacies is welded and matrix-supported with juvenile and lithic clasts in varying proportions. The matrix is typically dark grey and crystal-rich, with feldspar crystals up to 3 mm in length. The welding intensity varies from poorly to densely welded with well-developed eutaxitic texture (Figure 5C). Where densely welded, juvenile clasts are strongly flattened dense clasts, which form fiamme up to 30 cm in length. In poorly welded examples, juvenile clasts range from slightly flattened (oblate shapes) to subrounded clasts with scoriaceous texture. Lithic clasts have identical lithologies to those described in the non-welded massive lapilli-ash but are finer-grained (up to 3 cm in size) and less abundant. This lithofacies typically lacks internal stratification but diffuse juvenile-rich or lithic-rich horizons are recognized locally.
Interpretation: The massive nature of this lithofacies suggests that particles were predominately deposited from a current with fluid escape-dominated to granular flow-dominated flow-boundary zones (Branney and Kokelaar, 2002; Scarpati et al., 2020), similarly to the non-welded lithofacies. The welding of the deposits, together with the coexistence of fiamme and oblate scoriaceous clasts, suggest rapid progressive aggradation from a high temperature PDC, where syn-depositional agglutination of pyroclasts occurred at temperatures exceeding the glass transition, rather than by load compaction (Russell and Quane, 2005; Dávila-Harris et al., 2013; Scarpati et al., 2020).
Diffuse-Stratified Lapilli-Ash
Description: Diffuse-stratified lapilli-ash (dsLA) is found locally in the Angra and Lajes ignimbrites. It consists of matrix-supported, poorly sorted deposits, with variable proportions of juvenile and lithic clasts in a light grey to dark grey ash matrix rich in feldspar crystals (up to 3 mm in length). This lithofacies is commonly lithified. The juvenile clasts are rounded to subrounded and comprise the same lithologies of those described in the massive lapilli-ash lithofacies. Lapilli-sized clasts are predominant, with maximum clast sizes up to 6 cm. Lithic clasts are angular to subrounded and have the same lithologies of those described in massive lithofacies but are finer-grained (up to 5 cm in size). The internal structure is marked by very thin layers (<1 cm thick) with diffuse parallel to sub-parallel stratification (Figure 5D). Individual layers are composed of juvenile and/or lithic fine lapilli clasts that alternate with ash layers. In places, the fine lapilli layers show normal or inverse grading, defined by subtle grain size variations. The layers often show small thickness variations and are lateral discontinuous over a few meters.
Interpretation: Diffuse stratification and laterally discontinuous layering are indicative of subtle unsteadiness during deposition from a sustained high particle concentration PDC with transitional flow-boundary zones between fluid escape-dominated and granular flow-dominated, with short periods of traction-dominated conditions (Branney and Kokelaar, 2002; Brown and Branney, 2004; Brand et al., 2014). The unsteadiness within the flow-boundary zone likely arises from one or more mechanisms: 1) successive surges or waves in a fluctuating sustained PDC, 2) periodic impingement into the flow-boundary zone of turbulent eddies, 3) intrinsic frictional effects within the thickening granular flow-dominated flow-boundary zone (Branney and Kokelaar, 2002), and 4) flow-boundary zone perturbations travelling up-current in response to interaction with topography (Brand et al., 2014; Báez et al., 2020a).
Diffuse-Bedded Lapilli-Ash
Description: Diffuse-bedded lapilli-ash (dbLA) is only found in the upper part of the Lajes Ignimbrite, along the south coast. It is matrix-supported, poorly sorted, with juvenile and lithic clasts in varying proportions in a dark grey ash matrix with feldspar crystals (up to 3 mm in length). This lithofacies is commonly lithified. The juvenile clasts (rounded to subrounded) and lithic clasts (angular to subrounded) have the same lithologies of those described in the massive lapilli-ash lithofacies. Lapilli-sized clasts are predominant but maximum clast sizes of juveniles and lithics can reach up to 20 and 7 cm, respectively. The internal structure is defined by parallel to sub-parallel beds of juvenile clasts and few lithic clasts (Figure 5E). Individual beds are centimetre to decimetre thick (typically <25 cm) and laterally discontinuous over a few metres; local thickness variations are common. Most beds are internally massive but some show inverse grading of juvenile clasts and/or normal grading of lithic clasts. The tops and bases of individual beds show diffuse boundaries.
Interpretation: Diffuse bedding suggests local current unsteadiness during deposition from a sustained high particle concentration PDC with granular flow-dominated to fluid escape-dominated flow-boundary zones (Branney and Kokelaar, 2002; Brown and Branney, 2004). The mechanisms that generate the unsteadiness within the flow-boundary zone are the same as described for the diffuse-stratified lithofacies. The occurrence of normal or inverse grading patterns within beds record marked current unsteadiness, possibly with brief periods of tractional deposition. The lateral discontinuous nature and thickness variations of the beds may result from current non-uniformity (Brown et al., 2007; Kokelaar et al., 2007).
Massive Lithic Breccia
Description: Massive lithic breccia (mlBr) is only found in the Lajes Ignimbrite, on the northern part of the island. It consists of matrix-supported, poorly sorted, lithic-rich deposits with a dark grey lapilli-ash matrix rich in feldspar crystals (up to 3 mm in length). Lithic clasts are angular to subrounded, with variable lithologies including basaltic and trachytic lavas, hydrothermally altered clasts (orange to reddish colour), syenite xenoliths, obsidian chips and basaltic scoria clasts, in decreasing order of abundance (Figure 5F). Lapilli-sized lithic clasts are predominant but larger clasts with maximum clast sizes of 30–35 cm are common. Juvenile clasts are subordinate, rounded to subrounded and comprise scoria and dense clasts. The juvenile clasts reach up to 6 cm in size. The lithofacies is massive, without recognizable internal structures.
Interpretation: The massive nature and poor sorting suggest progressive aggradation from a sustained granular fluid-based lithic-rich PDC with transitional flow-boundary zones between fluid escape-dominated and granular flow-dominated (Branney and Kokelaar, 2002; Brown and Branney, 2004; Dávila-Harris et al., 2013; Báez et al., 2020a), where the high lithic clast concentration was enough to suppress basal turbulence and traction. It reflects a greater competence of the current with respect to the massive lapilli-ash lithofacies. The abundance of lithic clasts in these deposits may indicate significant conduit wall erosion or partial edifice (caldera) collapse (Branney and Kokelaar, 2002; Báez et al., 2020a).
Massive Ash
Description: Massive ash (mA) is only found in the lower part of the Lajes Ignimbrite. It consists of well sorted to poorly sorted (1.5 < σϕ < 3.0; Self, 1974) light grey vitric ash, that locally grades upward to dark grey, abundant feldspar crystals (up to 3 mm in length) and rare juvenile and lithic fine lapilli clasts (Figure 5G). Juvenile clasts are rounded to subrounded and comprise pumice and scoria (up to 1 cm in size). Lithic clasts are angular to subrounded and have the same lithologies of other lithofacies (up to 0.6 cm in size). The massive ash deposits are structureless, locally lithified, and contain abundant carbonized wood fragments.
Interpretation: The massive nature, absence of internal structures, clast lenses and alignments, and fine grain size suggest steady progressive aggradation from a sustained high particle concentration, fine-grained granular fluid-based PDC with a fluid escape-dominated flow-boundary zone and a minimal granular flow-dominated component (Branney and Kokelaar, 2002; Brown et al., 2007; Kokelaar et al., 2007).
Crystal-Rich (Lithic-Rich) Ash
Description: Crystal-rich ash (crA) is commonly found at the base of Lajes Ignimbrite and locally at the base of the Angra Ignimbrite. It is clast-supported, well sorted, although rare poorly sorted examples exist (Angra 1.0 < σϕ < 2.2 vs. Lajes 1.1 < σϕ < 2.8; Self, 1974), and fines-depleted, mostly composed of feldspar crystals up to 3 mm in length (Figure 5H). In places, it is rich in ash-sized to fine lapilli lithics (lA). Lithic clasts are angular to subangular and comprise basaltic and trachytic lavas, hydrothermally altered clasts, and syenite xenoliths, in decreasing order of abundance. Juvenile clasts are subordinate, rounded to subrounded, ash-sized to fine lapilli pumice clasts; rare dense clasts are only found in the Lajes Ignimbrite. Small, carbonized wood fragments are common. This lithofacies is typically massive (structureless) but plane-parallel bedding and imbrication of the denser clasts (crystals or lithics) are observed locally.
Interpretation: The typically good sorting, crystal-rich (lithic-rich) nature and depletion of fine ash suggest rapid deposition of material from a dilute PDC or dilute part of a PDC (Branney and Kokelaar, 2002; Cas et al., 2011; Scarpati et al., 2015). The depletion of fine vitric ash and enrichment in crystals and lithic clasts implies density segregation during turbulent flow transport, where fine juvenile ash is elutriated. The ingestion and heating of cold air at the front of a PDC generates vigorous turbulence and consequently segregation of the denser clasts (crystals or lithics). The present of carbonized wood fragments reveals the current was hot enough to burn vegetation, which may have also enhanced elutriation of fine ash (Branney and Kokelaar, 2002; Scarpati et al., 2015). The crystal-rich (lithic-rich) ash bed at the base of the ignimbrites is interpreted as a ground layer (Sparks, 1976; Walker et al., 1981; Wilson, 1985; Scarpati et al., 2015).
Ignimbrite Architecture
Vertical Variations
The Angra Ignimbrite typically starts with a light grey, fine-grained massive lapilli-ash base, although locally diffuse-stratified lapilli-ash is observed (Figure 6A). In a few places a thin fines-poor, crystal-rich bed is present at the base. At one outcrop on the costal cliff (TERL72, see Figure 7 for location), the lowermost part of the ignimbrite is incipiently welded, showing weak eutaxitic texture. Upwards it grades diffusely into non-welded light grey, coarse-grained massive lapilli-ash. Pumice-rich lenses are present within the massive lapilli-ash. The topmost portion of the ignimbrite is typically finer grained.
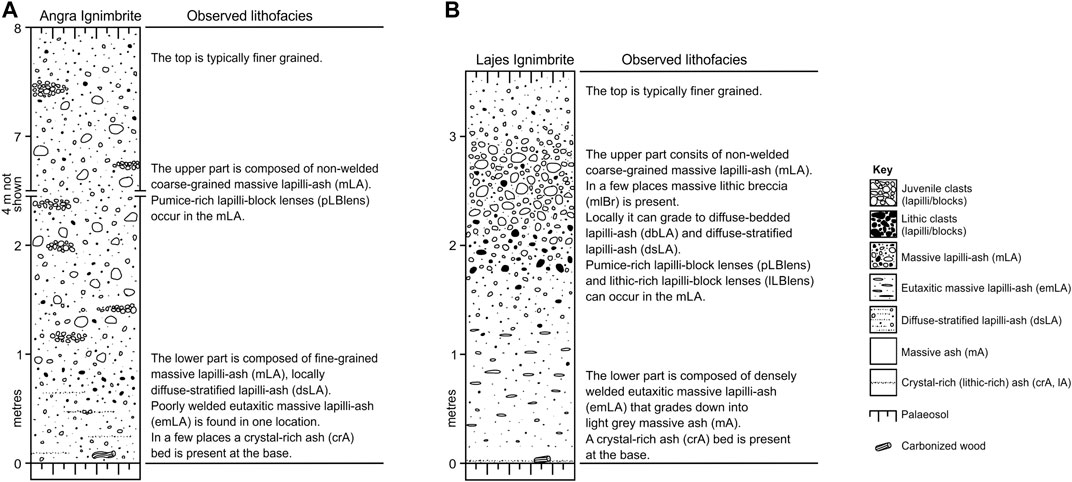
FIGURE 6. Generalized stratigraphic sections of the Angra Ignimbrite (A) and the Lajes Ignimbrite (B), with description of the observed lithofacies.
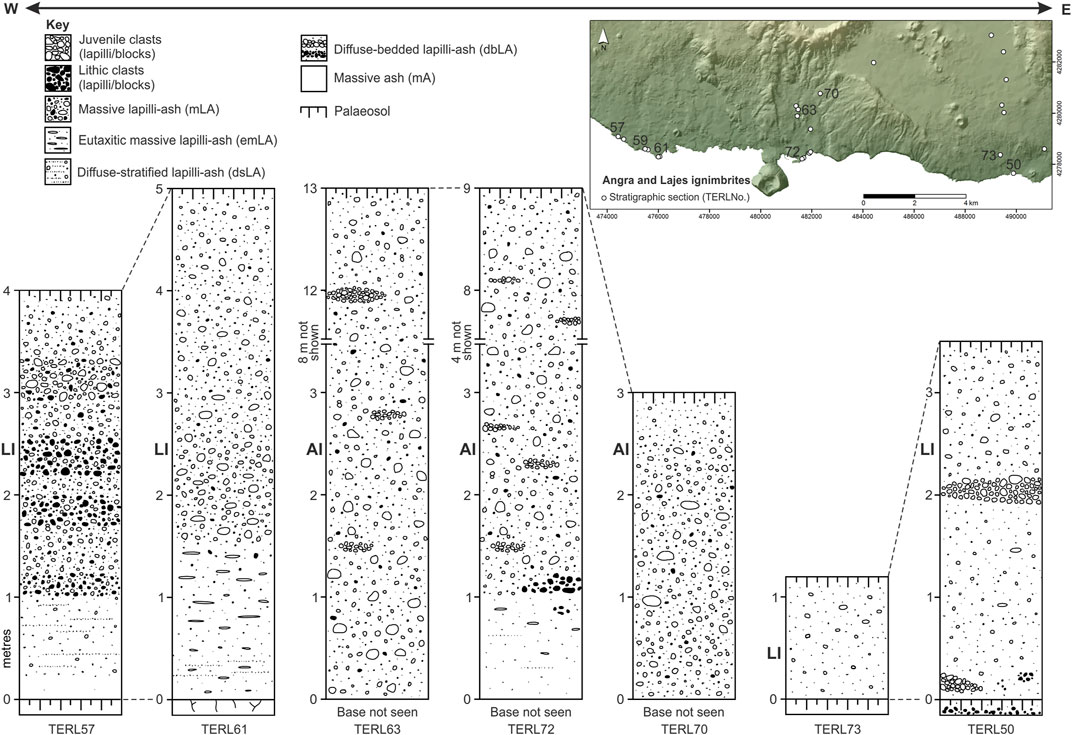
FIGURE 7. Representative stratigraphic sections of the Angra and Lajes ignimbrites along the south coast of Terceira. AI—Angra Ignimbrite.; LI—Lajes Ignimbrite.
The Lajes Ignimbrite typically starts with a thin basal fines-poor, crystal-rich, locally lithic-rich bed (<4 cm thick on average). This basal crystal-rich bed is locally absent, for example along the south coast. The upper contact of this thin bed is usually sharp but in places can be diffuse. It is conformably overlain by light grey massive ash. It diffusely grades up into dark grey, fine-grained massive lapilli-ash, which is commonly welded showing well-developed eutaxitic texture. Upwards it gradually passes through incipiently welded to non-welded dark grey, coarse-grained massive lapilli-ash (Figure 6B). Pumice-rich or lithic-rich lenses are locally present in the massive lapilli-ash. Locally it can grade from diffuse-stratified to diffuse-bedded lapilli-ash. In a few places in the north part of the island it includes a massive lithic breccia. The topmost portion of the ignimbrite is typically finer grained. The vertical contacts between the different lapilli-ash lithofacies are diffuse.
There is no evidence of unconformities (e.g., major erosion surfaces, reworked horizons, pumice/ash fall layers) in the ignimbrites. Neither the Lajes nor Angra ignimbrites have pumice fall deposits at the base or the top.
Lateral Variations
No significant lateral variations of lithofacies were observed in the Angra Ignimbrite as it has a very restricted outcropping area, which is topographically constrained to one valley (Figure 7).
The Lajes Ignimbrite shows lateral lithofacies variations along the north and south coastal areas (Figures 7, 8). On the north coast, where the Lajes Ignimbrite is best exposed, it is possible to observe lateral variations closely related to the underlying topography. Along the coastal cliffs, the deposit has a rather uniform thickness (3.5 m on average) and shows the same vertical lithofacies sequence (described previously) over several hundreds of metres (Figure 9). However, thicker deposits (up to 10 m thick) are found in palaeovalleys and tend to be coarser-grained. Where thick, coarse-grained ignimbrite gradually thins against a topographic feature, it can be traced uphill to thin (typically <1 m) fine-grained massive lapilli-ash or massive ash deposit with crystal-rich base. This lateral transition is gradational and generally marked by the thinning and fining of the sequence.
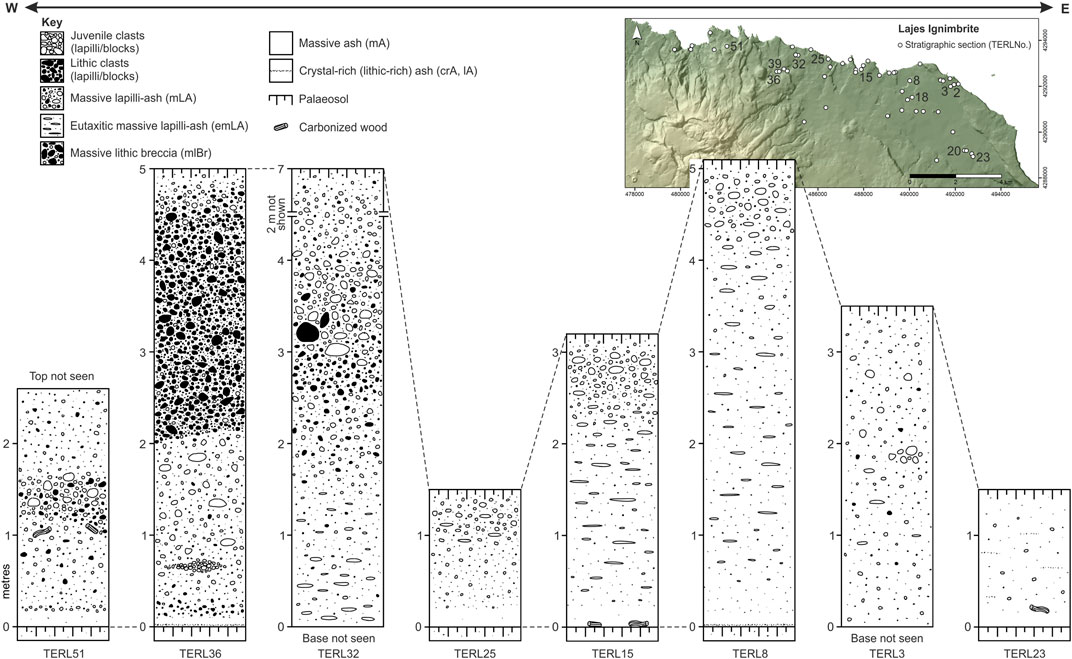
FIGURE 8. Representative stratigraphic sections of Lajes Ignimbrite along the north coast of Terceira.
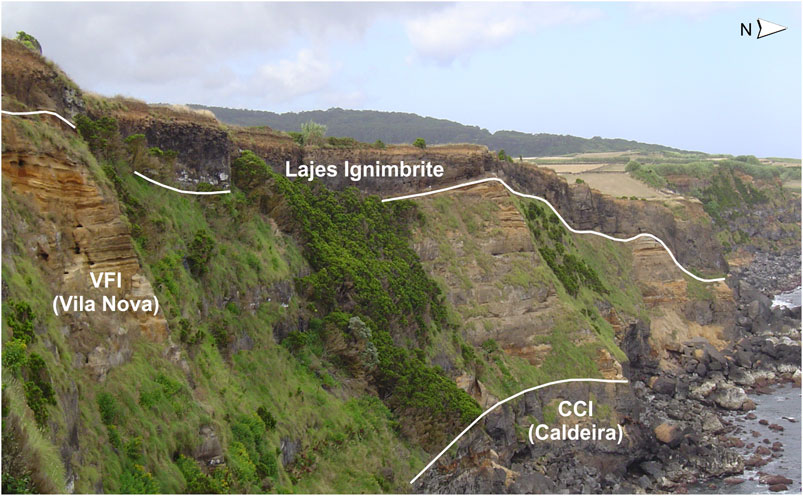
FIGURE 9. View of cliffs along the north coast of Terceira showing the Lajes Ignimbrite overlying a sequence of older pyroclastic formations containing ignimbrites (VFI and CCI) separated by major erosion surfaces. Abbreviations are as given in Figure 2; members are given in parenthesis. Cliff is 30 m high in the foreground (left side of the picture).
Petrography and Geochemistry
Petrography and Mineral Chemistry
The juvenile clasts of the LAI include light grey pumices (Figure 10A), which may be porphyritic, dark grey/black coarsely porphyritic scoriae (Figure 10C), and black coarsely porphyritic dense clasts (Figure 10E) that are exclusive to the Lajes Ignimbrite. Pumices are highly vesicular (53–81 vol. %) with low phenocryst contents (typically <10 vol. % but up to 20 vol. % in some instances). Phenocrysts are set in a whitish glassy groundmass with few to no microlites. Vesicles range from several millimetres to a few micrometres in size and have subcircular to slightly elongated shapes (Figure 10B). Some examples show extreme vesicle elongation (i.e., tube pumice). Glomerophyric aggregates of phenocrysts are found sporadically.
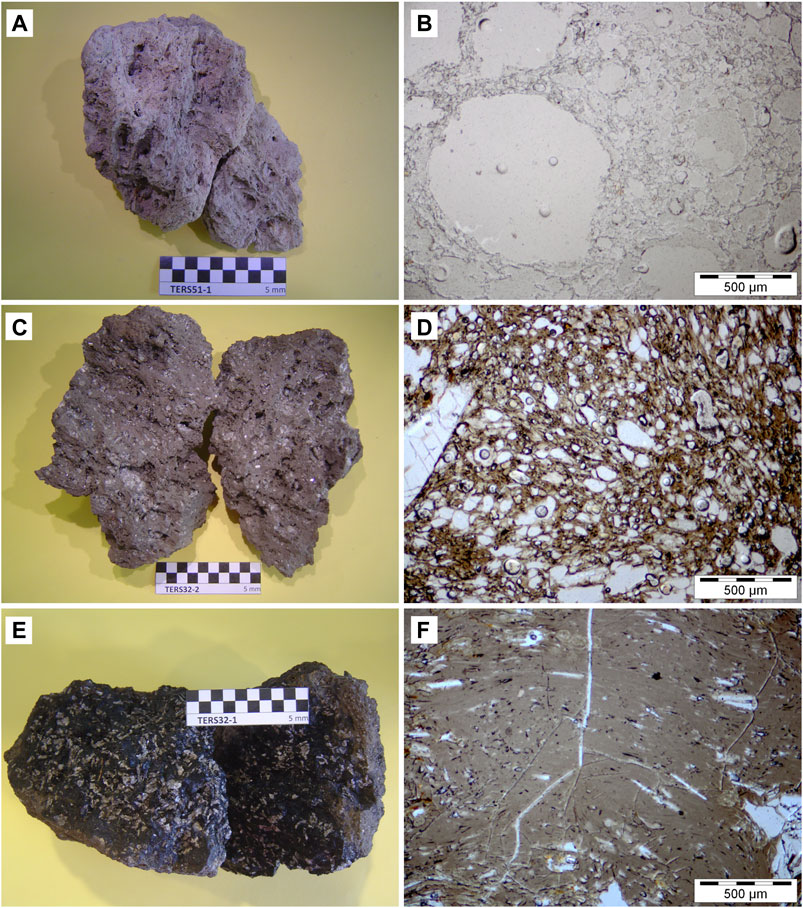
FIGURE 10. Representative juvenile clasts of the LAI and photomicrographs showing their textures. (A) Light grey pumice clasts. (B) Highly vesicular pumice with millimetre-sized subcircular vesicles. (C) Dark grey coarsely porphyritic scoria clats. (D) Microvesicular scoria with subcircular to slightly elongated vesicles and an alkali feldspar phenocryst. (E) Black coarsely porphyritic dense clasts. (F) Dense clast with brown glassy groundmass, collapsed vesicles, and dispersed alkali feldspar microphenocrysts.
Scoriae have lower vesicularity (30–47 vol. %) and higher phenocryst content (14–23 vol. %) than pumices. Coarsely porphyritic scoriae show large phenocrysts (4–5 mm) set in brown glassy, or more rarely microcrystalline, groundmass. Glomerophyric aggregates are more common than in pumices. Scoriae are typically microvesicular, with vesicles reaching up to a few millimetres in size (Figure 10D), and subcircular to irregularly shaped (separated by thicker walls). Vesicles are frequently coalescent and in some cases are strongly stretched. Cryptocrystalline bands of devitrified glass are occasionally found alternating with microlite-free brown glass.
Dense clasts are characterized by low vesicularity (16–27 vol. %) and high phenocryst content (15–22 vol. %). They are coarsely porphyritic and characterized by brown glassy microlite-free or microlite-poor groundmasses. Glomerophyric aggregates of phenocrysts are common. The vesicles are small (not more than a few tens of micrometres in size), ranging from irregularly shaped, elongated to strongly stretched. In the most extreme cases, vesicles are completely collapsed (Figure 10F). Alternating bands of dark brown and light brown glass are distinguishable and, in many cases, deformed, clearly draping around phenocrysts (i.e., flow structures). Isolated or clustered spherulites are present occasionally.
The mineral contents, proportions, and compositions of the Angra and Lajes ignimbrites are similar. Anorthoclase (An0-4, Ab64-71, Or25-35) phenocrysts represent the predominant mineral phase in the juvenile clasts of both ignimbrites (≤22 vol. %), reaching sizes of up to 5 mm in length, and exhibiting variable morphologies, including euhedral crystals (Figure 11A), subhedral crystals with rounded edges, crystals with embayments, and large crystals with resorbed cores filled by glassy pockets (which are frequently vesicular; Figure 11B). Small microphenocrysts, and microlites when present, are euhedral and do not show disequilibrium features. Glomerocrysts comprising predominantly anorthoclase are common. Euhedral to subhedral augite (En24-38, Fs20-34, Wo39-43) phenocrysts are less common (≤1.2 vol. %), reaching up to 3 mm in length. Large phenocrysts are frequently fractured and show disequilibrium features with the host rock (e.g., reaction rims and rounded edges). Microphenocrysts of clinopyroxene can also be found partially or fully included within alkali feldspars. Clinopyroxene phenocrysts are commonly associated with smaller Fe-Ti oxides (Figure 11C) and acicular apatite. Olivine is rare (<1 vol. %) and occurs as phenocrysts (up to 800 μm), but more frequently as microphenocrysts. Phenocrysts of olivine commonly show disequilibrium features, such as anhedral morphologies, or partially resorbed crystals with reaction rims (Figure 11D). Microphenocrysts tend to be subhedral. Olivine occurs as either isolated crystals or in glomerophyric aggregates with other mineral phases. Compositionally, they cover a narrow range of chemical compositions of Fo30-41. Fe-Ti oxides (≤0.6 vol. %) are euhedral to anhedral and range from phenocryst (up to 400 μm) to microlites. They are frequently found in association with, or as inclusions in, clinopyroxene and olivine (Figures 11C,D). Apatite is an accessory mineral phase and is found as isolated microphenocrysts (<300 μm) or as acicular inclusions within clinopyroxene and olivine (Figure 11D). In compositional terms it can be classified as fluorapatite (3.2 wt. % F). In addition to mineral phases, LAI juvenile material contains rare, medium-grained syenitic xenoliths comprised predominantly of alkali feldspar (Gertisser et al., 2010; Jeffery et al., 2017).
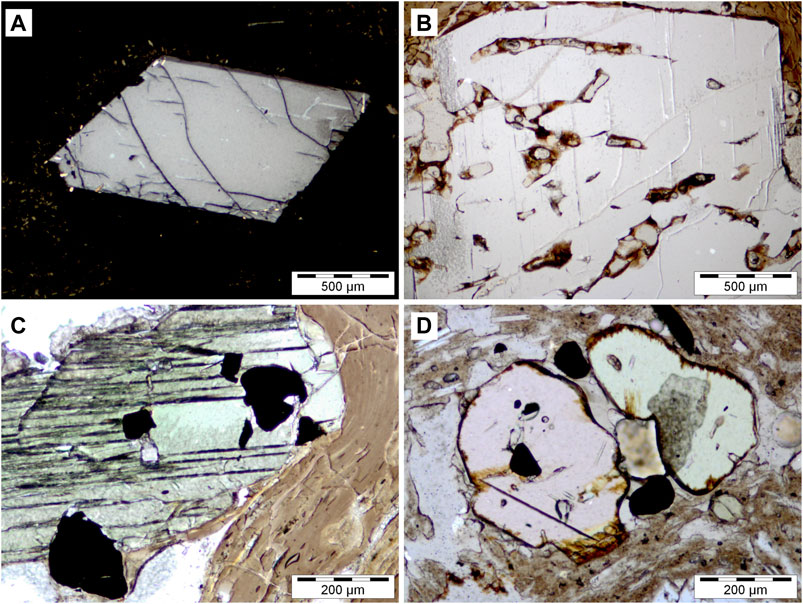
FIGURE 11. Representative photomicrographs of mineral phases from the Angra and Lajes ignimbrites. (A) Euhedral alkali feldspar phenocryst. (B) Alkali feldspar phenocryst with partially resorbed core filled by vesicular glassy pockets. (C) Subhedral clinopyroxene phenocryst with Fe-Ti oxide inclusions. (D) Olivine phenocrysts with reaction rims and inclusions of Fe-Ti oxides and small acicular apatite.
Whole Rock and Glass Composition
Whole rock major element geochemical data indicate that both the Angra and Lajes ignimbrites are classified as peralkaline trachyte, with SiO2 contents that range from 65 to 66 wt. %, total alkali contents of 11–12 wt. % (Figure 12A), and peralkalinity indices (PI = molar Na2O + K2O/Al2O3) between 1.00 and 1.12. Groundmass glass analyses for both ignimbrites show slightly more chemical diversity and some minor differences between the two (e.g., Angra total alkalis = 9.6 to 11.4 vs. Lajes total alkalis = 10.1 to 13.1 wt. %; Angra PI = 0.88 to 0.99 vs. Lajes PI = 0.91 to 1.29; Figure 12B). In the Al2O3 vs. FeOT classification scheme of Macdonald (1974), all the peralkaline samples of this study (whole rock and groundmass glass) are comenditic trachyte, excluding a small number of glass analyses from the Lajes Ignimbrite which plot as comendite (Figure 12C).
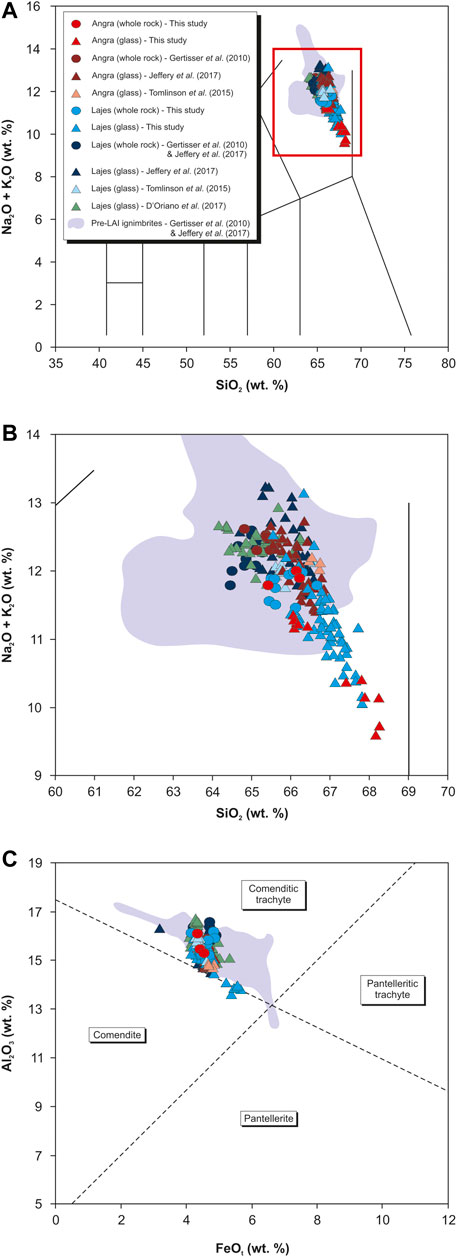
FIGURE 12. Major element classification plots for the Angra and Lajes ignimbrites. Whole rock and groundmass glass analyses are distinguished for clarity. Ignimbrites that pre-date the LAI are also shown for reference. (A) Chemical compositions of the Angra and Lajes ignimbrites plotted in the total alkali vs. silica diagram (Le Maitre et al., 2002). (B) Enlargement of panel 12A, highlighting the chemical variation within the LAI. (C) Peralkaline compositions for the Angra and Lajes ignimbrites plotted on the Al2O3 vs. FeOT classification scheme for oversaturated peralkaline rocks (Macdonald, 1974).
On the basis of major element chemistry alone, the two ignimbrites are difficult to distinguish from each other. However, trace element compositions yield further information. Zirconium concentrations, which are used here as a differentiation index, show a considerable range between 435 and 963 ppm. Plotted against Zr, FeOT concentrations increase with differentiation, whilst MgO and CaO contents decrease (Figure 13). When plotted against other incompatible elements such as Nb and Rb, the LAI is defined by a positive, linear trend (Figures 13D,E). Groundmass glass compositions from the Lajes Ignimbrite extend across the full length of this range, whereas whole rock analyses are limited to <760 ppm. The whole rock data for the Angra Ignimbrite are similar to those of the Lajes Ignimbrite; however, the groundmass glass compositions are bimodal, with the majority of data clustering around 790 ppm, and a smaller cluster around 520 ppm. Strontium concentrations are characteristically low for peralkaline magmas (<20 ppm) and exhibit a scattered but broadly negative correlation with Zr (Figure 13F). Barium exhibits a similar relationship with Zr but also shows considerable scatter and range (55–737 ppm; Figure 13G). Chrondrite-normalised REE patterns are typically LREE enriched relative to the HREE, with both ignimbrites having average LaN/YbN ratios of 9.7 (Figure 14). Variably negative Eu anomalies occur in both the Angra and Lajes ignimbrites, with average Eu/Eu* of 0.61 and 0.51, respectively. Furthermore, Eu/Eu* values show a negative correlation with Zr concentrations (Figure 13H).
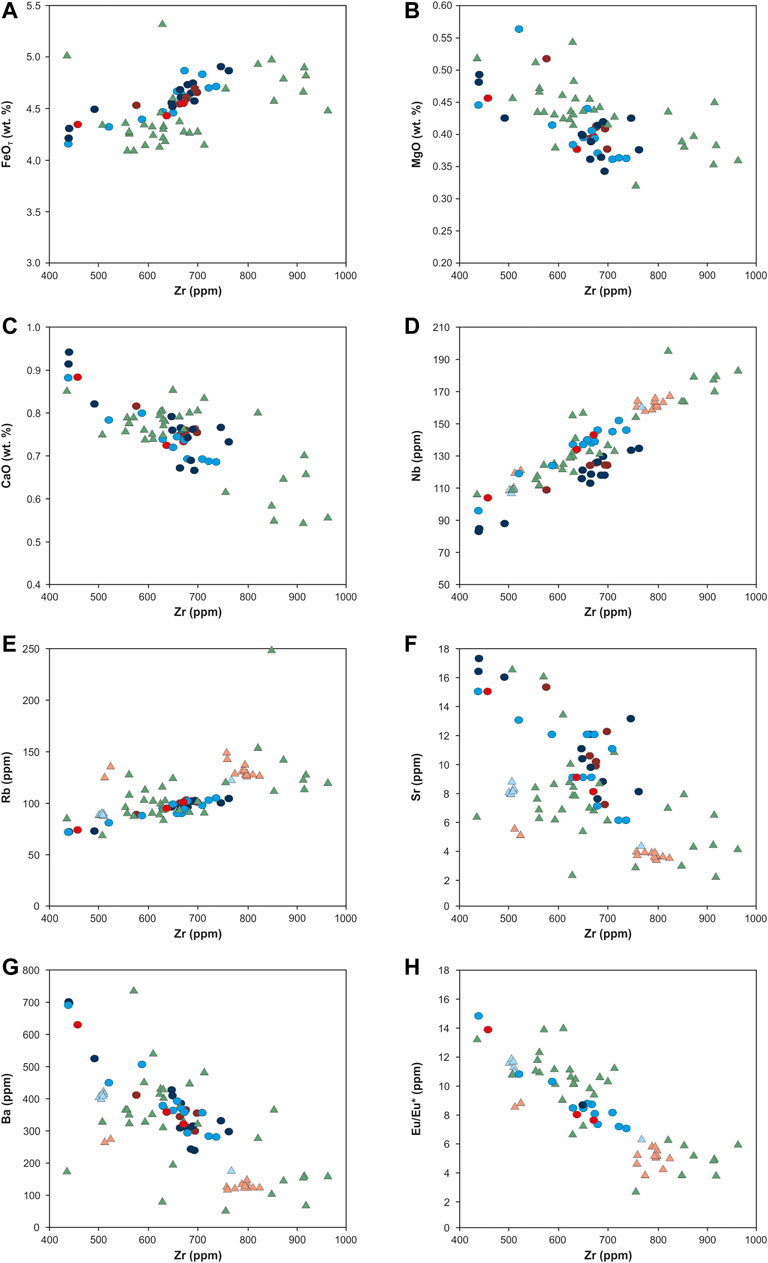
FIGURE 13. Selected major and trace element data plotted vs. Zr, which is applied as an index of differentiation. Major element data are reported in wt. %, trace elements as ppm. All symbols and data sources are as given in Figure 12.
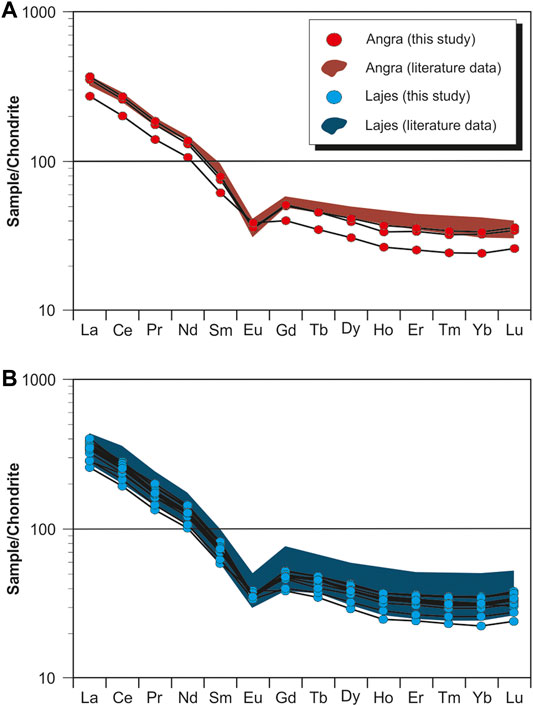
FIGURE 14. Chondrite-normalised REE patterns for the Angra (A) and the Lajes (B) ignimbrites. Coloured fields denote the total range of literature data derived from Tomlinson et al. (2015), D’Oriano et al. (2017), and Jeffery et al. (2017). Chondrite values taken from Sun and McDonough (1989).
Discussion
Ignimbrite Aspect Ratio and Volume
The aspect ratio of the Angra Ignimbrite was estimated based on an average thickness of 8 m and the diameter of a circle with an equal area to that reconstructed for the ignimbrite (10 km2; Figure 3). This yields an aspect ratio of 1:500 (H/L = 2 × 10–3) which is in the range of typical values considered for HARIs (Walker et al., 1980; Giordano and Doronzo, 2017). The aspect ratio of the Lajes Ignimbrite was estimated assuming an average thickness of 3.5 m and the diameter of a circle with the same area as that reconstructed for the ignimbrite (168 km2; Figure 4). This corresponds to an aspect ratio of 1:4200 (H/L = 2.4 × 10–4). Alternatively, if the maximum run-out distance of the Lajes Ignimbrite is considered, from Pico Alto caldera to Porto Judeu coastal cliffs (15 km; Figure 4), then the aspect ratio of the Lajes Ignimbrite is in the order of 1:8600 (H/L = 1.2 × 10–4). Both these values are within the typical range considered for LARIs (Walker et al., 1980; Dade, 2003; Giordano and Doronzo, 2017).
Volumes of the Angra and Lajes ignimbrites were conservatively estimated by multiplying the average thickness of the deposits by the area of the reconstructed extents (Figures 3, 4). The bulk on-land volume estimated for the Angra Ignimbrite is 0.08 km3. This converts to a dense rock equivalent (DRE) on-land volume of 0.04–0.06 km3, based on ignimbrite densities of 1,300–1800 kg/m3 and a trachytic magma density of 2,500 kg/m3 (Self, 1974). The Lajes Ignimbrite yields a bulk on-land volume of 0.59 km3. This corresponds to a DRE on-land volume of 0.31–0.42 km3 using the same range of ignimbrite densities and trachytic magma density as for the Angra Ignimbrite.
Estimating physical parameters of old pyroclastic deposits on islands such as Terceira is challenging due to various factors, including the small size of the island, the thick cover of younger UTG products, the luxuriant vegetation and the absence of deeply dissecting streams, which limit the number of exposures. Taking into account the thickness of the outcrops along the north and south coastal cliffs it is reasonable to assume that a significant (unknown) amount of erupted material was deposited at sea, as commonly reported for other islands such as Montserrat (Lesser Antilles), Stromboli (Aeolian Islands), Faial and São Miguel (Azores archipelago) (e.g., Trofimovs et al., 2006; Andronico and Pistolesi, 2010; Pimentel et al., 2015; Porreca et al., 2018). Therefore, the values obtained for the Lajes and Angra ignimbrites are minimal.
Nevertheless, the volume of erupted magma estimated for the Lajes Ignimbrite (0.31–0.42 km3 DRE) is within the same order of magnitude of other major explosive eruptions recorded in the Azores, such as the 4.6 ka Fogo A Plinian eruption (Fogo volcano, São Miguel) with 0.64 km3 DRE (Booth et al., 1978) and the 16 ka Santa Bárbara caldera-forming eruption (Sete Cidades volcano, São Miguel) with 0.25–0.54 km3 DRE (Kueppers et al., 2019). However, these differ from the Lajes Ignimbrite-forming eruption as they produced thick pumice fall deposits in addition to ignimbrites (Pensa et al., 2015; Queiroz et al., 2015).
Magmatic System and Eruption Style
The Angra and Lajes ignimbrites share the same comenditic trachyte whole rock major element composition (Figure 12) and mineral assemblage dominated by anorthoclase. However, their whole rock trace element and groundmass glass compositions vary considerably (Figure 13). Such variability is also observed in trace element composition of groundmass glasses and in textures and compositions of anorthoclase crystals of the Lajes Ignimbrite (D'Oriano et al., 2017). The data presented in this study are consistent with the presence of a zoned magma reservoir which fed the Angra and Lajes ignimbrite-forming eruptions. This is a recurrent theme for the magma system of Pico Alto volcano (and potentially Guilherme Moniz), where the interplay of complex petrogenetic processes acting in the magma reservoir has been identified (D'Oriano et al., 2017; Jeffery et al., 2017). These include the derivation of the ignimbrite-forming comenditic trachyte from a zoned magma reservoir, with thermometric and hygrometric gradients, dominated by extended fractional crystallization and important contributions from in-situ crystallization, remobilization of cumulate material (crystal mush) and magma mixing processes. All these processes are, to some extent, masked by the seemingly homogeneous trachytic major element compositions of the eruptive products.
Chemically zoned magma reservoirs are common in other peralkaline volcanic systems, such as Furnas volcano on São Miguel (Jeffery et al., 2016), and are recorded by peralkaline ignimbrites such as the 13.9 Ma Ignimbrite “TL” (Sumner and Branney, 2002), the 13.6 Ma Ignimbrite “A” (Troll and Schmincke, 2002), and the 11.8 Ma Ayagaures ignimbrite (Jutzeler et al., 2010), on Gran Canaria, and the 45 ka Green Tuff Ignimbrite, Pantelleria (Williams, 2010), as well as a range of other ocean island systems (Jeffery and Gertisser, 2018 and references therein). Despite this, the data presented in this study cannot be accounted for adequately by a traditional zoned magma reservoir model featuring a single magma body with an upward gradational transition from less to more evolved magma, and may instead highlight a more complex magmatic plumbing system, potentially comprising multiple magma bodies.
Although the eruption triggering mechanism is uncertain, D'Oriano et al. (2017) proposed that the magmatic system of Pico Alto was disturbed by a heat source, most probably induced by the arrival of a hotter magma from depth, but without mixing with the resident comenditic trachyte magma reservoir. The thermal destabilization at the base of the reservoir and the onset of eruption caused syn-eruptive mixing between different portions of the zoned magma reservoir, which contributed to the homogenization of the resident magma. We speculate that the first eruptive event tapped the uppermost part of the reservoir, which is recorded by the Angra Ignimbrite. Some (unknown) time after the initial event, a second eruptive event took place involving the withdrawal of a larger volume of magma and probably reaching the lower parts of the reservoir. The latter is recorded by the Lajes Ignimbrite.
The Angra Ignimbrite-forming eruption was characterized by short-lived low pyroclastic fountaining, most probably located in the southern part of Pico Alto caldera. The event did not form a convective eruptive column, as no pumice fall deposit was found in the ignimbrite sequence. The pyroclastic fountain generated a small but sustained PDC that seems to have been mostly channelled along a valley on the southern part of Terceira (Figure 3). The eruption of the Lajes Ignimbrite was dominated by vigorous and prolonged low pyroclastic fountaining (or boiling-over) from Pico Alto caldera generating a sustained PDC, which spread radially towards both the north and south coasts of the island (Figure 4). Other ignimbrites worldwide have also been interpreted to result from boiling-over eruptions (e.g., Cas et al., 2011; Willcock et al., 2013; Pacheco-Hoyos et al., 2018). Like the Angra Ignimbrite, the Lajes Ignimbrite-forming eruption was neither preceded nor followed by the formation of a convective eruptive column, as there are no pumice fall deposits associated with this ignimbrite sequence.
Pyroclastic Density Current Emplacement Dynamics
The vertical and lateral lithofacies variations observed in the Angra and Lajes ignimbrites reflect different conditions of the parent PDCs during deposition. Vertical lithofacies variations (Figure 6) record changes in PDC deposition through time (i.e., current unsteadiness), while lateral lithofacies variations (Figures 7, 8) record spatial variability of the PDC during deposition and its interaction with the topography (i.e., current non-uniformity) (Branney and Kokelaar, 2002).
The Angra Ignimbrite is composed of non-welded massive lapilli-ash throughout most of its thickness (Figure 6A). The massive nature and small volume of this ignimbrite indicate that it was deposited from a small but sustained high particle concentration granular fluid-based PDC. The dilute front of the current, which left in places a thin, fines-poor and crystal-rich bed, rapidly changed to high particle concentration, granular fluid-based flow conditions, overall dominated by steady deposition from fluid escape-dominated to granular flow-dominated flow-boundary zones (Branney and Kokelaar, 2002). Locally, subtle unsteadiness developed within the flow-boundary zone, leading to deposition of diffuse-stratified lapilli-ash. No unconformities were observed within the ignimbrite, which implies that the emplacement was continuous without significant time breaks, representing a single depositional unit. The Angra Ignimbrite has a high-aspect ratio geometry and limited areal extent, cropping out in only one valley south of Guilherme Moniz caldera, suggesting that the parent PDC may have been mostly channelled along this valley (Figure 3). However, it cannot be excluded that the Angra Ignimbrite is buried under younger deposits in the middle of the island, particularly on the floor of Guilherme Moniz and Cinco Picos calderas. The features of the Angra Ignimbrite are typical of HARIs deposited from relatively low velocity PDCs confined to topographic depressions (e.g., Walker, 1983; Cas and Wright, 1987; Cas et al., 2011; Martí et al., 2019).
The vertical lithofacies variations observed in the Lajes Ignimbrite reveal an overall inverse grading pattern, starting as a finer-grained base and grading to a coarser-grained upper part. However, the topmost portion of the ignimbrite is typically finer grained (Figure 6B). From its characteristics, we can infer that the ignimbrite was mostly deposited from a sustained, quasi-steady, high particle concentration granular fluid-based PDC that progressively waxed in competence during emplacement and then rapidly waned at the end (Kneller and Branney, 1995; Branney and Kokelaar, 2002). This reveals that the PDC dynamics changed as the eruption intensified. The initial dilute front of the PDC deposited a basal, fines-poor and crystal-rich bed (similar to the ground layer of other ignimbrites; Sparks, 1976; Scarpati et al., 2015), but it rapidly evolved to a high particle-concentration, granular fluid-based current dominated by steady deposition from fluid escape-dominated to granular flow-dominated flow-boundary zones. The high temperature of the PDC promoted the syn-depositional agglutination of pyroclasts and thus welding of the deposit, which is recorded by the eutaxitic massive lapilli-ash. As the PDC progressively waxed in competence, it deposited non-welded, coarse-grained massive lapilli-ash. The increase in the concentration and size of lithic clasts had a cooling effect on the current (Eichelberger and Koch, 1979; Martí et al., 1991), sufficient to inhibit welding of the upper part of the deposit. Local current unsteadiness, with transitional flow-boundary zones between fluid escape-dominated and granular flow-dominated, with brief periods of traction-dominated conditions (Branney and Kokelaar, 2002), led to deposition of diffuse-stratified and diffuse-bedded lapilli-ash.
Overall, the vertical sequence of the Lajes Ignimbrite indicates that this thin ignimbrite sheet (3.5 m average thickness) corresponds to a single depositional unit from a sustained quasi-steady PDC. This contrasts with previous studies (Self, 1974; Self, 1976; Gertisser et al., 2010) that interpreted the presence of two depositional layers based on strong pumice concentration zones and sharp boundaries between welded and non-welded lithofacies. However, these pumice concentrations are not ubiquitous; they are found in a few places in the northern part of the island, and the sharp welded/non-welded boundaries are only seen locally at São Mateus (south coast). Several lines of evidence support the single depositional unit, including the gradual transitions of lithofacies (i.e., diffuse boundaries), the gradual variation of welding intensity, and the absence of unconformities such as intra-ignimbrite pumice or ash fall layers and erosion surfaces.
The lateral variations of lithofacies observed in the Lajes Ignimbrite (Figures 7, 8) cannot be interpreted as typical valley-pond ignimbrite to veneer deposit transitions commonly seen in LARIs. The thin fine-grained massive lapilli-ash or massive ash deposit with crystal-rich base observed where the ignimbrite thins against topography differs from the stratified veneer lithofacies described for intermediate to small volume ignimbrites (e.g., Walker et al., 1980; Walker et al., 1981; Wilson and Walker, 1982; Wilson, 1985; Giordano et al., 2002). In the present case, the lateral transition corresponds to a condensed sequence of the Lajes Ignimbrite, including the lateral extent of the ground layer. The absence of stratified lithofacies suggests that the upper part of the current was not as turbulent as in other more dynamic PDCs. This sequence clearly differs from the fine ash deposit (commonly weathered into soil) that displays mantle bedding and is widespread across the island, including on topographic highs (Figure 4), which we interpret to correspond to the co-ignimbrite ash fall deposit.
Thus, the lateral lithofacies variations can be interpreted as recording non-uniformity within the flow-boundary zone (Branney and Kokelaar, 2002). The simultaneous deposition of coarse-grained massive lapilli-ash in a certain location and massive ash in another location may result from current instabilities and relatively non-uniform deposition. This is evident where the Lajes Ignimbrite parent PDC interacted with more irregular topography, such as stream valleys, fault scarps or caldera walls, which led to marked lateral variations of lithofacies in the space of just a few metres distance.
Although the Lajes Ignimbrite has a low-aspect ratio geometry, the parent PDC generally flowed along areas of low-lying ground with limited capacity to overcome topographic highs, such as the faults scarps of the Lajes Graben (10–70 m high) and the caldera walls of Cinco Picos and Guilherme Moniz volcanoes (up to 200 m high). In the western part of the island, the PDC was limited by the slopes of Santa Bárbara volcano (Figure 4). This contrasts with the typical flow behaviour of PDCs that generate LARIs, which are interpreted as highly energetic currents capable of surmounting significant topographic barriers (Walker et al., 1980; Cas and Wright, 1987; Wilson et al., 1995; Cas et al., 2011).
Therefore, the low-aspect ratio of the Lajes Ignimbrite may simply result from deposition over a generally flat topography such as the coastal areas and the floor of the Lajes Graben, rather than from a highly energetic PDC. We propose that the ignimbrite was emplaced from a sustained high particle concentration, granular-fluid-based PDC with relatively low velocity and low heat loss. The low energy, placid style of emplacement helped conserve heat and promote welding in the Lajes Ignimbrite. Similar emplacement dynamics have been proposed for large volume ignimbrites such as the 4.3 Ma Kizilkaya ignimbrite, Turkey (Schumacher and Mues-Schumacher, 1996), the 2.08 Ma Cerro Galán Ignimbrite, Argentina (Cas et al., 2011), and the <73 ka Campo de la Piedra Pómez ignimbrite, Argentina (Báez et al., 2020b), but this mechanism apparently also holds for smaller volume ignimbrites.
The different flow paths from Pico Alto caldera towards the north and south coasts of the island (Figure 4) mostly reflect the interaction of the PDC with palaeotopography, in particular with the caldera walls of Guilherme Moniz and Cinco Picos volcanoes, and the fault scarps of the Lajes Graben. The parent PDC seems to have been deflected by the fault scarps of the graben to reach the eastern end of the island at Praia da Vitória. The detailed geochemical reconstruction of the Lajes Ignimbrite indicates that the PDC was not simultaneously emplaced radially but rather different flow paths were emplaced at different times (D'Oriano et al., 2017). Similar flow behaviour has been recognized for the 45 ka Green Tuff Ignimbrite, Pantelleria (Williams et al., 2014) and for the 39 ka Campanian Ignimbrite, Italy (Fedele et al., 2016) through detailed chemical stratigraphy of the deposit.
Implications for Hazard Assessment
The geological record of Terceira shows that several ignimbrite-forming eruptions coming from Pico Alto and, possibly, Guilherme Moniz volcanoes have occurred in the last ca. 100 kyr (Gertisser et al., 2010). Although the frequency of ignimbrite-forming eruptions is relatively low and the last events occurred ca. 25 cal ka BP ago (recorded by the LAI), Pico Alto is an active central volcano and the possibility of other similar eruptions in the future should not be underestimated. The probability of an ignimbrite-forming eruption occurring from Santa Bárbara volcano is low, as its stratigraphy does not include ignimbrites, although a lithic-rich PDC deposit has been reported (Calvert et al., 2006). However, Santa Bárbara volcano has the potential to produce explosive and, therefore, ignimbrite-forming eruptions, thus a future event cannot be ruled out.
A future ignimbrite-forming eruption of Pico Alto volcano could strongly impact the island, with devastating and long-term socio-economic consequences for its 55,000 inhabitants (PORDATA, 2020) and for the entire Azores archipelago (Medeiros et al., 2021). An eruption with similar characteristics to the Lajes Ignimbrite-forming event would be a worst-case scenario in terms of hazard assessment for a small island such as Terceira (400 km2). If a future event behaved similarly to the Lajes Ignimbrite parent PDC, it would affect all of the central part of island and flow towards the sea on the north and south coasts (Figure 4). The relatively low velocity of the PDC might mean it would have limited ability to surmount topographic barriers, such as the fault scarps of the Lajes Graben or the caldera walls of Cinco Picos and Guilherme Moniz volcanoes, but it could be channelled to more distal areas maintaining high temperatures.
The well-populated villages along the north coast of the island between Biscoitos and Lajes would be strongly impacted due to their proximity to Pico Alto caldera (Figure 4). However, other densely populated areas on the eastern part of the island between Lajes and Praia da Vitória, and several villages on the south coast between São Mateus and Porto Judeu, including the city of Angra do Heroísmo could also be affected by channelled PDCs. In this scenario the western part of the island would not be affected and could possibly serve as a retreat area in case of evacuation of the affected villages. Moreover, the hazard would be especially compounded by the lack of an opening Plinian fallout phase that could give warning of impending PDCs and during which evacuations or other mitigation operations could be prepared.
Apart from dwellings, all critical infrastructure of the island might be expected to be damaged or destroyed. The geothermal power plant located on the southwest flank of Pico Alto caldera would most likely also be destroyed. All of the island’s communication hubs, including the only airport and NATO Air Base on the floor of Lajes Graben and the main harbour located on Praia da Vitória could suffer significant damage (see Figure 4 for location). This would make Terceira difficult to be reached by air or maritime transport, with major implications for evacuations and emergency response. The only hospital and the headquarters of the Azores Regional Civil Protection and Fire Services, both located in the city of Angra do Heroísmo (see Figure 4 for location), could also be severely affected.
In the light of this and despite the relatively low probability of such an event, this worst-case scenario should be considered by regional and national authorities in risk management plans. The potential risk is aggravated by the small size, remote location, high population density and weak economy of Terceira, as is commonly the case of volcanic islands (Pelling and Uitto, 2001; Wilkinson et al., 2016; Pimentel et al., 2020), such as demonstrated recently by the 2021 explosive eruption of La Soufrière volcano, St. Vincent (Lesser Antilles).
Conclusions
The Lajes-Angra Ignimbrite Formation (LAI) is the youngest (ca. 25 cal ka BP) of at least seven major formations containing peralkaline ignimbrites on Terceira Island (Azores). Most of the older ignimbrites share physical and geochemical similarities with the LAI, suggesting that the processes involved in ignimbrite-forming eruptions have been recurrent in the last ca. 100 kyr, with significant implications for volcanic hazard assessment. In this paper we combined lithofacies analysis and ignimbrite architecture with petrographic and geochemical data to provide insights into the pre-eruptive magmatic system of Pico Alto volcano and infer the eruption style and emplacement dynamics of the parent PDCs of the Angra and Lajes ignimbrites.
The two ignimbrites are difficult to distinguish based on whole rock major element chemistry alone, as both have comenditic trachyte compositions. However, whole rock trace element and groundmass glass compositions show considerable variability, which together with disequilibrium textures and compositions of anorthoclase crystals, reveal the occurrence of complex petrogenetic processes in the Pico Alto magma reservoir prior to eruption. Although the two ignimbrite-forming events were closely spaced in time (unresolvable by radiocarbon dating), their PDCs had dissimilar eruptive and depositional processes. The Angra Ignimbrite was formed by a small-volume sustained PDC that was fed by a short-lived, low pyroclastic fountain, which generated a high particle concentration, granular fluid-based PDC. This current seems to have been mostly channelled along a single valley on the south part of the island, forming a high-aspect ratio ignimbrite. On the other hand, the Lajes Ignimbrite resulted from a sustained quasi-steady PDC that was fed by vigorous and prolonged pyroclastic fountaining. Ignimbrite architecture suggests evolving depositional conditions of the parent PDC through time and space. The dilute front of the current rapidly evolved to a high particle concentration, granular fluid-based PDC as the eruption waxed. The PDC spread radially from Pico Alto caldera to both the north and south coasts with limited capacity to overcome topographic highs. The low-aspect ratio of the Lajes Ignimbrite most probably resulted from deposition of a relatively low velocity PDC over a generally flat topography, rather than from a highly energetic current. This placid style of emplacement allowed the PDC to conserve heat, which in turn, prompted welding in this thin ignimbrite sheet. We highlight that ignimbrite geometry (aspect ratio) does not directly reflect the kinetic energy of a PDC and therefore should not be used to infer on PDC emplacement dynamics.
A future ignimbrite-forming eruption of Pico Alto volcano would have devastating consequences for Terceira. In this worst-case scenario, the central part of the island and the villages located along the north and south coasts would be affected by PDCs, including all critical infrastructure and communication hubs. Despite the relatively low probability, such an event presents a considerable challenge for civil protection authorities. The findings of this study can provide a framework to better understand ignimbrite-forming eruptions on other active volcanic islands, as well as their potential impacts in case of future volcanic unrest.
Data Availability Statement
The original contributions presented in the study are included in the article/Supplementary Material, further inquiries can be directed to the corresponding author.
Author Contributions
AP was responsible for the formulation of this study as part of his PhD project, collection of field data, data analysis, and preparing the manuscript. SS took part in the fieldwork (including during the 1970s) and assisted with data analysis. JP contributed to the formulation of the study and data analysis. AJ and RG took part in fieldwork, compilation of geochemical data, provided figures, and assisted with data analysis. All authors contributed to writing and reviewing the paper.
Funding
This study was funded by a PhD scholarship of Fundo Regional da Ciência e Tecnologia to AP (M3.1.2/F/022/2007) and partially supported by Fundação para a Ciência e Tecnologia through MARES project (PTDC/GEO-FIQ/1088/2014). SS and RG thank The Open University, United Kingdom, for supporting field trips to Terceira in the period 2005–2010. Publication made possible in part by support from the Berkeley Research Impact Initiative (BRII) sponsored by the UC Berkeley Library.
Conflict of Interest
The authors declare that the research was conducted in the absence of any commercial or financial relationships that could be construed as a potential conflict of interest.
Acknowledgments
We thank Claudio Scarpati, Dario Pedrazzi and Chief Editor Valerio Acocella for their constructive comments that significantly improved this paper. We also thank Guest Associate Editor Joan Martí for the editorial handling. Vittorio Zanon of the Instituto de Investigação em Vulcanologia e Avaliação de Riscos (IVAR), Universidade dos Açores, Andrea Risplendente and Stefano Poli of the Dipartimento di Scienze della Terra “Ardito Desio”, Università degli Studi di Milano are acknowledged for the help with microprobe analyses.
Supplementary Material
The Supplementary Material for this article can be found online at: https://www.frontiersin.org/articles/10.3389/feart.2021.673686/full#supplementary-material
References
Andronico, D., and Pistolesi, M. (2010). The November 2009 Paroxysmal Explosions at Stromboli. J. Volcanology Geothermal Res. 196, 120–125. doi:10.1016/j.jvolgeores.2010.06.005
Báez, W., Bustos, E., Chiodi, A., Reckziegel, F., Arnosio, M., de Silva, S., et al. (2020a). Eruptive Style and Flow Dynamics of the Pyroclastic Density Currents Related to the Holocene Cerro Blanco Eruption (Southern Puna Plateau, Argentina). J. South Am. Earth Sci. 98, 102482. doi:10.1016/j.jsames.2019.102482
Báez, W., de Silva, S., Chiodi, A., Bustos, E., Giordano, G., Arnosio, M., et al. (2020b). Pulsating flow dynamics of sustained, forced pyroclastic density currents: insights from a facies analysis of the Campo de la Piedra Pómez ignimbrite, southern Puna, Argentina. Bull. Volcanol. 82, 53. doi:10.1007/s00445-020-01385-5
Bogaard, P. v. d., and Schmincke, H.-U. (1998). “Chronostratigraphy of Gran Canaria,” in Proc. ODP, Sci. Res. 157. Editors P.P.E. Weaver, H.-U. Schmincke, J.V. Firth, and W. Duffield (College Station, TX: Ocean Drilling Program), 127–140. doi:10.2973/odp.proc.sr.157.116.1998
Booth, B., Croasdale, R., and Walker, G. (1978). A Quantitative Study of Five Thousand Years of Volcanism on Sao Miguel, Azores. Phil. Trans. Roy. Soc. London, Ser. A, Math. Phys. Sci. 288, 271–319. doi:10.1098/rsta.1978.0018
Brand, B. D., Mackaman-Lofland, C., Pollock, N. M., Bendaña, S., Dawson, B., and Wichgers, P. (2014). Dynamics of Pyroclastic Density Currents: Conditions that Promote Substrate Erosion and Self-Channelization - Mount St Helens, Washington (USA). J. Volcanology Geothermal Res. 276, 189–214. doi:10.1016/j.jvolgeores.2014.01.007
Branney, M. J., and Kokelaar, P. (2002). Pyroclastic Density Currents and the Sedimentation of Ignimbrites. Geol. Soc. London, Mems 27, 152. doi:10.1144/GSL.MEM.2003.027
Brown, R. J., Barry, T. L., Branney, M. J., Pringle, M. S., and Bryan, S. E. (2003). The Quaternary Pyroclastic Succession of Southeast Tenerife, Canary Islands: Explosive Eruptions, Related Caldera Subsidence, and Sector Collapse. Geol. Mag. 140, 265–288. doi:10.1017/S0016756802007252
Brown, R. J., and Branney, M. J. (2004). Event-stratigraphy of a Caldera-Forming Ignimbrite Eruption on Tenerife: the 273 ka Poris Formation. Bull. Volcanol. 66, 392–416. doi:10.1007/s00445-003-0321-y
Brown, R. J., Kokelaar, B. P., and Branney, M. J. (2007). Widespread Transport of Pyroclastic Density Currents from a Large Silicic Tuff Ring: the Glaramara Tuff, Scafell Caldera, English Lake District, UK. Sedimentology 54, 1163–1190. doi:10.1111/j.1365-3091.2007.00877.x
Calvert, A. T., Moore, R. B., McGeehin, J. P., and Rodrigues da Silva, A. M. (2006). Volcanic History and 40Ar/39Ar and 14C Geochronology of Terceira Island, Azores, Portugal. J. Volcanology Geothermal Res. 156, 103–115. doi:10.1016/j.jvolgeores.2006.03.016
Cas, R. A. F., Wright, H. M. N., Folkes, C. B., Lesti, C., Porreca, M., Giordano, G., et al. (2011). The Flow Dynamics of an Extremely Large Volume Pyroclastic Flow, the 2.08-Ma Cerro Galán Ignimbrite, NW Argentina, and Comparison with Other Flow Types. Bull. Volcanol. 73, 1583–1609. doi:10.1007/s00445-011-0564-y
Cas, R. A. F., and Wright, J. V. (1987). Volcanic Successions. Modern and Ancient. London: Allen & Unwin, 528.
Casalbore, D., Romagnoli, C., Pimentel, A., Quartau, R., Casas, D., Ercilla, G., et al. (2015). Volcanic, Tectonic and Mass-Wasting Processes Offshore Terceira Island (Azores) Revealed by High-Resolution Seafloor Mapping. Bull. Volcanol. 77, 24. doi:10.1007/s00445-015-0905-3
Casas, D., Pimentel, A., Pacheco, J., Martorelli, E., Sposato, A., Ercilla, G., et al. (2018). Serreta 1998-2001 Submarine Volcanic Eruption, Offshore Terceira (Azores): Characterization of the Vent and Inferences about the Eruptive Dynamics. J. Volcanology Geothermal Res. 356, 127–140. doi:10.1016/j.jvolgeores.2018.02.017
Chiocci, F. L., Romagnoli, C., Casalbore, D., Sposato, A., Martorelli, E., Alonso, B., et al. (2013). Bathy-morphological Setting of Terceira Island (Azores) after the FAIVI Cruise. J. Maps 9, 590–595. doi:10.1080/17445647.2013.831381
D'Oriano, C., Landi, P., Pimentel, A., and Zanon, V. (2017). Magmatic Processes Revealed by Anorthoclase Textures and Trace Element Modeling: The Case of the Lajes Ignimbrite Eruption (Terceira Island, Azores). J. Volcanology Geothermal Res. 347, 44–63. doi:10.1016/j.jvolgeores.2017.08.012
Dade, W. B. (2003). The Emplacement of Low-Aspect Ratio Ignimbrites by Turbulent Parent Flows. J. Geophys. Res. 108, 2211–2220. doi:10.1029/2001JB001010
Dávila-Harris, P., Ellis, B. S., Branney, M. J., and Carrasco-Núñez, G. (2013). Lithostratigraphic Analysis and Geochemistry of a Vitric Spatter-Bearing Ignimbrite: the Quaternary Adeje Formation, Cañadas Volcano, Tenerife. Bull. Volcanol. 75, 722. doi:10.1007/s00445-013-0722-5
Druitt, T. H. (1998). “Pyroclastic Density Currents,”. The Physics of Explosive Volcanic Eruptions. Editors J.S. Gilbert, and R.S.J. Sparks (London, UK: Geol. Soc., London, Special Publications), 145, 145–182. doi:10.1144/GSL.SP.1996.145.01.08
Duncan, A. M., Queiroz, G., Guest, J. E., Cole, P. D., Wallenstein, N., and Pacheco, J. M. (1999). The Povoação Ignimbrite, Furnas Volcano, São Miguel, Azores. J. Volcanology Geothermal Res. 92, 55–65. doi:10.1016/S0377-0273(99)00067-0
Eichelberger, J. C., and Koch, F. G. (1979). Lithic Fragments in the Bandelier Tuff, Jemez Mountains, New Mexico. J. Volcanology Geothermal Res. 5, 115–134. doi:10.1016/0377-0273(79)90036-2
Eisele, S., Freundt, A., Kutterolf, S., Ramalho, R. S., Kwasnitschka, T., Wang, K.-L., et al. (2015). Stratigraphy of the Pleistocene, Phonolitic Cão Grande Formation on Santo Antão, Cape Verde. J. Volcanology Geothermal Res. 301, 204–220. doi:10.1016/j.jvolgeores.2015.03.012
Fedele, L., Scarpati, C., Sparice, D., Perrotta, A., and Laiena, F. (2016). A Chemostratigraphic Study of the Campanian Ignimbrite Eruption (Campi Flegrei, Italy): Insights on Magma Chamber Withdrawal and deposit Accumulation as Revealed by Compositionally Zoned Stratigraphic and Facies Framework. J. Volcanology Geothermal Res. 324, 105–117. doi:10.1016/j.jvolgeores.2016.05.019
Féraud, G., Kaneoka, I., and Allègre, C. (1980). K/Ar Ages and Stress Pattern in the Azores: Geodynamic Implications. Earth Planet. Sci. Lett. 46, 275–286. doi:10.1016/0012-821X(80)90013-8
Freundt, A., and Schmincke, H.-U. (1986). Emplacement of Small-Volume Pyroclastic Flows at Laacher See (East-Eifel, Germany). Bull. Volcanol. 48, 39–59. doi:10.1007/BF01073512
Gaspar, J. L. (1996). Ilha Graciosa (Açores). História vulcanológica e avaliação do hazard. PhD thesis, Ponta Delgada: Universidade dos Açores, 361.
Gaspar, J. L., Queiroz, G., Pacheco, J. M., Ferreira, T., Wallenstein, N., Almeida, M. H., et al. (2003). “Basaltic Lava Balloons Produced during the 1998-2001 Serreta Submarine Ridge Eruption (Azores), Explosive Subaqueous Volcanism. Editors J. D. L. White, J. L. Smellie, and D. A. Clague (Washington DC, USA: AGU Geophysical Monograph), 140, 205–212. doi:10.1029/140gm13
Gertisser, R., Self, S., Gaspar, J. L., Kelley, S. P., Pimentel, A., Eikenberg, J., et al. (2010). “Ignimbrite Stratigraphy and Chronology on Terceira Island, Azores,”Stratigraphy and Geology of Volcanic Areas. Editors G. Groppelli, and L. Viereck-Goette (Boulder, USA: Geol. Soc. Amer., Spec. Pap.), 464, 133–154. doi:10.1130/2010.2464(07)
Giordano, G., De Rita, D., Cas, R., and Rodani, S. (2002). Valley Pond and Ignimbrite Veneer Deposits in the Small-Volume Phreatomagmatic 'Peperino Albano' Basic Ignimbrite, Lago Albano Maar, Colli Albani Volcano, Italy: Influence of Topography. J. Volcanology Geothermal Res. 118, 131–144. doi:10.1016/S0377-0273(02)00253-6
Giordano, G., and Doronzo, D. M. (2017). Sedimentation and Mobility of PDCs: a Reappraisal of Ignimbrites' Aspect Ratio. Sci. Rep. 7, 4444. doi:10.1038/s41598-017-04880-6
Giordano, G. (1998). The Effect of Paleotopography on Lithic Distribution and Facies Associations of Small Volume Ignimbrites: the WTT Cupa (Roccamonfina Volcano, Italy). J. Volcanology Geothermal Res. 87, 255–273. doi:10.1016/S0377-0273(98)00096-1
Hildenbrand, A., Weis, D., Madureira, P., and Marques, F. O. (2014). Recent Plate Re-organization at the Azores Triple Junction: Evidence from Combined Geochemical and Geochronological Data on Faial, S. Jorge and Terceira Volcanic Islands. Lithos 210-211, 27–39. doi:10.1016/j.Lithos.2014.09.009
Hildreth, W., and Fierstein, J. (2012). The Novarupta-Katmai Eruption of 1912 - Largest Eruption of the Twentieth century: Centennial Perspectives. U.S. Geol. Surv. Prof. Pap. 1791, 259.
Jeffery, A. J., Gertisser, R., O’Driscoll, B., Pacheco, J. M., Whitley, S., Pimentel, A., et al. (2016). Temporal Evolution of a post-caldera, Mildly Peralkaline Magmatic System: Furnas Volcano, São Miguel, Azores. Contrib. Mineral. Petrol. 171, 42. doi:10.1007/s00410-016-1235-y
Jeffery, A. J., and Gertisser, R. (2018). Peralkaline Felsic Magmatism of the Atlantic Islands. Front. Earth Sci. 6, 145. doi:10.3389/feart.2018.00145
Jeffery, A. J., Gertisser, R., Self, S., Pimentel, A., O’Driscoll, B., and Pacheco, J. M. (2017). Petrogenesis of the Peralkaline Ignimbrites of Terceira, Azores. J. Pet. 58, 2365–2402. doi:10.1093/petrology/egy012
Jørgensen, K. A. (1980). The Thorsmörk Ignimbrite: An Unusual Comenditic Pyroclastic Flow in Southern Iceland. J. Volcanol. Geotherm. Res. 8, 7–22. doi:10.1016/0377-0273(80)90004-9
Jutzeler, M., Schmincke, H.-U., and Sumita, M. (2010). The Incrementally Zoned Miocene Ayagaures Ignimbrite (Gran Canaria, Canary Islands). J. Volcanology Geothermal Res. 196, 1–19. doi:10.1016/j.jvolgeores.2010.07.002
Kneller, B. C., and Branney, M. J. (1995). Sustained High-Density Turbidity Currents and the Deposition of Thick Massive Sands. Sedimentology 42, 607–616. doi:10.1111/j.1365-3091.1995.tb00395.x
Kokelaar, P., Raine, P., and Branney, M. J. (2007). Incursion of a Large-Volume, Spatter-Bearing Pyroclastic Density Current into a Caldera lake: Pavey Ark Ignimbrite, Scafell Caldera, England. Bull. Volcanol. 70, 23–54. doi:10.1007/s00445-007-0118-5
Kueppers, U., Pimentel, A., Ellis, B., Forni, F., Neukampf, J., Pacheco, J., et al. (2019). Biased Volcanic Hazard Assessment Due to Incomplete Eruption Records on Ocean Islands: An Example of Sete Cidades Volcano, Azores. Front. Earth Sci. 7, 122. doi:10.3389/feart.2019.00122
Lipman, P. W. (1997). Subsidence of Ash-Flow Calderas: Relation to Caldera Size and Magma-Chamber Geometry. Bull. Volcanology 59, 198–218. doi:10.1007/s004450050186
Macdonald, R. (1974). Nomenclature and Petrochemistry of the Peralkaline Oversaturated Extrusive Rocks. Bull. Volcanol. 38, 498–516. doi:10.1007/BF02596896
Madeira, J. (2005). The Volcanoes of Azores Islands: A World-Class Heritage. Examples from Terceira, Pico and Faial Islands. IV International Symposium ProGEO on the Conservation of the Geological Heritage - Field Trip Guidebook. Braga: Universidade do Minho, 104.
Martí, J., Diez-Gil, J. L., and Ortiz, R. (1991). Conduction Model for the Thermal Influence of Lithic Clasts in Mixtures of Hot Gases and Ejecta. J. Geophys. Res. 96 (B13), 21879–21885. doi:10.1029/91JB02149
Martí, J., Doronzo, D. M., Pedrazzi, D., and Colombo, F. (2019). Topographical Controls on Small‐volume Pyroclastic Flows. Sedimentology 66, 2297–2317. doi:10.1111/sed.12600
Medeiros, J., Carmo, R., Pimentel, A., Vieira, J. C., and Queiroz, G. (2021). Assessing the Impact of Explosive Eruptions of Fogo Volcano (São Miguel, Azores) on the Tourism Economy. Nat. Hazards Earth Syst. Sci. 21, 417–437. doi:10.5194/nhess-21-439-202110.5194/nhess-21-417-2021
Mungall, J. E., and Martin, R. F. (1995). Petrogenesis of Basalt-comendite and Basalt-pantellerite Suites, Terceira, Azores, and Some Implications for the Origin of Ocean-Island Rhyolites. Contr. Mineral. Petrol. 119, 43–55. doi:10.1007/BF00310716
Pacheco-Hoyos, J. G., Aguirre-Díaz, G. J., and Dávila-Harris, P. (2018). Boiling-over Dense Pyroclastic Density Currents during the Formation of the ∼ 100 km3 Huichapan Ignimbrite in Central Mexico: Stratigraphic and Lithofacies Analysis. J. Volcanology Geothermal Res. 349, 268–282. doi:10.1016/j.jvolgeores.2017.11.007
Pelling, M., and Uitto, J. I. (2001). Small Island Developing States: Natural Disaster Vulnerability and Global Change. Glob. Environ. Change B: Environ. Hazards 3, 4962. doi:10.1016/S1464-2867(01)00018-3
Pensa, A., Cas, R., Giordano, G., Porreca, M., and Wallenstein, N. (2015). Transition from Steady to Unsteady Plinian Eruption Column: the VEI 5, 4.6 ka Fogo A Plinian Eruption, São Miguel, Azores. J. Volcanology Geothermal Res. 305, 1–18. doi:10.1016/j.jvolgeores.2015.09.012
Pimentel, A. (2006). Domos e coulées da Ilha Terceira (Açores): Contribuição para o estudo dos mecanismos de instalação. Ponta Delgada: MSc thesis, Universidade dos Açores, 143.
Pimentel, A., Pacheco, J., and Self, S. (2015). The ∼1000-years BP Explosive Eruption of Caldeira Volcano (Faial, Azores): the First Stage of Incremental Caldera Formation. Bull. Volcanol. 77, 42. doi:10.1007/s00445-015-0930-2
Pimentel, A., Ramalho, R. S., Becerril, L., Larrea, P., and Brown, R. J. (2020). Editorial: Ocean Island Volcanoes: Genesis, Evolution and Impact. Front. Earth Sci. 8, 82. doi:10.3389/feart.2020.00082
Pimentel, A., Zanon, V., de Groot, L. V., Hipólito, A., Di Chiara, A., and Self, S. (2016). Stress-induced Comenditic Trachyte Effusion Triggered by Trachybasalt Intrusion: Multidisciplinary Study of the AD 1761 Eruption at Terceira Island (Azores). Bull. Volcanol. 78, 22. doi:10.1007/s00445-016-1015-6
PORDATA (2020). Retrato Dos Açores PORDATA, Edição 2020. Lisboa: Fundação Francisco Manuel dos Santos, 76.
Porreca, M., Pimentel, A., Kueppers, U., Izquierdo, T., Pacheco, J., and Queiroz, G. (2018). Event Stratigraphy and Emplacement Mechanisms of the Last Major Caldera Eruption on Sete Cidades Volcano (São Miguel, Azores): the 16 ka Santa Bárbara Formation. Bull. Volcanol. 80, 76. doi:10.1007/s00445-018-1250-0
Queiroz, G., Gaspar, J. L., Guest, J. E., Gomes, A., and Almeida, M. H. (2015). “Eruptive History and Evolution of Sete Cidades Volcano, São Miguel Island, Azores,”. Volcanic Geology of São Miguel Island (Azores Archipelago). Editors J. L. Gaspar, J. E. Guest, A. M. Duncan, F. J. A. S. Barriga, and D. K. Chester (London, Mems: Geol. Soc.), 44, 87–104. doi:10.1144/M44.7
Reimer, P. J., Austin, W. E. N., Bard, E., Bayliss, A., Blackwell, P. G., Bronk Ramsey, C., et al. (2020). The IntCal20 Northern Hemisphere Radiocarbon Age Calibration Curve (0-55 cal kBP). Radiocarbon 62, 725–757. doi:10.1017/RDC.2020.41
Le Maitre, R., Streckeisen, A., Zanettin, B., Le Bas, M., Bonin, B., and Bateman, P. (2002). Igneous Rocks: A Classification and Glossary of Terms: Recommendations of the International Union of Geological Sciences Subcommission on the Systematics of Igneous Rocks. 2nd Ed. (Cambridge University Press. Cambridge, United Kingdom), doi:10.1017/CBO9780511535581
Russell, J. K., and Quane, S. L. (2005). Rheology of Welding: Inversion of Field Constraints. J. Volcanology Geothermal Res. 142, 173–191. doi:10.1016/j.jvolgeores.2004.10.017
Scarpati, C., Sparice, D., and Perrotta, A. (2020). Dynamics of Large Pyroclastic Currents Inferred by the Internal Architecture of the Campanian Ignimbrite. Sci. Rep. 10, 22230. doi:10.1038/s41598-020-79164-7
Scarpati, C., Sparice, D., and Perrotta, A. (2015). The Ground Layer of the Campanian Ignimbrite: an Example of Deposition from a Dilute Pyroclastic Density Current. Bull. Volcanol. 77, 97. doi:10.1007/s00445-015-0985-0
Schumacher, R., and Mues-Schumacher, U. (1996). The Kizilkaya Ignimbrite - an Unusual Low-Aspect-Ratio Ignimbrite from Cappadocia, central Turkey. J. Volcanology Geothermal Res. 70, 107–121. doi:10.1016/0377-0273(95)00046-1
Scott, W. E., Hoblitt, R. P., Torres, R. C., Self, S., Martinez, M. M. L., and Nillos, T. (1996). “Pyroclastic Flows of the June 15, 1991, Climactic Eruption of Mount Pinatubo,” in Fire and Mud: Eruptions and Lahars of Mount Pinatubo, Philippines. Editors C. G. Newhall, and R. S. Punongbayan (Seattle: PHIVOLCS, Quezon City and University of Washington Press), 545–570.
Self, S., and Gunn, B. M. (1976). Petrology, Volume and Age Relations of Alkaline and Saturated Peralkaline Volcanics from Terceira, Azores. Contrib. Mineral. Petrol. 54, 293–313. doi:10.1007/BF00389409
Self, S. (1971). The Lajes Ignimbrite, Ilha Terceira, Açores. Comun. Serv. Geól. Portugal 55, 165–184.
Self, S. (1976). The Recent Volcanology of Terceira, Azores. J. Geol. Soc. 132, 645–666. doi:10.1144/gsjgs.132.6.0645
Shea, T., and Owen, J. (2016). Discovery of a Trachyte Ignimbrite Sequence at Hualālai, Hawaii. Bull. Volcanol 78, 34. doi:10.1007/s00445-016-1027-2
Shotton, F. W., and Williams, R. E. G. (1973). Birmingham University Radiocarbon Dates VII. Radiocarbon 15, 451–468. doi:10.1017/S0033822200008924
Shotton, F. W., Williams, R. E. G., and Johnson, A. S. (1974). Birmingham University Radiocarbon Dates VIII. Radiocarbon 16, 285–303. doi:10.1017/S0033822200059610
Sohn, Y. K., and Chough, S. K. (1989). Depositional Processes of the Suwolbong Tuff Ring, Cheju Island (Korea). Sedimentology 36, 837–855. doi:10.1111/j.1365-3091.1989.tb01749.x
Sparks, R. S. J. (1976). Grain Size Variations in Ignimbrites and Implications for the Transport of Pyroclastic Flows. Sedimentology 23, 147–188. doi:10.1111/j.1365-3091.1976.tb00045.x
Sparks, R. S. J., Self, S., and Walker, G. P. L. (1973). Products of Ignimbrite Eruptions. Geology 1, 115–118. doi:10.1130/0091-7613(1973)1<115:POIE>2.0.CO;2
Sulpizio, R., Bonasia, R., Dellino, P., Mele, D., Di Vito, M. A., and La Volpe, L. (2010). The Pomici di Avellino eruption of Somma-Vesuvius (3.9 ka BP). Part II: sedimentology and physical volcanology of pyroclastic density current deposits. Bull. Volcanol. 72, 559–577. doi:10.1007/s00445-009-0340-4
Sulpizio, R., and Dellino, P. (2008). “Chapter 2 Sedimentology, Depositional Mechanisms and Pulsating Behaviour of Pyroclastic Density Currents,” in Caldera Volcanism: Analysis, Modelling, and Response. Developments in Volcanology 10. Editors J. Gottsman, and J. Marti (Amsterdam: Elsevier), 57–96. doi:10.1016/s1871-644x(07)00002-2
Sulpizio, R., Mele, D., Dellino, P., and La Volpe, L. (2007). Deposits and Physical Properties of Pyroclastic Density Currents during Complex Subplinian Eruptions: the AD 472 (Pollena) Eruption of Somma-Vesuvius, Italy. Sedimentology 54, 607–635. doi:10.1111/j.1365-3091.2006.00852.x
Sumner, J. M., and Branney, M. J. (2002). The Emplacement History of a Remarkable Heterogeneous, Chemically Zoned, Rheomorphic and Locally Lava-like Ignimbrite: 'TL' on Gran Canaria. J. Volcanology Geothermal Res. 115, 109–138. doi:10.1016/S0377-0273(01)00311-0
Sun, S.-s., and McDonough, W. F. (1989). “Chemical and Isotopic Systematics of Oceanic Basalts: Implications for Mantle Composition and Processes,”. Magmatism in the Ocean Basins. Editors A. D. Saunders, and M. J. Norry (London: Geol. Society.), 42, 313–345. doi:10.1144/gsl.sp.1989.042.01.19
Tomlinson, E. L., Smith, V. C., Albert, P. G., Aydar, E., Civetta, L., Cioni, R., et al. (2015). The Major and Trace Element Glass Compositions of the Productive Mediterranean Volcanic Sources: Tools for Correlating Distal Tephra Layers in and Around Europe. Quat. Sci. Rev. 118, 48–66. doi:10.1016/j.quascirev.2014.10.028
Trofimovs, J., Amy, L., Boudon, G., Deplus, C., Doyle, E., Fournier, N., et al. (2006). Submarine Pyroclastic Deposits Formed at the Soufrière Hills Volcano, Montserrat (1995-2003): What Happens when Pyroclastic Flows Enter the Ocean? Geol. 34, 549–552. doi:10.1130/G22424.1
Troll, V. R., and Schmincke, H.-U. (2002). Magma Mixing and Crustal Recycling Recorded in Ternary Feldspar from Compositionally Zoned Peralkaline Ignimbrite A', Gran Canaria, Canary Islands. J. Pet. 43, 243–270. doi:10.1093/petrology/43.2.243
Walker, G. P. L., Heming, R. F., and Wilson, C. J. N. (1980). Low-aspect Ratio Ignimbrites. Nature 283, 286–287. doi:10.1038/283286a0
Walker, G. P. L. (1983). Ignimbrite Types and Ignimbrite Problems. J. Volcanology Geothermal Res. 17, 65–88. doi:10.1016/0377-0273(83)90062-8
Walker, G. P. L., Self, S., and Froggatt, P. C. (1981). The Ground Layer of the Taupo Ignimbrite: a Striking Example of Sedimentation from a Pyroclastic Flow. J. Volcanology Geothermal Res. 10, 1–11. doi:10.1016/0377-0273(81)90051-2
Weston, F. S. (1964). List of Recorded Volcanic Eruptions in the Azores with Brief Reports. Boletim do Museu e Laboratório Mineralógico e Geológico da Faculdade de Ciências de Lisboa 10, 3–18.
Wilkinson, E., Lovell, E., Carby, B., Barclay, J., and Robertson, R. (2016). The Dilemmas of Risk-Sensitive Development on a Small Volcanic Island. Resources 5, 21. doi:10.3390/resources5020021
Willcock, M. A. W., Cas, R. A. F., Giordano, G., and Morelli, C. (2013). The Eruption, Pyroclastic Flow Behaviour, and Caldera In-Filling Processes of the Extremely Large Volume (>1290 km3), Intra- to Extra-caldera, Permian Ora (Ignimbrite) Formation, Southern Alps, Italy. J. Volcanology Geothermal Res. 265, 102–126. doi:10.1016/j.jvolgeores.2013.08.012
Williams, R., Branney, M. J., and Barry, T. L. (2014). Temporal and Spatial Evolution of a Waxing Then Waning Catastrophic Density Current Revealed by Chemical Mapping. Geology 42, 107–110. doi:10.1130/G34830.1
Williams, R. (2010). Emplacement of Radial Pyroclastic Density Currents over Irregular Topography: The Chemically Zoned, Low Aspect-Ratio Green Tuff Ignimbrite, Pantelleria, Italy. PhD Thesis. Leicester: University of Leicester, 224.
Wilson, C. (1991). Ignimbrite Morphology and the Effects of Erosion: a New Zealand Case Study. Bull. Volcanol. 53, 635–644. doi:10.1007/BF00493690
Wilson, C. J. N., and Hildreth, W. (1997). The Bishop Tuff: New Insights from Eruptive Stratigraphy. J. Geology 105, 407–440. doi:10.1086/515937
Wilson, C. J. N., Houghton, B. F., Kampt, P. J. J., and McWilliamst, M. O. (1995). An Exceptionally Widespread Ignimbrite with Implications for Pyroclastic Flow Emplacement. Nature 378, 605–607. doi:10.1038/378605a0
Wilson, C. J. N. (1985). The Taupo Eruption, New Zealand II. The Taupo Ignimbrite. Phil. Trans. Roy. Soc. A314, 229–310. doi:10.1098/rsta.1985.0020
Wilson, C. J. N., and Walker, G. P. L. (1982). Ignimbrite Depositional Facies: the Anatomy of a Pyroclastic Flow. J. Geol. Soc. 139, 581–592. doi:10.1144/gsjgs.139.5.0581
Keywords: explosive volcanism, oceanic island, pyroclastic fountaining, pyroclastic density current, ignimbrite aspect ratio, comenditic trachyte, volcanic hazard
Citation: Pimentel A, Self S, Pacheco JM, Jeffery AJ and Gertisser R (2021) Eruption Style, Emplacement Dynamics and Geometry of Peralkaline Ignimbrites: Insights From the Lajes-Angra Ignimbrite Formation, Terceira Island, Azores. Front. Earth Sci. 9:673686. doi: 10.3389/feart.2021.673686
Received: 28 February 2021; Accepted: 07 June 2021;
Published: 25 June 2021.
Edited by:
Joan Marti, Consejo Superior de Investigaciones Científicas (CSIC), SpainReviewed by:
Claudio Scarpati, University of Naples Federico II, ItalyDario Pedrazzi, Instituto de Ciencias de la Tierra Jaume Almera (ICTJA), Spain
Copyright © 2021 Pimentel, Self, Pacheco, Jeffery and Gertisser. This is an open-access article distributed under the terms of the Creative Commons Attribution License (CC BY). The use, distribution or reproduction in other forums is permitted, provided the original author(s) and the copyright owner(s) are credited and that the original publication in this journal is cited, in accordance with accepted academic practice. No use, distribution or reproduction is permitted which does not comply with these terms.
*Correspondence: Adriano Pimentel, adriano.hg.pimentel@azores.gov.pt